ABSTRACT
This study represents the green synthesis of silver nanoparticles (AgNPs) using Ipomoea aquatica (Water Spinach) leaf extract, and investigate the cytotoxicity and antimicrobial activities. The Ipomoea aquatica (IA) mediated green synthesized AgNPs (IA-AgNPs) was characterized by ultraviolet–visible (UV–VIS.) spectrophotometry, Fourier transform-infrared (FT-IR) spectroscopy, atomic absorption spectrometry (AAS), X-ray deffractometry (XRD), transmission electron microscopy (TEM), and energy dispersive X-ray (EDX) spectroscopy. The results showed that the material was formed in nano-dimension with particle size in the range between 15–30 nm. The nanoparticles (NPs) were almost spherical in shape and demonstrated excellent material stability. The PC12 cell model in-vitro was used to determine the cytotoxicity of the IA-AgNPs, and for antimicrobial study, E. coli, Salmonella, and Staphylococcus sp. were employed. The cell viability results showed that the NPs did not induce any cytotoxicity until 10 ppm for 48 h of incubation, and it was further confirmed by the lactate dehydrogenase (LDH) assay. Moreover, IA-AgNPs demonstrated good antibacterial activity against three bacteria: E. coli, Salmonella, and Staphylococcus sp. Thus, this study may create an important synthetic route for the production of green-AgNPs and their safe usages, and may also be used to augment the bioactivities of the material with minimum toxicity.
GRAPHICAL ABSTRACT
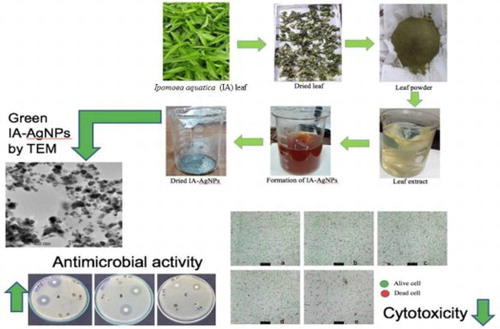
Introduction
Because of unique properties and design combinations compared with conventional materials, the nanomaterials reported to be the materials of the twenty-first century (Citation1). Among the several nanomaterials, silver nanoparticles (AgNPs) are one of the most important and vastly used materials in recent days (Citation2). AgNPs have a wide and excellent physicochemical property (Citation3) and have potential use in the field of chemistry, material science, environmental science, physics, optoelectronics, and biomedical devices (Citation4). It is also chemically highly stable, and has high catalytic and antibacterial activities (Citation5). AgNPs have presently achieved greater attention due to its antimicrobial activities (Citation6). There are a wide range of application of AgNPs as antibacterial agents for disinfecting medical devices, water treatment, and home appliances (Citation7–11). Silver nanocomposite fibers were produced which contains AgNPs inside the fabric (Citation12). The AgNPs containing cotton fibers exhibited high antibacterial (E. coli) activity (Citation12–14). Although it is found that different shapes and sizes of AgNPs have a significant impact on the interaction with organisms, subcellular organelles, cells, and tissues (Citation15). The study revealed that AgNPs also showed a significant amount of antiviral activities against respiratory pathogens, such as adenovirus, parainfluenza, influenza and especially respiratory syncytial virus (RSV) (Citation16).
In recent years, AgNPs have a vast application in cosmetics and paints industry (Citation17, Citation18), and are also widely used in the food storage, health industry, textile coatings, and environmental applications as an anti-bacterial agent (Citation15). Even though it has been used for decades, the reason of toxicity of AgNPs is still not clear (Citation19). To ensure the safe and viable use of AgNPs, it is essential to establish an acceptable method for AgNPs synthesis and that has to be cost effective, less toxic, with more antibacterial activity. It will also be environmentally friendly. A number of chemical and physical methods for AgNPs synthesis have been developed such as photo chemical reduction (Citation20–22), chemical reduction (Citation23–25), electro chemical reduction (Citation26, Citation27), heat evaporation (Citation28), sonochemical process (Citation29), electrochemical and chemical reduction (Citation30–32), but these are not environmentally friendly (Citation33). Toxic chemicals may be present on the surface of chemically synthesized AgNPs and it may have adverse effects during its application in terms of potential toxicity. It may also pollute the environment (Citation34). Moreover, these process are technically laborious and also expensive (Citation35). The toxic effect of AgNPs on different cell lines such as A-549, PC12, HepG-2, and NIH 3T3 was also reported (Citation36). There is also adverse long-term effect of AgNPs on epigenetic imbalance as well as reprogramming of genetic materials (Citation37). Therefore, it is important to induce less toxic and worthy method for AgNPs synthesis, which should have a significant amount of antibacterial activities with minimum toxicity for the safe use of human.
The AgNPs synthesized by green approach is nontoxic and effective antibacterial agent that can function against about 650 types of diseases caused by microorganisms (Citation38). Therefore, the interest on green synthesized AgNPs is increasing (Citation39) and also being projected as future generations antimicrobial agent (Citation40). Nanoparticles synthesis through environment friendly process is known as green synthesis of nanoparticles (Citation41), where the extract from plant material act as reducing agent for silver ion (Ag+) and as encapsulating material to stabilize the newly formed NPs in the synthesis process. This synthesis process is more advantageous than other biological processes as it is less time consuming and inexpensive (Citation42). The green synthesis of NPs is preferred because of environmental friendliness, single step method process, and safety for human therapeutic use (Citation31). This synthesis technique could be a potential future method to replace chemical synthesis of NPs in a conventional way. The plant extract during the green synthesis is very important as it provides bio-redactor agents such as enzymes, flavonoids, proteins, phenolic compounds, alkaloids, polysaccharides, amino acids, and terpenoids (Citation43, Citation44).
Although, several natural material sources have been utilized for the synthesis of AgNPs (Citation45–63), Ipomoea aquatic (IA) leaf extract mediated green synthesis of AgNPs (IA-AgNPs) is not reported yet. Ipomoea aquatica (Water Spinach) is widely available edible green vegetable in the Indian sub-continent. In Bangladesh, it grows round the year both in wild and in cultivation format, thus it is abundant in nature. It is also full of nutrients and antioxidants (Citation64). The leaf of this plant contains adequate amount of potassium, manganese, iron and magnesium. It also contains high amount of alkaloids, reducing sugar, soluble carbohydrate, flavonoids (Citation65), and significant amount of crude lipid, carbohydrate (Citation66), and highest number of flavonoids (Citation67). The biosynthesis of NPs from this plant might bring some special advantages in terms of their properties, bioactivities and may reduce the toxicity of AgNPs.
Therefore, the aim of this work is to synthesize silver nanoparticles using Ipomoea aquatica (Water Spinach) leaf extract (IA-AgNPs) following the green synthesis approach, and to characterize the nanoparticles utilizing UV-Visible spectrophotometry, energy dispersive X-ray (EDX) spectroscopy, X-ray diffractometry (XRD), transmission electron microscope (TEM), Fourier transform infrared spectroscopy (FT-IR), and atomic absorption spectrometer (AAS). Moreover, the cytotoxicity (cell viability and LDH assay) experiments on the IA-AgNPs exposure to PC12 cell were conducted to evaluate the toxicity of the NPs on biological cell in-vitro. And, E. coli, Salmonella, and Staphylococcus sp. were used to assess the antibacterial activity of the newly synthesized IA-AgNPs for evaluating its antibacterial activity.
Materials and methods
Materials
The fresh and healthy leaves of Ipomoea aquatica (water spinach) were purchased from the local market (Kochukhet, Dhaka) of Bangladesh. Analytical grade silver nitrate (AgNO3) with 99.99% purity was procured from Merck Germany. Whatman qualitative 1 filter papers were collected from local supplier. The deionized water prepared in the laboratory was used frequently throughout the whole experiment.
Preparation of Ipomoea aquatica leaf extract (IAE)
First of all, the collected Ipomoea aquatica leaves were separated from the petiole and then washed thoroughly with normal tap water followed by deionized water for three times ensuring the existence of no visible dust (Citation15). The washed leaves were then dried naturally under the sun using a tray covered with aluminum foil paper for 5 days. When the leaves become crunchy, these were blended with the help of a blender to make finely ground leaf powder. The dried leaf powder was collected and stored in an airtight box. After that, 1 g of fine leaf powder was mixed with 50 mL of deionized water in a glass beaker and heated on a hot plate at 80–100°C for 15 min (Citation15). While heating the broth, the mixture was stirred occasionally with a clean glass rod. The mixture was then cooled at room temperature (25–28°C) and filtered using a filter paper. The leaf extract (filtrate) was collected and preserved in a glass bottle at 4–5°C temperature (Citation15) for using in the further experiments.
Synthesis of IA-AgNPs
In this technique, 10 mL of IAE was added drop wise in a 100 mL of 1 mM freshly prepared aqueous AgNO3 solution (Citation15) in a glass beaker under constant magnetic stirring (250 rpm) at room temperature. After complete addition of IAE, the temperature of the solution was raised to about 80°C and the stirring was continued for 1 h. The color of the solution was changed from greenish yellow to dark brown () indicating the initial formation of the expected AgNPs by the reduction of Ag+ ions to Ag0. After cooling down the solution to room temperature, a small portion of the suspension was taken out from the beaker and UV–VIS absorption of the suspension was measured using a UV–VIS. spectrophotometer for the immediate confirmation of the formation of the desired AgNPs. The remaining portion of the suspension was centrifuged at 12,000rpm for 15 min and the process was repeated two times, washing the solid material with deionized water to ensure the removal of all impurities. The solid product was then collected and dried in a small beaker at 80°C for 6 h in an electric oven. The dried solid product was collected in an airtight ember bottle and stored in a desiccator for further analysis.
Characterization techniques for the synthesized IA-AgNPs
UV–VIS spectrophotometric analysis
An UV–VIS spectrophotometer (Model: UV-1650PC, Shimadzu, Japan) was used to identify the primary formation of the expected AgNPs. The dark brown colored sample was diluted for 5 times with deionized water and taken in a small quartz cell for the recording the UV–VIS absorption spectrum at wavelength of 200–800 nm. Prior to measurement, the baseline of the instrument was adjusted using deionized water which was also used as a sample blank in this experiment.
Energy dispersive X-ray (EDX) analysis
The elemental composition of the synthesized material was obtained from EDX spectrometer (Talos F200x, Thermofisher, U.S.A.). The measurement was performed at an accelerating voltage of 200 KV and a current emission of 0.12 micro apm.
X-ray diffraction (XRD) analysis
XRD were used to identify the crystalline phase and particle size of the prepared material. The sample was placed on a grid of XRD instrument and the diffraction patterns were recorded at 40 kV and 40 mA of voltage with Kβ filter 1D for Cu. The experiment was conducted under the following conditions: Length-limiting slit was 10 mm, incident slit box 1.0 mm, scan speed 10° min−1, and scan range 2 ∼ 90°.
Transmission electron microscopy (TEM) analysis
The particle size and crystalline state of the synthesized material were determined by TEM (Model: Talos F200x, Thermo Fisher Scientific, U.S.A.). Prior to the analysis, the sample was mixed with ethanol and ultasonicated to prepare a suspension which was then put on the copper grid and allowed to dry at 25°C (room temperature). Then, the sample was positioned on a holder (specimen) for TEM analysis. The images were taken under 200 KV 0.12 micro apm accelerating voltage.
Fourier transform infrared (FT-IR) analysis
To identify the functional groups that bound distinctively with the expected AgNPs surfaces, FT-IR spectra of both the synthesized expected AgNPs and Ipomoea aquatica leaf extract were recorded. Highly pure KBr powder was mixed with 1% (w/w) samples and then pressed into a sheer slice. FT-IR spectra of the samples were recorded from 500 to 4000 cm−1 with a FT-IR Spectrometer (PerkinElmer Frontier, U.S.A.).
Assessment of stability of IA-AgNPs
The stability of the expected IA-AgNPs was evaluated from the AgNPs release test. Two suspensions of IA-AgNPs of 35 ppm concentration were prepared in deionized water with ultasonication for about 15 min. The one suspension was kept undisturbed for 1 h while another was kept for 24 h at 25°C (room temperature). Both suspensions were then centrifuged for 10 min at 3000 rpm and the clear liquids were collected in previously cleaned and dried 25-mL volumetric flask. The clear liquid solutions were then analyzed for Ag-metal content by AAS (Model: AA240FS, Varian, Australia) following the procedure reported earlier (Citation68). The analysis of samples was performed in air/acetylene flame of 99.99% purity at 4.0 mA hollow cathode lamp current, 328.1 nm light wavelength, and 0.5 nm of slit width for passing the light. The experiment was repeated three times to ensure the reproducibility of the results.
Assessment of cytotoxicity of IA-AgNPs
Cell viability assay
For the culture of PC12 cells, DMEM (Dulbecco’s Modified Eagle Medium) was used with 10% fetal bovine serum (FBS) in an incubator with 5% CO2 at 37°C in 25 cm2 flasks. Thereafter, the desired IA-AgNPs treatment was applied at a dose range from 0 (control) to 10 ppm after 24 h culture followed by a 48 h treatment to be harvested. The trypan blue exclusion method was applied to measure the dead and live cell % following the reported work (Citation69, Citation70) using an automated cell counter (Bio-Rad cell counter CA, U.S.A.). For statistical reproducibility and validity each experiment was repeated at least 4 times.
Lactate dehydrogenase (LDH) activity assay
The LDH activity was measured in the PC12 cell culture medium after the treatment with different concentration (0–10 ppm) of expected IA-AgNPs for 48 h. Cytotoxicity assay nonradioactive kit (Promega, state of Wisconsin, WI, U.S.A.) was used as depicted in the previous work (Citation63, Citation69, Citation71). For validity and reproducibility, the measurement was carried out at least three times.
Antibacterial activity of IA-AgNPs
In this experiment, the disc diffusion method (Citation72) was used for the evaluation of antibacterial activity of the expected IA-AgNPs against two gram negative bacteria: E. coli and Salmonella, and one gram positive bacteria: Staphylococcus sp. The strains were cultured and incubated in Luria Broth (LB) medium which was supplemented with 1.5% tryptone, 0.75% yeast extract, 1.2% sodium chloride at 37°C and equipped with shaking chamber. For treatment with synthesized sample, antibiotics disc was used with/or without antibiotic. The disc was soaked in a 15 μL suspensions of the synthesized sample (10 ppm) and then it was placed along with the antibiotic tetracycline disc (30 μg) at 37°C for 24 h in the culture plate. Experiments were carried out in triplicate to ensure the reproducibility and validity of the measurements. The zone of inhibition was observed to examine the antibiotic activity of the expected IA-AgNPs using a Hi-Media laboratory zone scale (mm).
Results
Characterization of IA-AgNPs
After a visual inspection of the color change for the formation of AgNPs, the UV–VIS spectrum of the colored suspension was recorded for further confirmation of the NPs formation. The maximum UV–VIS absorption peak for our sample was found at 433 nm () which may be considered as the confirmed formation of our desired AgNPs, since UV–VIS absorption of AgNPs occurs at the wavelength range of 400–500 nm due to surface plasma resonance (Citation70). In order to confirm the presence of elemental silver, the solid sample was subjected to EDX analysis which showed the highest percentage of elemental Ag in the sample signaling prominent peak of highest intensity in the EDX spectrum as shown in and thus confirming the existence of silver metal in our synthesized sample. The presence of some other peaks of relatively low intensity for oxygen, nitrogen, and carbon in the spectrum are attributed to the adherence of biomolecules from leaf extract (IAE). To identify the crystalline phase and for further confirmation of the AgNPs formation, the material was subjected to XRD analysis and from the corresponding diffraction patterns (), our synthesized material was identified as cubic structure of silver (DB Card Number: 01-071-4613, Space Group: 225: Fm-3 m, Lattice parameters: a = b = c = 4.09 Å and α = β = γ = 90°). The XRD patterns displayed five characteristic peaks at 38.01°, 44.18°, 64.26°, 77.16°, and 81.29° corresponding to the crystal planes of (111), (200), (220), (311), and (222), respectively which are very similar to our previous study (Citation15, Citation73). In addition to these characteristic peaks, XRD patterns also show some unknown peaks (marked with stars) at 46.11°, 54.72°, 57.31° and 85.52° which may be due to the crystallization of bioorganic phase on the AgNPs surface originating from the leaf extract (Citation74, Citation75). Similar XRD patterns were also reported by many researchers (Citation76–80). The lattice parameters (a, b, c) of the AgNPs were also calculated from the XRD data using Nelson-Riley function (Citation81) and the average value was found to be equal to 4.0964 Å which is well agreed with our standard (DB Card Number: 01-071-4613) and reported value (Citation82, Citation83). Further, the crystallite size of the material was also calculated from the XRD patterns using Debye-Scherrer approximation (Citation84, Citation85) and the value was found as 20.44 nm. The dispersion, aggregation, crystalline state and the size of the IA-AgNPs were examined using TEM as shown in . From TEM analysis, the average particles size of AgNPs was found equal to 18.81 nm (). The surface morphology studied by TEM showed a clear evidence for the metallic crystal formation of AgNPs which was dispersed uniformly with less particles aggregation and the particles are formed in spherical shape (). In order to identify the probable functional groups of the biomolecules present in Ipomoea aquatic leaf extract and adhered to IA-AgNPs as responsible for the formation and stabilization of AgNPs, FTIR spectra of both leaf extract and IA-AgNPs were recorded and are shown in . The strong band at 3432, 2924, 2858, 1696, 1632, 1450, 1384, and 1244 cm−1 are corroborated to the capping agents responsible for the AgNPs formation (). The vibration band at 3422 cm−1 in the spectra is assigned to the presence of O–H stretching in the molecules (Citation15). The C–H stretching vibration bands of aromatic compounds were observed at 2924 and 2858 cm−1 region. The C–C stretching (non-conjugated) bands were assigned for 1696 cm−1. The C–N and C–C stretching at 1632 cm−1 in the spectra are due to the presence of proteins (Citation86). The N–H stretching vibration appeared at 1450 cm−1 are assigned to the amide linkages of the proteins which have been reported to have a significant role in the stabilization and capping of AgNPs (Citation86, Citation87). The N=O symmetry stretching at 1384 cm−1 exemplifies the nitro compounds. The C–N stretching of amines corresponds to the band at 1244 cm−1. The FT-IR data reported in this work are similar to the previous studies (Citation15).
Figure 3. EDX spectrum of IA-AgNPs showing strong metal peak for silver with some relatively weaker peaks for oxygen, nitrogen, and carbon.
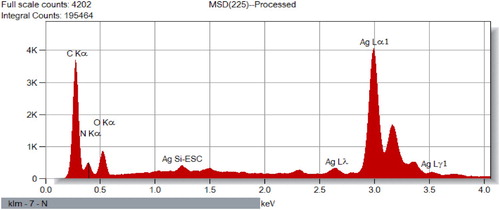
Figure 4. XRD patterns of the synthesized IA-AgNPs. Unassigned peaks (marked with stars) are probably due to the crystallization of bioorganic phase on the AgNPs surface originating from the leaf extract (Citation74, Citation75).
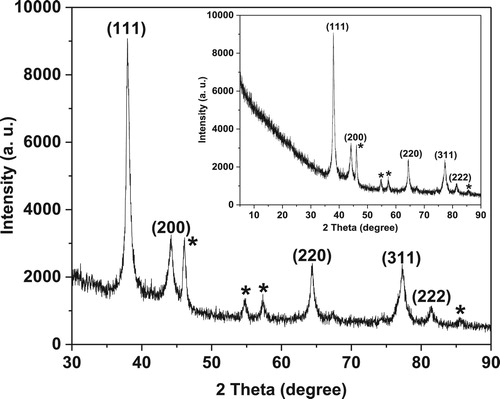
Stability assessment of IA-AgNPs
Initially, considering the 35 ppm aqueous suspension of IA-AgNPs for a leaching time periods of 1 and 24 h at room temperature, we found that the release of Ag+ from IA-AgNPs was only 1.84 and 2.00 ppm after 1 and 24 h, respectively. The release of Ag+ ion from IA-AgNPs was measured by AAS.
Cytotoxicity study of IA-AgNPs
Cell viability and LDH assay
Cytotoxicity of the green synthesized AgNPs was evaluated with a well-known cell line using PC12 cells for toxicity assessment. Cell viability was conducted after exposure of PC12 cells to different concentrations (0–10 ppm) of IA-AgNPs for 48 h incubation (Supplementary Figure 1). Results showed that IA-AgNPs did not induce any cytotoxicity until 10 ppm dose () compared to the control. The cell viability results are further supported by the LDH results of the study. LDH did not increase significantly until 5 ppm of IA-AgNPs dose for 48 h incubation.
Figure 8. (a) Cell viability of PC12 cells exposed to green synthesized AgNPs for 48 h measured by trypan blue exclusion method. (b) The LDH activity in PC12 cell culture medium after exposure to green synthesized AgNPs for 48 h measured by cytotoxicity assay kit. Error bars indicate mean ± SD (n = 4) and star symbol (*) indicate the level of significance (p < 0.05) against control.
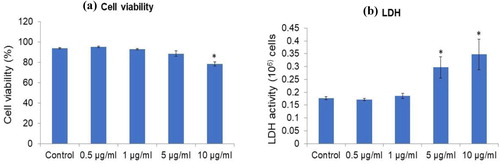
Antibacterial activity studies of IA-AgNPs
Antibacterial activity of the synthesized IA-AgNPs was studied against three bacteria E. coli, Salmonella, and Staphylococcus and the zone of inhibitions (ZOI) imposed by IA-AgNPs were assessed. Using a 10 ppm concentration of IA-AgNPs disc, a clear ZOI was detected for all the three studied bacteria (Supplementary Figure 2). The maximum ZOI for Staphylococcus sp. was about 12.67 ± 1.04 mm, whereas Salmonella and E. coli exhibited a ZOI of 9.10 ± 0.50 mm and 9.25 ± 1.15 mm, respectively (). No effect on bacterial growth was observed for the negative control such as leaf extract and deionized water whereas, the positive control showed a clear ZOI. Thus, the probable existence of the crystalline bioorganic phase (from leaf extract) on the AgNPs surface as observed in the XRD patterns (marked with stars) of the material has no single but may have a combined or synergic effect on the antibacterial activity in the form of IA-AgNPs which is expected.
Discussion
In this work, AgNO3 solution was used as a precursor source of Ag+ ions for the synthesis of IA-AgNPs and Ipomoea aquatica leaf extract was used to reduce the Ag+ to Ag0 as well as to cape or stabilize the AgNPs. The reduction of Ag+ to Ag0 was observed by a definite color change from greenish yellow to dark brown, indicating the formation of AgNPs (Citation88). Furthermore, the UV–VIS spectrophotometry also demonstrated the evidence for the formation of AgNPs showing a characteristic absorption maxima (λmax) at the wavelength of 433 nm. It was the result of the reduction of Ag+ (Citation89, Citation90).
EDX analysis of IA-AgNPs disclosed the elemental characters of AgNPs. The elemental Ag exhibits its signature peak at 3 keV (). Few other peaks for oxygen, nitrogen and carbon were also found which might be originated from Ipomoea aquatica leaf extract. The AgNPs formed crystalline face cantered cubic structure as detected by XRD analysis (). The broad peaks observed in the XRD patterns indicate the formation of AgNPs in nano-dimension which are also confirmed from the obtained calculated values of the particles size by XRD pattern (20.44 nm) and TEM images (18.81 nm). The calculated particles size values from XRD and TEM analysis are very closer to each other and a slight variation of 1.63 nm may be due to the variation in the marking of the distance line in the TEM image. More clearly the magnified TEM images showed the formation of mono dispersed spherical and crystalline AgNPs with a visible bio-coating (). This feature of our synthesized IA-AgNPs may enhance its characteristics properties.
The FT-IR spectroscopic data revealed that the organic functional groups exist in the biomolecules of the leaf extract such as C–N, N–H, OH etc. containing the lone pair electrons in their central electronegative atom are probably the key factors for reducing Ag+ to Ag0 to form stable and encapsulated metallic AgNPs (Citation15). The C–N and N–H functional groups as identified in the FT-IR spectra are due to the presence of amine and secondary structure of proteins in the biomolecules. The N–H group (1450 cm−1) also represents the amide linkages of the proteins. The C–H and C–C groups indicate the existence of aromatic compounds (non-conjugated) in the leaf extract. In addition, the presence of alkaloids, flavones, anthracenes and amide in IAE have a significant role in enhancing the stability and capping of Ag+ to form IA-AgNPs (Citation15, Citation91). It can also be assumed from the FT-IR spectroscopic study that the proteins (secondary structure) that present in the IAE interacted significantly with Ag+ or AgNPs (Citation92).
The results of the leaching test for AgNPs from IA-AgNPs indicate a good stability of AgNPs and may be attributed to the encapsulation of NPs by the biomolecules from leaf extract. These findings are in close agreement with the results obtained from EDX, XRD, TEM and FT-IR which indicated the coexistence of biomolecules from IAE with AgNPs through encapsulation giving the crystalline product IA-AgNPs (as confirmed from XRD).
The stabilized IA-AgNPs were given to the culture medium of PC12 cells (model cell line for toxicity study) to examine its cytotoxicity. It was also established that commercially produced conventional AgNPs exerted cytotoxicity in several cell lines, e.g. HeLa, U937 (Citation93), PC12 cells (Citation63) and human hepatoma cell line (Citation93). In this study, IA-AgNPs showed no significant toxicity in terms of cell viability to PC12 cells up to 5 ppm ((a)). It was found that the cell viability was decreased to 78% with the 10 ppm exposer of IA-AgNPs to the cells. Similarly, Akter et al. (Citation63) reported at a concentration of 12 ppm on PC12 cells, 15–25 nm sized Brassica AgNPs significantly reduced cell viability, whereas Arora et al. (Citation94) disclosed that 7–20 nm sized commercial AgNPs induced toxicity on A431 cell line with a threshold value of 1.56 ppm only. LDH activity analysis which is an indicator of cell membrane damage and toxicity induction, supported the results of cell viability analysis, showing cell membrane damage with a higher concentration of IA-AgNPs (5 and 10 ppm) and no toxicity below 5 ppm of IA-AgNPs ((b)). However, previously by Akter et al. (Citation95), it was reported that commercial AgNPs induced LDH activity increase and toxicity within 1 ppm concentration, which is smaller than the concentration of IA-AgNPs to induce toxicity in PC12 cells. These cytotoxicity results suggested that IA-AgNPs synthesized in this research is less toxic on cells upon exposure and is a better option to be used in consumer products.
Similarly, various biogenic sources mediated green synthesized AgNPs were reported to induce toxicity in different cell lines at different concentrations. For instance, Albizia adianthifolia leaf extract (Citation96), S. grandiflora leaf extract (Citation97) and watermelon rind extract (Citation98) coated AgNPs induced toxicity in different cell lines (A549, MCF-7 and rat splenocytes, respectively) at the concentration of 10, 5 and 4 ppm, respectively; whereas chitosan (Citation99) and fungi (Citation100) based synthesized AgNPs reported to be less toxic than other green synthesized AgNPs. Again, compared to commercial AgNPs (2 ppm) which reported to induce severe toxicity (Citation93), green synthesized IA-AgNPs is less toxic.
Although the exact mechanism for the cytotoxicity of AgNPs is still unknown, it was suggested that AgNPs induce toxicity via the mechanism type of troazan horse, through the NPs ionization inside the cells and initiate toxicity in different pathways (Citation94). Therefore, the stability of NPs is a crucial factor for toxicity induction. Because of less stability, Ag+ ions from commercial NPs easily induce toxicity, whereas photosynthesized/green-synthesized NPs show less toxicity. However, further investigation on the detailed mechanism of AgNPs-induced toxicity is needed. One of the important properties of the green synthesized NPs is its antibacterial activity. For considering as effective antibacterial material, it should exhibit a significant growth inhibition against bacterial species. For instance, in case of zone of inhibition (ZOI), a material must have to produce >9 mm inhibition diameter (Citation95). This study demonstrated a potential antibacterial activity of IA-AgNPs at low dose against gram-positive as well as gram-negative bacteria. The mechanism behind the growth inhibition by green nanoparticle (IA-AgNPs) need to be studied. However, the antibacterial performance of the IA-AgNPs is comparable to other green synthesized AgNPs such as Brassica rapa var. japonica (Citation63), and Cocos nucifera (Citation15) mediated AgNPs.
Conclusion
In this study, a worthy approach for the green synthesis of AgNPs using Ipomoea aquatica (Water Spinach) leaf extract is established for its antimicrobial applications. The shape of the synthesized AgNPs was found almost spherical with particles size ranging from 15 to 30 nm as observed in TEM analysis. The encapsulated IA-AgNPs showed less cytotoxic activity and found nontoxic to PC12 cell probably due to the material stability caused by the presence of capping agent from Ipomoea aquatic. IA-AgNPs showed a potential antibacterial activity at low dose against a range of gram-positive and gram-negative pathogenic bacteria. Thus, Ipomoea aquatica mediated green synthesized IA-AgNPs may be an easy and cost-effective potential candidate for several antibacterial applications. The material might have also wide application in antibiotics (against multidrug resistant bacteria), antiseptic, food processing and packaging, soaps, toothpastes, pharmaceutical, cosmetics, and textiles industries.(101)
Supplemental Material
Download MS Word (1.9 MB)Acknowledgements
Authors are grateful to the laboratory supervisor of Graduate School of Environmental Science, Hokkaido University, Japan and all other laboratories of Jahangirnagar University, Bangladesh Council of Scientific and Industrial Research (BCSIR), and Atomic Energy Center, Dhaka for their cordial support in performing the experiments.
Disclosure statement
No potential conflict of interest was reported by the author(s).
Notes on contributors
Mr. Md. Rokonujaman Khan was born in 1980. He obtained his Bachelor and Master's degrees in Chemistry from National University of Bangladesh. He joined Bangladesh Army's Education Corps in the year of 2007. He has served Bangladesh Military Academy and Military Institute of Science and Technology as a faculty member of Chemistry Department. At present, he is working as an instructor in Bangladesh Army Medical Corps Center and School. He has been perusing PhD under the Department of Environmental Sciences from Jahangirnagar University. He is working on the Green Synthesis of Silver Nanoparticles, Characterization and Bio-activities Assays. His research interest is green chemistry, green synthesis of nanoparticles, wastewater and environmental pollution remediation.
Dr. Sheikh Manjura Hoque is a Chief Scientific Officer and Head of the Materials Science Division, Atomic Energy Centre Dhaka, Bangladesh Atomic Energy Commission (BAEC). She obtained PhD in 2007 from Bangladesh University of Engineering and Technology, (Sandwich PhD Program under the umbrella of International Science Programme (ISP), Uppsala University, Sweden). Thereafter, she worked as a Post-Doctoral Researcher at Department of Diagnostic Radiology, School of Medicine, Yale University, April 2012 to March, 2013 and Materials Engineering Department, Indian Institute of Science, Bangalore, November, 2010 to October, 2011. She is also Senior Research Fellow of the fellowship program of Ministry of Science, Information and Communication Technology, in the Materials Science Division, Atomic Energy Centre, Dhaka, Bangladesh Atomic Energy Commission from April, 2001 to July, 2003. She began work at BAEC in 2003 as a Senior Scientific Officer. She is also the group leader of an ISP-funded research group (BAN-02/2). She achieved numerous awards for her outstanding contribution in material sciences.
Dr. Kaniz Fatima Binte Hossain received her PhD in Environmental Science Development in 2020 from the Graduate School of Environmental Science, Hokkaido University, Japan. She is working as a post-doctoral researcher at the Faculty of Environmental Earth Science, Hokkaido University. She synthesized several remediation technologies for toxic heavy metals using cell culture techniques. She took her BSc (Hons.) and MS in Environmental Sciences degree from Jahangirnagar University, Bangladesh. She is now working on the effects of polyphenols on organisms by using different cell lines (normal cells and cancer cells). She is highly courageous to carry out quality researches and emerging as the young researcher in her time. She has already published a good number of scientific articles as author/co-author in peer reviewed journals having high impact factor.
Mr. Md. Abu Bakar Siddique completed his BSc. (Hons.) and MS (Thesis) in Chemistry from the University of Chittagong, Bangladesh. He got M.Phil. in Chemistry from Bangladesh University of Engineering and Technology (BUET), Dhaka, Bangladesh, in 2018. He is a life member of Bangladesh Chemical Society (BCS), Network of Instrument Technical personnel and User scientists of Bangladesh (NITUB) and a technical assessor of Bangladesh Accreditation Board (BAB). His research areas are Inorganic, Analytical, Environmental, and Nanochemistry, and published many scientific articles in the relevant fields in well-reputed peer-reviewed journals. He has participated in many National and International conferences and has presented his research work. He worked in the implementation of ISO/IEC 17025:2017 quality management system and strongly involved in the relevant research and development works. Currently, he is working as a Senior Scientific Officer at the Institute of National Analytical Research and Service (INARS), Bangladesh Council of Scientific and Industrial Research (BCSIR), Ministry of Science and Technology, Government of the People's Republic of Bangladesh.
Prof. Md. Khabir Uddin is a renowned Environmental Scientist in Bangladesh. He was born in 1964. He has been working in the Department of Environmental Sciences, Jahangirnagar University, Bangladesh since 2000. Before that he had been working in Bangladesh Atomic Energy Commission from 1993 to 2000. He did his JSPS (Japan Society for the Promotion of Science) postdoctoral fellowship from 2001 to 2004. He obtained PhD in Environmental Science from Hokkaido University, Japan in 1999. His research interest revolves around industrial wastewater treatment and waste to energy conversion technology. He has more than one hundred publications in reputed journals in home and abroad journals. He has supervised research work nearly 60 MS and 6 PhD students. He was also a visiting professor of The University of Shiga Prefecture, Japan and adjunct faculty member of many private and public universities in Bangladesh. Prof. Uddin has been awarded National Environmental Award-2014 for his outstanding contribution in Environmental development in Bangladesh.
Dr. Md. Mostafizur Rahman is an associate professor of Environmental Sciences at the Department of Environmental Sciences, Jahangirnagar University, Bangladesh. His research is focused on ecotoxicology, environmental pollution remediation, and exposure risk assessment for health and environment. Particularly, his research theme covers the area of monitoring of emerging organic micro pollutants in environmental matrices and their health implications around the urban area of Bangladesh, water security issues under changing climate and total sanitation to achieve SDG6. He is MEXT (Japan), EPLC (Korea) and CONEM (Norway) fellow/member. Dr. Rahman awarded his PhD in Environmental Sciences from Hokkaido University, Japan. He received the prestigious Matsuno Environment Award-2019 for his outstanding achievements in research. He published more than seventy-five (75) scientific articles in peer-review international journals. His current research activities are available in the following websites;
ResearchGate: <https://www.researchgate.net/profile/Md_Mostafizur_Rahman4>
Google Scholar: <https://scholar.google.com/citations?pli=1&user=nzljxJcAAAAJ>
Publons: <https://publons.com/researcher/3103840/md-mostafizur-rahman/>
References
- Camargo, P.H.C.; Satyanarayana, K.G.; Wypych, F. Mater. Res. 2009, 12, 1–39.
- Edwards-Jones, V. Lett. Appl. Microbiol. 2009, 49, 147–152.
- Khamhaengpol, A.; Siri, S. Mater. Lett. 2017, 192, 72–75.
- Jamil, M.; Murtaza, G.; Mehmood, A. Mater. Lett. 2015, 153, 10–13.
- Hinojos-Márquez, E.A.; López-Esparza, J.; Espinosa-Cristóbal, L.F.; Donohue-Cornejo, A.; Reyes-López, S.Y. Indust. Eng. Chem. Res. 2016, 55 (49), 12532–12538.
- Ivan, S.; Branka Salopek, S. J. Colloid Interface Sci. 2004, 275, 177–182.
- Bosetti, M.; Masse, A.; Tobin, E.; Cannas, M. Biomaterials 2002, 23, 887–892.
- Cho, M.; Chung, H.; Choi, W.; Yoon, J. Appl. Environ. Microbiol. 2005, 71 (1), 270–275.
- Gupta, A.; Silver, S. Nat. Biotechnol. 1998, 16, 888.
- Jain, P.; Pradeep, T. Biotechnol. Bioeng. 2005, 90 (1), 59.
- Li, Q.; Mahendra, S.; Lyon, D.; Brunet, L.; Liga, M.; Li, D.; Alvarez, P. Water Res. 2008, 42, 4591.
- Yeo, S.; Lee, H.; Jeong, S. J. Mater. Sci. 2003, 38, 2143.
- Duran, N.; Marcarto, P.; De Souza, G.; Alves, O.; Esposito, E. J. Biomed. Nanotechnol. 2007, 3, 203.
- Chen, C.; Chiang, C. Mater. Lett. 2008, 62, 3607.
- Uddin, A.K.M.R.; Siddique, M.A.B.; Rahman, F.; Ullah, A.K.M.A.; Khan, R. J. Inorg. Organomet. Polym. 2020, 30, 1–12.
- Dorothea, M.; Maria, A.; Janice, S.; Teodora, I.; Yue, Q.; Antonella, C.; Roberto, P.G. Viruses 2019, 11 (8), 732.
- Hoet, P.H.M.; Bruske-Hohlfeld, I.; Salata, O.V. J. Nanobiotechnology 2004, 2, 12.
- Park, E.J.; Yi, J.; Kim, Y.; Choi, K.; Park, K. Toxicol. In Vitro 2010, 24, 872–878.
- Kholoud, M.M.A.E.-N.; Ala’a, E.; Abdulrhman, A.-W.; Ammar, R.A.A. Arab. J. Chem. 2010, 3, 135–140.
- Sandor, K.; Janos, T.; Gyorgy, D.; Lajos, D.; Miklos, Z. J. Colloid Interface Sci. 2000, 229, 550–553.
- Vorobyova, S.A.; Lesnikovich, A.I.; Sobal, N.S. Colloids Surf. A. 1999, 152, 375–379.
- Mallick, K.; Witcombb, M.J.; Scurrella, M.S. Mater. Chem. Phys. 2005, 90, 221–224.
- Yu, D.G. Colloids Surf. B. 2007, 59, 171–178.
- Yiwei, T.; You, W.; Lei, J.; Daoben, Z. J. Colloid Interface Sci. 2002, 249, 336–345.
- Petit, C.; Lixon, P.; Pileni, M.P. J. Phys. Chem. 1993, 97, 12974–12983.
- Liu, Y.C.; Lin, L.H. Electrochem. Commun. 2004, 6, 1163–1168.
- Sandmann, G.; Dietz, H.; Plieth, W.J. Electroanal. Chem. 2000, 491, 78–86.
- Smetana, A.B.; Klabunde, K.J.; Sorensen, C.M. J. Colloid Interface Sci. 2005, 284, 521–526.
- Hangxun, X.; Brad, W.Z.; Kenneth, S. Chem. Soc. Rev. 2013, 42, 2555–2567.
- Starowicz, M.; Stypula, B.; Banas, J. Electrochem. Commun. 2006, 8, 227–230.
- Guzmán, M.G.; Dille, J.; Godet, S. Int. J. Chem. Biomol. Eng. 2009, 2, 104–111.
- Mao, C.; Xiang, Y.; Liu, X.; Cui, Z.; Yang, X.; Yeung, K.W.; Pan, H.; Wang, X.; Chu, P.K.; Wu, S. ACS. Nano. 2017, 11, 9010–9021.
- Dwivedi, A.D.; Gopal, K. Colloids Surf. A Physicochem. Eng. Asp. 2010, 369, 27–33.
- Suman, T.Y.; Radhika, R.S.R.; Kanchana, A.; Elizabeth, S.B. Colloids Surf. B. Biointerfaces 2013, 106, 74–78.
- Gopinath, V.; MubarakAli, D.; Priyadarshini, S.; Meera Priyadharsshini, N.; Thajuddin, N.; Velusamy, P. Colloids Surf. B Biointerfaces 2012, 96, 69–74.
- Sambale, F.; Wagner, S.; Stahl, F.; Khaydarov, R.R.; Scheper, T.; Bahnemann, D.J. Nanomaterials 2015, 9, 136765.
- Mytych, J.; Zebrowski, J.; Lewinska, A.; Wnuk, M. Mol. Neurobiol. 2016, 54, 1–16.
- Jeong, S.H.; Yeo, S.Y.; Yi, S.C. J. Mater. Sci. 2005, 40, 5407–5411.
- Choi, O.; Deng, K.K.; Kim, N.J.; Ross, L.; Surampalli, R.Y.; Hu, Z. Water Res. 2008, 42, 3066–3074.
- Rai, M.; Yadav, A.; Gade, A. Biotechnol. Adv. 2009, 27, 76–83.
- Sumit, S.L.; Nayak, P.L. Int. J. Sci. Innov. Discov. 2012, 3, 325–350.
- Valli, J.S.; Vaseeharan, B. Mater. Lett. 2012, 82, 171–173.
- Rajan, R.; Chandran, K.; Harper, S.L.; Yun, S.I.; Kalaichelvan, P.T. Indust. Crops Prod. 2015, 70, 356–373.
- Rauwel, P.; Rauwel, E.; Ferdov, S.; Singh, M.P. Adv. Mater. Sci. Eng. 2015, 2015, 624394.
- Bar, H.; Bhui, D.K.; Sahoo, G.P.; Sarkar, P.; Pyne, S.; Misra, A. Colloids Surf. 2009, 348, 212–216.
- Parashar, U.K.; Saxena, P.S.; Srivastava, A. Digest J. Nanomater. Biol. 2009, 4, 159–166.
- Bar, H.; Bhui, D.K.; Sahoo, G.P.; Sarkar, P.; De, S.P.; Misra, A. Colloids Surf. A. 2009, 339, 134–139.
- Kasthuri, J.; Veerapandian, S.; Rajendiran, N. Colloids Surf. A. 2009, 68, 55–60.
- Wang, Y.; He, X.; Wang, K.; Zhang, X.; Tan, W. Colloids Surf. B. 2009, 73, 75–79.
- Parashar, V.; Parashar, R.; Sharma, B.; Pandey, A.C. Digest J. Nanomater. Biostruct. 2009, 4, 45–50.
- Shankar, S.S.; Rai, A.; Ahmad, A.; Sastry, M.J. Colloid Interface Sci. 2004, 275, 496–502.
- Shankar, S.S.; Ahmad, A.; Sastry, M. Biotechnol. Prog. 2003, 19, 1627–1631.
- Dubey, M.; Bhadauria, S.; Kushwah, B.S. Digest J. Nanomater. Biostruct. 2009, 4, 537–543.
- Begum, N.A.; Mondal, S.; Basu, S.; Laskar, R.A.; Mandal, D. Colloids Surf. B. 2009, 71, 113–118.
- Krishnaraj, C.; Jagan, E.G.; Rajasekar, S.; Selvakumar, P.; Kalaichelvan, P.T.; Mohan, N. Colloids Surf. B. 2010, 76, 50–56.
- Philip, D. Physica. E. 2010, 42, 1417–1424.
- Sithara, R.; Selvakumar, P.; Arun, C.; Anandan, S.; Sivashanmugam, P. J. Adv. Res. 2017, 8, 561–568.
- Yu, C.; Tang, J.; Liu, X.; Ren, X.; Zhen, M.; Wang, L. Materials 2019, 12, 189.
- Gopinath, K.; Kumaraguru, S.; Bhakyaraj, K.; Mohan, S.; Venkatesh, K.S.; Esakkirajan, M.; Kaleeswarran, P.; Alharbi, N.S.; Kadaikunnan, S.; Govindarajan, M.; Benelli, G. Microb. Pathog. 2016, 101, 1–11.
- Huang, J.; Li, Q.; Sun, D.; Lu, Y.; Su, Y.; Yang, X.; Yinghua, L.; Yuanbo, S.; Huixuan, W.; Yuanpeng, W.; Wenyao, S.; Ning, H.; Jinqing, H.; Cuixue, C. Nanotechnology 2007, 18 (11), 105104.
- Khalil, M.M.H.; Ismail, E.H.; Baghdady, Z.E.K.; Mohamed, D. Arab. J. Chem. 2014, 7, 1131–1139.
- Dada, A.O.; Inyinbor, A.A.; Bello, I.E.I.; Bello, O.M.; Oluyori, A.P.; Akande, T.A.A.; Okunola, A.A.; Dada, O. Peer J. 2018, 6, 5865.
- Akter, M.; Rahman, M.M.; Ullah, A.K.M.A.; Sikder, M.T.; Hosokawa, T.; Saito, T.; Kurasaki, M. J. Inorg. Organomet. Polym. 2018, 28, 1483–1493.
- Kala, A.; Prakash, J. Int. J. Food Properties. 2004, 7, 659–669.
- Igwenyi, I.O.; Offor, C.E.; Ajah, D.A.; Nwankwo, O.C.; Ukaomah, J.I.; Aja, P.M. Int. J. Pharma. Bio. Sci. 2011, 2, 593–598.
- Umar, K.J.; Hassan, L.G.; Dangoggo, S.M.; Ladan, M.J. J. Appl. Sci. 2007, 7 (6), 803–809.
- Huang, D.J.; Chen, H.J.; Lin, C.D.; Lin, Y.H. Bot. Bull. Acad. Sin. 2005, 46, 99–106.
- Ahsan, M.A.; Satter, F.; Siddique, M.A.B.; Akbor, M.A.; Ahmed, S.; Shajahan, M.; Khan, R. Environ. Monit. Assess. 2019, 191 (9), 575.
- Rahman, M.M.; Lopez, R.U.; Sikder, M.T.; Tan, G.; Hosokawa, T.; Saito, T.; Kurasaki, M. Chemosphere 2018, 196, 453–466.
- Ashraf, J.M.; Ansari, M.A.; Khan, H.M.; Alzohairy, A.M.A.; Choi, I. Sci. Rep. 2016, 6, 20414.
- Hossain, K.F.B.; Rahman, M.M.; Sikder, M.T.; Hosokawa, T.; Saito, T.; Kurasaki, M. Ecotoxicol. Environ. Saf. 2020, 192, 110238.
- Saravanan, M.; Vemu, A.K.; Barik, S.K. Colloids Surf. B Biointerfac. 2011, 88, 325–331.
- Hsueh, Y.H.; Lin, K.S.; Ke, W.J.; Hsieh, C.T.; Chiang, C.L. PLoS One 2015, 10 (12), 0144306.
- Vanaja, M.; Annadurai, G. Appl. Nanosci. 2013, 3 (3), 217–223.
- Awwad, A.M.; Salem, N.M.; Abdeen, A.O. Int. J. Ind. Chem. 2013, 4 (1), 29.
- Zonooz, N.F.; Salouti, M. Sci. Iran. 2011, 18 (6), 1631–1635.
- Roopan, S.M.; Madhumitha, R.G.; Rahuman, A.A.; Kamaraj, C.; Bharathi, A.; Surendra, T.V. Indust. Crop Prod. 2013, 43, 631–635.
- Aravinthan, A.; Govarthanan, M.; Selvam, K.; Praburaman, L.; Selvankumar, T.; Balamurugan, R.; Kamala-Kannan, S.; Kim, J.H. Int. J. Nanomed. 2015, 10, 1977.
- Chauhan, R.; Reddy, A.; Abraham, J. Appl. Nanosci. 2015, 5 (1), 63–71.
- Salehi, S.; Shandiz, S.A.S.; Ghanbar, F.; Darvish, M.R.; Ardestani, M.S.; Mirzaie, A.; Jafari, M. Int. J. Nanomed. 2016, 11, 1835.
- Ullah, A.K.M.A.; Kibria, A.K.M.F.; Akter, M.; Khan, M.N.I.; Tareq, A.R.M.; Firoz, S.H. Water Conserv. Sci. Eng. 2017, 1, 249–256.
- Sagar, G.; Ashok, B. Eur. Exp. Biol. 2012, 2, 1654–1658.
- Ullah, A.K.M.A.; Kabir, M.F.; Akter, M.; Tamanna, A.N.; Hossain, A.; Tareq, A.R.M.; Khan, M.N.I.; Kibria, A.F.; Kurasaki, M.; Rahman, M.M. RSC Adv. 2018, 8, 37176–37183.
- Ullah, A.K.M.A.; Kibria, A.K.M.F.; Akter, M.; Khan, M.N.I.; Maksud, M.A.; Jahan, R.A.; Firoz, S.H. J. Saudi Chem. Soc. 2017, 21, 830–836.
- Alam, H.M.B.; Das, R.; Shajahan, M.; Ullah, A.K.M.A.; Kibria, A.K.M.F. Int. J. Hydrog. Energy 2018, 43, 1998–2008.
- Prakash, P.; Gnanaprakasam, P.; Emmanuel, R.; Arokiyaraj, S.; Saravanan, M. Colloids Surf. B Biointerfaces 2013, 108, 255–259.
- Niraimathi, K.L.; Sudha, V.; Lavanya, R.; Brindha, P. Colloids Surf. B Biointerfaces 2013, 102, 288–291.
- Adil, M.; Khan, T.; Aasim, M.; Khan, A.A.; Ashraf, M. AMB Expr. 2019, 9, 75.
- Rahman, M.M.; Ukiana, J.; Lopez, R.U.; Sikder, M.T.; Saito, T.; Kurasaki, M. Chem. Biol. Interact. 2017, 269, 41–49.
- Vadiraj, K.T.; Belagali, S.L. J. Mater. Sci. 2016, 27, 2885–2889.
- Dong-Jiann, H.; Hsien-Jung, C.; Chun-Der, L.; Yaw-Huei, L. Bot. Bull. Acad. Sin. 2005, 46, 99–106.
- Kumari, J.; Mamta, B.; Ajeet, S. J. Radiat. Res. Appl. Sci. 2015, 9 (3), 217–227.
- Kim, S.; Choi, J.E.; Choi, J.; Chung, K.H.; Park, K.; Jongheop, Y.; Doug-Young, R. Toxicol. In Vitro 2009, 23 (6), 1076–1084.
- Arora, S.; Jain, J.; Rajwade, J.M.; Paknikar, K.M. Toxicol. Lett. 2008, 179, 93–100.
- Akter, M.; Sikder, M.T.; Rahman, M.M.; Ullah, A.K.M.A.; Hossain, K.F.B.; Banik, S.; Hosokawa, T.; Saito, T.; Kurasaki, M. J. Adv. Res. 2018, 9, 1–16.
- Gengan, R.M.; Anand, K.; Phulukdaree, A.; Chuturgoon, A. Colloids Surf. B Biointerfaces 2013, 105, 87–91.
- Kaba, S.I.; Egorova, E.M. Nanotechnol. Sci. Appl. 2015, 8, 19–29.
- Velmurugan, P.; Hong, S.C.; Aravinthan, A.; Jang, S.H.; Yi, P.I.; Song, Y.C.; Jung, E.S.; Park, J.S.; Sivakumar, S. Arab. J. Sci. Eng. 2017, 42, 201–208.
- Noghabi, M.P.; Parizadeh, M.R.; Mobarhan, M.G.; Taherzadeh, D.; Darroudi, H.A.H.M. J. Mol. Struct. 2017, 1146, 499–503.
- Saraswati, S.S.; Kundu, G.C.; Ahmad, A. Spectrochim. Acta A Mol. Spectrosc. 2013, 114, 144–147.