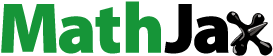
ABSTRACT
A simple, one-pot procedure for the synthesis of novel 3-phenyl-3,4-dihydro-2H-benzo[a][1,3] oxazino[5,6-c]phenazine derivatives by four-component coupling reaction between benzo[a]phenazine-5-ol, formaldehyde and amine in the presence of a catalytic amount ZnO-PTA@Fe3O4/EN-MIL-101(Cr) nanopowder in ethanol at room temperature under stirring condition. ZnO-PTA@Fe3O4/EN-MIL-101(Cr) An inorganic magnetic catalyst was analyzes and described by the XRD, TEM, FESEM, TGA, AFM, VSM and ICP-OES. This study presents simple, efficient, and one-pot multicomponent protocol, which provides several advantages such as short reaction times, high yields, easy working up, chromatography-free technique, catalyst recovery, and reusability are other highlights of this work.
GRAPHICAL ABSTRACT
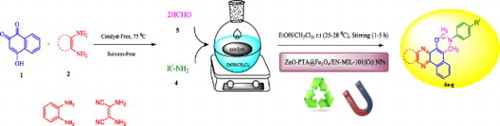
1. Introduction
Organic metal frameworks (MOFs) are made of organic liners and metal ions, which are themselves a new classification of crystalline porous materials with significant application potentials. Also, this group of materials is very porous ( Citation1–3). The characteristics of this category of materials include very high surface area, high porosity, suitable size, and flexibility ( Citation4–6). These compounds are suitable for storing gases such as hydrogen and carbon dioxide ( Citation7,Citation8). Other uses include refining and separating gases, catalyzing, and using sensors and supercapacitors. The attachment of magnetic nanoparticles to metal–organic frameworks (MMOFs) provides an opportunity to create multifunctional organic–metal magnetic catalysts that are easy to separate and recycle ( Citation9–12). Here, our attempt to create a catalyst with easy recovery and high thermal stability for organic synthesis through the design of an MMOF includes a party molecule composed of nano ZnO-HPA as an overnight acid for synthesis benzo[a][1,3]oxazino[5,6-c]phenazine derivatives ( Citation13). Heteropoly acids (HPAs) are a large family of anionic metal–oxygen clusters of early transition metals and have attracted much attention as catalysts due to their strong acidity and redox properties, which can be tuned by replacing the protons with metal cations and/or by changing the heteroatom or the framework transition metal atoms ( Citation14–17). 12-Tungstophosphoric Heteropolyacid (HPW), with the strongest Brønsted acidity in the HPA series, shows promise as a solid–acid catalyst for many acid-catalyzed organic transformations, such as hydration, esterification, alkylation, hydrolysis, etc ( Citation18–21).
In recent years, several new approaches to synthetic organic chemistry have helped to revolutionize the field. Among these is the use of multicomponent reactions (MCRs) ( Citation22–25). MCRs typically offer ease of application and efficiency, and for these reasons, they have drawn the attention of researchers in drug design and discovery ( Citation26–28).
Phenazine compounds and derivatives are heterocyclic compounds that are readily found in nature and are among biological agents; these compounds are used in fungicides, anti-malarial ( Citation29) drugs, and anti-tumor activity ( Citation30). For example, benzo[a]phenazine-5-ol and pyridazinophenazinedione derivatives are antitumor agents ( Citation31).
In this study, we firstly synthesized Nano ZnO-H3PW12O40@Fe3O4/EN-MIL-101 via hydrothermal method and proved its successful synthesis through a series of characterization means. Then, the Nano ZnO-H3PW12O40@Fe3O4/EN-MIL-101 was applied as an efficient magnetic catalyst for synthesis (Scheme 1).
2. Experimental section
2.1. Materials and methods
All solvents and reactants were obtained from commercial sources and used without any further purification. Terephthalic acid was purchased from Sigma Aldrich. Scanning electron microscope (SEM) micrographs were taken using a VPLEO–Germany 1450 microscope. TEM measurements were performed on a Philips 120 CM electronic microscope. Atomic Force Microscope (AFM) were taken using an Easy scan 2 Nanosurf microscope. Fourier transform infrared (FT-IR) spectra were recorded on a Bomem MB-Series FT-IR spectrometer. Melting points were recorded on a Barnstead electro thermal type 9200 melting point apparatus. 1H- and 13C NMR spectra were recorded on a Bruker AVANCE 500 MHz spectrometer. Thermal analysis (TGA-DTA) was carried out using a Bahr STA-503 instrument at a heating rate of 10 ◦C min−1 in air. Powder X-ray diffraction (XRD) patterns were obtained on a STOE diffract meter using Cu K radiation (=0.15418 nm). Samples were degassed at 120 0C for 4 h prior to analysis by nitrogen adsorption at −196 0C, with BET surface areas calculated over the range P/P0 = 0.05–0.35 where a linear relationship was maintained, while pore size distributions were calculated using the BJH model from the desorption isotherm. A freeze dryer (Model FD-10, Pishtaz Equipment Engineering Co, Iran) was utilized for occasional drying of samples if needed. Elemental analysis was performed with a Varian Vista-PRO ICP-OES and Thermo Scientific Flash 2000 organic elemental analyzer. Synthesis of MIL-101(Cr) was confirmed by comparison of its spectral and physical data with those of previously reported.
2.2. Synthesis of MIL-101(Cr) metal–organic framework
MIL-101 was prepared according to a procedure described by ferry et al. With a slight modification. Typically, Cr(NO3)3.9H2O (4.0 g, 9.96 mmol) was dissolved in de-ionized water (48 ml, 2650 mmol) and mixed with H2BDC (1.64 g, 9.87 mmol) via stirring. F (0.5 ml, 10 mmol) was added drop-wise to the mixture. It was then transferred to an autoclave and heated at 493 K for 8 h in a preheated oven. After natural cooling to room temperature, the resultant solid mingled with significant amounts of unreacted and recrystallized terephthalic acid ( Citation32) in solution was filtered doubly filtered using two glass filters with pore sizes between 40 and 100 mm. After drying at 423 K overnight, the crystalline MIL-101 product was obtained. To remove residual carboxylic acid accommodated in the pore, the as-synthesized MIL-101 was further purified by multi-step solvent treatments using ultrapure water, NH4F aqueous solutions, and hot ethanol, respectively ( Citation33). Subsequently, the mixture was cooled, filtered, and washed several times with water. The resulted product was finally dried overnight at 353 K under the air atmosphere.
2.3. Synthesis of EN-MIL-101
The functionalized procedure for MIL-101 was similar to reported literature. In brief, the activated MIL-101 (0.5 g) was dehydrated at 423 K in a vacuum oven for 12 h to generate CUSs. Then, the dehydrated solid was dispersed in anhydrous toluene (50 mL). An appropriate amount (1 mmol) of ED was added dropwise to the slurry and the mixture was refluxed at 110 for 12 h with continuous stirring. Finally, the as-synthesized sample was collected by filtrating and washing extensively with excess ethanol and deionized water, it was then dried in a vacuum oven at 80 C for 4 h. The product is denoted as ED-MIL-101 throughout the rest of the paper.
2.4. Synthesis of Fe3O4/EN-MIL-101
FeCl2.4H2O (2 mmol) and FeCl3.6H2O (4 mmol) were added to an aqueous suspension containing 1 g of EN-MIL-101 in 400 cc of deionized water, then of nitrogen gas passed is through ultrasonic conditions in The ambient temperature. The mixture is placed in a bath under reflux conditions for one hour to dissolve at this temperature and atmosphere, then 15 ml of 25% ammonium hydroxide solution to the mixture drop by drop to get a pH of 11. The colloidal mixture or dark suspension was initially stirred at ambient temperature for 1 h and then, 1 h of reflux until a brown colloidal mixture was obtained. The magnetic nanoparticles were washed with water to pH neutralize and dried. The bulk of iron was reported by the ICP-OES analysis of 29%.
2.5. Synthesis of nano ZnO-PTA@Fe3O4/EN-MIL-101
1 g of Fe3O4 / EN-MIL-101 was suspended in a methanolic solution of phosphotungstic acid (1.0 g in 50 mL), and mixture for 1 h sonicated and stirred at ambient temperature for 8 h, then the mixture was washed methanol and water. The amount of iron and chromium was reported by ICP-OES analysis, 23.1%, and 10.9% (Scheme 2).
3. Results and discussion
3.1. Structural characterization
X-ray diffraction patterns of MIL-101and Nano TiO2-PTA@Fe3O4/EN-MIL-101(Cr) are shown in . It is observed that all the prominent peaks of the MIL-101 sample are in good agreement with the reported literature ( Citation34), indicating that the MIL-101 has been synthesized. After being added to the ED, there are also prominent MIL-101 peaks in ED-MIL-101. ED-MIL-101 indicates that the underlying framework remains stable throughout the process. At the same time, a slight decrease in the intensity of angles was observed, which can be attributed to ED. All these observations suggest that ED has been successfully transplanted into MIL-101. However, almost all the simulated reflections of MIL-101 in XRD were retained in the final catalyst, and no characteristic reflection of phosphotungstic acid was observed in the TiO2-PTA@Fe3O4/EN-MIL-101 catalyst.
3.2. TEM and SEM analysis
The SEM and TEM images of the MIL-101 and ED-MIL-101 were shown in . As seen, crystals have been reported as a normal octahedral shape size of 0.5–1.5 µm. which were consistent with the previous report implying the material is well-synthetized. The core–shell diameter of TiO2-PTA@Fe3O4/EN-MIL-101 nanomaterial varies in the range of 35–48 nm. Bulk elemental mapping by energy-dispersive X-ray (EDX) analysis revealed a uniform distribution of tungsten. The integrity of the cubic symmetry of MIL-101(Cr) after generating magnetite nanoparticles on it is also supported by the TEM pictures (). Due to this high particle accumulation, it is difficult to distinguish between particles and calculate the average particle size, and the lack of a clear boundary between the particles causes the particle size calculation to be erroneous. The diameter of the core–shell particles of the catalysts varies in the range of 35–48 nm.
Figure 2. FESEM and TEM images of MIL-101 (a), nano ZnO-PTA@Fe3O4/EN-MIL-101 (b,c) and EDX analyses of nano ZnO-PTA@Fe3O4/EN-MIL-101 (d).
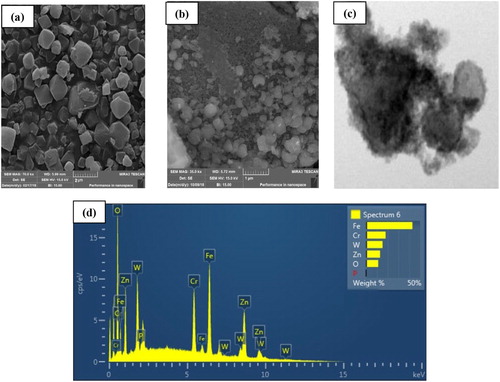
The size of the catalyst nanoparticles varied in different ranges as reported in . Below the normal distribution function below the mean particle size is obtained. The average particle size diagram is given by fitting the normal distribution function to the final catalyst ().
Figure 3. The average size of nano ZnO-HPA@Fe3O4/EN-MIL-101 particles by the normal distribution function.
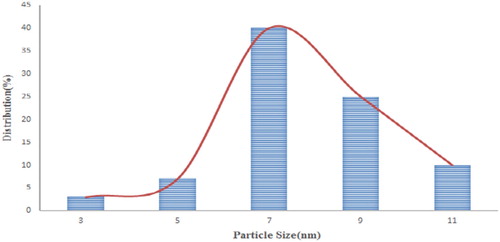
Table 1. The size of the catalyst components at different gates.
3.3. The thermal stability
The thermal stability of MIL-101, EN-MIL-101, Fe3O4/ENMIL-101, and nano ZnO-PTA@Fe3O4/EN-MIL-101 was assessed by TGA-DTA (). The curves indicate that modification with ED decreases slightly the thermal stability of MIL-101. While the ED-MIL-101 framework is still stable up to 300 0C. The TGA profile of MIL-101 showed two major mass losses in the range of 25–300 0C and 300–500 0C. The first is attributed to the loss of guest water molecules and DMF from the larger mesopore cage of MIL-101(Cr) below 200 0C and that between 200 and 300 0C from the smaller mesoporous cage Higher temperature loss is attributed to collapse of the MIL-101 framework between 300 and 500 0C due to decomposition of the dicarboxylate linkers and OH elimination. MIL-101(Cr) thus appears thermally stable to 300 0C, with a total mass loss approaching 90% by 800 0C. The addition of Nano ZnO-PTA to Fe3O4/EN-MIL-101 resulted in a reduction in total mass losses by up to 40% due to the addition of the sustained (inorganic) molecules to the restorative composition and the associated displacement in the molecules of inorganic molecules (unstable heat).
3.4. BET surface area analysis
Nitrogen Porosimetry of the parent and functionalized MOFs revealed a significant decrease in the surface area from around 2000 m2 g−1 for nano ZnO-PTA@Fe3O4/EN-MIL-101 to < 150 m2 g−1 (accompanied by a similar magnitude decrease in the pore volume), even following the first ethylenediamine functionalization step, evidencing significant pore blockage and hence in-pore incorporation of the various functionalities consistent with the structural distortion of the parent MIL-101(Cr) observed by XRD ().
3.5. AFM analysis
To further characterize the nano ZnO-PTA to Fe3O4/EN-MIL-101 Nanocomposite, the AFM analysis was applied. AFM is a beneficial instrument for investigating various structural features and parameters and has the advantage of probing in deep insights of surface topography qualitatively due to its both straight and vertical nanometer-scale spatial resolution. shows an AFM image of the nano ZnO-PTA to Fe3O4/EN-MIL-101 and its surface morphology. As observed in (a), AFM image revealed the presence of sphere-like nanoparticles and their respective particle size and morphology were close to those determined by the SEM images. In (b), the surface of composite showed an approximately uniform structure indicating that the size (height) of the particles to be approximately 35 nm.
3.6. VSM analysis
Magnetic Properties of Fe3O4, Fe3O4-EN-MIL-101, and nano ZnO-PTA@Fe3O4/EN-MIL-101(Cr) nano-particles by the vibrating sample magnetometer was examined. Magnetization diagram in terms of the magnetic field applied to each specimen in . As you can see in all three samples, there is a very narrow residual loop and a sudden increase in magnetization with a slight increase in field magnetically, thereby having a paramagnetic cloud property. For all three samples, the magnetization in the fields does not reach saturation and still has a slight increase.
3.7. The effect of catalyst components on the synthesis of the reaction
The effect of each component of the nano ZnO-PTA@Fe3O4/EN-MIL-101(Cr) catalyst on the synthesis reaction was investigated (). The Lewis Fe3O4 acid has a moderate activity of about 44% and is comparable to that of Lewis MIL-101, which has a good performance of 60%, and Lewis/Brønsted basic EN-MIL-101, which is 49% after 20 min the synthesis of the reaction has been affected. In contrast to pure PBS (homogeneous), the PTA produced 67%. The combination of Lewis acidity and nano ZnO-PTA@ Fe3O4/EN-MIL-101 (Cr) as a catalyst in compounds significantly increased activity and a higher percentage of the product than the use of acid or partial reaction in the time 20 min lead to 96 percent production.
3.8. Optimization of conditions and reaction catalyst
In this study, the one-pot synthesis of a multi-component of benzo[a][1,3]oxazino [5,6-c]phenazine derivatives of various benzene-1,2-diamines of formaldehyde and aniline Going through these optimal conditions has expanded. All reactions were performed efficiently and with high yields without the formation of lateral products, and the results are presented in . All reactions were complete within 1–3 h and resulted in the formation of the desired structure with good yield by nano ZnO-PTA@Fe3O4/EN-MIL-101(Cr) as an acid-efficient catalytic additive (60% mol) in the desired solvent Done. In this reaction, the highest yield was related to the 4a product, which used aromatic 1,2-diamines and various derivatives of formaldehyde and aniline as raw material, The percentage of the product was 96 percent. The heterogeneous nature of the present Lewis/Brönsted acid catalyst supported on a magnetic MIL-101 nanostructure is in line with the efforts on the development of new eco-compatible strategies for the synthesis of benzo[a][1,3]oxazino[5,6-c]phenazine derivatives and offers facile product separation and catalyst recycling in contrast to the catalyst. Increasing the amount of 60% nano ZnO-PTA@Fe3O4/EN-MIL-101(Cr) to more than 0.06 g showed no substantial improvement in the yield, whereas the yield decreased by decreasing the amount of the catalyst to 0.015 g. as shown in , employing magnetic separation of the catalyst and subsequent ethanol washing and drying at 90 0C for 4 h in between each reaction. We found that the catalyst could be used at least five times with only slight reduction inactivity, for example, the yield (6a) typically declined from 88% to 85%. It can be seen that the activity of the recovered catalyst with the same function is very appropriate during the two stages. In the later stages, the activity of the magnetic catalyst is reduced, which can be expressed due to the weight of the catalyst in the later stages of synthesis during the recovery process. This proper performance of the catalyst indicates its excellent performance in the reaction under solvent-free conditions and microwave radiation.
Table 2. Optimization of reaction conditions to the multicomponent synthesis of compound 6a.
We then used the optimized conditions to examine a variety of different substrates for the procedure, and the results are summarized in . Yields for these reactions were generally very good to excellent (mean yield 96%). Yields were slightly higher for compounds containing electron-withdrawing groups. The analytical data are consistent with the proposed structures (see Experimental section). For example, the 500 MHz 1H-NMR spectra of 6a exhibit multiplets at δ 6.80–8.02 ppm for the aromatic protons. The 13C-NMR spectrum for 6a displays the expected signal at δ 48.2 and 80.34 (2CH2) ppm.
Table 3. Sequential one-pot four-component synthesis of 3-phenyl-3,4-dihydro-2H-benzo[a][1,3] oxazino[5,6-c]phenazine derivativesa.
4. Experimental
4.1. General procedure for the preparation of 3-phenyl-3,4-dihydro-2H-benzo[a][1,3] oxazino[5,6-c]phenazine derivatives
To benzo[a]phenazin-5-ol (1 mmol) was added formaldehyde (1 mmol) and amine (1 mmol) and ethanol/dichloromethane as solvent (6 mL). The reaction mixture was stirred at room temperature for 10 min and then Nano ZnO-PTA@Fe3O4/EN-MIL-101(Cr) (60% mol) was added to the mixture. Stirring was continued for 1–5 h, the progress of the reaction being monitored by thin-layer chromatography (TLC). After completion of the reaction, the resulting precipitate was collected by filtration on a Buchner funnel, washed with ethanol (20 mL) and recrystallized from EtOH to afford the pure title compounds.
4.1.1. 3-phenyl-3,4-dihydro-2H-benzo[a][1,3]oxazino[5,6-c]phenazine (6a)
Red powder; yield 0.491 g (96%), m.p 279–281 °C; IR (KBr) (νmax cm−1): 3030, 2890, 1582, 1532, 1469, 1377, 1332, 1255, 1207, 1156, 1088, 745, 692 cm–1; 1H NMR (500 MHz, DMSO-d) δ 4.82 (s, 2H), 5.65 (s, 2H), 6.81–6.87 (m, 2H), 6.92 (tt, J = 7.5, 1.5 Hz, 1H), 7.27–7.34 (m, 2H), 7.38–7.47 (m, 2H), 7.48 (ddd, J = 7.3, 5.6, 3.5 Hz, 1H), 7.61 (dd, J = 5.6, 3.4 Hz, 2H), 7.84–7.97 (m, 1H), 7.90–7.97 (m, 1H), 7.97–8.03 (m, 1H) ppm; 13C NMR (125 MHz, DMSO-d) δ 46.1, 80.3, 115.0, 116.2, 117.8, 123.4, 125.5, 127.5, 127.5, 127.7, 128.4, 128.5, 128.8, 128.9, 129.3, 131.7, 134.8, 142.3, 142.9, 144.0, 146.2, 150.4; MS (m/z, %): 363 (M+, 35); Anal. Calcd for C24H17N3O: C, 79.32; H, 4.72; N, 11.56%. Found: C, 79.45; H, 4.86; N, 11.46%.
4.1.2. 3-(p-tolyl)-3,4-dihydro-2H-benzo[a][1,3]oxazino[5,6-c]phenazine (6b)
Red powder; yield 0.472 g (94%), m.p 249–251 °C; IR (KBr) (νmax cm−1): 3010, 2880, 1572, 1527, 1504, 1468, 1375, 1320, 1209, 1157, 1085, 874, 751, 677 cm–1; 1H NMR (500 MHz, DMSO-d) δ 2.35 (d, J = 0.9 Hz, 3H), 4.82 (s, 2H), 5.64 (s, 2H), 6.80–6.86 (m, 2H), 7.14–7.20 (m, 2H), 7.39–7.48 (m, 2H), 7.49 (ddd, J = 7.5, 5.5, 3.6 Hz, 1H), 7.58–7.65 (m, 2H), 7.82–7.87 (m, 1H), 7.90–7.97 (m, 1H), 7.95–8.02 (m, 1H) ppm; 13C NMR (125 MHz, DMSO-d) δ 20.7, 46.1, 80.4, 115.0, 115.6, 123.3, 125.4, 127.0, 127.4, 127.6, 127.7, 128.8, 128.8, 129.2, 129.3, 129.7, 131.7, 134.8, 142.2, 142.9, 143.9, 144.0, 150.4; MS (m/z, %): 377 (M+, 65); Anal. Calcd for C25H19N3O: C, 79.55; H, 5.07; N, 11.13%. Found: C, 79.50; H, 5.12; N, 11.22%.
4.1.3. 3-(o-tolyl)-3,4-dihydro-2H-benzo[a][1,3]oxazino[5,6-c]phenazine (6c)
Red powder; yield 0.465 g (95%), m.p 252–254 °C; IR (KBr) (νmax cm−1): 2990, 1584, 1540, 1490, 1404, 1330, 1224, 1075, 8835, 751, 708 cm–1; 1H NMR (500 MHz, DMSO-d) δ 2.30 (d, J = 0.7 Hz, 3H), 4.68 (s, 2H), 5.63 (s, 2H), 6.60 (dd, J = 7.5, 1.3 Hz, 1H), 7.10 (ddd, J = 7.5, 6.6, 2.5 Hz, 1H), 7.14–7.21 (m, 2H), 7.39–7.48 (m, 2H), 7.49 (ddd, J = 7.5, 5.5, 3.6 Hz, 1H), 7.58–7.65 (m, 2H), 7.85 (dt, J = 7.3, 1.0 Hz, 1H), 7.90–7.97 (m, 1H), 7.95–8.02 (m, 1H) ppm; 13C NMR (125 MHz, DMSO-d) δ 17.7, 45.9, 81.7, 114.9, 119.9, 123.3, 123.4, 125.4, 127.3, 127.4, 127.6, 127.7, 128.8, 128.8, 129.2, 129.3, 130.1, 131.7, 133.0, 134.8, 142.2, 142.9, 143.97, 144.4, 151.0; MS (m/z, %): 377 (M+, 91); Anal. Calcd for C25H19N3O: C, 79.35; H, 5.69; N, 11.45%. Found: C, 79.38; H, 5.85; N, 11.52%.
4.1.4. 3-(naphthalen-1-yl)-3,4-dihydro-2H-benzo[a][1,3]oxazino[5,6-c]phenazine (6d)
Brown powder; yield 0.411 g (83%), m.p 245–247 °C; IR (KBr) (νmax cm−1): 3030, 2830, 1589, 1534, 1447, 1391, 1336, 1217, 1132, 1078, 846, 753 cm–1; 1H NMR (500 MHz, DMSO-d) δ 4.61 (s, 1H), 4.71 (s, 1H), 5.66 (s, 2H), 7.18 (dd, J = 7.5, 1.6 Hz, 1H), 7.47–7.40 (m, 2H), 7.52–7.44 (m, 4H), 7.65–7.57 (m, 2H), 7.74 (dt, J = 7.5, 1.5 Hz, 1H), 7.86–7.77 (m, 2H), 7.99–7.90 (m, 1H), 8.03–7.96 (m, 1H), 8.21–8.14 (m, 1H) ppm; 13C NMR (125 MHz, DMSO-d) δ 45.9, 81.6, 115.0, 115.3, 123.3, 123.7, 124.2, 125.6, 125.7, 126.1, 126.5, 127.0, 127.3, 127.8, 128.0, 128.1, 128.8, 128.9, 129.0, 129.3, 129.3, 131.7, 134.6, 134.8, 142.2, 142.9, 143.7, 144.1, 150.4; MS (m/z, %): 413 (M+, 65); Anal. Calcd for C28H19N3O: C, 81.34; H, 4.63; N, 10.16%. Found: C, 81.43; H, 4.69; N, 10.25%.
4.1.5. 3-(o-tolyl)-3,4-dihydro-2H-benzo[f][1,3]oxazino[5,6-h]quinoxaline-10,11-dicarbonitrile (6e)
Green jade powder; yield 0.388 g (68%), m.p 223–225 °C; IR (KBr) (νmax cm−1): 1589, 1502, 1417, 1228, 1144, 1054, 899, 753, 671 cm–1; 1H NMR (500 MHz, DMSO-d) δ 2.30 (d, J = 0.6 Hz, 3H), 4.68 (s, 2H), 5.63 (s, 2H), 6.60 (dd, J = 7.5, 1.3 Hz, 1H), 7.10 (ddd, J = 7.5, 6.7, 2.3 Hz, 1H), 7.14–7.22 (m, 2H), 7.39–7.47 (m, 2H), 7.51–7.44 (m, 1H), 7.84 (dd, J = 7.4, 1.4 Hz, 1H) ppm; 13C NMR (125 MHz, DMSO-d) δ 17.7, 45.9, 81.7, 114.0, 114.9, 114.9, 120.0, 123.3, 123.5, 125.6, 126.9, 127.3, 127.5, 127.6, 127.7, 128.4, 130.2, 132.1, 133.0, 137.7, 144.4, 144.5, 149.8; MS (m/z, %): 377 (M+, 78); Anal. Calcd for C23H15N5O: C, 73.20; H, 4.01; N, 18.56%. Found: C, 73.15; H, 4.35; N, 18.49%.
4.1.6. 3-(naphthalen-1-yl)-3,4-dihydro-2H-benzo[f][1,3]oxazino[5,6-h]quinoxaline-10,11-dicarbonitrile (6f)
Green jade; yield 0.395 g (75%), m.p 220–222 °C; IR (KBr) (νmax cm−1): 3035, 2845, 1575, 1495, 1442, 1388, 1226, 1151, 1057, 1053, 1090, 888, 751, 654 cm–1; 1H NMR (500 MHz, DMSO-d) δ 4.61 (s, 1H), 4.71 (s, 1H), 5.66 (s, 2H), 7.18 (dd, J = 7.5, 1.6 Hz, 1H), 7.53–7.41 (m, 6H), 7.74 (dt, J = 7.3, 1.6 Hz, 1H), 7.86–7.77 (m, 2H), 8.20–8.14 (m, 1H), ppm; 13C NMR (125 MHz, DMSO-d) δ 45.9, 81.6, 114.0, 114.5, 115.3, 123.3, 123.7, 124.2, 125.7, 125.7, 126.1, 126.4, 126.6, 127.4, 127.6, 127.8, 128.0, 128.1, 128.4, 132.0, 134.6, 137.6, 143.7, 144.9, 149.8; MS (m/z, %): 413 (M+, 83); Anal. Calcd for C26H15N5O: C, 75.53; H, 3.66; N, 16.94%. Found: C, 75.61; H, 3.59; N, 16.90%.
4.1.7. 3-phenyl-3,4-dihydro-2H-benzo[f][1,3]oxazino[5,6-h]quinoxaline-10,11-dicarbonitrile (6 g)
Green jade; yield 0.398 g (81%), m.p 275–277 °C; IR (KBr) (νmax cm−1): 3000, 2895, 1581, 1493, 1437, 1407, 1286, 1246, 1190, 1090, 898, 855, 771, 689 cm–1; 1H NMR (500 MHz, DMSO-d) δ 4.82 (s, 2H), 5.65 (s, 2H), 6.86–6.82 (m, 2H), 6.92 (tt, J = 7.5, 1.5 Hz, 1H), 7.33–7.26 (m, 2H), 7.52–7.39 (m, 3H), 7.87–7.82 (m, 1H) ppm; 13C NMR (125 MHz, DMSO-d) δ 46.1, 80.3, 114.2, 114.6, 114.7, 116.2, 117.8, 123.5, 125.5, 127.0, 127.5, 127.5, 127.8, 128.4, 129.3, 132.0, 137.7, 144.9, 146.2, 149.8; MS (m/z, %): 363 (M+, 78); Anal. Calcd for C22H13N5O: C, 72.72; H, 3.61; N, 19.27%. Found: C, 72.69; H, 3.56; N, 19.20%.
4.2. The suggested mechanism for heterogeneous catalyst in the reaction
A plausible rational mechanism for the four-part reaction is illustrated under the results in Scheme 3. Based on this mechanism, at first, 2-hydroxynaphthalene-1,4-dione 1 tautomrizes to intermediate 6. The condensation of 4-hydroxy-1,2-naphthoquinone 8 with aromatic 1,2-diamines 2 produces benzo[a]phenazin-5-ol 3. At first, amination reaction occurs between the activated formaldehyde (by coordination with catalyst nanoparticle) and amine (RNH2) followed by H2O elimination provides imine intermediate “A” that is further activated by nano catalyst. “A” is then attacked by 3-hydroxy coumarin to form “B” which further reacts with formaldehyde and eliminate H2O. Next cyclization occurs to form the final product. Activation of the formaldehyde through the weak interaction between the lone pair electron of oxygen and Lewis acidic sites on the surface of nano ZnO-PTA@Fe3O4/EN-MIL-101 makes it susceptible to nucleophilic attack by amine as well as 3-hydroxy coumarin. The size of the nanocatalyst is more important for synthesizing the target product. The smaller size nanocatalyst are more efficient for the reaction because as the particle size decreases surface area for the contact of the reactants increases.
4.3. Antimicrobial activity
The synthesized compounds 6a–h were screened for their anti-microbial activities using standard drugs. The results are summarized in (Scheme 4). First, it prepared concentrations of 250, 500 and 1000 ppm of the samples and mixed 500 µl of each sample with 500 µl of TSB (Trypticase Soy Broth) medium and finally 100 µl of Staphylococcus aureus (Gram-positive bacteria) with the concentration of half McFarland (1.5 × 106 CFU). The samples were incubated at 37 °C for 24 h in a pre-incubated incubator. After 24 h, 100 μl of each sample was removed and cultured on Müller Hinton agar for 24 h at 37 °C and then put inside the incubator. He observed the specimens, with the following results (Scheme 4). The antimicrobial activity data revealed that almost all the compounds 6a–h exhibited promising antibacterial activity against Gram-positive bacteria Staphylococcus aureus. A close look at the structure–activity relationship clearly shows the intrinsic antimicrobial activity associated with the structure of these compounds, which in some cases is reinforced by some substitutions and in some cases decreases.
Scheme 4. Antimicrobial activity of 3-phenyl-3,4-dihydro-2H-benzo[a][1,3]oxazino[5,6-c]phenazine derivatives via Gram-positive bacteria Staphylococcus aureus.
![Scheme 4. Antimicrobial activity of 3-phenyl-3,4-dihydro-2H-benzo[a][1,3]oxazino[5,6-c]phenazine derivatives via Gram-positive bacteria Staphylococcus aureus.](/cms/asset/d83b9361-8a65-4a91-9f88-b6c7e76fdc10/tgcl_a_1800830_f0013_oc.jpg)
Compound 6a showed the highest antibacterial effectiveness against Gram-positive bacteria Staphylococcus aureus. Compounds 6d and 6e were found to exhibit poor but appropriate activity compared to compound 6c. The decrease in antibacterial activity was observed when the electron-donating groups replace in the loop (CH3). Among the product, compound 6e (R = Cl) (electron-withdrawing groups) showed excellent antibacterial activity against Gram-positive bacteria Staphylococcus aureus. The results show that, for example, in combination, at low concentrations, the antibacterial activity is very low and if the concentration is elevated it is increased.
5. Conclusion
In summary, we have observed a multi-Componentcondensation reaction that offers an easy and effective one-pot synthesis of 3-phenyl-3,4-dihydro-2H-benzo[a][1,3]oxazino[5,6-c] phenazine derivatives employing readily available starting materials. These are the first reported compounds in which two new C–N bonds, one C–O bond, and one new C–C bond are formed in a single operation by the pseudo-four-component coupling reaction. The present method has the advantage that the reaction is performed under neutral conditions and the substances can be mixed without any activation or modification. The salient features of this protocol are excellent yields and high purity, short reaction times, easy work-up, and use of an inexpensive and nontoxic catalyst. A magnetically-separable heterogeneous catalyst, nano ZnO-PTA@Fe3O4/EN-MIL-101(Cr) was prepared and detached. The catalyst was confirmed by the aforementioned analyzes of mass and surface area, indicating the presence of iron nanoparticles distributed on MIL-101 crystals, and organic amine molecules in MOF cavities. This catalyst, and design strategy, afford new and green routes to pharmacologically important benzo[a][1,3]oxazino[5,6-c]phenazine derivatives.
Supplemental Material
Download Zip (2.2 MB)Acknowledgment
We gratefully acknowledge financial support from the Research Council of the Islamic Azad University of Yazd.
Disclosure statement
No potential conflict of interest was reported by the author(s).
Notes on contributors
Milad Taheri is currently a postdoctoral fellow in the Research Department at the Islamic Azad University of Yazd. He obtained his doctorate working in the field of organic chemistry by synthesized iron catalysts and green chemistry.
Razieh Mohebat is currently an Associate Professor candidate at the University of Islamic Azad University of Yazd and has been co-chair of the Graduate since 2019. After obtaining his bachelor of science in chemistry at Yazd University in 1990, he began graduate studies in the field of main group chemistry synthesizing based Lewis acid catalysts and photochemistry for organic transformations.
Mohammad Hossein Moslemin obtained her B.Sc. at Tehran University in 1990 followed by her Ph.D. at the University of Tehran in 2000, he began graduate studies in the field of main group chemistry synthesizing nitrogen- sulfur and phosphorus- based Lewis acid catalysts for organic transformations. She currently works at the Department of Chemistry Research, Islamic Azad University of Yazd and organizes the technical programming for its two annual conferences.
Correction Statement
This article has been republished with minor changes. These changes do not impact the academic content of the article.
References
- Chen, W.S.; Juan, C.N.; Wei, K.M. Chemosphere 2005, 60, 1072. doi: 10.1016/j.chemosphere.2005.01.021
- Low, G.K.C.; Mcevoy, S.R.; Matthews, R.W. Environ. Sci. Technol. 1991, 25, 460. doi: 10.1021/es00015a013
- Kumar, S.; Davis, A.P. Water. Environ. Res. 1997, 69, 1238. doi: 10.2175/106143097X125993
- Li, L.; Wu, Q.Y.; Guo, Y.H.; Hu, C.W. Microporous. Mesoporous. Mater. 2005, 87, 1. doi: 10.1016/j.micromeso.2005.07.035
- Li, L.; Li, Y.J.; Ma, Y.; Guo, Y.H. J. Rare. Earths. 2007, 25, 68. doi: 10.1016/S1002-0721(07)60047-7
- Zhang, L.; Huang, T.; Guo, X.J.; Liu, X.Y. Chem. Res. Chinese. Universities. 2010, 26, 1020.
- Feng, C.G.; Shang, H.R. Chem. Res. Chinese. Universities. 2012, 28, 366.
- Wang, Y.J.; Lu, K.C.; Feng, C.G. J. Rare. Earths. 2011, 29, 866. doi: 10.1016/S1002-0721(10)60557-1
- Feng, C.G.; Li, Y.Z.; Liu, X. Chin. J. Chem. 2012, 30, 127. doi: 10.1002/cjoc.201180453
- Feng, C.G.; Xu, G.; Liu, X. J. Rare. Earths. 2013, 31, 44. doi: 10.1016/S1002-0721(12)60232-4
- Zhao, X.Q.; Han, Y.T.; Sun, X.L.; Wang, Y.J. Chin. J. Catal. 2007, 28, 91. doi: 10.1016/S1872-2067(07)60011-4
- Mishra, B.G.; Kumar, D.; Rao, V.S. Catal. Commun. 2006, 7, 457. doi: 10.1016/j.catcom.2006.01.002
- Jermy, B.R.; Pandurangan, A. Catal. Commun. 2006, 7, 921. doi: 10.1016/j.catcom.2006.03.021
- Li, L.; Guo, Y.H.; Zhou, P.; Yu, X.D.; Kang, W.L. Chin. J. Catal. 2005, 26, 209.
- Perchet, G.; Merlina, G.; Revel, J.C.; Hafidi, M.; Richard, C.; Pinelli, E. J. Hazard. Mater. 2009, 166, 284. doi: 10.1016/j.jhazmat.2008.11.013
- Son, H.S.; Lee, S.J.; Cho, I.H.; Zoh, K.D. Chemosphere 2004, 57, 309. doi: 10.1016/j.chemosphere.2004.05.008
- Cropek, D.; Kemme, P.A.; Makarova, O.V.; Chen, L.X.; Rajh, T. J. Phys. Chem. 2008, 112, 8311. doi: 10.1021/jp8009468
- Goswami, P.; Ganguli, J.N. Bull. Mater. Sci. 2012, 35, 889. doi: 10.1007/s12034-012-0364-9
- Xiang, Q.J.; Lv, K.L.; Yu, J.G. Appl. Catal. B. 2010, 96, 557. doi: 10.1016/j.apcatb.2010.03.020
- Liu, S.W.; Yu, J.G. J. Solid. State. Chem. 2008, 181, 1048. doi: 10.1016/j.jssc.2008.01.049
- Li, Z.; Gao, B.; Chen, G.Z.; Mokaya, R.; Sotiropoulos, S.; Puma, G.L. Appl. Catal. B. 2011, 110, 50. doi: 10.1016/j.apcatb.2011.08.023
- Lv, K.L.; Yu, J.G.; Cui, L.Z.; Chen, S.L.; Li, M. J. Alloys. Compd. 2011, 509, 4557. doi: 10.1016/j.jallcom.2011.01.103
- Deltcheff, C.R.; Fournier, M.; Franck, R.; Thouvenot, R. Inorg. Chem. 1983, 22, 207. doi: 10.1021/ic00144a006
- Fan, C.M.; Xue, P.; Sun, Y.P. J. Rare. Earths. 2006, 24, 309. doi: 10.1016/S1002-0721(06)60115-4
- Choi, W.Y.; Termin, A.; Hoffmann, M.R. J. Phys. Chem. 1994, 98, 13669. doi: 10.1021/j100102a038
- O’Sullivan, D.W.; Denzel, J.R.; Prak, D.J.L. Aquat. Geochem. 2010, 16, 491. doi: 10.1007/s10498-010-9089-9
- Cai, H.S.; Liu, G.G.; Lv, W.Y.; Li, X.X.; Yu, L.; Li, D.G. J. Rare. Earths. 2008, 26, 71. doi: 10.1016/S1002-0721(08)60040-X
- Dam, B.; Saha, M.; Jamatia, R.; Pal, A.K. RSC Adv. 2016, 6, 54768–54776. doi: 10.1039/C6RA06376D
- Davod, F.; Kiasat, A.R.; Enjilzadeh, M.; Cheraghchi, M. Lett. Org. Chem. 2016, 13, 58–66. doi: 10.2174/1570178612666151026235326
- Nikoorazm, M.; Ghorbani-Choghamarani, A.; Khanmoradi, M. RSC Adv. 2016, 6, 56549–56561. doi: 10.1039/C6RA09371J
- Vekariya, R.H.; Prajapati, N.P.; Patel, H.D. Synth. Commun. 2016, 46, 197–219. doi: 10.1080/00397911.2015.1114633
- Zhang, H.; Deng, J.; Wu, Y.; Sustain, A.C.S. Chem. Eng. 2017, 5, 658–666.
- Chng, L.L.; Erathodiyil, N.; Ying, J.Y. Acc. Chem. Res. 2013, 46, 1825–1837. doi: 10.1021/ar300197s
- Li, S.; Huo, F. Nanoscale 2015, 7, 7482–7501. doi: 10.1039/C5NR00518C