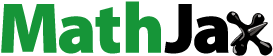
ABSTRACT
In the current paper, NiO-NPs were synthesized through a sol–gel method by the usage of a biocompatible polymer known as Arabic gum (GA) as a stabilizing agent. The structural, optical, and magnetic properties of the obtained product was investigated by the application of TGA/DTA, FT-IR, UV-Vis, XRD, FESEM/EDX, and VSM analyzes. The FESEM images of NiO-NPs displayed its spherical shape and average size of about 59 nm. The antimicrobial activity of NiO-NPs was investigated against Gram-positive bacteria including Staphylococcus aureus, Bacillus subtilis and Gram-negative bacteria including Escherichia coli, Pseudomonas aeruginosa. The achieved findings indicated that all of the bacterial resistance was attributed to NiO-NPs, except for the case of Bacillus subtilis bacteria that did not grow at the concentration of 10 mM. We also evaluated the photocatalytic activity of nanoparticles in regards to the MB degradation and observed a photocatalytic degradation efficiency of about 80% throughout the results. The cytotoxicity of NiO-NPs has evaluated towards the cancer U87MG cell and normal Nervous Neurospheres (NCs) cells lines by means of MTT method. Detailed in vitro biological activities revealed the significant therapeutic potential of NiO-NPs. This product was able to exhibit potent cytotoxicity against U87MG cancer cells (IC50: 37.84 μg mL−1).
GRAPHICAL ABSTRACT
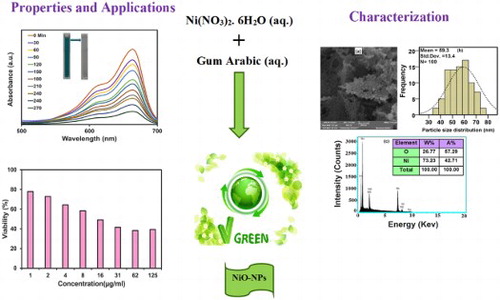
1. Introduction
Due to the individual physical–chemical features of nanoparticles in inhibiting bacterial growth, an enhancement has occurred in performing researches on nanoparticles and their applications as antimicrobial agents (Citation1). In recent years, various metal nanoparticles or metal oxides, including copper, gold (Citation2), and NiO were utilized in the fields of medicine, biology, and pharmacy (Citation3). The biological application of nanoparticles relies on their antibacterial activity towards different types of bacteria (Citation4, Citation5). According to previous studies, the antibacterial influence of nanoparticles can result in altering the their shape, morphology, size, and functional group (Citation6). Instead, the usage of inorganic nanoparticles can multiply their application due to their stability and safety after being matched to organic nanoparticles (Citation7). Some of the essential properties of metal oxide nanoparticles are their antibacterial activity against gram-negative bacteria and gram-positive bacteria (Citation8, Citation9), in which nanoparticles cause them to be inactivated by binding to biological macromolecules and consequently lead to the annihilation of bacteria, viruses, and fungi (Citation10, Citation11). Among the metal oxide nanoparticles, NiO is a p-type semiconductor with electrochemical, catalytic, photocatalytic, and antibacterial properties (Citation12, Citation13), while containing an strong antimicrobial effect against a wide range of microorganisms, even at deficient concentrations (Citation14). The detected features of NiO-NPs include high chemical stability, low dielectric constant, rechargeable batteries, high catalytic activity, and intelligent drugs, as well as being applicable as an energy storage in supercapacitors. This product is also capable of exhibiting antibacterial, cytotoxic, and superparamagnetic qualities that can be exerted throughout the preparation of MRI images (Citation14–17). Several approaches are used to perform the green synthesis of nanoparticles such as sol–gel (Citation18, Citation19), microemulsion (Citation20), hydrothermal (Citation21), chemical vapor deposition, co-precipitation (Citation22), microwave (Citation23), sonochemical (Citation24), pulsed laser ablation, and high-energy ball milling (Citation25). Among these techniques, the procedure of sol–gel can offer many benefits including being cheap, requiring a low-temperature synthesis, the simplicity of its tools, producing high purity products, and not being harmful to the environment (Citation26). In the present study, we performed a green synthesis of NiO-NPs through a sol–gel method and had the optical and structural features of the resultant examined, while employing natural materials throughout the process to minimize their hazardous effects on humans (Citation27). Lately, the application of polymer materials for the production of nanomaterials has become quiet frequent due to their low costs and environmental compatibility (Citation28). On the other hand, the utilization of natural biopolymer that does not cause any cytotoxicity can provide the opportunity of using these nanoparticles as green and safe materials in the medical applications (Citation29–31). Arabic gum biopolymer is known to be non-toxic and biocompatible, which can be exerted for the production NiO-NPs (Citation32). Today, performed researches on metal oxide nanoparticles and their application in cancer diagnosis and treatment are increasing (Citation33). In the current paper, the cytotoxicity effect of NiO-NPs was investigated on human glioblastoma cells lines (U87MG) (Citation34). Throughout recent years, very few articles have been published on the topic of synthesizing approaches or the assessment of NiO-NPs photocatalytic influence on eliminating the organic dyes of aqueous environments. In 2018, Abdul Rahman et al., reported a degradation percentage of 72% for MB (Citation35), while in 2018, Ezhilarasi et al., reported an efficiency removal of 80% (Citation36). Moreover, in 2018, Ramesh et al., reported the degradation percentages of 87.2% and 70.2% for the cases of RB-5 and MB textile dyes, respectively (Citation37). In the past years, many reports investigated the topics of green synthesis and biological activities of different nanoparticles such as cerium oxide nanoparticles (Citation38), zinc oxide nanoparticles (Citation39), and silver nanoparticles (Citation40). Also, biological activities of the different drugs investigated on U87MG cell lines. In 2013, Mickiewicz et al., reported the anticancer activity of temozolomide on U87MG cell line (Citation41). In 2020, Abbaset al., reported the cytotoxic effects of cinnamaldehyde (CA) on U87MG cells alone and in combination with doxorubicin (DOX) (IC50 of CA = 11.6 μg/mL and IC50 of DOX = 5 μg/mL) (Citation42). In our previous work, we described a synthesizing route and investigated the biological activity of NiO nanoparticles by the utilization of tragacanth gum (Citation43). Angel Ezhilarasi et al. presented a report on the green synthesis and biological activities of NiO nanoparticles using Aegle marmelos leaf extract (Citation36). In the current study, NiO-NPs were synthesized through a sol–gel method that involves the usage of Arabic gum as the stabilizer, Nickel (II) nitrate hexahydrate (Ni(NO3)2.6H2O) salt as the supplier of nickel, and water as the solvent, whole lacking the application of any surfactants or reducing agents in order to have a low-cost and friendly process to the nature. Furthermore, this green synthesizing procedure is simple and does not require high temperatures, pressures, or harmful reducers. The novelty of this study would the proposal of an Arabic gum polymer as a unique and natural stabilizer in the synthesis of NiO-NPs, which can improve the photocatalytic properties and biological activities. This procedure has many advantages such as being nontoxic, versatile, low cost, environment friendly, simplest, homogeneity, reliability, reproducibility, and controllability, while being reusable for the synthesis of other metal oxides (Citation44–46). Therefore, the chief goal of the current paper was to improve an economical and eco-friendly way to perform the biosynthesis of NiO-NPs via the application of Arabic gum as the stabilizing agent to prevent nanoparticle aggregation, evaluate the photocatalytic activity of nanoparticles upon MB dye removal, and achieve a higher degradation efficiency of 82%. Moreover, we also performed an evaluation on the antimicrobial activity of NiO-NPs against gram-negative bacteria including Escherichia coli and Pseudomonas aeruginos, as well as gram-positive bacteria including Staphylococcus aureus and Bacillus subtilis. Additionally, the cytotoxicity effects of NiO-NPs were investigated on cancer U87MG cell and normal Nervous Neurospheres (NCs) cell lines by the means of the MTT assay.
2. Experimental
2.1. Materials
Arabic gum polymer was acquired from a local health food store. Nickel (II) nitrate hexahydrate (Ni(NO3)2.6H2O) salt (Merck chemicals, 99% purity), functioning as the precursors of nickel, was purchased from Germany Merck. Distilled water was also used as the solvent. All of the chemicals were exerted without further purification. UV-Vis spectrophotometer (model, Japan) was employed to measure the adsorption, while the application of a UV-A lamp (11 W) was considered to carry out the photocatalyst tests.
2.2. Synthesis of NiO-NPs
In this study, NiO-NPs were synthesized in different sizes through the application of sol–gel technique and natural materials. To achieve this goal, Ni (NO3)2.6H2O and Arabic gum were utilized to function as the source of nickel element and control agent of particle size respectively. Initially, 3.634 g of (Ni (NO3)2.6H2O) salt was dissolved in 100 mL of distilled water and stirred at room temperature for 20 min. Simultaneously, 1 g of Arabic gum was (has been) dissolved in 50 mL of distilled water and stirred at 50°C for 100 min until a clear and colorless solution was gained. Then, nickel nitrate solution was slowly added to the Arabic gum solution. In order to concentrate the mixture solution as the next step, it was stirred at 80°C for 12 h and converted to an aerogel. To complete the drying process and remove the solvent, the aerogel was placed in an oven and heated at 180°C for 2 h. The resultant of this process was a dried gel that functioned as a catalyst precursor, which was calcinated at the temperatures of 300, 400, 500, and 600°C for 2 h, to obtain the black colored powder of NiO-NPs. The applied biosynthesis plan of our product is exhibited in .
The biosynthesis mechanism of metal oxide nanoparticles by the usage of Arabic gum is displayed in . Green chemistry accommodates a set of ‘sustainable’ principles to reduce or eliminate the application of hazardous substances throughout the synthesizing procedures. Arabic gum can stand as an appropriate substance in green synthesis due to being available, renewable, and abled to act as a stabilizer agent in the synthesis of metal oxide nanoparticles. In conformity to observations, the hydroxyl and carboxyl groups of Arabic gum can be oxidized. Arabic gum is a proper stabilizing substance for the green synthesis of nanoparticles, which can offer a comparable efficiency to that of the hazardous polymers or surfactants.
2.3. Anticancer activity studies
2.3.1. Cell culture
In the current research, the cytotoxicity influences of NiO-NPs were examined on normal nervous neurospheres (NCs) cell and cancer human glioblastoma cancer (U87MG) cells lines. The cells cultured in a DMEM medium were detected to be enhanced by 10% FBS (Serum fetal bovine) and 1% antibiotics (Penicillin, Streptomycin), which were then incubated at 37°C in a 95% humidified air and 5% CO2 atmosphere. Three days after the cell culturing process, the product was passaged by 0/25 Trypsin enzyme and 0/02 EDTA solution (Ethylenediaminetetraacetic acid).
2.3.2. In-vitro cytotoxic activity NiO-NPs by MTT assay
The MTT (3-(4,5-dimethylthiazol-2-yl)−2,5-diphenyltetrazolium bromide) assay was applied to investigated the cytotoxicity effects of NiO-NPs on normal nervous neurospheres (NCs) cell and cancer human glioblastoma cancer (U87MG) cell lines (Citation47, Citation48). As it is known, the utilization of spectrophotometry can measure the produced color. In the current paper, cell lines were cultured in a DMEM medium with 10% FBS and incubated at 37°C in a 95% humidified air and 5% CO2. In outline, the U87 cells were cultured in 96-well plates and incubated for 24 h. Afterwards, the cells were treated by several concentrations of NiO-NPs (1, 2, 4, 8, 16, 31, 62, and 125 μg mL−1) for 48 h. As the next step, 200 μL of the solution, containing 180 μL of fresh culture medium and 20 μL of MTT, was transferred to the media of each well; then, 100 µL of DMSO was added to each well. The absorption of different concentrations was read by the employment of an ELISA reader at the specified wavelength (540 nm) (Citation49).
2.4. Antibacterial activity studies
The Antimicrobial effect of NiO-NPs was assessed through an agar diffusion method against gram-negative bacteria Escherichia coli and Pseudomonas aeruginosa and gram-positive bacteria Staphylococcus aureus and Bacillus subtilis (Citation8). First, the solution of NiO-NPs was prepared and diluted in 9 tubes, each containing 4 mL of H2O. Then, about 4 mL of each dilution was appended to the plates that included the agar culture medium in a way that the dishes would be set at varying concentrations (including 10, 5, 2.5, 1.25, 0.625, 0.312, 0.156, 0.078, and 0.039 mM). Subsequently, the bacterial suspension of each bacteria was placed in various places of the plates by the usage of a sterile swab to be incubated afterwards at 37°C for 24 h.
2.5. Photocatalytic activity study
The photocatalytic activity of NiO-NPs was assessed through the utilization of methylene blue (MB) solution at ∼ 663 nm. Initially, a photocatalytic investigation was completed in the lack of UVA light, and the degradation percentage was assessed by Equation (1), which was about 17%. In the following, photocatalytic decomposition was performed under UVA light exposure (11W) (Citation50). For every state, 10 mg L−1 of NiO compounds were dispersed in 100 mL of MB (10−5 M) and stirred for 1 h. The initial pH of the solution was controlled to be about 9 throughout all of the performed experiments. The photocatalytic test was done under UV-A light irradiation as the UV-A lamp was placed vertically at a distance of 20 cm from the solution in the container for a period of 30 min. Once the specified time intervals had passed, we filtered about 2 mL of the suspension and determined its absorbance to be at 663 nm. The degradation percentage of MB was estimated by the application of Equation (1) (Citation51).
(1)
(1) In which
and
Stand as the absorbance ago of radiation and at time t, respectively.
3. Result and discussion
3.1. Characterization
In general, different methods were used to evaluate the surface properties including FT-IR, UV–Vis, FESEM, EDX, XRD, VSM, and TGA/DTA. Furthermore, the antimicrobial outcome of NiO-NPs was studied in opposition to Gram-negative bacteria Escherichia coli and Pseudomonas aeruginosa and Gram-positive bacteria Staphylococcus aureus and Bacillus subtilis. In the following, the photocatalytic activity of nanoparticles was evaluated for MB degradation while their cytotoxicity effects were examined on cancer U87MG cell and normal NCs cell lines by the means of MTT method.
3.2. TGA/DTA analysis
The thermal behavior of a dried gel sample was examined by the usage of TGA/DTA analysis within an air atmosphere through the speed of 10°C/min at a temperature range of 20–900°C, which is displayed in . The TGA diagram of Ni(OH)2 dry gel displayed the occurrence of a weight loss in 3 steps. The first weight loss of about 45% happened from 94 to 161°C, and an endothermic peak was detected at approximately 113°C, which was related to the evaporation of H2O on the surface and precursor building (Citation52). The second and third stages of weight losses reported to be 10% and 16%, occurred in the temperature range of 161–316°C and 316–347°C, respectively. These losses were attributed to the degradation of organic compounds and the removal of nitrates, as well as the decomposition of nickel complexes (Citation53). In conformity to the DTA chart, the exothermic peaks are associated with these particular developments. There were no signs of any specific weight losses throughout the range of 350–900°C.
3.3. XRD study
The X-ray diffraction plan of NiO-NPs in 2θ = 20–80° is presented in . The diffraction peaks observed at the angles of 36.9, 42.8, 62.6, 75.3, and 79.2◦ were related to the (111), (200), (220), (311), and (222) crystal planes, respectively. By comparing the XRD pattern of synthesized nanoparticles with the standard sample, the concluded diffraction pattern (JCPDS card No 04-835) (Citation54) determined that the synthesized NiO-NPs contained a cubic crystalline structure (FCC) and a space group of (Fm3 m) (Citation55). The size of nanoparticles was assessed by Debye-Scherrer formula (Equation (2)).
(2)
(2)
where D is the particle size (nm), k = 0.94 stands as the fixed number, would be the wavelength (0.154 nm),
represents the Full With at Half Maximum of peak (rad), and
refers to the angle (degree). The sizes of nanoparticles at 300, 400, 500, and 600°C were detected to be about 39, 39, 43, and 62 nm, respectively, and therefore, it could be confirmed that the calcined nanoparticles were in the right arrangement with FESEM images (D = 59 nm). Furthermore, exhibits the occurrence of an increase in the calcination temperature that resulted in heightening the intensity of peaks and decreasing their width. An increase in the strength of mountains is indicative of an increase in the crystalline degree of nanoparticles, while a decrease in their width is suggestive of an increase in crystalline particle size that can be caused by the particle joining and their growth at high temperatures (Citation56). Obtained results of NiO-NPs XRD pattern are presented in .
Table 1. Obtained results XRD pattern of NiO-NPs.
3.4. FTIR analysis
displays the FT-IR chart of nanoparticles within the limit of 4000–400 cm−1 at the various temperatures of 300, 400, 500, and 600°C. Accordingly, the appearance of several absorption peaks can be perceived that are associated with the O-H bond of H2O. The peak observed at 3480 cm−1 was correlated to the stretching vibrations that belonged to the O-H band of H2O. Another peak was detected in the range of 1400–1600 cm−1, associated with O-H bending vibrations. Due to the existence of CO2 molecules in the air, the absorption peak observed at the range of 2360-2390 cm−1 could be related to CO2 molecules (Citation57). The stretching vibrations related to the Ni-O band were reported to be approximately in the area of 421 cm−1 (Citation58).
3.5. UV-Vis analyze
(a) exhibits the UV-Vis chart of NiO-NPs within the limit of 200–800 nm at the various temperatures of 300, 400, 500, and 600°C, which displays the maximum optical absorption to be at 280, 284 nm, 312, and 322 nm for the temperature range of 300–600°C (Citation39). The Eg for different temperatures of calcinated nanoparticles can be calculated by the usage of Equation (3), where hν stands for the photon energy, α refers to the absorption amount, B would be an amount, and n is equaled to 2 for a direct bandgap while being equated to 0.5 in the case of indirect bandgap. By utilizing the appointed equation (Equation (3)) and for n = 2, since NiO is recognized as a direct bandgap semiconductor, the curve (αhν)n in terms of hν was plotted for nanoparticles by the usage of UV-Vis spectrum and the Tauc equation ((b)) (Citation59). Position intersection tangent line on the section linear this curve with the x-axis, which were obtained through an extrapolation method, displayed the energy gap at a range of 3.05–3.30 eV for the synthesized nanoparticles. The energy band gap of NiO nanoparticles is demonstrated in .
(3)
(3)
Table 2. The obtained results of UV-Vis spectra.
3.6. FESEM/EDX/PSA images
(a–c) displays the FESEM image, PSA, and EDX of NiO-NPs that were calcinated at the temperature of 500°C and observed in the scale of 200 nm. Accordingly, the morphology of particles was spherical with a little amount of agglomeration, yet appearing on the scale of nanometer (Citation5). The average nanoparticle size was determined to be around 59 nm (Citation60). By the attained findings from EDX illustrated in (c), the synthesized NiO-NPs are consisted of oxygen and nickel elements (Citation61).
3.7. VSM analysis
Considering how NiO-NPs belong to the category of magnetic nanoparticles, it is essential to study their magnetic properties. In the current paper, the magnetic nature of NiO nanoparticles was examined throughout the magnetic field of +20,000 to–20,000 Oersted by the application of VSM analysis. demonstrates the hysteresis curve of nanoparticles, which were calcinated at the temperature of 400°C in the magnetic field (H) of +20,000 to −20,000 Oersted. Given this curve and intensity of magnetization, it can be concluded that the nanoparticles were superparamagnetic. Due to the absence of hysteresis loop throughout the M-H curves and coercive field (Hc = 0), while the remanent magnetization (Mr = 0) equaled to zero and reached a state of full saturation, it can be indicated that the nanoparticles contained superparamagnetic properties (Citation62). Furthermore, the saturation magnetization (Ms) value of this compound was about 23 emu/g, which stands as a proof of the strong magnetic properties of this compound (Citation63).
3.8. Evaluation of cytotoxicity activity using NiO-NPs
Today, research into nanoparticles for the diagnosis and treatment of cancer is increasing. In this study, we evaluated the cytotoxic effect of synthesized NiO-NPs by the usage of Arabic gum polymer on normal CNs cell lines and cancer U87MG cell lines; the obtained results are exhibited in (a,b). CNs and U87MG cell lines were treated with different concentrations of NiO-NPs for 48 h and cell viability and IC50 value were calculated by MTT method (Citation9). The cytotoxicity outcomes were reported using Equation (4) as the nanoparticles concentration caused a growth inhibition of 50% in the cancer cells. The obtained results indicated that NiO-NPs were capable of eliminating 50% of U87MG cells at the concentration of 16 μg mL−1, meaning that the nanoparticles destroyed about 50% of cells, which was considered as the Half-maximal inhibitory concentration (IC50). The MTT results showed that the cytotoxicity effect of NiO-NPs is dose-dependent on concentration and can significantly reduce the cell survival. We described the basis of viability versus the concentration (μg mL−1), which was determined through Equation (4) (Citation47). In conformity to the results of this study, the lethal effect of synthesized NiO-NPs by green method on cancer cells was greater than normal cells. Therefore, the exertion of these nanoparticles can be considered in the treatment of cancer.
(4)
(4)
3.9. Evaluation antibacterial activity of NiO-NPs
The antibacterial activity of NiO-NPs, synthesized by the usage of Arabic gum, was assessed by the means of an agar diffusion method against two Gram-negative bacteria including Escherichia coli and Pseudomonas aeruginosa and two Gram-positive bacteria including Staphylococcus aureus and Bacillus subtilis (Citation8). The obtained findings indicated that all of the bacteria displayed signs of growth in all of the involved concentrations, meaning that the bacterial resistance was relative to NiO-NPs, except for the case of Bacillus subtilis bacteria that did not grow at the concentration of 10 Mm. The outcomes of nanoparticles antibacterial activity are presented in .
3.10. Evaluation photocatalytic activity of NiO-NPs
In this study, NiO-NPs were used as the photocatalyst for the degradation of methylene blue (MB) dye in the absence of UVA light and under UVA light irradiation (Citation37, Citation64). Initially, a photocatalytic investigation was completed in the lack of UVA light, and the degradation percentage was assessed by Equation (1), which was about 17% and can be perceived in (a). In addition, the photocatalytic activity graph of NiO-NPs under UVA light irradiation in regards to MB dye decomposition is exhibited in (b), while the degradation percentage of MB was detected to be about 82% after 270 min.
4. Conclusion
In the present paper, NiO-NPs was produced via a sol–gel procedure by the usage of Arabic-gum as a polymerization agent at different calcination temperatures. The produced nanoparticles were characterization by the exertion of FT-IR, UV-Vis, XRD, FESEM / EDX, TGA/DTA, and VSM procedures. Their morphology and crystallite size was confirmed through the XRD and FESEM studies. The bandgap energy of NiO-NPs was observed to have a range of 3.00–3.30 eV. The photocatalytic results confirmed the photocatalyst role of NiO-NPs throughout the MB degradation process under UVA irradiation, while the obtained degradation percentage was about 80%. Moreover, the antimicrobial activity of this product was evaluated against gram-negative bacteria and gram-positive bacteria. According to the results, every experimented bacteria displayed a resistant behavior relative to NiO-NPs except for the case of Bacillus subtilis bacteria that did not grow at the concentration of 10 mM. Through the employment of MTT assay, the cytotoxicity of NiO-NPs was assessed on cancer U87MG cell and normal CNs cell lines.
Acknowledgments
This study is the results of a research project and thesis presented for a Post-Doctoral student (Dr. Z. Sabouri).
Disclosure statement
No potential conflict of interest was reported by the author(s).
Additional information
Funding
References
- Rios, J.; Recio, M. Medicinal Plants and Antimicrobial Activity. J. Ethnopharmacol. 2005, 100 (1–2), 80–84.
- Nadeem, M.; Abbasi, B.H.; Younas, M.; Ahmad, W.; Khan, T. A Review of the Green Syntheses and Anti-Microbial Applications of Gold Nanoparticles. Green Chem. Lett. Rev. 2017, 10 (4), 216–227.
- Singh, J.; Dutta, T.; Kim, K.-H.; Rawat, M.; Samddar, P.; Kumar, P. ‘Green’ Synthesis of Metals and Their Oxide Nanoparticles: Applications for Environmental Remediation. J. Nanobiotechnology 2018, 16 (1), 84.
- Taylor, E.; Webster, T. J. Reducing Infections Through Nanotechnology. In The Twenty-first International Offshore and Polar Engineering Conference: International Society of Offshore and Polar Engineers, 2011.
- Khan, S.A.; Shahid, S.; Hanif, S.; Almoallim, H.S.; Alharbi, S.A.; Sellami, H. Green Synthesis of Chromium Oxide Nanoparticles for Antibacterial, Antioxidant Anticancer, and Biocompatibility Activities. Int. J. Mol. Sci. 2021, 22 (2), 502.
- Narayanan, K.B.; Sakthivel, N. Green Synthesis of Biogenic Metal Nanoparticles by Terrestrial and Aquatic Phototrophic and Heterotrophic Eukaryotes and Biocompatible Agents. Adv. Colloid Interface Sci. 2011, 169 (2), 59–79.
- Taghavi, S.M.; Momenpour, M.; Azarian, M.; Ahmadian, M.; Souri, F.; Taghavi, S.A.; Sadeghain, M.; Karchani, M. Effects of Nanoparticles on the Environment and Outdoor Workplaces. Electron. Physician 2013, 5 (4), 706.
- Khatami, M.; Sharifi, I.; Nobre, M.A.; Zafarnia, N.; Aflatoonian, M.R. Waste-Grass-Mediated Green Synthesis of Silver Nanoparticles and Evaluation of Their Anticancer, Antifungal and Antibacterial Activity. Green Chem. Lett. Rev. 2018, 11 (2), 125–134.
- Khan, S.A.; Shahid, S.; Ayaz, A.; Alkahtani, J.; Elshikh, M.S.; Riaz, T. Phytomolecules-Coated NiO Nanoparticles Synthesis Using Abutilon Indicum Leaf Extract: Antioxidant, Antibacterial, and Anticancer Activities. Int. J. Nanomed. 2021, 16, 1757.
- Azam, A.; Ahmed, A.S.; Oves, M.; Khan, M.S.; Habib, S.S.; Memic, A. Antimicrobial Activity of Metal Oxide Nanoparticles Against Gram-Positive and Gram-Negative Bacteria: A Comparative Study. Int. J. Nanomed. 2012, 7, 6003.
- Helan, V.; Prince, J.J.; Al-Dhabi, N.A.; Arasu, M.V.; Ayeshamariam, A.; Madhumitha, G.; Roopan, S.M.; Jayachandran, M. Neem Leaves Mediated Preparation of NiO Nanoparticles and Its Magnetization, Coercivity and Antibacterial Analysis. Results Phys. 2016, 6, 712–718.
- Aplan, F.; Spearin, E.; Simkovich, G. Flotation of the p-Type Semiconductor NiO as a Function of Point Defects. Colloids Surf. 1980, 1 (3–4), 361–371.
- Roopan, S.M.; Elango, G.; Priya, D.D.; Asharani, I.; Kishore, B.; Vinayprabhakar, S.; Pragatheshwaran, N.; Mohanraj, K.; Harshpriya, R.; Shanavas, S. Sunlight Mediated Photocatalytic Degradation of Organic Pollutants by Statistical Optimization of Green Synthesized NiO NPs as Catalyst. J. Mol. Liq. 2019, 293, 111509.
- Gondal, M.; Dastageer, M.; Khalil, A. Nano-NiO as a Photocatalyst in Antimicrobial Activity of Infected Water Using Laser Induced Photo-Catalysis. In Electronics, Communications and Photonics Conference (SIECPC), 2011 Saudi International: IEEE; 2011. pp 1–5.
- Shanaj, B.; John, X. Effect of Calcination Time on Structural, Optical and Antimicrobial Properties of Nickel Oxide Nanoparticles. J. Theor. Comput. Sci. 2016, 3 (2), 149–159.
- Khan, S.T.; Ahamed, M.; Alhadlaq, H.A.; Musarrat, J.; Al-Khedhairy, A. Comparative Effectiveness of NiCl2, Ni-and NiO-NPs in Controlling Oral Bacterial Growth and Biofilm Formation on Oral Surfaces. Arch. Oral Biol. 2013, 58 (12), 1804–1811.
- Zhang, Y.; Mahdavi, B.; Mohammadhosseini, M.; Rezaei-Seresht, E.; Paydarfard, S.; Qorbani, M.; Karimian, M.; Abbasi, N.; Ghaneialvar, H.; Karimi, E. Green Synthesis of NiO Nanoparticles Using Calendula Officinalis Extract: Chemical Charactrization, Antioxidant, Cytotoxicity, and Anti-Esophageal Carcinoma Properties. Arab. J. Chem. 2021, 14, 103105.
- Turgut, G.; Sonmez, E.; Duman, S. Determination of Certain Sol-Gel Growth Parameters of Nickel Oxide Films. Ceram. Int. 2015, 41 (2, Part B), 2976–2989.
- Fakhari, S.; Jamzad, M.; Kabiri Fard, H. Green Synthesis of Zinc Oxide Nanoparticles: A Comparison. Green Chem. Lett. Rev. 2019, 12 (1), 19–24.
- Du, Y.; Wang, W.; Li, X.; Zhao, J.; Ma, J.; Liu, Y.; Lu, G. Preparation of NiO Nanoparticles in Microemulsion and Its Gas Sensing Performance. Mater. Lett. 2012, 68, 168–170.
- Ma, J.; Lian, J.; Duan, X.; Liu, X.; Zheng, W. α-Fe2O3: Hydrothermal Synthesis, Magnetic and Electrochemical Properties. J. Phys. Chem. C 2010, 114 (24), 10671–10676.
- Rahman, M.A.; Radhakrishnan, R.; Gopalakrishnan, R. Structural, Optical, Magnetic and Antibacterial Properties of Nd Doped NiO Nanoparticles Prepared by Co-Precipitation Method. J. Alloys Compd. 2018, 742, 421–429.
- Lai, T.-L.; Shu, Y.-Y.; Huang, G.-L.; Lee, C.-C.; Wang, C.-B. Microwave-Assisted and Liquid Oxidation Combination Techniques for the Preparation of Nickel Oxide Nanoparticles. J. Alloys Compd. 2008, 450 (1-2), 318–322.
- Rahman, M.M.; Khan, S.B.; Jamal, A.; Faisal, M.; Asiri, A.M. Fabrication of Highly Sensitive Acetone Sensor Based on Sonochemically Prepared As-Grown Ag2O Nanostructures. Chem. Eng. J. 2012, 192, 122–128.
- Rane AV, Kanny K, Abitha V, Thomas S. Methods for Synthesis of Nanoparticles and Fabrication Of Nanocomposites. In Synthesis of Inorganic Nanomaterials. Elsevier; 2018. pp. 121–139.
- Darroudi, M.; Sabouri, Z.; Kazemi Oskuee, R.; Khorsand Zak, A.; Kargar, H.; Hamid, M.H.N.A. Sol–gel Synthesis, Characterization, and Neurotoxicity Effect of Zinc Oxide Nanoparticles Using Gum Tragacanth. Ceram. Int. 2013, 39 (8), 9195–9199.
- Jeevanandam, J.; Barhoum, A.; Chan, Y.S.; Dufresne, A.; Danquah, M.K. Review on Nanoparticles and Nanostructured Materials: History, Sources, Toxicity and Regulations. Beilstein J. Nanotechnol. 2018, 9 (1), 1050–1074.
- Mohan S, Oluwafemi OS, Kalarikkal N, Thomas S, Songca SP. Biopolymers – Application in Nanoscience and Nanotechnology. In Recent Advances in Biopolymers. IntechOpen; 2016. pp. 47–66.
- Nitta, S.; Numata, K. Biopolymer-Based Nanoparticles for Drug/Gene Delivery and Tissue Engineering. Int. J. Mol. Sci. 2013, 14 (1), 1629–1654.
- Nadeem, M.; Tungmunnithum, D.; Hano, C.; Abbasi, B.H.; Hashmi, S.S.; Ahmad, W.; Zahir, A. The Current Trends in the Green Syntheses of Titanium Oxide Nanoparticles and Their Applications. Green Chem. Lett. Rev. 2018, 11 (4), 492–502.
- Shahid, S.; Fatima, U.; Sajjad, R.; Khan, S. Bioinspired Nanotheranostic Agent: Zinc Oxide; Green Synthesis and Biomedical Potential. Dig. J. Nanomater. Biostruct. 2019, 14, 1023–1031.
- Barik, P.; Bhattacharjee, A.; Roy, M. Preparation, Characterization and Electrical Study of Gum Arabic/ZnO Nanocomposites. Bull. Mater. Sci. 2015, 38 (6), 1609–1616.
- Ostrovsky, S.; Kazimirsky, G.; Gedanken, A.; Brodie, C. Selective Cytotoxic Effect of ZnO Nanoparticles on Glioma Cells. Nano Res. 2009, 2 (11), 882–890.
- Barabadi, H.; Ovais, M.; Shinwari, Z.K.; Saravanan, M. Anti-Cancer Green Bionanomaterials: Present Status and Future Prospects. Green Chem. Lett. Rev. 2017, 10 (4), 285–314.
- Abdul, R.; Ayob, M.; Radiman, S. Enhanced Photo Catalytic Performance of NiO-Decorated ZnO Nano Whiskers for Methylene Blue Degradation. J. Nanotechnol 2014, 2014, 212694.
- Ezhilarasi, A.A.; Vijaya, J.J.; Kaviyarasu, K.; Kennedy, L.J.; Ramalingam, R.J.; Al-Lohedan, H.A. Green Synthesis of NiO Nanoparticles Using Aegle Marmelos Leaf Extract for the Evaluation of In-Vitro Cytotoxicity, Antibacterial and Photocatalytic Properties. J. Photochem. Photobiol. B: Biol. 2018, 180, 39–50.
- Ramesh, M.; Rao, M.P.C.; Anandan, S.; Nagaraja, H. Adsorption and Photocatalytic Properties of NiO Nanoparticles Synthesized via a Thermal Decomposition Process. J. Mater. Res. 2018, 33 (5), 601–610.
- Sabouri, Z.; Sabouri, M.; Amiri, M.S.; Khatami, M.; Darroudi, M. Plant-Based Synthesis of Cerium Oxide Nanoparticles Using Rheum Turkestanicum Extract and Evaluation of Their Cytotoxicity and Photocatalytic Properties. Mater. Technol. 2020, 2020, 1–14.
- Darroudi, M.; Sabouri, Z.; Kazemi Oskuee, R.; Khorsand Zak, A.; Kargar, H.; Abd Hamid, M.H.N. Green Chemistry Approach for the Synthesis of ZnO Nanopowders and Their Cytotoxic Effects. Ceram. Int. 2014, 40 (3), 4827–4831.
- Khandannasab, N.; Sabouri, Z.; Ghazal, S.; Darroudi, M. Green-Based Synthesis of Mixed-Phase Silver Nanoparticles as an Effective Photocatalyst and Investigation of Their Antibacterial Properties. J. Mol. Struct. 2019, 1203, 127411.
- Markiewicz-Żukowska, R.; Borawska, M.H.; Fiedorowicz, A.; Naliwajko, S.K.; Sawicka, D.; Car, H. Propolis Changes the Anticancer Activity of Temozolomide in U87MG Human Glioblastoma Cell Line. BMC Complement. Altern. Med. 2013, 13 (1), 1–9.
- Abbasi, A.; Hajialyani, M.; Hosseinzadeh, L.; Jalilian, F.; Yaghmaei, P.; Navid, S.J.; Motamed, H. Evaluation of the Cytotoxic and Apoptogenic Effects of Cinnamaldehyde on U87MG Cells Alone and in Combination with Doxorubicin. Res. Pharm. Sci. 2020, 15 (1), 26.
- Sabouri, Z.; Akbari, A.; Hosseini, H.A.; Khatami, M.; Darroudi, M. Tragacanth-Mediate Synthesis of NiO Nanosheets for Cytotoxicity and Photocatalytic Degradation of Organic Dyes. Bioprocess Biosyst. Eng. 2020, 43, 1209–1218.
- Khan, S.A.; Shahid, S.; Shahid, B.; Fatima, U.; Abbasi, S.A. Green Synthesis of MnO Nanoparticles Using Abutilon Indicum Leaf Extract for Biological, Photocatalytic, and Adsorption Activities. Biomolecules 2020, 10 (5), 785.
- Khan, S.A.; Shahid, S.; Lee, C.-S. Green Synthesis of Gold and Silver Nanoparticles Using Leaf Extract of Clerodendrum Inerme; Characterization, Antimicrobial, and Antioxidant Activities. Biomolecules 2020, 10 (6), 835.
- Ijaz, F.; Shahid, S.; Khan, S.A.; Ahmad, W.; Zaman, S. Green Synthesis of Copper Oxide Nanoparticles Using Abutilon Indicum Leaf Extract: Antimicrobial, Antioxidant and Photocatalytic Dye Degradation Activities. Trop. J. Pharm. Res. 2017, 16 (4), 743–753.
- van Meerloo J, Kaspers GJ, Cloos J. Cell Sensitivity Assays: The MTT Assay. In. Cancer Cell Culture., Springer: London; 2011. pp. 237–245
- Ahamed, M.; Ali, D.; Alhadlaq, H.A.; Akhtar, M.J. Nickel Oxide Nanoparticles Exert Cytotoxicity via Oxidative Stress and Induce Apoptotic Response in Human Liver Cells (HepG2). Chemosphere 2013, 93 (10), 2514–2522.
- Kora, A.J.; Sashidhar, R. Biogenic Silver Nanoparticles Synthesized with Rhamnogalacturonan Gum: Antibacterial Activity, Cytotoxicity and Its Mode of Action. Arab. J. Chem. 2018, 11 (3), 313–323.
- Rong, X.; Qiu, F.; Zhang, C.; Fu, L.; Wang, Y.; Yang, D. Adsorption–Photodegradation Synergetic Removal of Methylene Blue from Aqueous Solution by NiO/Graphene Oxide Nanocomposite. Powder Technol. 2015, 275, 322–328.
- Soofivand, F.; Salavati-Niasari, M. Step Synthesis and Photocatalytic Activity of NiO/Graphene Nanocomposite Under UV and Visible Light as an Effective Photocatalyst. J. Photochem. Photobiol. A: Chem. 2017, 337, 44–53.
- Ziegler-Borowska, M.; Chełminiak, D.; Kaczmarek, H. Thermal Stability of Magnetic Nanoparticles Coated by Blends of Modified Chitosan and Poly (Quaternary Ammonium) Salt. J. Therm. Anal. Calorim. 2015, 119 (1), 499–506.
- Mallick, G.; Labh, J.; Giri, L.; Pandey, A.C.; Karna, S.P. Facile Synthesis and Electron Transport Properties of NiO Nanostructures Investigated by Scanning Tunneling Microscopy. AIP Adv. 2017, 7 (8), 085007.
- Dumala, N.; Mangalampalli, B.; Chinde, S.; Kumari, S.I.; Mahoob, M.; Rahman, M.F.; Grover, P. Genotoxicity Study of Nickel Oxide Nanoparticles in Female Wistar Rats After Acute Oral Exposure. Mutagenesis 2017, 32 (4), 417–427.
- Jahromi, S.P.; Huang, N.; Muhamad, M.; Lim, H. Green Gelatine-Assisted Sol–Gel Synthesis of Ultrasmall Nickel Oxide Nanoparticles. Ceram. Int. 2013, 39 (4), 3909–3914.
- Soleimani, E.; Mohammadi, M. Synthesis, Characterization and Properties of Polystyrene/NiO Nanocomposites. J. Mater. Sci.: Mater. Electron 2018, 29, 9494–9508.
- Kargar, H.; Ghasemi, F.; Darroudi, M. Bioorganic Polymer-Based Synthesis of Cerium Oxide Nanoparticles and Their Cell Viability Assays. Ceram. Int. 2015, 41 (1), 1589–1594.
- Saleem, S.; Ahmed, B.; Khan, M.S.; Al-Shaeri, M.; Musarrat, J. Inhibition of Growth and Biofilm Formation of Clinical Bacterial Isolates by NiO Nanoparticles Synthesized from Eucalyptus Globulus Plants. Microb. Pathog. 2017, 111, 375–387.
- Hussain, M.M.; Rahman, M.M.; Asiri, A.M. Ultrasensitive and Selective 4-Aminophenol Chemical Sensor Development Based on Nickel Oxide Nanoparticles Decorated Carbon Nanotube Nanocomposites for Green Environment. J. Environ. Sci. 2017, 53, 27–38.
- Sabouri, Z.; Akbari, A.; Hosseini, H.A.; Darroudi, M. Facile Green Synthesis of NiO Nanoparticles and Investigation of Dye Degradation and Cytotoxicity Effects. J. Mol. Struct. 2018, 53, 37–38.
- Parsaee, Z. Synthesis of Novel Amperometric Urea-Sensor Using Hybrid Synthesized NiO-NPs/GO Modified GCE in Aqueous Solution of Cetrimonium Bromide. Ultrason. Sonochemistry 2018, 44, 120–128.
- Rahdar, A.; Aliahmad, M.; Azizi, Y. NiO Nanoparticles: Synthesis and Characterization. J. Nanostructures 2015, 5 (2), 145–151.
- Jabbar, A.H. Study Magnetic Properties and Synthesis with Characterization of Nickel Oxide (NiO) Nanoparticles. J. Nanostructures 2015, 6 (8), 94–98.
- Senobari, S.; Nezamzadeh-Ejhieh, A. A p-n Junction NiO-CdS Nanoparticles with Enhanced Photocatalytic Activity: A Response Surface Methodology Study. J. Mol. Liq. 2018, 257, 173–183.