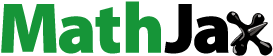
ABSTRACT
Luminescent nanoparticles synthesized via bio-based protocols that generate nanoparticles having different chemical compositions along with other functionalities (size and morphology) have received huge attention. We have focused our research on gold sulfide nanoparticles (Au2S NPs) and have biosynthesized these NPs using the fungus Humicola sp. The nanoparticles were characterized by Transmission Electron Microscopy, which showed spherical morphology of Au2S. UV–Visible-NIR spectrophotometry, luminescence spectrophotometry, Selected Area Electron Diffraction, Energy Dispersive Analysis of X-rays, and X-ray diffraction were performed. FTIR confirmed that the fungal metabolites including biomolecules secreted in the reaction medium are primarily responsible for nanoparticle synthesis and stabilization. The fungus reduced the precursor solution (HAuCl4 and Na2SO3) and at the same time capped them with secreted biomolecules. The anti-leishmanial activity of Au2S NPs was determined against L. donovani promastigote (Ag83 strain). Au2S NPs displayed less cytotoxicity towards both normal and cancer (Daudi, ZR-75-1) cell lines. Hemocompatibility was determined via hemolysis assays. This novel fungal-based system demonstrates an economical and environmentally benign process for biosynthesis of Au2S nanoparticles which may find application in bioimaging and labeling studies.
GRAPHICAL ABSTRACT
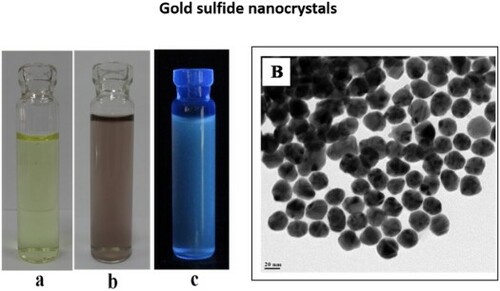
1. Introduction
Nanotechnology has emerged as a novel platform to explore a diverse array of applications [Citation1–9]. Chalcogenide luminescent nanoparticles are categorized under quantum dots and are composed of group II–VI (e.g. CdTe, CdSe, CdS) [Citation10] and group III–V (e.g. InAs, InP) [Citation11–13]. Owing to its optical absorption and emission spectra as a function of size and shape, these dots have received substantial interest in nanoscale fluorescence visualization and detection [Citation14,Citation15]. The application of these semiconductor nanoparticles has been extended successfully to cell labeling, in vivo imaging [Citation16], diagnostics [Citation17] and multidisciplinary applications in solar energy conversion, optics, and photonics [Citation18, Citation19]. The unique optical properties of Au-based materials which distinguish it from other chalcogenides is localized surface plasmon resonance [Citation20]. Gold-based NPs are used widely in bioimaging techniques including imaging of single cells, cell structures and cell imaging, and imaging of animals in vivo in real time [Citation21]. Today, the great challenge that hinders application is the development of eco-friendly, efficient, reliable processes for nanomaterials synthesis [Citation22]. Over the years, various synthesis strategies were designed for metal chalcogenide nanoparticles and their structures. Nonetheless, very limited data regarding synthesis and characterization of Au2S nanoparticles has been reported. To date, gold sulfide nanoparticles are synthesized by chemical reactions [Citation23] using Au(I) complexes such as KAu(CN)2 and Na3Au(SO3)2. These nanoparticles tend to agglomerate during the chemical synthesis process. This concern was addressed by the usage of apoferritin as an alternative in the synthesis procedure [Citation24].
Biological methods of NPs preparation have received substantial attention as they are environmentally friendly and non-hazardous [Citation25]. Biogenic NPs have been prepared either extracellularly or intracellularly utilizing fungi, bacteria, and plants. In this context, the reducing agents are biomolecules that stabilize NPs [Citation26]. Fungal species are important as they can produce particles with high stability thus preventing aggregation and enhancing longevity [Citation27]. Fungi are relatively more resourceful than bacteria in the biosynthesis of nanoparticles due to the presence of a number of bioactive metabolites, high accumulation of metals, and enhanced production [Citation28]. Similar to CuAlO2 [Citation29], gold sulfide nanoparticles show p-type semiconductivity [Citation30]. In most previous studies conducted, a little success was noted in the formation of stable gold sulfide nanocrystals. Limited number of investigations was carried out and only a few researches have been successful. However, studies indicate that, a great challenge still remains in the synthesis of Au2S NPs from Au(III) complexes [Citation31]. Further, the most common gold compounds are Au(III) complexes i.e. auric chloride (AuCl3) and chlorauric acid (HAuCl4). Thus motivations towards this purpose lead to the synthesis of stable nanoparticles in our previous research on metal, metal sulfide, and other nanosystems [Citation32–34]. In the present investigation, we have biosynthesized gold sulfide nanoparticles with the HAuCl4 precursor using Humicola sp., which was completely characterized by various standard techniques.
Leishmania spp., an obligate intracellular parasite, causes a vector-borne infection known as leishmaniasis [Citation35]. Paromomycin, pentamidine, miltefosine, amphotericin B, and pentavalent antimonials are some of the pharmaceuticals used to treat this parasitic infection. Unfortunately, due to the appearance of resistant parasites, these drugs require long-term administration and are of poor efficiency with several side effects [Citation36]. Recent investigations have addressed the anti-leishmanial effects of certain NPs including Ag, Au, TiO2, ZnO, and MgO NPs on L. major [Citation37]. Herein, we focused on the anti-leishmanial activity of bio-based Au2S against the promastigote and its cell-specificity. The potential cytotoxicity of Au2S NPs was evaluated against both normal and cancer (Daudi, ZR-75-1) cell lines. Intravenously administered drugs interact with erythrocytes, which may culminate in hemolysis [Citation38]. These undesirable effects have limited the use of anti-leishmanial drugs such as miltefosine. Hence, hemolytic studies have been performed with biosynthesized Au2S.
2. Materials and methods
2.1. Materials
HAuCl4 and Na2SO3 were purchased from Sigma Aldrich (U.S.A.). Peptone, glucose, yeast extract, and malt extract were procured from HiMedia (city, state) and used as-received.
2.2. Cell culture and reagents
Human lymphoma cancer (Daudi) and human breast cancer (ZR-75-1) cell lines were procured from NCCS, Pune, and RPMI-1640 media (Invitrogen) was employed to grow the cell lines with fetal bovine serum (FBS) (10%) (Pan Biotech, Germany) supplement and Gentamycin (100 µg/mL). In a 95% humidified atmosphere at 37°C, cancer cells were grown in the presence or absence of 5% CO2. MTT dye was obtained from HiMedia.
2.3. Fungal growth and maintenance
The fungus was isolated from self-heating compost and was preserved on MGYP [yeast extract (0.3%), malt extract (0.3%), glucose (1%) and peptone (0.5%)] agar slants. The maintenance of stock cultures was carried out by monthly sub-culturing. For 4 days, the fungus slants were grown at 50°C and pH 9 and preserved at 15°C. The subcultures on fresh slants were made from an actively growing stock culture. The slants were incubated for 4 days at 50°C and pH 9. The above-prepared slant was used for the biosynthesis of Au2S NPs.
2.4. Extracellular biosynthesis of αAu2s nanoparticles by Humicola sp.
For αAu2S NPs biosynthesis, MGYP medium (100 mL) was used to grow fungus in 250-mL Erlenmeyer flasks. The medium pH was adjusted to 9.0 with sterile 10% sodium carbonate. Thereafter, the culture was grown for 96 h at 50°C using a rotary shaker at 200 rpm continuous shaking. After fermentation for 96 h, the broth culture was centrifuged at 5000 rpm for 20 min at 20°C to separate the mycelia. Using sterile distilled water, the collected biomass was washed three times in sterile conditions. The mycelial (cell) wet mass harvested (20 g) was resuspended in HAuCl4 (1 mM) and a sulfur source 1 mM Na2SO3 (100 mL). The suspension was added to the same Erlenmeyer flasks (250 mL) and placed on a shaker (200 rpm, 50°C). The reaction was allowed to proceed for 96 h and then the biomass was separated using filter paper in sterile conditions. At regular intervals, portions of the filtrate were removed and UV–visible spectra were recorded to identify extracellularly formed NPs. Later, the NPs were lyophilized and re-dispersed in water for further experiments.
2.5. Characterization
Optical properties of Au2S NPs were examined with a UV–VisibleNIR spectrophotometer (Lambda 750) operated at 1 nm resolution. A Cary Eclipse luminescence spectrophotometer was used for fluorescence measurements. Structural analysis and morphology were studied using X-ray powder diffraction measurements and transmission electron microscopy (TEM). A PW 1830 instrument was used to obtain XRD patterns (CuKα radiation, 40 kV, 30 mA, λ = 1.5404 Å). FTIR analysis was performed using a Tensor 27 (Bruker) instrument. The biosynthesized Au2S NPs were placed on a copper grid and coated with carbon for EDAX analysis. At 200 kV accelerating voltage, the grid was analyzed with a FEI Technai G2 system. The SAED analysis and EDAX were performed on the same grid. Using spot profile mode, EDAX was recorded where the electron beam was focused on the NP-coated surface.
2.6. Toxicity studies
2.6.1. Parasite culture and maintenance
Leishmania donovani promastigotes (Ag83) strain was obtained from the Department of Biotechnology, UoP, Pune. It was grown in RPMI-1640 (Roswell Park Memorial Institute Medium) liquid medium with 100 U/ml Gentamycin (Cipla) and FBS (10%, heat inactivated, Pan Biotech, Germany) supplements at 23°C.
2.6.2. Nanoparticle dilution
Au2S nanoparticles and Amphotericin-B were dissolved in 1X phosphate buffer saline at 5 mg concentration and stored at 4 °C. At the time of the experiment, the drugs were reconstituted and dissolved in liquid media RPMI-1640 at various concentrations.
2.6.3. In vitro anti-promastigote activity
Following filter sterilization (0.22 µm), nanoparticles and Amphotericin-B were dissolved in RPMI-1640 medium. The anti-leishmanial activity of the NPs was examined with various concentrations of lyophilized Au2S (100 µL) after being re-dispersed in water. This was accomplished by seeding L. donovani promastigotes (2×106 cells/ml) in 100 µL culture medium with 20% FBS in 96-well microtiter plates with NPs and incubated for 48 h (23°C). Parasites viability assay was conducted calorimetrically after incubating for 48 h using the mitochondrial oxidation of 3-[4,5-dimethylthiazol-2-yl]-2,5 diphenyl tetrazolium bromide (MTT) (HiMedia, India) assay [Citation39]. Briefly, MTT was dissolved in RPMI-1640 medium (2 mg/ml) and filter-sterilized (0.22 μm). After adding MTT (200 μg/ml) to plates, it was incubated for 3 h at 23°C. Finally, to dissolve the produced MTT formazan 100 μL of DMSO was added to each well and kept for 15 min. Absorbance was recorded at 550 nm using an ELISA reader and correlated with living promastigotes count standardized for each plate. The control in all experiments was Amphotericin-B and for comparison, growth in medium alone was considered as 100% growth. All experiments were performed in triplicate with three independent assays. After 48 h incubation, anti-promastigote activity was expressed as the 50% inhibitory concentration (IC50). The IC50 value was calculated with a sigmoid dose–response curve.
2.6.4. Cell viability assay
In 96-well plates, Daudi and ZR-75-1 cells (2 × 104) were seeded. Cells were treated for 24 h with Au2S at (50-500 μg/mL). The Modified MTT test proposed by Mosmann [Citation39] was used in determining the survival of cells. For terminating the treatment, the media was removed followed by the addition of MTT (2 mg/ml). In DMSO, particles were dissolved after 4 h and absorbance at 550 nm was recorded.
2.6.5. Preparation of peripheral blood mononuclear cells (PBMC)
Blood was collected from two healthy volunteers, and human PBMC separation was carried out by the Ficoll-paque gradient centrifugation from whole heparinized blood [Citation40]. Using PBS (pH 7.2), the collected interface cells were washed three times, counted, and resuspended in cRPMI medium. The Ethics Committee of the University of Pune (Ethics-UoP/2012/19) approved the investigation protocol. Written informed consent was obtained from each healthy donor.
2.6.6. Cytotoxicity assay
In 96-well microtiter plates, seeding of PBMC (2 × 104 cells per ml) was made into cRPMI-1640. After 30 min, cells were treated for 24 h in individual wells with the necessary concentration of Au2S. Au2S was absent in control well and all experiments were conducted in triplicate. A modified MTT test proposed by Mosmann [Citation39] was used in determining the survival of cells. Briefly, following 24 h Au2S treatment, MTT solution was added to each well. Samples were incubated for 4 h and then DMSO (100 μL) was added. Absorbance at 550 nm was recorded.
2.6.7. Hemolysis assay
As per US FDA Guidelines, a hemolysis assay was performed with Au2S-treated human red blood cells (RBCs) to determine percent hemolysis [Citation41]. From two healthy volunteers whole heparinized RBCs were collected by repeated saline (pH 7.4) wash. Afterward, the RBC suspension (1%) was made and incubated for 45 min (37°C) with various Au2S concentrations or corresponding Triton X-100 (0.1%) positive controls. Samples were centrifuged for 5 min at 400×g and A540 was recorded. By comparing with 100% lysed RBD, percent hemolysis was determined.
2.7. Statistical analysis
Data are shown as mean (±SD) of triplicate samples from three independent assays. Using dose–response curves, 50% inhibitory concentration (IC50) values were determined. Using the Student’s t-test, statistical differences were calculated. The p-value < 0.05 was considered significant.
3. Results and discussion:
3.1. Synthesis of Au2S
Incubation (50°C, pH 9, 96 h) of the fungus Humicola sp. with HAuCl4 and Na2SO3 (1 mM) solution produced water-dispersible extracellular Au2S (gold sulfide) NPs. The color change from pale yellow to light brown indicates Au2S NPs were formed in the reaction mixture ()
Figure 1. (A) (a) precursor, (b) biosynthesized Au2S nanoparticles, and (c) fluorescence from Au2S nanoparticles when excited with a UV lamp at 365 nm. (B) UV spectroscopy and (C) Tauc’s plot.
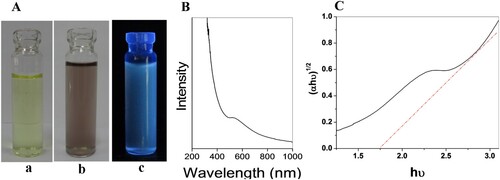
In order to determine whether the nascent nanoparticles were formed extracellularly or intracellularly, the contents of the reaction flask were filtered after 4 days of reaction and the biomass and aqueous filtrate were characterized by standard techniques. The biomass retained on the filter paper contained no metal sulfide nanoparticles. As the biomass did not show any synthesis of nanoparticles, we conclude there was no possibility of metal sulfide nanoparticles being formed intracellularly and that nanoparticles were formed extracellularly in solution. Even months past reaction, the solution showed high stability with no evidence of flocculation of nanoparticles. It is established that capping and stabilization of the nanoparticles is brought about by different proteins and other biomolecules secreted by microorganisms [Citation42]. Extracellular synthesis of nanoparticles involves trapping metal ions on the surface of the cells and reducing them in the presence of enzymes [Citation43]. Recently, different filamentous fungi were reported to be proficient in the biosynthesis of Au NPs. The researchers employed different methods for the biosynthesis of Au NPs and proposed that fungal compounds, reductase enzymes, and fungal media components played a role in stabilizing the nanoparticles [Citation44]. The authors indicated that reducing sugars were involved to tailor spherical Au NPs. They also established the role of specific fungal proteins in the capping Au NPs [Citation44, Citation45]. In order to validate the extracellular synthesis of NPs, the microbial cells were collected and washed after the synthesis procedure. Then the cells were subjected to atomic absorption spectroscopy. The presence of negligible amounts of Au in the cells indicates extracellular synthesis.
3.2. Characterization
The shape and size of the Au2S NPs were determined by TEM analysis ((A,B)). The morphology of the nanoparticles was uniform and particles were well dispersed due to the capping protein. Sizes of the nanoparticles ranged between 20 and 30 nm. (C) shows SAED patterns exhibiting a crystalline nature as well as superstructures of Au2S/Au nanoparticles. (D) shows the EDAX spectrum, including the signal from Au together with those of C and O contributed by the secreted biomolecules which cap nanoparticle surfaces.
The formation of the newly synthesized nanoparticles and composition was verified via XRD analysis (). The Bragg’s reflection (111), (200), (220), (311), and (222) of Au2S are identified in the spectrum, confirming the formation of Au2S nanoparticles [Citation30]. XRD peaks from gold nanoparticles were also recorded in the same pattern as also indicated by TEM analysis. The broadening in the spectrum shows the nanoscale dimension of the nanoparticles. The XRD pattern of Au2S NPs was determined by applying the Scherrer equation,
where D = effective particle size; λ = wavelength of X-ray source; β = full width of a diffraction peak at half maximum; and θ = Braggs angle. The middling size of Au2S NPs was approximately estimated to be 22 nm.
FTIR analysis was carried out to determine the possibility of fungal secretions with functional groups of bioactive molecules that facilitated the reduction of gold ions and stabilization of Au2S NPs from the fungus Humicola sp. The nanoparticles were further analyzed to confirm the protein layer on the particle surfaces. The FTIR spectrum depicts the amide I and amide II signature at 1650 and 1544 cm−1, respectively [Citation36], and 3240 cm−1 corresponds to the hydroxide (−OH) [Citation46,Citation47] stretching vibration. The OH− or NH− may include alcohols, phenols, carbohydrates, or proteins that play a key role in bio-based synthesis of Au2S NPs. This possibly suggests that protein coincides with the Au2S NPs. The small band around 2900 cm−1 represents the CH− stretching of phenol aromatic group. Au2S NPs solutions displayed a band at around 1000–1100 cm−1 that attributes to the CN− stretching vibrations of aliphatic amines. This existence of amide links that could gather the amino acid residue of the protein, including tryptophan/tyrosine is liable for the conceivable stabilization of the bio-based synthesized Au2S NPs [Citation48, Citation49]. The protein layer on NPs provides a water-dispersible nature and stability against particle aggregation. It can be beneficial in conjugation with protein, DNA, peptide, and chemotherapeutic drug applications. The vigorous protein- and enzyme-secreting ability of microbes ensures NP coating with protein, enhanced NP production, and prevention of NP agglomeration [Citation50, Citation51].
3.3. In vitro anti-promastigote activity:
The anti-leishmanial potential of biologically synthesized Au2S nanoparticles was investigated using IC50 values (50–500 µg/ml). The viability (a reflection of cell cytotoxicity) of the promastigotes on the addition of 100µg/ml nanoparticles was compared to promastigote treated with medium alone (n = 3). Amphotericin-B, which was also tested in the assay, is a commercially available potential anti-leishmanial drug used in clinics for the treatment of ‘Kala-azar’ patients who show refractoriness to standard antimony compounds; however, it is expensive and toxic with numerous side effects [Citation52, Citation53]. Nanoparticles decreased the viability of promastigotes at concentrations of 50–500 µg/ml of Au2S (IC50 value) and above ((A)). The NP uptake efficiency through the lipid bilayer of the parasite is hindered by parameters including irregular NP shape and size [Citation54]. Hence, an anti-leishmanial potential is promoted by the preparation of NPs of small size to avoid the aggregate formation and ready uptake [Citation55]. The biologically synthesized nanoparticles provided comparable results to Amphotericin-B and thus can possibly be developed as an inexpensive substitute.
3.4. Au2S antiproliferative activity
The cytotoxicity investigation showed that ZR-75-1 and Daudi cells had markedly low sensitivity to Au2S. Nanoparticles exhibited significantly less toxicity in cancer cells at concentrations of 50–500 µg/ml and induced about 20% cell death at the 500 µg/ml concentration. Au2S had relatively less effect on ZR-75-1 and Daudi cells at higher concentrations ((B)). Au2S exhibited cell-specific and less significant antiproliferative activity.
3.5. Percent cytotoxicity and hemolysis activity
Greater than 80% viability of PBMC was observed when treated with the 50–500 µg/ml concentration of Au2S. The 50–500 µg/ml concentration showed dose-dependent viability reduction ((B)). However, in the concentrations mentioned above there was a steady percentage of viability from 98% to 99% ((C)).
Percent hemolysis was determined to be less than 1.5% from 50–500 µg/ml concentrations, indicating these concentrations of Au2S to be nontoxic to RBCs and confirming its safety to human cells.
4. Conclusion
The present investigation employed the fungus Humicola sp. for the biosynthesis of gold sulfide nanoparticles. The fungus produced extracellular nanoparticles upon reaction with precursors HAuCl4 and Na2SO3. Particle morphology is spherical; fungal metabolites including biomolecules secreted in the reaction medium are primarily responsible for nanoparticle synthesis and stabilization. Nanoparticles decreased the viability of promastigotes at 50 µg/mL of Au2S (IC50 value) and above. Low toxicity (20%) was observed when 500 µg/mL NPs were exposed to cancer cells. The effects of NPs were relatively low on ZR-75-1 and Daudi cells at high concentrations. More than 80% viability of PBMC and less than 1.5% hemolysis were observed at 50–500 µg/mL NPs. Hence, the prepared particles are considered nontoxic to humans. In future applications, these NPs are therefore suggested for leishmania treatment, diagnostics, and drug carrier applications.
Acknowledgments
A acknowledges the Department of Biotechnology (DBT), Government of India, New Delhi for setting up a Centre of Excellence (COE, BT/PR1-3584/COE/34/29/2015) at Interdisciplinary Nanotechnology Centre (INC), Aligarh Muslim University (AMU). The authors thank the Centre for Materials Characterization (CSIR-NCL) for assistance regarding TEM and XRD measurements. The authors extend the appreciation to the Researchers Supporting Project Number (RSP-2021/15), King Saud University, Riyadh, Saudi Arabia.
Disclosure statement
No potential conflict of interest was reported by the author(s).
Additional information
Funding
References
- Kargozar, S.; Mozafari, M. Nanotechnology and Nanomedicine: Start Small, Think big. Mater. Today: Proc. 2018, 5, 15492–15500.
- Shirzadi-Ahodashti, M.; Ebrahimzadeh, M.A.; Ghoreishi, S.M.; Naghizadeh, A.; Mortazavi-Derazkola, S. Facile and Eco-benign Synthesis of a Novel MnFe2O4@SiO2@Au Magnetic Nanocomposite with Antibacterial Properties and Enhanced Photocatalytic Activity Under UV and Visible-Light Irradiations. Appl. Organomet. Chem. 2020, 34 (5), e5614.
- Ebrahimzadeh, M.A.; Naghizadeh, A.; Mohammadi-Aghdam, S.; Khojasteh, H.; Ghoreishi, S.M.; Mortazavi-Derazkola, S. Enhanced Catalytic and Antibacterial Efficiency of Biosynthesized Convolvulus Fruticosus Extract Capped Gold Nanoparticles (CFE@AuNPs). J. Photochem. Photobiol., B 2020, 209, 111949.
- Mohammadzadeh, P.; Ardestani, M.S.; Mortazavi-Derazkola, S.; Bitarafan-Rajabi, A.; Ghoreishi, S.M. PEG-Citrate Dendrimer Second Generation: Is This a Good Carrier for Imaging Agents in Vitro and in Vivo? IET Nanobiotechnol. 2019, 13, 560–564.
- Ebrahimzadeh, M.A.; Mortazavi-Derazkola, S.; Zazouli, M.A. Eco-friendly Green Synthesis of Novel Magnetic Fe3O4/SiO2/ZnO-Pr6O11 Nanocomposites for Photocatalytic Degradation of Organic Pollutant. J. Rare Earths 2020, 38, 13–20.
- Ebrahimzadeh, M.A.; Mortazavi-Derazkola, S.; Zazouli, M.A. Eco-friendly Green Synthesis and Characterization of Novel Fe3O4/SiO2/Cu2O–Ag Nanocomposites Using Crataegus Pentagyna Fruit Extract for Photocatalytic Degradation of Organic Contaminants. J. Mater. Sci.: Mater. Electron. 2019, 30, 10994–11004.
- Mortazavi-Derazkola, S.; Ebrahimzadeh, M.A.; Amiri, O.; Goli, H.R.; Rafiei, A.; Kardan, M.; Salavati-Niasari, M. Facile Green Synthesis and Characterization of Crataegus Microphylla Extract-Capped Silver Nanoparticles (CME@Ag-NPs) and its Potential Antibacterial and Anticancer Activities Against AGS and MCF-7 Human Cancer Cells. J. Alloys Compd. 2020, 820, 153186.
- Safari-Amiri, M.; Mortazavi-Derazkola, S.; Salavati-Niasari, M.; Ghoreishi, S.M. Synthesis and Characterization of Dy2O3 Nanostructures: Enhanced Photocatalytic Degradation of Rhodamine B Under UV Irradiation. J. Mater. Sci.: Mater. Electron. 2017, 28, 6467–6474.
- Mortazavi-Derazkola, S.; Naimi-Jamal, M.R.; Ghoreishi, S.M. Synthesis, Characterization and Atenolol Delivery Application of Functionalized Mesoporous Hydroxyapatite Nanoparticles Prepared by Microwave-Assisted Co-Precipitation Method. Curr. Drug Delivery 2016, 13, 1123–1129.
- Nideep, T.K.; Ramya, M.; Kailasnath, M. An Investigation on the Photovoltaic Performance of Quantum Dot Solar Cells Sensitized by CdTe, CdSe and CdS Having Comparable Size. Superlattices Microstruct. 2020, 141, 106477.
- Shamsi, J.; Urban, A.S.; Imran, M.; De Trizio, L.; Manna, L. Metal Halide Perovskite Nanocrystals: Synthesis, Post-Synthesis Modifications, and Their Optical Properties. Chem. Rev. 2019, 119 (2019), 3296–3348.
- Xu, Z.; Li, Y.; Li, J.; Pu, C.; Zhou, J.; Lv, L.; Peng, X. Formation of Size-Tunable and Nearly Monodisperse InP Nanocrystals: Chemical Reactions and Controlled Synthesis. Chem. Mater. 2019, 31, 5331–5341.
- Ng, S.M.; Koneswaran, M.; Narayanaswamy, R. A Review on Fluorescent Inorganic Nanoparticles for Optical Sensing Applications. RSC Adv. 2016, 6, 21624.
- Shamsipur, M.; Molaabasi, F.; Shanehsaz, M.; Moosavi-Movahedi, A.A. Novel Blue-Emitting Gold Nanoclusters Confined in Human Hemoglobin, and Their Use as Fluorescent Probes for Copper(II) and Histidine. Microchim. Acta 2015, 182, 1131–1141.
- Bian, W.; Wang, F.; Wei, Y.L.; Wang, L.; Liu, Q.L.; Dong, W.J.; Shuang, S.M.; Choi, M.M.F. Doped Zinc Sulfide Quantum Dots Based Phosphorescence Turn-off/on Probe for Detecting Histidine in Biological Fluid. Anal. Chim. Acta 2015, 856, 82–89.
- Takahashi, T.; Watanabe, S. Recent Progress in CdTe and CdZnTe Detectors. IEEE Trans. Nucl. Sci. 2001, 48, 950–959.
- Farias, P.M.A.; Santos, B.S., Fontes, A., et al. Colloidal Semiconductor Quantum Dots: Potential Tools for new Diagnostic Methods. Appl. Surf. Sci. 2008, 255, 691–693.
- Zhu, C., Chen, Z., et al. Recent Advances in Non-Toxic Quantum Dots and Their Biomedical Applications. Progress in Nat. Sci.: Mater. Internat. 2019, 29, 628–640.
- Reshma, V.G.; Mohanan, P.V. Quantum Dots: Applications and Safety Consequences. J. Lumin. 2019, 205, 287–298.
- Depciuch, J.; Stec, M.; Kandler, M.; Baran, J.; Parlinska-Wojtan, M. From Spherical to Bone-Shaped Gold Nanoparticles—Time Factor in the Formation of Au NPs, Their Optical and Photothermal Properties. Photodiagn. Photodyn. Ther. 2020, 30, 101670.
- Hutter, E.; Maysinger, D. Gold Nanoparticles and Quantum Dots for Bioimaging. Microsc. Res. Tech. 2010, 74, 592–604.
- Hasan, M.; Ullah, I.; Zulfiqar, H.; Naeem, K.; Iqbal, A.; Gul, H.; Ashfaq, M.; Mahmood, N. Biological Entities as Chemical Reactors for Synthesis of Nanomaterials: Progress, Challenges and Future Perspective. Mater. Today Chem. 2018, 8, 13–28.
- Zhou, H.S.; Honma, I.; Haus, J.W.; Sasab, H.; Komiyama, H. Synthesis and Optical Properties of Coated Nanoparticle Composites. J. Lumin. 1996, 70, 21–34.
- Yoshizawa, K.; Iwahori, K.; Sugimoto, K.; Yamashita, I. Fabrication of Gold Sulfide Nanoparticles Using the Protein Cage of Apoferritin. Chem. Letter 2006, 35, 1192.
- Pereira, L.; Mehboob, F.; Stams, A.J.M.; Mota, M.M.; Rijnaarts, H.H.M.; Alves, M.M. Metallic Nanoparticles: Microbial Synthesis and Unique Properties for Biotechnological Applications, Bioavailability and Biotransformation. Crit. Rev. Biotechnol. 2015, 35, 114–128.
- Boroumand Moghaddam, A.; Namvar, F.; Moniri, M.; Tahir, P.M.; Azizi, S.; Mohamad, R. Nanoparticles Biosynthesized by Fungi and Yeast: A Review of Their Preparation, Properties, and Medical Applications. Molecules 2015, 20 (9), 16540–16565.
- Castro-Longoriaa, E.; Vilchis-Nestor, A.R.; Avalos-Borja, M. Biosynthesis of Silver, Gold and Bimetallic Nanoparticles Using the Filamentous Fungus Neurospora Crassa. Colloids Surf., B 2011, 83, 42–48.
- Alghuthaymi, M.A.; Almoammar, H.; Rai, M.; Said-Galiev, E.; Abd-Elsalam, K.A. Myconanoparticles: Synthesis and Their Role in Phytopathogens Management. Biotechnol. Biotechnological Equip. 2015, 29, 221–236.
- Miclau, M.; Miclau, N.; Banica, R.; Ursu, D. Effect of Polymorphism on Photovoltaic Performance of CuAlO2 Delafossite Nanomaterials for p-Type dye-Sensitized Solar Cells Application. Mater. Proc. 2017, 4, 6975–6981.
- Ishikawa, K.; Isonaga, T.; Wakita, S.; Suzuki, Y. Structure and Electrical Properties of Au2S. Solid State Ionics 1995, 79, 60–66.
- Kuo, C.; Huang, M.H. Hydrothermal Synthesis of Free-Floating Au2S Nanoparticle Superstructures. J. Phys. Chem. C 2008, 112, 11661–11666.
- Sonawane, S.; Ahmad, A.; Chinnathambi, S. Protein-Capped Metal Nanoparticles Inhibit Tau Aggregation in Alzheimer's Disease. ACS Omega 2019.
- Moeez, S.; Siddiqui, E.A., Khan, S.; A. Ahmad. Size Reduction of Bulk Alumina for Mass Production of Fluorescent Nanoalumina by Fungus Humicola sp. J. Cluster Sci. 2017, 28, 1981–1993.
- Khan, S.A.; Ahmad, A. Phase, Size and Shape Transformation by Fungal Biotransformation of Bulk TiO2. Chem. Eng. J. 2013, 230, 367–371.
- Reithinger, R. Dujardin, J.C.; Louzir, H.; Pirmez, C.; Alexander, B.; Brooker, S. Cutaneous Leishmaniasis. Lancet Infect. Dis. 2007, 7, 581–596.
- Aureliano, D.P.; Ribeiro, M.S.; Lindoso, J.A.L.; Pogliani, F.C.; Sellera, F.P.; Song, D.; Baptista, M.S. Treatment and Control of Leishmaniasis Using Photodynamic Therapy, Leishmaniasis Trends in Epidemiology. Diagnosis and Treatment 2014, 393–412.
- Jebali, A.; Kazemi, B. Nano-based Antileishmanial Agents: A Toxicological Study on Nanoparticles for Future Treatment of Cutaneous Leishmaniasis. Toxicol. Vitro 2013, 27 (6), 1896–1904.
- Bitencourt, J.J.G., Pazin, W.M., et al. Miltefosine-loaded Lipid Nanoparticles: Improving Miltefosine Stability and Reducing its Hemolytic Potential Toward Erythtocytes and its Cytotoxic Effect on Macrophages. Biophys. Chem. 2016, 217, 20–31.
- Mosmann, T. Rapid Colorimetric Assay for Cellular Growth and Survival: Application to Proliferation and Cytotoxicity Assays. J. Immunol. Meth. 1983, 65, 55–63.
- Boyum, A. Isolation of Mononuclear Cells and Granulocytes from Human Blood. Isolation of Monuclear Cells by one Centrifugation, and of Granulocytes by Combining Centrifugation and Sedimentation at 1 g. Scand. J. Clin. Lab. Invest. 1968, 97, 77–89.
- FDA Guidance for Industry. Nonclinical Studies for the Safety Evaluation of Pharmaceutical Excipients. 2005.
- Mazumdar, B.; Uddin, I.; Khan, S.; Ravi, V.; Selvraj, K., Poddar, P.; Ahmad, A. Bio-milling Technique for the Size Reduction of Chemically Synthesized BiMnO3 Nanoplates. J. Mater. Chem. 2007, 17, 3910–3914.
- Li, X.; Xu, H.; Chen, Z.; Che, G. Biosyn. Nanoparticles Microorgan. Appl. 2011, 1–16, 270974.
- Molnár, Z.; Bódai, V.; Szakacs, G.; Erdélyi, B.; Fogarassy, Z.; Sáfrán, G.; Varga, T.; Kónya, Z.; Tóth-Szeles, E.; Szűcs, R.; Lagzi, I. Green Synthesis of Gold Nanoparticles by Thermophilic Filamentous Fungi. Sci. Rep. 2018, 8, 3943.
- Zhang, X.; He, X.; Wang, K.; Yang, X. Different Active Biomolecules Involved in Biosynthesis of Gold Nanoparticles by Three Fungus Species. J. Biomed. Nanotechnol. 2011, 7, 245–254.
- Zhang, H., Zhou, H., et al. Biosynthesis of Selenium Nanoparticles Mediated by Fungus Mariannaea sp. HJ and Their Characterization. Colloids Surf., A 2019, 571, 9–16.
- Mishra, A.; Tripathy, S.K.; Wahab, R.; Jeong, S.-H.; Hwang, I., Yang, Y., et al. Microbial Synthesis of Gold Nanoparticles Using the Fungus Penicillium Brevicompactum and Their Cytotoxic Effects Against Mouse Mayo Blast Cancer C2C12 Cells. Appl. Microbiol. Biotechnol. 2011, 92, 617–630.
- Abdel-Kareem, M.M.; Zohri, A. Extracellular Mycosynthesis of Gold Nanoparticles Using Trichoderma Hamatum: Optimization, Characterization and Antimicrobial Activity. Lett. Appl. Microbiol. 2018, 67, 465–475.
- Suganthy, N.; Ramkumar, V.S.; Pugazhendhi, A.; Benelli, G.; Archunan, G. Biogenic Synthesis of Gold Nanoparticles from Terminalia Arjuna Bark Extract: Assessment of Safety Aspects and Neuroprotective Potential via Antioxidant, Anticholinesterase, and Antiamyloidogenic Effects. Environ. Sci. Pollut. Res. Int. 2018, 25, 10418–10433.
- Jain, N.; Bhargava, A.; Majumdar, S.; Tarafdar, J.C.; Panwar, J. Extracellular Biosynthesis and Characterization of Silver Nanoparticles Using Aspergillus Flavus NJP08: a Mechanism Perspective. Nanoscale. 2011, 3, 635–641.
- Kadam, V.V.; Ettiyappan, J.P.; Balakrishnan, R.M. Mechanistic Insight Into the Endophytic Fungus Mediated Synthesis of Protein Capped ZnO Nanoparticles. Mater. Sci. Eng.: B 2019, 243, 214–221.
- Ehrenfreund-Kleinman, T., et al. Synthesis and Characterization of Novel Water Soluble Amphotericin B–Arabinogalactan Conjugates. Biomaterials 2002, 23, 1327–1335.
- Herwaldt, B.L. Leishmaniasis. Lancet 1999, 354, 1191–1199.
- Lopera, A.A.; Velásquez, A.M.A.; Clementino, L.C.; Robledo, S.; Montoya, A.; de Freitas, L.M.; Bezzon, V.D.N.; Fontana, C.R.; Garcia, C.; Graminha, M.A.S. Solution-combustion Synthesis of Doped TiO2 Compounds and its Potential Antileishmanial Activity Mediated by Photodynamic Therapy. J. Photochem. Photobiol. B Biol. 2018, 183, 64–74.
- Sepúlveda, A.A.L., Velásquez, A.M.A., et al. Efficacy of Photodynamic Therapy Using TiO2 Nanoparticles Doped with Zn and Hypericin in the Treatment of Cutaneous Leishmaniasis Caused by Leishmania Amazonensis. Photodiagn. Photodyn. Ther. 2020, 30, 101676.