ABSTRACT
A synthesis of two series of 3-substituted quinazolinones was performed utilizing a green chemistry approach, deep eutectic solvents and microwaves, namely. 2-Methyl-3-substituted-quinazolin-4(3H)-one derivatives were synthesized in a two-step reaction, using choline chloride:urea deep eutectic solvent (DES). 3-Substituted-quinazolin-4(3H)-ones were synthesized in one-pot one-step reaction of anthranilic acid, amines and orthoester in a microwave reactor. For the synthesis of 2-methyl-3-substituted-quinazolin-4(3H)-ones, first conventional synthesis of benzoxazinone, as an intermediate, was performed. Further, benzoxazinone in reaction with corresponding amines, in choline choline:urea deep eutectic solvent, furnished desired compounds. These procedures are based on green principles with the aim of developing synthetic routes for the potential antitumor agents. All compounds were characterized by LC/MS, 1H NMR and 13C NMR spectral techniques. Compound 1 bearing trifluoromethoxyphenyl group showed promising activity against HuT-78 cell line with IC50 of 51.4 ± 5.1 µM.
GRAPHICAL ABSTRACT

Introduction
Quinazolinones are a group of heterocyclic compounds exhibiting a range of significant biological and pharmacological activities. Their structural diversity greatly affects their biological activity and they are proven to possess antitubercular ( Citation1, Citation2), antibacterial ( Citation3, Citation4), antitumor ( Citation5, Citation6), anticonvulsant ( Citation7, Citation8), anti-inflammatory ( Citation9, Citation10) and antiviral ( Citation11) activities ().
Figure 1. The quinazolinone structures core ( Citation12).
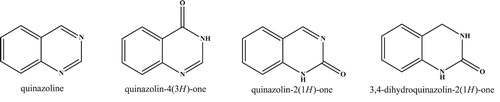
Their application in medicine includes various quinazolinone derivatives, such as a chemotherapy drug Raltitrexed (Tomudex®) used to treat bowel cancer, Afatinib, used for the treatment of metastatic non-small cell lung cancer (NSCLC), Alfuzosin, which is an alpha-adrenergic blocker for benign prostatic hyperplasia treatment, Dacomitinib, used in the treatment of metastatic non-small cell lung cancer ().
So far, many synthetic methods have been introduced in the synthesis of quinazolin-4(3H)-ones, conventional ones and green synthetic methods as well. Quinoazlin-4(3H)-ones were synthesized in Niementowski reaction from anthranilic acid and formamide (or other amides) ( Citation13), as well as in combination with formic acid and different amines ( Citation14). 2-Methyl-quinazolin-4(3H)-ones are usually synthesized from anthranilic acid, which is cyclized to benzoxazinone using acetic anhydride, and subsequently in reaction with different amines, a desired quinazolinone is formed ( Citation15). Furthermore, a cyclization of anthranilic acid to quinazolin-4(3H)-ones can be performed in a reaction of anthranilic acid, methyl or ethyl orthoformate and different amines ( Citation16–18). All these reaction utilize different volatile organic solvents or catalysts, which should be avoided to decrease the negative impact on the environment. Therefore, green synthetic methods are favorable in this manner. So far, some green techniques have been applied for their synthesis, like microwaves ( Citation19–21), ultrasound ( Citation22–24), grinding methods ( Citation25, Citation26) or ionic liquids ( Citation11, Citation24, Citation27). As one of the green synthetic methods, utilization of deep eutectic solvents in the synthesis of different heterocycles is getting more attention in the last few years, but only few papers describe their application in quinazolinone synthesis. Green one-pot synthesis of quinazolinones and 2-mercapto-quinazolinones was performed in deep eutectic solvents by Ghosh et al. ( Citation28) and Molnar et al. ( Citation29), while Lobo et al. ( Citation30) used different choline chloride-based deep eutectic solvents in catalytic amounts for dihyroquinazolinone synthesis. A one-pot three-component synthesis of quinazoline derivatives was performed from 2-aminoaryl ketones, aldehyde, and ammonium acetate in maltose:dimethylurea DES ( Citation31).
A new generation of solvents, deep eutectic solvents (DESs) are solvents formed by eutectic mixture of two or more components, usually a hydrogen bond donor (HBD) and a quaternary ammonium salt as a hydrogen bong acceptor (HBA). Due to their specific and tuneable physicochemical properties, they can be used as both a reaction media as well as catalysts. Their main advantages compared to other organic solvents or ionic liquids are their biocompatibility, non-toxicity, cheapness and simple preparation. Components, which are mixed together, form an intermolecular net of hydrogen bonds resulting in a lower melting point of the mixture compared to the melting points of each individual component. Urea, dicarboxylic acids, sugars and different polyols are the most common hydrogen bond donors ( Citation32, Citation33).
In this research, we examined the application of deep eutectic solvents and microwaves in the synthesis of various quinazolinone derivatives. Newly synthesized compounds were tested for their antitumor activity to find the most efficient structural motif that could serve as the basis for further development, considering the fact that small modifications to chemical structure can lead to the improved biological activity.
Experimental
All chemicals used in the synthesis of desired compounds were purchased from commercial suppliers. Microwave synthesis was performed in Milestone flexiWAVE (Milestone Srl, Sorisole (BG), Italy) microwave system, equipped with rotating carousel with 15 positions for PTFE high-pressure vessels. Mass analysis was performed on Applied BioSystems/MDS SCIEX, API 2000, triple quadrupole (CA, USA). NMR spectra were recorded on Bruker Avance 600 MHz NMR Spectrometer (Bruker Biospin GmbH, Rheinstetten, Germany) at 293 K with DMSO-d6 as a solvent and tetramethylsilane (TMS) as an internal standard.
Synthesis of quinazolin-4(3H)-ones 1–4 under microwaves
Anthranilic acid (5 mmol), trimethyl orthoformate (6 mmol) and amine (6 mmol) were mixed together in EtOH (10 mL) and subjected to microwaves at 120°C for 30 min. The mixture was poured over crushed ice and a crude product was separated by filtration. Compounds were recrystallized from ethanol.
3-(4-(trifluoromethoxy)phenyl)quinazolin-4(3H)-one (1)
Yield: 48%; Mp = 138–139°C; Rf = 0.76; 1H-NMR (600 MHz, ppm, DMSO-d6): 7.56–7.60 (m, 3H, arom.), 7.70–7.74 (m, 3H, arom.), 7.86–7.89 (m, 1H, arom.), 8.19 (m, 1H, arom.), 8.36 (s, 1H, C-2).; 13C-NMR (150 MHz, DMSO-d6) δ (ppm): 159.9, 147.7, 136.5, 134.7, 129.7, 127.5, 127.3, 126.4, 121.8; MS: m/z: 307.10 (M+) (306.24).
3-(2,5-dimethoxyphenyl)quinazolin-4(3H)-one (2)
Yield: 54%; Mp = 159–161°C; Rf = 0.71; 1H-NMR (300 MHz, ppm, DMSO-d6): 3.72 (s, 3H, OCH3), 3.76 (s, 3H, OCH3), 7.11 (m, 1H, arom.), 7.15–7.21 (m,2H, arom.), 7.60 (t, J = 6.97 Hz, 1H, arom.), 7.76 (d, J = 8.29 Hz, 1H, arom.), 7.89 (m, 1H, =CH), 8.20 (s, 1H, C-2); 13C-NMR (150 MHz, DMSO-d6) δ (ppm): 160.2, 149.1, 148.4, 135.1, 127.8, 126.8, 122.5, 116.0, 115.8, 113.9, 56.7, 56.2; MS: m/z: 282.90 (M+) (282.29).
3-phenylquinazolin-4(3H)-one (3)
Yield: 12%; Mp = 143–145°C (lit. 139°C ( Citation34)); Rf = 0.73; 1H-NMR (300 MHz, ppm, DMSO-d6): 7.55–7.63 (m, 6H, arom.), 7.75 (m, 1H, arom.), 7.86 (dd, J = 6.97, 1.3 Hz, 1H, arom.), 8.21 (dd, J = 8.10, 0.9 Hz,1H, arom.), 8.36 (s, 1H, C-2).; 13C-NMR (150 MHz, DMSO-d6) δ (ppm): 160.44, 148.2, 147., 138.1, 135.1, 129.7, 129.2, 127.9, 127.8, 127.7, 126.9, 122.43; MS: m/z: 221.10 (M−) (222.24).
2-(4-oxoquinazolin-3(4H)-yl)benzoic Acid (4)
Anthranilic acid (7 mmol) and trimethyl orthoformate (3.5 mmol) were mixed together in EtOH (10 mL) and subjected to microwaves at 120°C for 30 min. The mixture was poured over crushed ice and a crude product was separated by filtration. Compound was recrystallized from ethanol.
Yield: 35%; Mp = 295°C (lit. 287–288°C ( Citation35)); Rf = 0.48; 1H-NMR (600 MHz, ppm, DMSO-d6): 7.68–7.72 (m, 2H, arom.), 7.77 (m, 1H, arom.), 7.84 (m, 1H, arom.), 7.88–7.92 (m, 1H, arom.), 7.98 (m, 1H, arom.), 8.18 (dd, J = 8.07, 1.4 Hz,1H, arom.), 8.27 (dd, J = 8.07, 1.4 Hz,1H, arom.), 8.42 (s, 1H, C-2), 13.18 (br.s., 1H, OH); 13C-NMR (150 MHz, DMSO-d6) δ (ppm): 165.8, 160.3, 147.9, 147.1, 137.1, 134.6, 133.4, 131.1, 129.5, 129.2, 127.2, 126.3, 121.9; MS: m/z: 267.00 (M+) (266.25).
Synthesis of 2-methyl-quinazolin-4(3H)-ones 5–10 in deep eutectic solvents
Synthesis of deep eutectic solvents. Choline chloride (0.05 mol) and urea (0.1 mol) were mixed together and heated at 90°C until a clear liquid was formed. DES was cooled to room temperature and used for the reactions without further purification.
Compounds 5, 6, 7. Anthranilic acid (5 mmol), acetic anhydride (AAA, 6 mmol) and amine (6 mmol) were added to the synthesized DES and stirred under heating at 80°C until the completion of reaction, monitored by TLC (benzene:acetone:acetic acid 8:1:1). Water was added to the mixture and a crude product filtered and dried. Compounds were recrystallized from ethanol.
Compounds 8, 9, 10. 2-Methyl-4H-benzoxazin-4-one was synthesized conventionally from anthranilic acid (25 mmol) and acetic anhydride (50 mmol) under reflux for 4 h. Acetic anhydride and acetic acid, formed in the reaction, were evaporated under reduced pressure and the crude benzoxazinone was obtained.
Benzoxazinone and corresponding amine were added to the DES containing molecular sieves and stirred under heating at 80°C. Reaction was TLC (benzene:acetone:acetic acid 8:1:1) monitored and when completed desired products were precipitated with water. Compounds were recrystallized from ethanol.
3-(4-methoxyphenyl)-2-methylquinazolin-4(3H)-one (5)
Yield: 71%; Mp = 170–173°C (lit.167–170 ( Citation36)) Rf = 0.62; 1H-NMR (300 MHz, ppm, DMSO-d6): 2.15 (s, 3H, CH3), 3.85 (s, 3H, OCH3), 7.01–7.12 (m, 2H, arom.), 7.34–7.37 (m, 2H, arom.), 7.51 (m, 1H, arom.), 7.66 (m, 1H, arom.), 7.84 (m, 1H, arom.), 8.09 (dd, 1H, J = 8.10, 1.32, arom); 13C-NMR (150 MHz, DMSO-d6) δ (ppm): 162.0, 159.7, 155.5, 147.9, 134.9, 130.8, 129.9, 127.1, 126.8, 115.2, 55.9, 24.5; MS: m/z: 267.10 (M+) (266.29).
3-(4-chlorophenyl)-2-methylquinazolin-4(3H)-one (6)
Yield: 66%; Mp = 159–161°C (lit. 159–160°C ( Citation36)); Rf = 0.70; 1H-NMR (300 MHz, ppm, DMSO-d6): 2.15 (s, 3H, CH3), 7.51–7.54 (m, 3H, arom.), 7.64–7.69 (m, 3H, arom.), 7.85 (m, 1H, arom.), 8.11 (dd, 1H, J = 7.91, 1.13, arom); 13C-NMR (150 MHz, DMSO-d6) δ (ppm): 161.82, 154.7, 147.9, 137.2, 135.1, 134.1, 130.9, 130.1, 127.1, 126.9, 126.7, 120.9, 24.5; MS: m/z: 271.20 (M+) (270.71).
3-(4-methoxyphenyl)-2-methyl-6-nitroquinazolin-4(3H)-one (7)
Yield: 84%; Mp = 229–230°C; Rf = 0.70; 1H-NMR (300 MHz, ppm, DMSO-d6): 2.20 (s, 3H, CH3), 3.85 (s, 3H, OCH3), 7.11–7.14 (d, 2H, J = 9.04, arom.), 7.40–7.43 (d, 2H, J = 8.67, arom.), 7.83 (d, 1H, J = 9.04, arom.), 8.55 (dd, 1H, J = 9.04, 2.64, arom.), 8.76 (s, 1H, arom.); 13C-NMR (150 MHz, DMSO-d6) δ (ppm): 161.3, 160.0, 159.7, 152.0, 145.1, 130.2, 129.9, 128.9, 122.9, 121.1, 115.3, 55.9, 24.9; MS: m/z: 312.20 (M+) (311.29).
2-(7-hydroxy-2-oxo-2H-chromen-4-yl)-N-(2-methyl-4-oxoquinazolin-3(4H)-yl)acetamide (8)
Yield: 79%; Mp = 200–204°C; Rf = 0.30; 1H-NMR (300 MHz, ppm, DMSO-d6): 2.36 (s, 3H, CH3), 4.02 (s, 2H, -CH2-), 6.39 (s, 1H, coum.), 6.75 (s, 1H, arom.), 6.83 (m, 1H, arom.), 7.53 (m, 1H, arom.), 7.63 (d, 1H, J = 7.91, arom.), 7.73 (d, 1H, J = 8.67, arom.), 7.85 (m, 1H, arom.), 8.10 (dd, 1H, J = 7.91, 1.13, arom); 13C-NMR (150 MHz, DMSO-d6) δ (ppm): 168.4, 161.8, 160.6, 159.3, 156.3, 155.5, 150.1, 147.0, 135.5, 120.9, 113.4, 112.8, 111.7, 102.9, 37.1, 21.5; MS: m/z: 376.20 (M−) (377.35).
3-(4-bromophenyl)-2-methylquinazolin-4(3H)-one (9)
Yield: 53%; Mp = 170–173°C (lit. 168–169°C ( Citation36)); Rf = 0.69; 1H-NMR (300 MHz, ppm, DMSO-d6): 2.14 (s, 3H, CH3), 7.51–7.54 (m, 3H, arom.), 7.63–7.68 (m, 3H, arom.), 7.85 (m, 1H, arom.), 8.08 (dd, J = 7.91, 1. 5 Hz, 1H, arom); 13C-NMR (150 MHz, DMSO-d6) δ (ppm): 161.92, 154.6, 147.8, 137.2, 135.1, 134.1, 130.9, 130.1, 127.1, 126.9, 126.8, 120.9, 24.5; MS: m/z: 314.80 (M−) (315.16).
2-((4-methyl-2-oxo-2H-chromen-7-yl)oxy)-N-(2-methyl-4-oxoquinazolin-3(4H)-yl)acetamide (10)
Yield: 75%; Mp = 230°C; Rf = 0.28; 1H-NMR (600 MHz, ppm, DMSO-d6): 2.11 (s, 3H, CH3), 2.36 (s, 3H, CH3), 5.01 (s, 2H, CH2), 6.20 (s, 1H; C-3 coum.),6.83 (dd, J = 8.80, 2.2 Hz, 1H, arom.), 6.93 (s, 1H, arom.), 7.54 (d, J = 8.07 Hz, 1H, arom.), 7.61 (d, J = 8.80 Hz, 1H, arom.), 7.66 (m, 1H, arom.), 7.76 (m, 1H, arom.), 8.05 (m, 1H, arom.); 13C-NMR (150 MHz, DMSO-d6) δ (ppm): 165.5, 160.2, 159.9, 154.3, 153.2, 152.8, 149.4, 133.3, 132.4, 131.4, 130.4, 129.6, 129.4, 126.3, 113.6, 112.2, 111.44, 101.6, 60.5, 18.1, 10.4; MS: m/z: 392.20 (M+) (391.38).
Anticancer activity investigation
Biological activity of the compounds was tested on a panel of tumor cells. Cells were both adherent and suspension cultures. Adherent cell lines used (BJ, MDCK-1, HeLa, CaCo-2) were grown in DMEM medium (Gibco, EU). Suspension cell lines (K562, CCRF-CEM, HuT-78) were grown in RPMI 1640 medium (Gibco, EU). Both media were supplemented with 10% heat-inactivated fetal bovine serum-FBS (Gibco, EU), 2 mM glutamine (Gibco, EU), 1 mM sodium pyruvate (Gibco, EU), 10 mM HEPES (Sigma-Aldrich, USA) and 100 U/0.1 mg antibiotic/antimycotic (Gibco, EU).
Cells were grown on 37°C, with 5% CO2 gas in humidified CO2 incubator (IGO 150 CELLlifeTM, JOUAN, Thermo Fisher Scientific, Waltham, MA, USA). A trypan blue dye exclusion method was used to assess cell viability before plating. Tested compounds were dissolved in DMSO (dimethyl sulfoxide) as a 1×10−2 M (mol dm-3) stock solution. Working dilutions were prepared by diluting stock solution in appropriate media to get a concentration range 10−3 - 10−5 M.
For the MTT test ( Citation37), cells were seeded on 96 micro well flat bottom plates (Greiner, Austria) at 2×104 cells/ml or 1×105 cells/ml (adherent and suspension cells respectively). After 72 h of incubation with the tested compounds, MTT (Merck, Germany) was added. DMSO (Merck, Germany) was used to dissolve the formed MTT-formazane crystals after 4 h incubation. Absorbance was measured at 595 nm on Elisa micro plate reader (iMark, BIO RAD, Hercules, CA, USA) and viability calculated compared to untreated controls. All experiments were performed in triplicate.
Results and discussion
Different quinazolinone derivatives were synthesized using a green synthetic approach, deep eutectic solvents and microwaves, according to . Our attempt was to investigate the potential of deep eutectic solvents in the synthesis of quinazolinone derivatives from anthranilic acid and different amines, using two different synthetic approaches. The first approach was intended to use anthranilic acid, amines and trimethyl orthoformate to form 3-substituted-quinazolin-4(3H)-ones (1-4), and the second was the reaction of anthranilic acid, acetic anhydride (AAA) and amine to form 3-substituted-2-methyl-quinazolin-4(3H)-ones (5-10). All our efforts to use DESs in synthesis of compounds 1–4 were not successful, therefore we applied a different method. The synthesis of these derivatives via this path usually requires temperatures up to 120°C, which was not applicable for DESs, since they could degrade at such temperatures. Thus, to keep the green character of the reaction, we applied microwaves in the synthesis of these compounds. Application of microwaves in organic synthesis accelerates the reaction rates, products are often obtained in high purity with higher yields, usually under milder conditions.
Compounds 1–4 were synthesized in a reaction of anthranilic acid, methyl orthoformate and corresponding amine, in molar ratio of 1:1.2:1.2, at 120°C for 30 min. Compound 4 was synthesized from anthranilic acid only, which was mixed with methyl orthoformate in a molar ratio 2:1. A self-cyclization of anthranilic acid into 2-(4-oxoquinazolin-3(4H)-yl)benzoic acid was observed earlier ( Citation35), where the authors performed reaction of anthranilic acid with triethyl orthoformate in the presence of a catalytic amount of sulfuric acid. A postulated mechanism for this reaction could also be adopted for other synthesized compounds as follows. The formation of the final product proceeds by the formation of the intermediate imidic ester 11, followed by the nucleophilic attack of the amine to the carbon atom. In this step amidine 12 is formed, and its cyclization, followed by the elimination of water molecule, yields the final product () ( Citation17, Citation35). 1H NMR for all compounds showed a characteristic peaks for aromatic protons at 7.11-7.89 ppm, characteristic quinazolinone C-2 peak around 8.36 ppm. Methoxy group protons for compound 2 are found at 3.72 and 3.76 ppm, while carboxy group proton for compound 4 is observed at 13.18 ppm.
Compounds 5–10 are synthesized in ChCl:U (1:2) DES at 80°C. First, benzoxazinone (I) was synthesized conventionally, according to the well-known procedure, from anthranilic acid and acetic anhydride ( Citation38). A proposed mechanism for the synthesis of benzoxazinone is shown in . First acetylated anthranilic acid is formed through the nucleophilic attack of the amine to the carbon atom and subsequent formation of acetic acid. Cyclization of N-acetyl anthranilic acid and further elimination of water molecule yields the final product 2-methyl-benzoxazinone I.
Upon its synthesis, benzoxazinone was added to the DES and mixed with the corresponding amine. Since the exposure of the benzoxazinone to air can result in formation of N-acetylanthranilic acid, for compounds 5, 6 and 7, molecular sieves were added to the reaction mixture, upon which the cyclized product was formed. Compounds 8, 9 and 10 were synthesized without molecular sieves. A proposed mechanism for the synthesis of these compounds is shown in Scheme 4. A nucleophilic attack of the amine to the carbon atom causes a ring opening of the benzoxazinone, which is stabilized by the hydrogen bonding net formed with the components of the deep eutectic solvent. Further elimination of the water molecule and cyclization yields a final product, 2-methyl-3-substituted-quinazolin-4(3H)-one. Therefore, DES serves in this reaction as both the solvent and catalyst as well ().
1H NMR spectra reveals characteristic peaks for aromatic protons at 7.51–8.01 ppm, C-2 methyl protons at 2.11–2.14 ppm. For compound 8, coumarin C-4 methyl protons are observed at 2.36 ppm, two methylene protons at 4.02 and coumarin C-3 proton at 6.39 ppm. For compound 10 coumarin C-4 methyl protons are observed at 2.36 ppm, C-3 proton at 6.20 ppm and methylene proton at 5.01 ppm.
A synthesis of desired products obtained in DES was easy to perform, at temperatures under 100°C, simply by stirring. DES was easy to synthesize, from non-toxic, biodegradable, cheap and easily available components. The desired products were isolated simply by the addition of water and filtration. No catalysts were required and the temperature of 80°C was high enough to perform this type of synthesis.
Cytotoxic properties of the synthesized compounds were tested on a panel of cell lines of different origin (BJ, MDCK-1, HeLa, CaCo-2, K562, CCRF-CEM and HuT-78). We included normal cell lines to determine selectivity as well as antitumor potential. The applied compounds displayed minimal cytotoxic effect with IC50 values in almost all cases, above 100 µM. While there was no significant cytotoxic effect, the compound 1 was the most efficient overall, with HuT-78 cell line showing the highest sensitivity (). Minimal effects can be partially explained by the slow absorption rate ( Citation12). These problems can be overcome by either structural changes or delivery approaches and should be the direction of further development of these compounds.
Table 1. Calculated IC50 values [μM] for two affected cancer cell lines and two non-cancerous cell lines as control for synthesized compounds.
Conclusions
In this study, all 3-substituted-quinazolin-4(3H)-one derivatives were synthesized in moderate to excellent yields, according to the principles of green chemistry. Microwaves and deep eutectic solvents were used in the synthesis. Deep eutectic solvents were convenient for the synthesis of 2-methyl-3-substituted-quinazolin-4(3H)-ones, while in the case of 3-substituted-quinazolin-4(3H)-ones, microwaves were more efficient. All derivatives were tested for their cytotoxic effects on cell lines including BJ, MDCK-1, HeLa, CaCo-2, K562, CCRF-CEM and HuT-78, where compound 1 showed potential cytotoxic activity against HuT-78 cell line and can be used as basis for further development.
Supplemental Material
Download MS Word (707.7 KB)Acknowledgements
This work has been supported in part by Croatian Science Foundation under the project “Green Technologies in Synthesis of Heterocyclic Compounds” (UIP-2017-05-6593) and J.J Strossmayer University of Osijek intramural project “Evaluation of antitumor effects of coumarin and quinazolinone derivatives in vitro” (ZUP-2018-41).
Disclosure statement
No potential conflict of interest was reported by the author(s).
Notes on contributors
Mario Komar, BSc is a PhD student at Faculty of Chemical Engineering and Technology in Zagreb and works as assistant on the Faculty of Food Technology Osijek. The main subject of his research is application of deep eutectic solvents in organic synthesis.
Maja Molnar, PhD is an associate professor at the Faculty of Food Technology Osijek. Her research work includes an organic synthesis of heterocyclic compounds with potential biological activity. The focus of her research is the application of green synthetic methods in coumarin, quinazolinone, rhodanine, triazole synthesis.
Marijana Jukić, PhD works as a senior teaching / research assistant at the Department of Medicinal Chemistry, Biochemistry and Clinical Chemistry, Faculty of Medicine. Her research work includes study of cancer biology and anticancer drugs with possible clinical application.
Ljubica Glavaš-Obrovac, PhD is a full professor and the head of the Department of Medicinal Chemistry, Biochemistry and Clinical Chemistry at the Faculty of Medicine. Her research is focused on molecular diagnostics in immunology and application of novel compounds in cancer treatment.
Teuta Opačak-Bernardi, PhD is an associate professor at the Department of Medicinal Chemistry, Biochemistry and Clinical Chemistry at the Faculty of Medicine. Her work is focused on targeted drug delivery and signaling pathways in the development of cancer.
Additional information
Funding
References
- Couturier, C.; Lair, C.; Pellet, A.; Upton, A.; Kaneko, T.; Perron, C.; Cogo, E.; Manegotto, J.; Bauer, A.; Scheiper, B.; Lagrange, S.; Bacque, E. Bioorg. Med. Chem. Lett. 2016, 26, 5290–5299. doi: 10.1016/j.bmcl.2016.09.043
- Subramaniam, R.; Rao, G.; Jaiswal, P.K. Der Pharm. Sinica 2014, 5, 37–40.
- Gatadi, S.; Gour, J.; Shukla, M.; Kaul, G.; Das, S.; Dasgupta, A.; Malasala, S.; Borra, R.S.; Madhavi, Y.V.; Chopra, S.; Nanduri, S. Eur. J. Med. Chem. 2018, 157, 1056–1067. doi: 10.1016/j.ejmech.2018.08.070
- Poojari, S.; Naik, P.P.; Krishnamurthy, G.; Kumara, K.J.; Kumar, N.S.; Naik, S. J. Taibah Univ. Sci. 2017, 11, 497–511. doi: 10.1016/j.jtusci.2016.10.003
- Al-Obaid, A.M.; Abdel-Kamide, S.G.; El-Kashef, H.A.; Abdel-Aziz, A.A.M.; El-Azab, A.S.; Al-Khamees, H.A.; El-Subbagh, H.I. Eur. J. Med. Chem. 2009, 44, 2379–2391. doi: 10.1016/j.ejmech.2008.09.015
- Al-Suwaidan, I.A.; Abdel-Aziz, A.A.M.; Shawer, T.Z.; Ayyad, R.R.; Alanazi, A.M.; El-Mosry, A.M.; Mohamed, M.A.; Abdel-Aziz, N.I.; El-Sayed, M.A.A.; El-Azab, A.S. J. Enzyme Inhib. Med. Chem. 2016, 31, 78–89. doi: 10.3109/14756366.2015.1004059
- Al-Salem, H.S.A.; Hegazy, G.H.; El-Taher, K.E.H.; El-Messery, S.M.; Al-Obaid, A.M.; El-Subbagh, H.I. Bioorg. Med. Chem. Lett. 2015, 25, 1490–1499. doi: 10.1016/j.bmcl.2015.02.025
- Noureldin, N.A.; Kothayer, H.; Lashine, E.M.; Baraka, M.M.; El-Eraky, W.; Awdan, S.A.E. Arch. Pharm. (Weinheim). 2017, 350, e1600332. doi: 10.1002/ardp.201600332
- Nanthakumar, R.; Muthumani, P.; Girija, K. Arab. J. Chem. 2014, 7, 1049–1054. doi: 10.1016/j.arabjc.2010.12.035
- Rajput, C.S.; Singhal, S. J. Pharm. (Cairo) 2013. doi: 10.1155/2013/907525
- Wang, Z.; Wang, M.; Yao, X.; Li, Y.; Tan, J.; Wang, L.; Qiao, W.; Geng, Y.; Liu, Y.; Wang, Q. Eur. J. Med. Chem. 2012, 53, 275–282. doi: 10.1016/j.ejmech.2012.04.010
- Tiwary, B.K.; Pradhan, K.; Nanda, A.K.; Chakraborty, R. J. Chem. Biol. Therap. 2015, 1, 1–7.
- Alexandre, F.-R.; Berecibar, A.; Besson, T. Tetrahedron Lett. 2002, 43, 3911–3913. doi: 10.1016/S0040-4039(02)00619-6
- Kidwai, M.; Rastogi, S.; Mohan, R.; Ruby, T. Croat. Chem. Acta 2003, 76, 365–369.
- Errede, L.A.; Oien, H.T.; Yarian, D.R. J. Org. Chem. 1977, 42, 12–18. doi: 10.1021/jo00421a003
- Wang, L.; Xia, J.; Qin, F.; Qian, C.; Sun, J. Synthesis. (Mass). 2003, 8, 1241–1247. doi: 10.1055/s-2003-39397
- Wang, M.; Song, Z.G.; Zhang, T.T. Chem. Heterocycl Comp. 2010, 46, 581–584. doi: 10.1007/s10593-010-0548-4
- Khosropour, A.R.; Mohammadpoor-Baltork, I.; Ghorbankhani, H. Tetrahedron Lett. 2006, 47, 3561–3564. doi: 10.1016/j.tetlet.2006.03.079
- Testard, A.; Picot, L.; Lozach, O.; Blairvacq, M.; Meijer, L.; Murillo, L.; Piot, J.M.; Thiery, V.; Besson, T. J. Enzyme Inhib. Med. Chem. 2005, 20, 557–568. doi: 10.1080/14756360500212399
- Dandia, A.; Singh, R.; Sarawgi, P. J. Fluorine Chem. 2004, 125, 1835–1840. doi: 10.1016/j.jfluchem.2004.06.009
- Baghbanzadeh, M.; Molnar, M.; Damm, M.; Reidlinger, C.; Dabiri, M.; Kappe, C.O. J. Comb. Chem. 2009, 11, 676–684. doi: 10.1021/cc900036a
- Zhang, J.; Ren, D.; Ma, Y.; Wang, W.; Wu, H. Tetrahedron 2014, 70, 5274–5282. doi: 10.1016/j.tet.2014.05.059
- Elfeky, S.; Sobahi, T.; Gineinah, M.M.; Ahmed, N.S. Egyptian J. Chem. 2019, 0. doi:10.21608/ejchem.2019.7130.1587.
- Dadhania, H.N.; Raval, D.K.; Dadhania, A.N. Res. Chem. Intermed. 2018, 44, 117–134. doi: 10.1007/s11164-017-3093-2
- Magyar, T.; Miklós, F.; Lázár, L.; Fülöp, F. Chem. Heterocycl. Comp. 2015, 50, 1464–1470. doi: 10.1007/s10593-014-1611-3
- Acharyulu, P.V.R.; Dubey, P.K.; Reddy, P.P.V.V.; Suresh, T. ARKIVOC 2008. http://dx.doi.org/10.3998/ark.5550190.0009.b10.
- Patel, T.S.; Bhatt, J.D.; Vanparia, S.F.; Patel, U.H.; Dixit, R.B.; Chudasama, C.J.; Patel, B.D.; Dixit, B.C. Bioorg. Med. Chem. 2017, 25, 6635–6646. doi: 10.1016/j.bmc.2017.10.041
- Ghosh, S.K.; Nagarajan, R. RSC Adv. 2016, 6, 27378–27387. doi: 10.1039/C6RA00855K
- Molnar, M.; Klenkar, J.; Tarnai, T. Synt. Comm. 2017, 47, 1040–1045. doi: 10.1080/00397911.2017.1291815
- Lobo, H.R.; Singh, B.S.; Shankarling, G.S. Catal. Commun. 2012, 27, 179–183. doi: 10.1016/j.catcom.2012.07.020
- Zhang, Z.-H.; Zhang, X.-N.; Mo, L.-P.; Li, Y.-X.; Ma, F.-P. Green Chem. 2012, 14, 1502–1506. doi: 10.1039/c2gc35258c
- Liu, Y.; Friesen, J.B.; McAlpine, J.B.; Lankin, D.C.; Chen, S.N.; Pauli, G.F. J. Nat. Prod. 2018, 81, 679–690. doi: 10.1021/acs.jnatprod.7b00945
- Zhang, Q.; Vigier, K.D.O.; Royer, S.; Jérôme, F. Chem. Soc. Rev. 2012, 41, 7108–7146. doi: 10.1039/c2cs35178a
- Ighilahriz, K.; Boutemeur, B.; Chami, F.; Rabia, C.; Hamdi, M.; Hamdi, S.M. Molecules 2008, 13, 779–789. doi: 10.3390/molecules13040779
- Cao, S.-L.; Zhang, M.; Feng, Y.-P.; Jiang, Y.-Y.; Zhang, N. Synth. Comm. 2008, 38, 2227–2236. doi: 10.1080/00397910802026584
- Kumar, D.; Jadhavar, P.S.; Nautiyal, M.; Sharma, H.; Meena, P.K.; Adane, L.; Pancholia, S.; Chakraborti, A.K. RSC Adv. 2015, 5, 30819–30825. doi: 10.1039/C5RA03888J
- Mickisch, G.; Fajta, S.; Keilhauer, G.; Schlick, E.; Tschada, R.; Alken, P. Urol. Res. 1990, 18, 131–136. doi: 10.1007/BF00302474
- Singh, T.; Sharma, S.; Srivastava, V.K.; Kumar, A. Ind. J. Chem.-B 2006, 45B, 2558–2565.