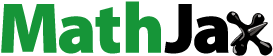
ABSTRACT
A three-component one-pot synthesis of new thiazolyl coumarin derivatives was carried out by condensing 3-acetyl-4-hydroxycoumarin, arylaldehydes, thiourea and ammonium acetate at reflux in DMC. The optimization details of the developed novel protocol are recorded. In addition, we have also synthesized these compounds via another second route in two steps. High atom-economy, excellent yields, simple procedure, and mild reaction conditions are the important features of this one-pot protocol. The chemical structures of the newly synthesized compounds were elucidated by using analytical (IR, 1H NMR, 13C NMR). Further, all the compounds were screened for their antioxidant activities.
GRAPHICAL ABSTRACT

1. Introduction
Multicomponent reactions (MCRs) have become a highly efficient and powerful tool in synthetic organic chemistry due to their atom-efficiency, straight forward reaction design, and flexibility in constructing diverse target organic molecules by the reaction of various precursors in a one-pot manner ( Citation1,Citation2). Moreover, purification of products gained from a multicomponent reaction is relatively simple because all active reactants are consumed and incorporated into the synthesis of the target product ( Citation3). Consequently, synthetic chemists are increasingly motivated to synthesize new compounds through MCRs. Alternately, the literature reports that coumarin derivatives have exhibited important biological activities, including antimicrobial ( Citation4–17), antifungal ( Citation18), anti-inflammatory ( Citation19), anticancer ( Citation20,Citation21), antitubercular ( Citation22), and antitumour activity ( Citation23). In view of this, we report herein (i) the synthesis of novel thiazolyl coumarin derivatives obtained by two different routes in the pursuit of finding biologically active compounds that can be further investigated for their antioxidant activity and (ii) the characterization of the structures of these new thiazolyl coumarin derivatives by spectroscopic methods (1H and 13C NMR and FT-IR).
2. Results and discussions
The acetylation of the 4-hydroxycoumarin by the method of Dholakia et al. ( Citation24) using glacial acetic acid as an acetylating agent in the presence of POCl3 afforded 3-Acetyl-4-hydroxycoumarin 1. The reaction involving any kind of competition from the intramolecular condensation of 4-hydroxycoumarin ( Citation25) (Scheme 1). The purity of this compound was checked by NMR, and the structure was confirmed by means of 1H NMR, 13C NMR, IR, and elemental analysis; the spectral data are in total agreement with the proposed structure.
This compound 1 was characterized by IR, 1H and 13C NMR. The 1H NMR shows an aromatic proton arrow as a multiplet between 7.15 and 7.92 ppm. A singlet at 2.45 ppm was assigned to the methylic proton, whilst the OH signal appeared at 17.72 ppm. This very high value of the chemical shift might be explained by only an intermolecular hydrogen bond ( Citation26). We report then the use of compound 1 in heterocyclic synthesis by exploring the scope and limitations of the functional groups present.
To seek an optimal solvent and optimal amounts of catalyst, we carried out a model reaction by stirring equimolecular amounts of 3-acetyl-4-hydroxycoumarin with benzaldehyde and thiourea in the presence of ammonium acetate (Scheme 2).
The model reaction was explored using different solvents such as DMC, DEC, ethanol, dichloromethane, THF and toluene at reflux. The results are summarized in .
Table 1. Synthesis of thiazolyl coumarins derivative 2a in the presence of different solvents.
It was found that the polarity of the solvent and the presence of ammonium acetate play important roles in the success of the reaction. This is supported in that, in chemistry, the solvent effects chemical reactivity or molecular associations. Solvents can also have an effect on solubility, stability and reaction rates. The results indicated that solvents also affected the yield of the products (). In the solvents such as dichloromethane, THF, ethanol, or toluene, the yields of compounds 2 were lower, and longer reaction times were required. This may be because when a high BP solvent is used for the synthesis, the heat supplied to the reaction mixture is largely consumed by the solvent itself and is not distributed among the reactants. Therefore, the yield is reduced because the energy needed for reactants to cross the energy barrier is lost in getting the solvent’s temperature close to its BP. It was observed that, among all solvents and media, the best result was obtained when DMC was chosen in the presence of ammonium acetate at 90°C. The desired product was obtained with excellent yield and high purity. Additionally, to optimize the amounts of ammonium acetate, the model reaction was performed with different amounts of catalyst at reflux over 4 h. The results are summarized in .
Table 2. Effect of catalyst amount on the condensation of 3-acetyl-4-hydroxycoumarin and benzaldehyde with thiourea in DMCa.
It was found out that, when the reaction was carried out in the presence of 5 mmol% of the catalyst, a 96% yield was obtained and further increase in the quantity of catalyst did not show appreciable improvement in the yield of the product. Thus, 5 mol% of catalyst was chosen as the maximum quantity of the catalyst for the reaction.
Finally, to determine the optimum reaction time in DMC, we ran the reaction at different reaction times.
The best reaction time was 240 min (, entry 6).
Table 3. Optimization of the time reaction in DMC.
The best reaction time was 240 min (, entry 6). In order to explore the generality of this multicomponent reaction (MCR), using the optimized reaction conditions (DMC, NH4OAc (5 mmol), 240 min) the scope of the method was investigated with a series of substituted aromatic aldehydes (Scheme 3). Thus, refluxing of 3-acetyl-4-hydroxycoumarin with arylbenzaldehyde and thiourea in DMC catalyzed by NH4OAc during 4 h at reflux led to the formation of thiazoles incorporated coumarins derivatives 2a-f with good yields. Remarkably, the reactions were clean, and all products were obtained after only filtration and simple washing with water and ethanol. Thus, a simple treatment gives the title products without need of chromatographic purification. The results are summarized in .
Table 4. Synthesis of thiazolyl coumarins derivatives 2a-f.
A variety of aryl aldehydes containing different substituents (e.g. –NO2, –OH, –CH3, –OCH3, p-benzyloxy) reacted in this MCR to provide excellent product yields (78–96%). All the reactions listed in are very clean and afforded excellent yields of the products. As seen from , the aromatic aldehydes carrying both electron-withdrawing (Entries 1–5) and electron-donating functional groups underwent successful condensation with ethyl 3-acetyl-4-hydroxycoumarine and thiouera in the presence of catalytic amount of ammonium acetate in DMC at reflux to afford the corresponding products 2a-f in good yields. The structure of the new compounds 2a-f was identified by their 1H- and 13C NMR data as well as by their elemental analysis and NMR 2D. The structural assignments of compounds 2a-f were based on their analytical and spectral data. The 1H NMR spectra of compounds 2c showed multiplet due to H12’ proton within δ = 6.51 ppm, signal of proton H13’ within δ = 6.98 ppm and protons H6,7 at δ = 7.31 ppm respectively, while δ = 7.44 ppm belonging to H5,8. A multiplet at 7.86 ppm corresponding to protons H9’ and H11’. The proton H7’ appear at 8.00 ppm and the proton H5’ resonated at 8.03 ppm. The 13C NMR spectra of the product 2c exhibited signals within δ = 124.0 ppm attributed to C5’ carbon, signals within δ = 136.59ppm, δ = 151.9ppm, δ = 154.9ppm, δ = 164.4ppm, δ = 177.8 ppm and δ = 205.4 ppm attributed to the C7’, C10, C2, C10’ and C4 carbons respectively. The FT-IR spectra of 2a-f in KBr disk showed the absorption bands within ν = 1550 cm−1 corresponding C = C group, within ν = 1710 cm−1 belonging to carbonyl group and within ν = 3400 cm−1 attributed to the OH group.
However, assignments of thiazolyl coumarins derivative 2c deduced from his HMBC 2D-NMR spectra. H8 correlate only with carbon C5. In a similar manner, aromatic protons H7’ and H9’ correlate with the carbons C9’ and C7’. Consequently, this latter correlation shows C2’—C7’ linkages.
A plausible mechanism for the synthesis of thiazolyl coumarins derivatives (2a-f) is depicted in Scheme 4.
The amine group of thiourea attacks the carbonyl group to give the intermediate (i) which is then stabilized by a prototropy, then dehydration followed by deprotonation of the intermediate (i) leads to the formation of intermediate (ii), the cyclization of (ii) gave the product 1’.
The amine group of compound 1’attack then the carbonyl of the aromatic aldehyde leads to the intermediate (j).
Dehydration of intermediate (j) followed by deprotonation leads to the formation of final product 2.
In the second path, we tried to synthetize thiazolyl coumarins derivatives (2a-f) with another route (Scheme 5).
First, the 3-acetyl-4-hydroxycoumarine condensed with thiourea in the presence of ammonium acetate at reflux in toluene during 5 h gave compound 1’. The structure of compound 1’ was confirmed by spectroscopic methods such as 1H NMR and 13C NMR. In the 1H NMR spectra, of compound 1’ showed a singlet proton at 12.02 ppm for the –NH2 – and a singlet around at 9.98 ppm for the H5’ proton. Aromatic protons appear between 7.26 and 7.92 ppm. The 13C NMR spectra of compounds 1’ showed the carbon C4’ at 95.9, the carbon C2 at 133.8 ppm and carbon C4 at 176.9 ppm. The 13C NMR spectra of compound 1’ showed absorption in the range 116-153.4 ppm corresponding respectively to carbons C5,6,7,8, C3,9, C5’, C2’ and C10.
Then the obtained compound was refluxed with arylaldehydes in DMC in the presence of NH4OAc for 4 h to achieve targeted molecule 2a-f. Completion of reaction was monitored by the thin layer chromatography technique. After completion of reaction mixture was treated with cold water, separated solid was filtered, dried and recrystallized. The products were obtained in moderate to good yields.
The FT-IR spectra of compound 2e, absorption bands attributed to stretching vibration of OH group appeared within ν = 3500 cm–1, as well as stretching vibrations of C=N group were recorded within ν = 1650 cm–1. In 1H NMR spectra, the singlet at 2.72 ppm corresponds to the methylic protons Hb of the compounds 2e. The presence of the signals of H5’ and H7’ at 8.06 and 7.87 ppm respectively support the formation of compound 2e. In 13C NMR, signal at δ 30 ppm assigned to Cb, signal at δ 159.7 confirmed lactone carbonyl of 2e and the signal at δ 116.8 ppm due to C3. Other signals are in good agreement with the target compounds in accordance with the literature data for other 4-hydroxycoumarin derivatives ( Citation27–30).
In summary, both synthetic routes successfully provided a strategy for synthesis of thiazolyl coumarin derivatives (2a-f). In the first route, the thiazolyl coumarin derivatives (2a-f) are available, and the starting material is low in cost and can be obtained easily. This synthetic route not only saves time but also lowers the cost of manufacturing. The second route is longer. For this reason, it is not suitable for large-scale preparation. The second route is based on the materials that are commercially available, and the reaction involves 2 steps.
3. Antioxidant activity
Hydrazino-thiazole derivatives have been shown recently to possess radical scavenging ability and some simple structure–activity relationships have been described for a small library of compounds (31), in this way the in-vitro antioxidant activities of the thiazolyl coumarin derivatives 2 were screened using DPPH (1,1-diphenyl-2-picrilhydrazyl). The activity results of the newly synthesized compounds are represented in .
The analysis of the results showed excellent antiradical activity according to the two used controls, butylated hydroxytoluene (BHT) and gallic acid (GA). In fact, the products 2d and 2g have average antioxidant activity. Alternately, the products 2a, 2b, 2c and 2e have very high activity. The activity of this thiazolyl coumarin compared closely with other known hydrazino-thiazoles (IC50 15–60 μM at a DPPH radical concentration of 0.1 mM) ( Citation31) implying that the coumarin and phenolic functions had not adversely affected antioxidant activity. The activity of these compounds was attributed for the most part to the hydrazinothiazole functionality but clearly was modulated and enhanced by the incorporation of other unique groups. The presence of the hydrazino N-H group (Scheme 4) and the phenolic hydroxyl were viewed as important structural features, both having the ability for hydrogen atom transfer (HAT) to the DPPH free radical to give a resonance stabilized radical.
4. Conclusion
In conclusion, we have successfully developed an easy and efficient method for the preparation of a variety of thiazolyl coumarin derivatives (2a-f) from the reaction of different aromatic aldehydes, 3-acetyl-4-hydroxycoumarin, and thiouera in DMC and in the presence of ammonium acetate. We have also synthesized the concerned thiazolyl coumarin derivatives (2a-f) via another route, and both synthetic routes successfully provided a strategy for synthesis of synthesized thiazolyl coumarin derivatives (2a-f).
After completion of the reaction as indicated by TLC, the crude product was purified by recrystallization from ethanol to yield highly pure thiazolyl coumarin derivatives. The first method has advantages such as high yields of products and short reaction times. Furthermore, the compounds 2 were investigated for antioxidant activities by DPPH (2,2-diphenyl-1-picrylhydrazyl). The physical data (M.p., IR, NMR) of known compounds were found to be identical to those reported in the literature. Spectroscopic data for selected examples are shown below.
5. Experimental
Experimental section was done according earlier procedures ( Citation31).
5.1. General procedure for the synthesis of 3-acetyl-4-hydroxycoumarin 1
A mixture of 12 g of 4-hydroxycoumarin (18.6 mmol), 64 ml of acetic acid (279 mmol) and 22.4 ml of phosphorus chloride oxide (60 mmol) was stirred at reflux. The mixture was maintained at 1 h, resulting in a yellow colored solid mass, which was separated out. It was recrystallized from ethanol to obtain the target compound.
Yield: 85%, mp:225°C, FT-IR (KBr) ν, cm−1: 1630 (C=O ketone); 1750 (C=O lactone); 3300 (OH). 1H NMR(300 MHz, CDCl3): δ (ppm) 2.65 (S, 3H, Hb); 7.42 (m, 2H, H6,7); 7.81 (m, 1H, H5); 7.97 (dd, 1H, H8). 13C NMR (75 MHz, CDCl3):δ (ppm) 29.5 (Cb); 101.3 (C3); 114.5 (C6); 1168. (C7); 124.5 (C5); 135.4 (C8); 136.7 (C9); 154.2 (C10); 159.1 (C2); 177.7 (Ca); 205.3 (C4).
5.2. General procedure for the synthesis of 3-(2-amino-thiazol-4-yl)-4-hydroxy-chromen-2-one 1’
A mixture of 3-acetyl-4-hydroxycoumarin (1.63 g, 8 mmol) and thiourea (0.6 g, 8 mmol) in toluene (10 mL) catalyzed by ammonium acetate (0.61 g, 8 mmol), was heated under reflux for 5 h. The reaction mixture was left to cool. The resulting precipitate was filtered off, dried, and recrystallized from MeOH to furnish 1’.
Yield: 48%, mp:180°C, FT-IR (KBr) ν, cm−1: = 3000 (OH); 1680(C=O). 1H NMR (300 MHz, DMSO-d6): δ (ppm) 7.26 (m, 4H, Hb, H6,7); 7.60 (m, 1H, H5); 7.92 (m, 1H, H8); 9.98 (s, 1H, H5’); 12.02(s, 1H, Ha). 13C NMR (75 MHz, DMSO-d6): δ (ppm) 95.9 (C4’); 116.0 (C5,6,7,8); 120.1 (C3,9); 123.7 (C5’); 125.9 (C2’); 133.8 (C2); 153.4 (C10); 1769. (C4).
5.3. General procedure for the synthesis of thiazolyl coumarins derivatives path 1
In a 25-mL round-bottomed flask was taken a mixture of 3-acetyl-4-hydroxycoumarin (8 mmol), ammonium acetate (8 mmol) and aromatic aldehyde (8 mmol) in 15 mL of DMC. Then, thiourea (8 mmol) was added into the above reaction mixture, and the reaction flask was transferred for refluxing into a heated oil-bath. The progress of the reaction was monitored by checking TLC from time to time. After that, the reaction flask was removed from the oil bath, and it was brought to room temperature to complete precipitation. The solid precipitate was just filtered through a Büchner funnel and dried through a vacuum pump. The pure product of thiazoles-incorporated coumarin derivatives (2a-f) was obtained after recrystallization from dichloromethane and hexane.
5.4. Path 2
A mixture of 3-acetyl-4-hydroxycoumarin (8 mmol) and thiourea (8 mmol) was stirred under reflux in toluene (10 ml) in the presence of ammonium acetate (8 mmol) for 5 h. The reaction mixture was allowed to cool to room temperature. The formed solid was filtered off and recrystallized from methanol to produce the 3-(2-amino-thiazol-4-yl)−4-hydroxy-chromen-2-one 1’ in good yields. After that, a mixture of compound 1’ (10 mmol) and the appropriate aromatic aldehyde (10 mmol) was stirred under reflux in DMC (15 mL) for 4 h. The (E)-3-(2-(arylidenamino)thiazol-4-yl)-4-hydroxy-2H-chromen-2-ones 2 was obtained after recrystallization.
5.5. (E)-3-(2-(benzylideneamino)thiazol-4-yl)-4-hyroxy-2H-chromen-2-one (2a)
Yield: 75%, mp:220°C, FT-IR (KBr) ν, cm−1: 3310 (OH); 1725(C=O); 1567 (C=N). 1H NMR(400 MHz, DMSO-d6): δ (ppm) 7.16(m, 5H, H9’,10’,11’,12’,13’); 7.34(m, 4H, H5,6,7,8); 7.59(m, 1H, H7’); 7.91(dd, 1H, H5’). 13C NMR (100 MHz, CDCl3): δ (ppm) 103.5(C4’);115.9(C10’,11’,12’);119.0(C9’,13’); 123.6(C5’);123.8(C5,6,7,8); 124.5(C9);128.4(C8’); 132.0(C3); 146.0(C7’); 150.7(C2’); 152.9(C2); 164.4(C10); 164.2(C4).
5.6. (E)-4-hydroxy-3-(2-((4-nitrobenzylidene)amino)thiazol-4-yl)-2H-chromen-2-one (2b)
Yield: 75%, mp:110°C, FT-IR (KBr) ν, cm−1: 3300 (OH); 1720(C=O); 1600(C=N); 1550(C=C). 1H NMR(400 MHz, DMSO-d6): δ (ppm): 7.43(m, 4H, H5,6,7,8); 7.80(m, 2H, H9’,13’); 7.98(dd, 2H, H10’, 12’); 8.15(d, 1H, H7’); 8.39(d, 1H, H5’); 10.16(s, 1H, Ha). 13C NMR (100 MHz, CDCl3): δ (ppm) 101.2(C4’);114.5(C3);116.9(C5’); 124.4(C9’,13’);124.7(C5,6,7,8); 125.2(C9,10’,12’);130.4(C8’); 136.5(C7’); 154.1(C11’); 159.0(C10); 177.0(C2); 192.1(C2’); 205.4(C4).
5.7. (E)-4-hydroxy-3-(2-((3-hydroxybenzylidene)amino)thiazol-4-yl)-2H-chromen-2-one (2c)
Yield: 75%, mp:120°C, FT-IR (KBr) ν, cm−1: 3400(OH); 1710(C=O); 1600(C=N); 1550(C=C).; 1H NMR(400 MHz, DMSO-d6): δ (ppm): 6.51(H12’); 6.98(H13’); 7.31(H6,7); 7.44(H5,8); 7.86(H9’,11’); 8.00(H7’); 8.03(H5’). 13C NMR (100 MHz, CDCl3): δ (ppm) 101.3(C4’); 104.0(C3); 112.3(C13’); 113.6(C12’); 114.7(C11’); 115.7(C6); 116.8(C7); 117.4(C5); 118.6(C8); 123.4(C9); 124.0(C5’); 124.8(C9’); 125.2(C8’); 136.59(C7’); 151.9(C10); 154.9(C2); 164.4(C2’); 177.8(C10’); 205.4(C4).
5.8. (E)-4-hydroxy-3-(2-((2-methylbenzylidene) amino)thiazol-4-yl)-2H-chromen-2-one (2d)
Yield: 75%, mp:220°C, FT-IR (KBr) ν, cm−1: 3450(OH); 1720(C=O); 1580(C=N).; 1H NMR(400 MHz, DMSO-d6): δ (ppm): 2.62(s, 3H, Hb); 7.42(m, 4H, H6,7,11’,12’); 7.80(dd, 2H, H10’,12’); 7.85(d, 1H, H7’); 8.00(dd, 1H, Ha). 13C NMR (100 MHz, CDCl3): δ (ppm) 30.0(Cb); 101.7(C4’); 115.8(C3); 117.7(C5’); 125.7-125.7(Carom); 137.16(C7’); 154.5(C10); 159.6(C2); 178.2(C2’); 205.9(C4’).
5.9. 4-hydroxy-3-(2-((4-methylbenzylidene)amino)thiazol-4-yl)-2H-chromen-2-one (2e)
Yield: 75%, mp:220°C, FT-IR (KBr) ν, cm−1: 3500(OH); 1710(C=O); 1650(C=N).; 1H NMR(400 MHz, DMSO-d6): δ (ppm): 2.72(s, 3H, Hb); 7.37(m, 2H, H10’,12’); 7.49(m, 2H, H9’,13’); 6.7(m, 2H, H6,7); 7.87(m, 2H, H5,8); 8.03(d, 1H, H7’); 8.06(d, 1H, H5’). 13C NMR (100 MHz, CDCl3): δ (ppm) 30.0(Cb); 102.7(C4’); 116.8(C3); 118.7(C5’); 126.7-125.7(Carom); 138.16(C7’); 155.5(C10); 159.7(C2); 177.2(C2’); 204.9(C4’).
5.10. (E)-4-hydroxy-3-(2-((3-methoxybenzaldiene)amino)thiazol-4-yl)2H-chromen-2-one (2f)
Yield: 75%, mp:220°C, FT-IR (KBr) ν, cm−1: 3500(OH); 1710(C = O); 1650(C = N).; 1H NMR(400 MHz, CDCl3): δ (ppm): 3.63(s, 3H, CH3); 6.29(s, 1H, H9’); 6.70(m, 3H, H11’,12’,13’); 7.31(m, 4H, H5,6,7,8); 7.54(dd, 1H, H7’); 7.89(d, 1H, H5’). 13C NMR (100 MHz, CDCl3): δ (ppm) 30.0(Cb); 54.5(CH3); 109.5(C6,8); 112.7(C5,8); 115.5(C9’,11’,12’,13’); 118.6(C9,8’); 123.0(C3); 123.7(C4’); 128.5(C7’); 131.4(C2’); 152.1(C2); 158.7(C10’); 164.3(C10); 165.6(C4).
5.11. Antioxidant activity: DPPH radical scavenging activity
Scavenging of 2,2-diphenyl-1-picrylhydrazyl radical (DPPH• examine) is the least difficult and most generally discussed technique for screening antioxidant activity. The methodology includes measurement of the decrease in absorbance of DPPH at its assimilation maxima of 517 nm. This test decides the scavenging of stable radical species as indicated by the method of Kirby and Schmidt (
Citation32,
Citation33), with slight alterations. Briefly, synthesized compounds were dissolved in dimethylsulfoxide (DMSO)/water (1/9; v/v) and diluted with ultrapure water at different concentrations (1, 0.5, 0.250, 0.125, 0.0625, and 0.03125 mg/ml). Then, 500 μl of a 4% (w/v) solution of DPPH radical in ethanol was mixed with 500 μl of samples. The mixture was incubated for 30 min in the dark at room temperature. The scavenging capacity was determined spectrophotometrically by monitoring the decrease in absorbance at 517 nm against a blank. The percentage of antiradical activity (% ArA) has been calculated as follows:All tests are examined in triplicate and communicated as the average ± standard deviation of the measurements to create the concentration scope of 0.0048–20 mg/ml.
Acknowledgments
This work was supported by the Research Supporting Project (RSP2019/75), King Saud University, Riyadh Saudi Arabia.
Disclosure statement
No potential conflict of interest was reported by the author(s).
Notes on contributors
Donia Bensalah is a PhD student.
Aziza Mnasri is a PhD student.
A. Chakchouk-Mtibaa is a post doctoral position with Witalag compagny.
Lamjed Mansour was born in Ghannouch (South of Tunisia) in 1973. He is Associate Professor in the College of Science at King Saud University since 2012. He received his bachelor’s degree in Natural Sciences and MSc degree of Biology from the Faculty of Science of Tunis and received his PhD degree in Biology from the Faculty of Sciences of Tunis and Blaise Pascal University (France). He was Assistant Professor at the Faculty of Sciences of Gafsa (2005–2008) and in Faculty of Sciences of Gabes (2008–2012) and promoted Associate Professor at Carthage University in Tunis. His filled of interest is Parasitology and human diseases and treatment, mainly cancer and autoimmune disorders. He was expert in drug screening from natural products and chemistry synthesis targeting human diseases and boosting the Immune system. He published more than 85 papers in peerreviewed international journals since 2004.
L. Mellouli Ing., PhD, Professor in Biotechnology, is an author of 65 international scientific publications with IF and inventor of 19 National Patents. He is awarded with 12 research grants of foreign, MESRS (Tunisia) and other organizations.
Dr Naceur Hamdi currently holds the position of Professor of Chemistry at the University of Carthage in Tunisia. He has more than 15 years of working experience in the field of organic and organometallic chemistry, homogeneous catalysis. I have a worldwide reputed recognition and I am well connected to the international inorganic and organometallic communities. His research interests include: (i) the activation of small inorganic and organic molecules (ii) the chemistry of transition-metal include Green Chemistry and homogenous catalysis transformations. Dr Hamdi has more than 80 publications in peer-reviewed international journals along with book contributions (with more than 1000 citations). I have been invited to deliver over 70 talks at international conferences, symposia and schools I am currently ranked by ISI Web of Science with h-index 24.
Additional information
Funding
References
- Farahi, M.; Karami, B.; Keshavarz, R.; Khosravian, F. RSC Adv. 2017, 7, 46644. doi: 10.1039/C7RA08253C
- Váradi, A.; Palmer, T.C.; Dardashti, R.N.; Majumdar, S. Molecules 2015, 21, 19. doi: 10.3390/molecules21010019
- Zareai, Z.; Khoobi, M.; Ramazani, A.; Foroumadi, A.; Souldozi, A.; Ślepokura, K.; Lis, T.; Shafiee, A. Tetrahedron 2012, 68, 6721. doi: 10.1016/j.tet.2012.05.112
- Raghu, M.; Nagaraj, A.; Sanjeeva Reddy, C. J. Heterocyclic Chem. 2009, 46, 261. doi: 10.1002/jhet.63
- Pereira, R.; Gaudon, C., Iglesias, B., Germain, P.; Gronemeyer, H.; de Lera A.R. Bioorg. Med. Chem. Lett. 2006, 16, 49. doi: 10.1016/j.bmcl.2005.09.060
- Tsurumi, S.; Ueda, H.; Hayashi, K.; Takase, S.; Nishikawa, M.; Kiyoto, S.; Okuhara, M. J. Anti-Biot. 1995, 48, 1066. doi: 10.7164/antibiotics.48.1066
- Bell, F.W.; Cantrell, A.S.; Hoberg, M.; Richard Jaskunas, S.; Johansson, N.G.; Jordan, C.L.; Kinnick, M.D.; Lind, P.; Morin, J.M. Jr; Noreen, R.; Oberg, B.; Palkowitz, J.A.; Parrish, C.A.; Pranc, P.; Sahlberg, C.; Ternansky, R.J.; Vasileff, R.T.; Vrang, L.; West, S.J.; Zhang, H.; Zhou, X.-X. J. Med. Chem. 1995, 38, 4929. doi: 10.1021/jm00025a010
- Millan, D.S.; Prager, R.H.; Brand, C.; Hart, P.H.Tetrahedron 2000, 56, 811. doi: 10.1016/S0040-4020(00)00019-3
- Wang, W.L.; Yao, D.Y., Gu, M., Fan, M.-Z.; Li, J.-Y.; Xing, Y.-C.; Nan, F.-J. Bioorg. Med. Chem. Lett. 2005, 15, 5284. doi: 10.1016/j.bmcl.2005.08.046
- Eicher, T.; Hauptmann, S. The Chemistry of Heterocycles: Structure, Reactions, Syntheses, and Applications, 2nd ed.; Wiley-VCH: Weinheim, 2003.
- Quin, L.D.; Tyrell, J.A. Fundamentals of Heterocyclic Chemistry: Importance in Nature and in the Synthesis of Pharmaceuticals; Wiley and Sons: Hoboken, 2010.
- Chen, B.; Heal, W. Comprehensive Heterocyclic Chemistry. III; Pergamon: New York, 2008, vol. 4, 635.
- Wu, Y.J.; Yang, B.V. Heterocyclic Chem. 2012, 29, 307.
- Usman, A.; Fun, H.; Chantraptomma, S. Acta Cyst. 2003, 59, 41.
- Breslow, R. Am. J. Chem. Soc. 1958, 80, 3719. doi: 10.1021/ja01547a064
- Matos, M.J.; Rodriguez, S.V.; Santana, L.E.; Uriarte, C.F.; Edfu, Y.; Santos, A.; Munoz-Crego. Med. Chem. 2012, 8, 1140.
- Yuan, S.; Cheng-He, Z. Bioorg. Med. Chem. Lett. 2011, 21, 956. doi: 10.1016/j.bmcl.2010.12.059
- Fsahin, C.; Sajak; Ertan, M. FABAB, Farm. Org. 1998, 13, 365; Steering I.T.; Worthington, P.A. PCT/N/APP/WO, 93, 08, 1991, 180. Chem. Abstr. 1993, 119, 180765K.
- Bansal, Y.; Sethi, P.; Bansal, G. Med. Chem. Res. 2013, 22, 3049. doi: 10.1007/s00044-012-0321-6
- Mahantesha, B.; Vishwanath, B.J.; Nivedita, N.B.; Sandeep, S.L.; Venkatesh, D.; Narayanachar, D. Eur. J. Med. Chem. 2014, 74, 225. doi: 10.1016/j.ejmech.2013.12.061
- Anuradha, T.; Ramit, S.; Vikas, J. Eur. J. Med. Chem. 2015, 101, 476. doi: 10.1016/j.ejmech.2015.07.010
- Yuan-Qiang, H.; Zhi, X.; Shu, Z.; Xiang, W.; Jun-Wei, D.; Zao-Sheng, L.; Lian-Shun, F. Eur. J. Med. Chem. 2017, 136, 122. doi: 10.1016/j.ejmech.2017.05.004
- Alessandra, B.; Concettina, C.; Angela, R.; Giovanna, F.; Azzurra, S.; Federica, B.; Rita, M.C.; Martino, D.; Emil, M.; Alessia, M.; Stefano, L.; Silvia, G. Eur. J. Med. Chem. 2017, 127, 577. doi: 10.1016/j.ejmech.2017.01.020
- Dholakia, V.N.; Parekh, M.G.; Trivedi, N.K. Aust. J. Chem. 1968, 22, 345.
- Eggenweiler, M.; Gassen, M.; Rochus, J.; Poeschke, O.; Wolf, M. Merck Patent Gmbh, Germany, PCT. Int. Appl. Chem Abstr. 2001, 134, 331.
- Fylaktakidou, K.C.; Hadjipavlou-Litina, D.J.; Litinas, K.E.; Nicolaides, D.N. Curr. Pharm 2004, 10, 3813; Hamdi, N.; Sakellariou, R.; Speziale, V. J. Heterocycl. Chem. 1992, 29, 1817.
- Moreau, F.; Desroy, N.; Genevard, J.M.; Vongsouthi, V.; Gerusz, V.; Le Fralliec, G.; Oliveira, C.; Floquet, S.; Denis, A.; Escaich, S.; Wolf, K.; Busemann, M. Med. Chem. Lett. 2008, 18, 4022. doi: 10.1016/j.bmcl.2008.05.117
- Dömling, A. Chem. Rev. 2006, 106, 17. doi: 10.1021/cr0505728
- Wang, J.J.; Feng, X.; Xun, Z.; Shi, D.Q.; Huang, Z.B. J. Org. Chem. 2015, 80, 8435. doi: 10.1021/acs.joc.5b01314
- Kumar, A.; Gupta, M.K.; Kumar, M. Tetrahedron Lett. 2011, 52, 4521. doi: 10.1016/j.tetlet.2011.06.040
- Tagg, J.R.; McGiven, A.R. Assay System for Bacteriocins. App. Microbiol. 1971, 21, 943. doi: 10.1128/AEM.21.5.943-943.1971
- Gulluce, M.; Sahin, F.; Sokmen, M.; Ozer, H.; Daferera, D.; Sokmen, A.; Polissiou, M.; Adiguzel, A.; Ozkan. H. Antimicrobial and Antioxidant Properties of the Essential Oils and Methanol Extract from Mentha Longifolia L. ssp. Longifolia. Food Chem. 2007, 103, 1449–1456. doi: 10.1016/j.foodchem.2006.10.061
- Kirby, A.J.; Schmidt, R.J. The Antioxidant Activity of Chinese Herbs for Eczema and of Placebo Herbs-1. J. Ethnopharmacol. 1997, 56, 103–108. doi: 10.1016/S0378-8741(97)01510-9