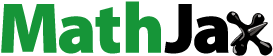
ABSTRACT
Green synthesis is a more eco-friendly approach compared to the chemical and physical methods. This simple green eco-friendly method was utilized to obtain silver nanoparticles mediated by an aqueous leaf extract as a precursor. The silver nanoparticles mediated by an aqueous solution of Daucus carota L. leaf extract (DCLE) as bio-reducer and stabilizer agents are described. The AgNPs were characterized using FT-IR, UV-Vis, PXRD, and TEM. Antimicrobial activities of the samples were investigated against drug-resistant bacterial isolates using the disk diffusion method, while in vitro cytotoxicity assay by MTS end-point and xCELLigence real-time assays. The synthesized AGNPs from DCLE exhibited face-centered cubic phase with average particle sizes of 18.26 (±6.86) nm and 16.81 (±7.72) nm for AgNP05 and AgNP01 respectively. The scavenging ability of DPPH radicals by the nanoparticles was studied for their antioxidant potentials. The study revealed that the compounds are capable of scavenging DPPH radicals in a dose-dependent pattern (IC50 = 2.61 ± 0.58 μM for extract, 2.79 ± 1.75 μM for AgNP05, 2.84 ± 0.96 μM for AgNP01). Antimicrobial results showed that the nanoparticles possess higher activities. Cytotoxicity of the nanoparticles (0.00 μg/ml – 100 μg/ml) against U87MG brain glioblastoma cells, showed ED50 = 87.31 and 109.48 μg/ml.
GRAPHICAL ABSTRACT
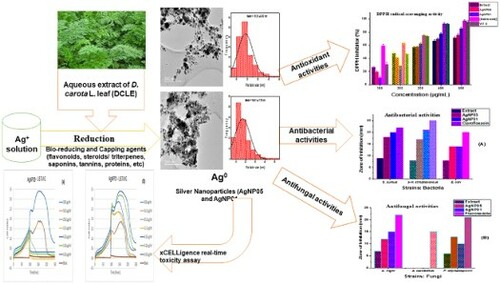
1. Introduction
Green synthesis of nanoparticles (NPs) has been a recent development in the field of nanotechnology. This kind of synthesis is a more eco-friendly approach compared to the chemical and physical methods. It involves the use of natural ingredients in the bio-reduction of metallic ions to nanoparticles (Citation1–4). This simple green eco-friendly method has been utilized to obtain metal nanoparticles using the leaf extract as a precursor (Citation5–13). Safer and easily metabolized drugs are natural compounds retrieved from plant materials as compared to other synthetic medicinal compounds (Citation1, Citation11, Citation14). Metallic nanoparticles are mostly synthesized from precious metals viz., silver (Ag), gold (Au), platinum (Pt), and lead (Pb). From these metals, silver is the most preferred metal in the area of the biological system due to its wide range of activities over other metals (Citation8, Citation15, Citation16). The development of these green nanoparticles has been intensified in recent research not only for their fundamental scientific relevance but also for diverse technological applications (Citation2, Citation4, Citation15, Citation17, Citation18).
Nanoparticles (NPs) have been found useful in various applications in the area of medicine and biology including drug delivery, delivery of genes, photography, pathogen detection, biosensing, protein detection, DNA testing, imaging, tissue engineering, biological labeling, cosmetics, anti-aging drugs, surface-enhanced raman scattering (SERS), and heat destruction of the tumor (hyperthermia) (Citation3, Citation14, Citation17, Citation19–Citation21). Although the preparation and stabilization of metal nanoparticles are mostly via physico-chemical methods, chemical reduction, photochemical reduction, and electrochemical techniques usually known as chemical approaches are widely used (Citation17, Citation22). Metal nanoparticles synthesis via plant extracts is advantageous as its scalability is easy and can function as a bio-reducing, capping, and/or stabilizing agent. Medicinal plants are known to contain various concentrations and components of reducing agents (Citation4, Citation10, Citation11, Citation14, Citation23). Green synthesis of AgNPs using extracts of Garcinia mangostana (Citation5), Lepidium draba (Citation6), Crocus Haussknechtii Bois (Citation23), Mimusops elengi (Citation7), Boerhaaviadiffusa (Citation24), Meliadubia leaf (Citation25), Pistacia atlantica (Citation26), Momordica cymbalaria (Citation27), Mentha aquatica (Citation28), Parquetina nigrescens and Synedrella nodiflora (Citation8), Terminalia chebula (Citation15), Costus afer (Citation10), Ormocarpum cochinchinense (Citation11), Callistemon citrinus (Citation4), and Tectona grandis (Citation13) have been reported in the literature.
In addition to previous reports, a wider range of applications of bio-inspired synthesized silver nanoparticles has been reported with diverse applications. Considerable catalytic activity for reduction of different types of dyes as environmental pollutants has been reported for ultrasonic-assisted green synthesis of silver nanoparticles from Mentha aquatica leaf extract, with enhanced antibacterial activity by lowering the minimum inhibitory concentration (MIC) against B. cereus, P. aeruginosa, S. aureus, and E. coli (Citation28). Characterization and antibacterial activity of silver nanoparticles from the root extract of L. draba weed (Citation6) were reported to exhibit antimicrobial effect on different pathogenic bacteria like E. Coli (ATCC35218), B. cereus (ATCC 14579), S. aureus (ATCC 29213), and S. typhimurium (ATCC 14028). The biosynthesis of silver nanoparticles from P. nigrescens and S. nodiflora (Citation8), and antimicrobial activity of the nanoparticles against ten human pathogens using the agar well diffusion method was investigated. The catalytic activity of silver nanoparticles using Crocus Haussknechtii Bois bulb extract facile green synthesis was evaluated in the presence of sodium borohydride for the degradation of a Congo Red dye, and evaluation of the antibacterial properties against two strains of bacteria, P. aeruginosa and S. aureus via serial dilution method (Citation23). Antimalaria, antitrypanosomal and antibacterial efficacy of silver nanoparticles with spherical shapes and an average size of 29 nm mediated by aqueous crude extracts of aerial parts of Callistemon citrinus plant (Citation4). The potent antiplasmodial potentials exhibited IC50 ranging from 2.99–5.34 μg/mL and a weak IC50 of 107.30 μg/mL for the antitrypanosomal activity of the leaf AgNPs. Electrochemical potentials alongside the antibacterial, antioxidant properties of silver nanoparticles (CA-AgNPs) mediated by Costus afer leaf extract (Citation10). The electrochemical characterization of a CA-AgNPs/multiwalled carbon nanotubes (MWCNT)-modified electrode was used to confirm the charge transfer properties of the nanocomposites, and the CA-AgNPs/MWCNT-modified electrode demonstrated faster charge transport behavior (Citation10).
Carrot (Daucus carota L.) are rich in dietary fiber, antioxidants, anthocyanins, carotenoids, vitamins A, B, and C, and minerals. Daucus carota, an edible carrot is an important root vegetable plant grown all over the world, and they belong to the family Apiaceae. The leaves are bristly pinnate in a pattern that separates into thin segments. The aerial parts are used as livestock feed in some parts of Africa and also as vegetables for human beings after harvesting or thrown away. Traditionally, parts of D. carota have been utilized as antidiabetic, diuretic, muscle and back pain reliever, and aphrodisiac. Ethnomedically, the roots of D. carota L. have been reported to be useful in the treatment of digestive problems, tonsillitis or constipation, and intestinal parasites (Citation19). Aqueous hot leaf extract is taken orally as a uterine stimulant during parturition and has abortifacient potential (Citation29, Citation30). The four most common kinds of phytochemicals dominant in carrots are polyacetylenes, carotenoids, phenolics, and ascorbic acid (Citation19, Citation29, Citation30).
Therefore, owing to the review of the green chemistry on AgNPs synthesis by chemical reaction. The current study describes the assessment of silver nanoparticles (AgNP05 and AgNP01) content using an aqueous solution of Daucus carota L. leaf extract (DCLE). Green synthesis was a method utilized for the preparation of silver nanoparticles (AgNP05 and AgNP01) and was characterized viz: UV-visible spectroscopy, X-ray diffraction (XRD), Fourier transform infrared spectroscopy (FT-IR), and Transmission Electron Microscope (TEM) analysis. Lastly, the key play of the AgNPs in the antioxidant assay (DPPH radicals) and antimicrobial potentials (pathogenic microorganisms: Escherichia coli, Staphylococcus aureus, and α-Hemolytic streptococcus, and fungi species viz Aspergillus candideus, Aspergillus niger, and Penicillium cephalosporin); in vitro cytotoxicity against U87MG brain, glioblastoma cells were experimented.
2. Materials and methods
2.1. Reagents and chemicals
Silver nitrate (99.9%) was received from Sigma-Aldrich (Johannesburg, South Africa). Leaves of Daucus carota L. were collected during the harvest season from the University of Fort Hare farm, Alice, South Africa with location coordinates of 32°47'09''S 26°60'45''E. Freshly prepared double-distilled deionized water was used throughout the study.
2.2. Preparation of plant extract
The fresh leaves of carrot (Daucus carota L.) without any infection, were collected and rinsed under running tap water, then with double-distilled water before use to eliminate dust particles and debrils. The leaves were air-dried for 20 days at room temperature and pulverized into fine powdered using Lasec Polymix PX-MFC 90D mechanical grinder. About 50 g of the crude powdered sample was weighed and macerated with 500 mL of double-distilled water. The mixture was heated at 90°C for 1 h. The cooled mixture was sieved through Whatman No. 1 filter paper. The extract was then refrigerated at 4°C for further experimental works (Citation4–8).
2.3. Synthesis of silver nanoparticles (AgNP05 and AgNP01)
In a typical synthetic procedure for the preparation of AgNP05, 80 mL of aqueous D. carota L. leaf extract (DCLE) was added slowly to 400 mL of 1 mM silver nitrate (AgNO3) solution. The resultant solution was refluxed for 90 min with continuous stirring at 80°C. The initial color when the extract was added to the solution of AgNO3 was pale cream. The formation of AgNPs was followed by a color change from pale cream to dark brown within 90 min. The appearance of darkish brown precipitation was a clear indication of AgNPs formation a suggestive of surface plasmon resonance. The nanoparticles synthesis success was monitored via UV-vis spectroscopy. The AgNPs precipitates obtained were separated through repeated washing and centrifugation at 5000 rpm for 1 h, followed by oven-drying at 60°C (Citation4, Citation8, Citation10).
For the preparation of AgNP01, 50 mL of aqueous D. carota L. leaf extract (DCLE) was added slowly to 400 mL of 1 mM silver nitrate (AgNO3) solution. Similar procedure as AgNP05 was followed.
2.4. Characterization of silver nanoparticles
The bio-reduced AgNP05 and AgNP01 were subjected to different characterization techniques. The silver nanoparticles surface plasmon resonance (SPR) was monitored via UV-Vis spectrophotometer (Model T80+ UV-Vis spectrometer) in the range 200–800 nm. Perkin Elmer FT-IR spectrometer (Spectrum 2000) was used for IR spectra data collection in the range 4000–400 cm−1. The morphology, crystallinity, and size of nanoparticles were assessed by X-ray diffraction spectroscopy (Bruker D8 Advanced x-ray diffractometer operated at a current of 20 mA and a voltage of 40 kV with Cu K radiation). The particle sizes were determined on Transmission electron microscopy by means of a JEOL JEM-2100F TEM operated at 200 kV accelerating voltage.
2.5. 2,2-Diphenyl-1-picrylhydrazyl (DPPH) radical scavenging activity
DPPH (2,2-Diphenyl-1-picrylhydrazyl) radical scavenging activity assay is a standard assay used in antiradical studies. The antioxidant activity of the D. carota L. leaf extract (DCLE) and silver nanoparticles (AgNP05 and AgNP01) samples were investigated spectrophotometrically via the DPPH system. It is a rapid technique for screening the radical scavenging activity of synthesized compounds (Citation21). The radical scavenging properties of the synthesized Ag nanoparticles at various concentrations prepared in DMF solutions (1 mL), were added to 0.4 mM DPPH in methanol (1.0 mL), and vortexed. The solutions were left to incubate for 30 min at room temperature in the dark, thereafter, the scavenging capabilities of the compounds were measured at 517 nm wavelength. The tests analysis was performed in triplicates, to determine the mean ± SD.
% Scavenging activity
(1)
(1)
2.6. Antimicrobial potential
The antimicrobial screening was carried out following a previously reported procedure (Citation5, Citation17). The DCLE and silver nanoparticles (AgNP05 and AgNP01) were investigated for their antimicrobial potentials by the disk diffusion plate method. The compounds were tested against the following selected pathogenic microorganisms: Escherichia coli, Staphylococcus aureus, and α-Hemolytic streptococcus. The antimicrobial effects of nanoparticles and DCLE extracts were also screened against the following fungi species Aspergillus candideus, Aspergillus niger, and Penicillium cephalosporin. The bacterial pure cultures were sub-cultured on sterile Nutrient Agar (NA), while fungi species were sub-cultured on sterile Potato Dextrose Agar (PDA). On the individual plates, each strain was swabbed uniformly using sterile cotton swabs. Sterile discs of 5 mm diameter (with 25 μg/ml silver nanoparticles) were used for the screening alongside a standard antibiotic placed on each plate. The positive control used for bacteria is ciprofloxacin and fluconazole. After incubation for 24 h at 37°C for antibacterial activity, and 24 h at 25°C for antifungal activity, the levels of zones of inhibition were measured.
2.7. In vitro cytotoxicity assay
2.7.1. MTS end-point toxicity assay
In vitro cytotoxicity assay known as MTS (3-(4,5-dimethylthiazol-2-yl)-5-(3-carboxymethoxy-phenyl)-2-(4-sulfophenyl)-2H-tetrazolium) was utilized to evaluate changes in cell viability by means of a color change. MTS compound (with yellow color) was transformed by viable cells giving rise to a dark purple compound. The cell viability is directly proportional to the absorbance. Brain glioblastoma cells (U87MG) were seeded via tissue culture techniques. Incubation of cells (1 × 105 cells/ml) in 96 well plates were achieved at 37°C overnight, followed by the addition of the silver nanoparticles (AgNP05 and AgNP01), in 100, 50.0, 25.0, 12.5, 6.25, 3.125 and 0 μg/ml concentrations. The cells were allowed to incubate for 4 days, after which MTS (5 μl) was added to each well. The absorbance was recorded at 490 nm after 1 h, 2 h, and 4 h gestation phases, averaged, and the viability curves determined. The negative control used was Auranofin. Three (Citation3) plates in duplicate (n = 6) were used to evaluate the samples across and the average values reported (Citation11, Citation27).
2.7.1. xCELLigence real-time toxicity assay
The xCELLigence assay is real-time and is based on a cell analysis system that employs impedance to measure cell viability. It uses 96-well plates with a coated gold electrode to quantify current across the base of a single well. A current impedance will be observed, as long as the cells are viable, and a graph is drawn to that effect. However, when the cells are no longer viable, no impedance current will be observed and no impedance graph is shown. Brain glioblastoma cells (U87MG) are grown via tissue culture techniques. The incubation of cells (1 × 105 cells/ml) in a gold electrode-coated 96 well E-plate was carried out at 37°C overnight, followed by the addition of the silver nanoparticles (AgNP05 and AgNP01), in 100, 50.0, 25.0, 12.5, 6.25, 3.125 and 0 μg/ml concentrations. The cells were allowed to incubate for 4 days, with impedance measurements observed at a different point in time during the incubation period. The data was recovered and a graphical representation of the test sample's toxicity was created. In all cases, the sample was run in triplicate and the average value was reported (Citation11, Citation27).
2.8. Statistical analysis
Data obtained from the analysis of the D. carota L. leaf extract (DCLE) and silver nanoparticles (AgNP05 and AgNP01) samples were subjected to statistical analysis using SPSS 17 software package (IBM cooperation, North Castle, NY, USA) and denoted as mean ± SD for triplicate experiments. One-way analysis of variance (ANOVA) was used for the analysis. Also, data analysis was carried out using Origin software (64-bit, riginLab Corporation, Northampton, MA 01060 USA).
3. Results and discussion
3.1. Biosynthesis of silver nanoparticles (AgNPs)
The reduction of silver ions into AgNPs by the D. carota L. leaf extract (DCLE), acting as a stabilizing and reducing agent was confirmed by a gradual change in color from colorless to pale-yellow/ cream to darkish brown precipitate. The bio-reduction activity can be attributed to the surface plasmon resonance phenomenon (). Biosynthesis of nanoparticles is advantageous over physical and chemical methods. This is because biosynthesis is cost-effective and eco-friendly as it does not necessarily involve the use of high energy, temperature, and toxic chemicals (Citation4, Citation6, Citation8, Citation10).
3.2. UV–vis analysis of synthesized AgNP05 and AgNP01 nanoparticles
UV-visible absorption spectra have been used to prove its significance and sensitivity towards silver colloids formation since silver nanoparticles display an intense absorption peak as a result of the surface plasmon (Citation13, Citation19). The appearance of darkish-brown coloration evident in the formation of silver nanoparticles (AgNP) in the mixture, and the effective bio-reduction of the Ag+ to Ag0, the formed color solution allowed the measurement of the absorbance against typical wavelength to affirm the preparation of silver nanoparticles. The distinct peak observed at 437 nm for AgNP05 and 429 nm for AgNP01 (), indicate the surface plasmon resonance (SPR) of the AgNPs (Citation6, Citation8, Citation16). The surface plasmon resonance (SPR) frequency is produced by an electric field of the incident electromagnetic (EM) radiation that displaces the electron of the particles from equilibrium, therefore, a restoring force is produced that results in the electrons oscillatory motion with a distinct frequency (Citation19, Citation20).
3.3. FT-IR analysis of synthesized AgNP05 and AgNP01 nanoparticles
The FTIR spectra of AgNP05 and AgNP01 () were recorded along the spectrum of the D. carota L. leaf extract (DCLE). An important feature in the spectra of AgNP05 and AgNP01 was the absence of a broad peak at 3291 cm−1 in the spectra of DCLE attributable to ν(O-H) vibrations from water molecule, phenolic and alcoholic compound. The FTIR spectra of DCLE and AgNP05 are displayed in . A slight shift was observed in the AgNP05 spectrum and the peaks intensity in the extract at 3291 cm−1, 2920 and 2843 cm−1, 1729, 1597, 1397, and 1015 cm−1 assignable to ν(OH), ν(CH3/ CH2), ν(C = O), ν(C = C), ν(C–N) and ν(C–O) vibrations, respectively, as this peaks became significantly reduced within the AgNP05 spectrum (Citation5, Citation31). This is a suggestion that these functional groups probably participate in the reduction, stabilization, and capping of the AgNO3 leading to the formation of AgNP05.
Figure 3. Comparative FTIR spectra of leaf extract, AgNP05 and AgNP01 obtained from D. carota L. (DCLE).
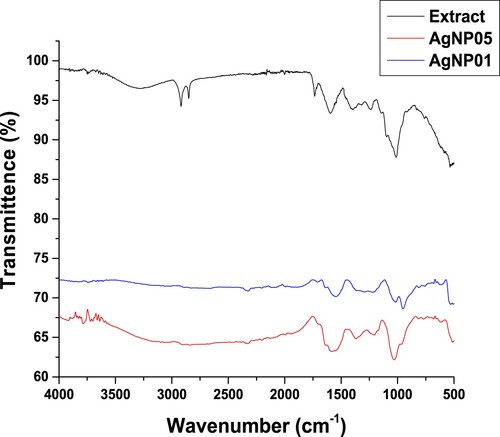
However, in the AgNP05 spectrum, the broadband around 3214 cm−1 is ascribed to ʋ(O-H) stretching, while the peaks at 1397 cm−1 shifted to 1369 and 1015 cm−1 shifted to 1029 cm−1 are attributable to the involvement of C–N stretch aromatic amines and C–O due to stretch of ethers on the bioreduction process (Citation8, Citation16, Citation31, Citation32). In the AgNP01 spectrum, 2760 and 1561 cm−1 are attributable to aliphatic ν(O-H) and ν(C–C) vibration frequencies within the aromatic ring (stretching vibrations of benzenoid), respectively (Citation16, Citation31). The shift in peaks from 1369 to 1350 cm−1 (C–N stretching for the aromatic amines) was observed, and a peak at 1033.3 cm−1 due to C–O stretchings of carboxylic acid, ether, alcohols, and esters indicating possible participation of these groups in the reduction of the silver salt (Citation6, Citation8, Citation14, Citation16, Citation31).
The disappearance of these prominent stretching vibrations in the AgNPs spectra showed that these are the main groups answerable for the capping and stabilizing of the synthesized AgNPs. Similar observations have been reported for the biosynthesis of AgNPs using leaf and seed extracts of Garcinia mangostana (Citation5), Lepidium draba (Citation6), Mimusops elengi (Citation7), Costus afer (Citation10), Ormocarpum cochinchinense (Citation11), Callistemon citrinus (Citation4), Tectona grandis (Citation13). The FT-IR further confirms that some distinctive phytochemicals such as polyphenols were answerable for the reduction and capping of AgNPs as shown from the assorted peaks described.
3.4. X-ray diffraction analysis
The crystalline nature of the AgNPs formed was confirmed. XRD spectra of silver nanoparticles mediated by aqueous extract of D. carota L. (DCLE) are displayed in . It was observed that the materials (AgNP05 and AgNP01) are crystalline in nature upon the bio-reduction of AgNO3. The AgNP05 synthesized from DCLE, ((a)) showed peaks at 2θ = 38.3, 46.4, 64.5, and 77.6° which were ascribed to the (111), (200), (220), and (311) face-centered cubic (FCC) structure of the silver in the 2θ range 20–80° (Citation19, Citation20).
Figure 4. X-ray diffraction patterns of synthesized (a) AgNP05 and (b) AgNP01 from D. carota leaf extract (DCLE).
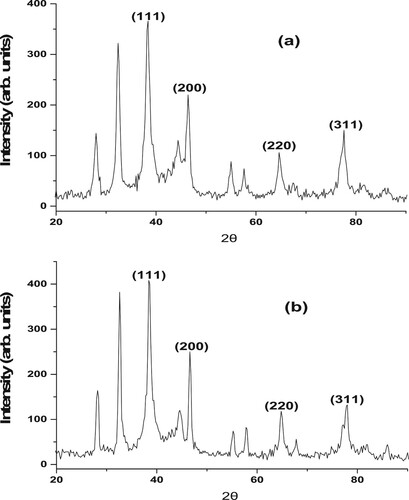
The XRD peaks for AgNP01 synthesized from DCLE ((b)) at 2θ = 38.5, 46.6, 64.9, and 77.8° was also be indexed to the (111), (200), (220), and (311) face-centered cubic (FCC) crystallographic planes of metallic silver. This is an act of pointing out that the synthesized silver nanoparticles (AgNPs) from an aqueous solution of DCLE were essentially crystalline in nature. The crystallite size of the nanomaterials with (111) diffraction peak via Scherrer formula identified that the synthesized materials are in nano-sized ranges. Diffraction peaks at (111), (200), (220), and (311) are answerable peaks of metal nanoparticles (Citation4, Citation20). Furthermore, residual peaks observed at 2θ values of 27.8° and 32.4° for AgNP05 and 2θ values of 28.0° and 32.6° for AgNP01 are an indication of successful synthesis of AgNPs from leaf extract. These peaks are ascribable to ascorbic acid existing in D. carota L. leaf extract (DCLE), and these diffraction peaks are well-matched with the standard data file JCPDS No 22–1536 (Citation19).
3.5. Transmission electron microscopy (TEM)
Transmission electron microscopy (TEM) was engaged for the characterization of the sizes, shapes, and morphologies of the designed AgNP05 and AgNP01 from D. carota L. leaf extract (DCLE). expresses the TEM micrograph of the silver AgNP05 ((a)) and AgNP01 ((b)). A limited agglomeration of Ag nanoparticles was evident in some places, thereby signifying probable sedimentation at a later period. As evident in the micrographs, it can be detected that spherical-shaped silver NPs were formed with a mean size distribution of 18.26 (±6.86) nm and 16.81 (±7.72) nm for AgNP05 and AgNP01 respectively. The average size achieved from TEM complements the result found from UV-visible and XRD spectral analyses confirming successful synthesis of the nanosized materials. Similar observations were reported by Rotimi et al. (Citation4), Elemike et al. (Citation10), Umadevi et al. (Citation19).
3.6. Antioxidant assay
Reactive oxygen species (ROS) are responsible for the pathological activities of diverse diseases like coronary heart disease, the aging process, hypertension, and Parkinson’s disease (Citation10, Citation31). Drugs that are antioxidants rich are administered to prevent free radical damage in the system. 2,2-diphenyl-1-picrylhydrazyl (DPPH) free radical was used for in vitro antioxidant assay of the synthesized nanosized materials spectrophotometrically. Vitamin C and Gallic acid were employed as standard agents. The ability of D carota L. leaf extract (DCLE) and silver nanoparticles (AgNP05 and AgNP01) to eliminate free radicals is an imperative property. The DPPH scavenging activity of the leaf extract (DCLE) was found higher than that of silver nanoparticles, indicating that functional groups of bioactive agents are responsible for the radical removal potential of the extract (). The radical scavenging potential of leaf extract (DCLE), AgNP05, and AgNP01 as well as the standards, were increased in a dose-dependent peculiarity. The violet color of DPPH solution transformed into yellow upon the annexation of test compounds at a time (t) as a result of test samples electron transfer to DPPH radical was described in the mechanism of reaction (), appropriating it into a stable hydrazine form (Citation10, Citation21, Citation33). IC50 and R2 (correlation coefficient) data of test compounds are reported in .
Figure 6. Mechanism of reaction for the action of silver nanoparticles (AgNP05 and AgNP01) as an antioxidant.
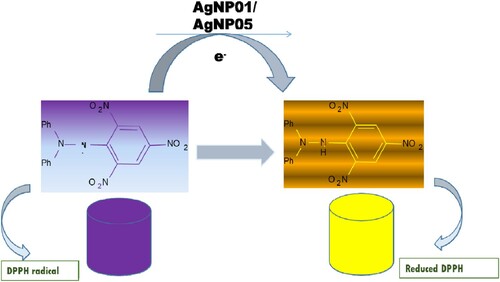
Table 1. Antioxidant activity of D carota L. leaf extract (DCLE) and silver nanoparticles against DPPH radical.
As observed in , at higher concentrations (300–500 μg/mL), the scavenging potentials of the silver nanoparticles increased significantly ranging from 57.38% to 75.71% for AgNP05 and 62.37% to 85.94% for AgNP01 due to the presence of Ag ion. Nevertheless, the leaf extract (DCLE), AgNP05, and AgNP01 exhibited lower scavenging potentials as compared to the standard compounds. The presence of hydroxyl group, carboxylic acid, ketone, esters, and ethers in the D carota L. leaf extract (DCLE) could also be responsible for the antioxidant property of the test compounds (Citation6, Citation10, Citation31). The order for the scavenging ability of the test samples can be given as (Gallic acid) > (Vitamin C) > (Extract (DCLE)) > (AgNP05) > (AgNP01) with IC50 values as 0.93 ± 0.68 > 1.97 ± 0.71 > 2.61 ± 0.58 > 2.79 ± 1.75 > 2.84 ± 0.96 µM. The anti-radical studies showed that the synthesized compounds are promising agents for averting cell oxidative damage and neurodegenerative diseases.
3.7. Antimicrobial activity
Antimicrobial activities of silver nanoparticles and D carota L. leaf extract (DCLE) against bacterial strains: Escherichia coli, Staphylococcus aureus, and α-hemolytic streptococcus, and fungi species: Aspergillus candideus, Aspergillus niger, and Penicillium cephalosporin were compared with the standard drug: ciprofloxacin and fluconazole (). A disk diffusion plate method was used to evaluate the bactericidal and fungicidal potentials of the bioactive ingredients of D carota leaf and silver nanoparticles (AgNP05 and AgNP01) (Citation8, Citation31). The zones of inhibition assessed in mm are recapitulated in . The nanoparticle synthesis by the green method was found to possess better activities against studied multi-drug resistant pathogens than the leaf extracts. AgNP01 from the leaf extract of D carota L. showed a zone of inhibitions: S. aureus (20 mm), α-H. streptococcus (21 mm), E. coli (14 mm), A. niger (15 mm), and P. cephalosporin (10 mm). Zone of inhibitions of AgNP05 were S. aureus (18 mm), α-H. streptococcus (17 mm), E. coli (14 mm), A. niger (15 mm), and P. cephalosporin (10 mm). The silver nanoparticles were active aginst the studies bacterial and fungi strains but showed no detectable activity was observed on A. candideus. Although the standard agents showed activities better than the test agents, the nanoparticles displayed higher bactericidal activity compared to the D carota L. leaf extract (DCLE) and showed favorable comparison with the anti-bacterial activity observed for ciprofloxacin. The synthesized AgNPs showed better antimicrobial properties () towards bacteria pathogens as compared to fungi pathogens under this investigation (Citation3, Citation4, Citation8, Citation31).
Figure 8. Antibacterial activities of D carota L. leaf extract (DCLE) and AgNPs (a), Antifungal activities of D carota L. leaf extract (DCLE) and AgNPs (b).
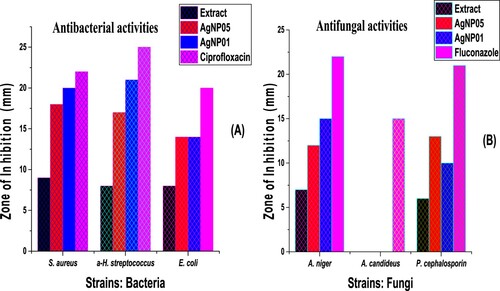
Table 2. Antimicrobial potential of D carota L. leaf extract (DCLE) and silver nanoparticles against bacteria and fungi pathogens.
The enhanced antimicrobial properties of silver nanoparticles (AgNP05 and AgNP01) could be related to the fact that the entrance of nanoparticles inside the cell causes cell wall damage, porosity, and ultimately resulting in necrobiosis, they tend to obstruct the growth signaling of the bacterial and bring about passageway by tempering the tyrosine phosphorylation of presumed peptides substrate vital for cell sustainability and detachment, attacking off the breathing chain, cell detachment finally leading to cell loss (Citation3, Citation5, Citation6, Citation10, Citation13, Citation34, Citation35). The enhanced bactericidal activity of AgNP05 and AgNP01 could be ascribed to their lipophilic character as exemplified by the model of Tweedy’s chelation and Overtone’s cell permeability theory (Citation10, Citation31, Citation33). Also, the microbial membrane encompasses sulfur and phosphorus-containing proteins compounds like DNA, and the AgNPs interaction with these proteins affects DNA replication, and thus deactivates diverse cellular enzymatic activities within the microbial cell (Citation1, Citation13, Citation34, Citation36).
Literature has shown that the antimicrobial activities of silver nanoparticles could be attributed to the silver (Ag) ion positive charge released from the nanoparticles, thus bringing about electrostatic attraction in the middle of the microorganism's negatively charged cell membrane and the positively charged AgNPs (Citation3, Citation5, Citation15, Citation17, Citation34). The oligodynamic effect of silver has been reported to possess antibacterial activity against microorganisms. This is largely due to the changes on the surface of the particles causing binding affinity towards the bacterial biomolecules, which in turn induces the penetration of cells and production of reactive oxygen species (ROS), that enhances their chemical reactivity leading to the antibacterial activity of AgNPs (Citation1, Citation6). Ogunsile et al. (Citation8), MubarakAlia et al. (Citation37), and Rotimi et al. (Citation4), also recounted moderate bactericidal activity of pure silver nanoparticles. Antibacterial effects of Ag nanoparticles as reported by Veerasamy et al. (Citation5) followed a dual-action phenomenon of antibacterial activity that is the anti-bacterial effect of Ag+ and membrane-disruptive outcome of the polymer subunits. A study by Rautela et al. (Citation13) has demonstrated that AgNPs using Tectona grandis seed by green synthesis possess significant bactericidal activity against E. coli and S. Aureus.
3.8. In vitro cytotoxicity evaluation
3.8.1. MTS end-point toxicity assay
The in vitro toxicity of silver nanomaterials (AgNP05 and AgNP01) was investigated against brain glioblastoma cells, U87MG at various concentrations stretching from 0.00 μg/ml to 100 μg/ml by MTS evaluation. The MTS assay was used to evaluate cell propagation, cell sustainability, and cytotoxicity. The MTS assay modus operandi is founded on the decline in the MTS (3-(4,5-dimethylthiazol-2-yl)-5-(3-carboxymethoxy-phenyl)-2-(4-sulfophenyl)-2H-tetrazolium) compound by feasible mammalian cells (and cells from additional species) to produce a colored soluble formazan dye in cell culture media. The MTS compound (yellow) was absorbed by feasible cells to form a dark-purple-colored complex, visible over UV-Vis spectrophotometer at 490 nm. The absorbance is directly proportionate to the cell viability. Low toxicity was observed for silver nanomaterials samples at the highest (100 μg/ml) concentration. The highest toxicity was observed for compounds AgNP05 confirmed by the effective dosages required to exhibit a 50% loss in cell viability (ED50), shown in . The nanomaterials showed a drastic increase in cell proliferation at concentrations 50.0, 25.0, and 12.5 μg/ml. This may be an indication of an attack on the cell nucleus and the cell attempt to rid itself of the toxin (Citation11, Citation38, Citation39).
Table 3. The ED50 (μg/ml) of silver nanomaterials (AgNP05 and AgNP01) against brain glioblastoma cells, U87MG.
3.8.2. xCELLigence real-time toxicity assay
The xCELLigence system is a kind of technology that supports accurate real-time monitoring of cell behavior. The system does not require any need to influence the medium during the experiment or the addition of any markers that may alter the cell function. Additionally, cells from this assay could be harvested from the plates for successive analysis (Citation40). xCELLigence analyzer was appropriate for real-time screening of the effect of silver nanoparticles viz: AgNP05 and AgNP01 from D. carota leaf extract (DCLE).in U87MG brain glioblastoma cells. In this study, xCELLigence real-time toxicity of AgNP05 and AgNP01 on U87MG cells showed growth inhibition and reduction of cell viability (), at concentrations (stretching from 0.00 μg/ml to 100 μg/ml). AgNP05 and AgNP01 tested against U87MG cells, showed toxicity toward the cell line, with AgNP05 exhibiting the greatest toxicity. AgNP01 showed concentration dependant toxicity; cell viability decreased with an increase in administered compound concentration (Citation11, Citation27, Citation39).
Figure 9. xCELLigence real-time toxicity for silver nanomaterials AgNP05 (a) and AgNP01 (b) on the U87MG cell line, at concentrations (100, 50.0, 25.0, 12.5, 6.25, 3.125 and 0 μg/ml).
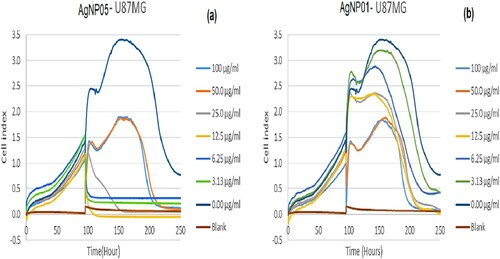
In a similar report by Le-Le et al. (Citation41), xCELLigence analyzer was used as an appropriate tool for real-time screening of the effect of alkaloids obtained from Rhizoma Coptidis in HepG2 cells. The xCELLigence system has been reported for evaluating motesanib (a small molecule inhibitor of vascular endothelial growth factor receptors) alone and in combination with the selective DuP-697 COX-2 inhibitor in an HT29 human colorectal cancer cell line (Citation42). In another study, the effect of callus extract of Chaenomeles japonica on viability, proliferation, and morphology of normal human skin fibroblasts has been investigated by Kikowska et al. (Citation43). It was conveyed that the extract caused a significant increase in the proliferation rate of the cells in comparison to control cells. Thus, indicating the potential of the extract as a cosmetic ingredient on human skin (Citation43).
4. Conclusion
An economical, efficient and environmentally friendly method has been used for the green synthesis of silver nanoparticles by D carota L. leaf extract (DCLE). Analytical techniques like UV–Vis spectrophotometer, XRD, FT-IR, and TEM techniques have established the bio-reduction of silver nitrate solution to silver nanoparticles. Spherical shapes of the synthesized silver nanoparticles were determined from TEM micrographs, and face-centered cubic phase with a mean particle size distribution of 18.26 ± 6.86 nm and 16.81 ± 7.72 nm for AgNP05 and AgNP01 respectively. The DPPH scavenging potential of the leaf extract (DCLE) was better in comparison to silver nanoparticles. Zones of inhibition (mm) obtained from the anti-microbial screening test, indicating that the silver nanomaterials (AgNP05 and AgNP01) synthesized in this study have an efficient antimicrobial activity against pathogenic bacteria but are less energetic against some pathogenic fungi. The in vitro MTS end-point and xCELLigence real-time toxicity assays of silver nanomaterials showed fewer toxicity activities on brain glioblastoma cells, U87MG. Therefore, isolation and identification of the bioactive components from the leaf extract of D carota L. would support the detection of an innovative anti-cancer drug. This method for the green AgNPs synthesis may find use in nanomedicine, environmental, and industrial applications as they possess greater effectiveness and reduced toxicity of an anticancer drug, therefore, further investigation will be implemented.
Disclosure statement
No potential conflict of interest was reported by the author(s).
Additional information
Funding
Notes on contributors
Ikechukwu P. Ejidike
Ikechukwu P. Ejidike holds an M.Sc. in Analytical/ Environmental Chemistry from the University of Ibadan and then proceeded to South Africa to pursue a doctoral degree. He obtained a Ph.D. in Chemistry (Inorganic/ Bio-inorganic Chemistry) from the University of Fort Hare, South Africa in 2016. His Ph.D. research activity focuses on the synthesis, characterization, and biological studies of metal complexes containing heterocyclic N- and Oligands for potential medicinal applications, and was a postdoctoral research fellow at the Vaal University of Technology, South Africa before taking up an appointment at the Anchor University, Lagos, and a NRF Research fellow at the University of South Africa. He has published over 45 peer-reviewed journal articles, 3 book chapters, and he is currently leading a thriving research group in the diverse applications of natural, inorganic, and nanomaterials in the university. He belongs to the following professional bodies and societies SACI, SAN, MSN, and SACNASP.
Hadley S. Clayton
Hadley S. Clayton received his Ph.D. in Chemistry from the University of Cape Town, South Africa. He joined the Department of Chemistry, College of Science, Engineering and Technology, University of South Africa, Florida campus, South Africa, where he is currently an associate professor of organometallic chemistry and Inorganic chemistry. He is currently working on the fabrication of organometallic compounds, their crystallography for advanced applications in various fields and biological activities. His area of interest includes Organometallic chemistry, Inorganic chemistry, Synthesis, Computational Chemistry, Coordination complexes, Organometallics, Conceptual theory, DFT Calculations. Prof. Clayton has published more than 50 peer-reviewed journal articles, many book chapters, and more than 30 national and international conference papers. He is leading a strong research group comprising of Post-docs and post-graduate candidates in the university. He is a member of several recognized international and national professional bodies.
References
- Salleh, A.; Naomi, R.; Utami, N.D.; Mohammad, A.W.; Mahmoudi, E.; Mustafa, N.; Fauzi, M.B. The Potential of Silver Nanoparticles for Antiviral and Antibacterial Applications: A Mechanism of Action. Nanomaterials (Basel) 2020, 10, 1566.
- Veisi, H.; Azizi, S.; Mohammadi, P. Green Synthesis of the Silver Nanoparticles Mediated by Thymbra Spicata Extract and its Application as a Heterogeneous and Recyclable Nanocatalyst for Catalytic Reduction of a Variety of Dyes in Water. J. Clean. Prod. 2018, 170, 1536–1543.
- Nisar, P.; Ali, N.; Rahman, L.; Ali, M.; Shinwari, Z.K. J. Clean. Prod. 2019, 24, 929–941.
- Rotimi, L.; Ojemaye, M.O.; Okoh, O.O.; Okoh, A.I. J. Mol. Liq. 2018, 273, 615–625.
- Veerasamy, R.; Xin, T.Z.; Gunasagaran, S.; Xiang, T.F.W.; Yang, E.F.C.; Jeyakumar, N.; Dhanaraj, S.A. Biosynthesis of Silver Nanoparticles Using Mangosteen Leaf Extract and Evaluation of Their Antimicrobial Activities. J. Saudi Chem. Soc. 2011, 15, 113–120.
- Benakashani, F.; Allafchian, A.; Jalali, S.A.H. Green Synthesis, Characterization and Antibacterial Activity of Silver Nanoparticles from Root Extract of Lepidium Draba Weed. Green Chem. Lett. Rev. 2017, 10, 324–330.
- Prakash, P.; Gnanaprakasam, P.; Emmanuel, R.; Arokiyaraj, S.; Saravanan, M. Green Synthesis of Silver Nanoparticles from Leaf Extract of Mimusops Elengi, Linn. for Enhanced Antibacterial Activity Against Multi Drug Resistant Clinical Isolates. Colloids Surf. B Biointerfaces 2013, 108, 255–259.
- Ogunsile, B.O.; Labulo, A.H.; Fajemilehin, A.M. Ife J. Sci. 2016, 18, 245–254.
- Pak, Z.H.; Abbaspour, H.; Karimi, N.; Fattahi, A. Eco-Friendly Synthesis and Antimicrobial Activity of Silver Nanoparticles Using Dracocephalum Moldavica Seed Extract. Appl. Sci. 2016, 6, 69.
- Elemike, E.E.; Fayemi, O.E.; Ekennia, A.C.; Onwudiwe, D.C.; Ebenso, E.E. Molecules 2017, 22 (701.
- Gnanavel, V.; Palanichamy, V.; Roopan, S.M. Biosynthesis and Characterization of Copper Oxide Nanoparticles and its Anticancer Activity on Human Colon Cancer Cell Lines (HCT-116). J. Photochem. Photobiol. B. 2017, 171, 133–138.
- Rivera-Rangel, R.D.; Gonzalez-Munoz, M.P.; Avila-Rodriguez, M.; Razo-Lazcano, T.A.; Solans, C. Green Synthesis of Silver Nanoparticles in oil-in-Water Microemulsion and Nano-Emulsion Using Geranium Leaf Aqueous Extract as a Reducing Agent. Colloids Surf. A: Physicochem. Eng. Aspects 2018, 536, 60–67.
- Rautela, A.; Rani, J.; Debnath (Das), M. Green Synthesis of Silver Nanoparticles from Tectona Grandis Seeds Extract: Characterization and Mechanism of Antimicrobial Action on Different Microorganisms. J. Anal. Sci. Technol. 2019, 10, 1–10.
- Rajan, R.; Chandran, K.; Harper, S.L.; Yun, S.; Kalaichelvan, P.T. Plant Extract Synthesized Silver Nanoparticles: An Ongoing Source of Novel Biocompatible Materials. Ind. Crops Prod. 2015, 70, 356–373.
- Espenti, C.S.; Krishna Rao, K.S.V.; Rao, K.M. Bio-synthesis and Characterization of Silver Nanoparticles Using Terminalia Chebula Leaf Extract and Evaluation of its Antimicrobial Potential. Mater. Lett. 2016, 174, 129–133.
- Elumalai, D.; Hemavathi, M.; Deepaa, C.V.; Kaleena, P.K. Evaluation of Phytosynthesised Silver Nanoparticles from Leaf Extracts of Leucas Aspera and Hyptis Suaveolens and Their Larvicidal Activity Against Malaria, Dengue and Filariasis Vectors. Parasite Epidemiol. Control 2017, 2, 15–26.
- Vijayakumara, M.; Priyab, K.; Nancyb, F.T.; Noorlidaha, A.; Ahmeda, A.B.A. Biosynthesis, Characterisation and Anti-Bacterial Effect of Plant-Mediated Silver Nanoparticles Using Artemisia Nilagirica. Ind. Crops Prod. 2013, 41, 235–240.
- Andleeb, A.; Andleeb, A.; Asghar, S.; Zaman, G.; Tariq, M.; Mehmood, A.; Nadeem, M.; Hano, C.; Lorenzo, J.M.; Abbasi, B.H. A Systematic Review of Biosynthesized Metallic Nanoparticles as a Promising Anti-Cancer-Strategy. Cancers (Basel) 2021, 13, 2818.
- Umadevi, M.; Shalini, S.; Bindhu, M.R. Synthesis of Silver Nanoparticle Using D. Carota Extract. Adv. Nat. Sci. Nanosci. Nanotechnol. 2012, 3, 025008. (6pp).
- Pattanayak, M.; Muralikrishnan, T.; Nayak, P.L. World J. Nano Sci. Tech. 2014, 3, 52–58.
- Ejidike, I.P.; Bamigboye, M.O.; Ijimdiya, R.U.; Seyinde, D.O.; Ojo, O.O. Proceed. Niger. Acad. Sci. 2020, 13, 136–147.
- Gour, A.; Jain, N.K. Advances in Green Synthesis of Nanoparticles. Artif. Cells Nanomed. Biotechnol. 2019, 47, 844–851.
- Mosaviniya, M.; Kikhavani, T.; Tanzifi, M.; Yaraki, M.T.; Tajbakhsh, P.; Lajevardi, A. Facile Green Synthesis of Silver Nanoparticles Using Crocus Haussknechtii Bois Bulb Extract: Catalytic Activity and Antibacterial Properties. Colloids Interface Sci. Commun. 2019, 33, 100211.
- Nakkala, J.R.; Mata, R.; Gupta, A.K.; Sadras, S.R. Green Synthesis and Characterization of Silver Nanoparticles Using Boerhaavia Diffusa Plant Extract and Their Anti Bacterial Activity. Indus. Crop Prod. 2014, 52, 562–566.
- Kathiravan, V.; Ravi, S.; Ashokkumar, S. Synthesis of Silver Nanoparticles from Melia Dubia Leaf Extract and Their in Vitro Anticancer Activity. Spectrochim. Acta A Mol. Biomol. Spectrosc. 2014, 130, 116–121.
- Sadeghi, B.; Rostami, A.; Momeni, S.S. Facile Green Synthesis of Silver Nanoparticles Using Seed Aqueous Extract of Pistacia Atlantica and its Antibacterial Activity. Spectrochim. Acta A Mol. Biomol. Spectrosc. 2015, 134, 326–332.
- Swamy, M.K.; Akhtar, M.S.; Mohanty, S.K.; Sinniah, U.R. Synthesis and Characterization of Silver Nanoparticles Using Fruit Extract of Momordica Cymbalaria and Assessment of Their in Vitro Antimicrobial, Antioxidant and Cytotoxicity Activities. Spectrochim Acta A Mol. Biomol. Spectrosc. 2015, 151, 939–944.
- Nouri, A.; Yaraki, M.T.; Lajevardi, A.; Rezaei, Z.; Ghorbanpour, M.; Tanzifi, M. Ultrasonic-assisted Green Synthesis of Silver Nanoparticles Using Mentha Aquatica Leaf Extract for Enhanced Antibacterial Properties and Catalytic Activity. Colloids Interface Sci. Commun. 2020, 35, 100252.
- Brglez Mojzer, E.; Knez Hrnèiè, M.; Škerget, M.; Knez, Ž; Bren, U. Polyphenols: Extraction Methods, Antioxidative Action, Bioavailability and Anticarcinogenic Effects. Molecules 2016, 21, 901.
- Ayeni, E.A.; Ahmed, A.; Ibrahim, G.; Vallada, A.; Muhammad, Z. Phytochemical, Nutraceutical and Antioxidant Studies of the Aerial Parts of Daucus Carota L. (Apiaceae). J. Herbmed. Pharmacol. 2018, 7, 68–73.
- Ejidike, I.P.; Bamigboye, M.O.; Clayton, H.S. Spectral, in Vitro Antiradical and Antimicrobial Assessment of Copper Complexes Containing Tridentate Schiff Base Derived from Dihydroxybenzene Functionality with Diaminoethylene Bridge. Spectrosc. Lett. 2021, 54, 212–230.
- Ullah, N.; Ullah, A.; Rasheed, S. Lett. Appl. NanoBioScience 2020, 9, 897–901.
- Akila, E.; Usharani, M.; Rajavel, R. Int. J. Pharm. Sci. 2013, 5, 573–581.
- Logeswari, P.; Silambarasan, S.; Abraham, J. Synthesis of Silver Nanoparticles Using Plants Extract and Analysis of Their Antimicrobial Property. J. Saudi Chem. Soc. 2012, 19, 311–317.
- Mittal, J.; Singh, A.; Batra, A.; Sharma, M.M. Synthesis and Characterization of Silver Nanoparticles and Their Antimicrobial Efficacy. Particulate Sci. Technol. 2016, 35, 338–345.
- Prabhu, S.; Poulose, E.K. Int. Nano. Lett. 2012, 8, 526–537.
- MubarakAlia, D.; Thajuddina, N.; Jeganathanb, K.; Gunasekaranc, M. Plant Extract Mediated Synthesis of Silver and Gold Nanoparticles and its Antibacterial Activity Against Clinically Isolated Pathogens. Colloids Surf., B. 2011, 85, 360–365.
- Devaraj, P.; Kumari, P.; Aarti, C.; Renganathan, A. Synthesis and Characterization of Silver Nanoparticles Using Cannonball Leaves and Their Cytotoxic Activity Against MCF-7 Cell Line. J. Nanotechnol. 2013, 2013, 1–5.
- Ejidike, I.P.; Ajibade, P.A. Synthesis, Characterization, and in Vitro Antioxidant and Anticancer Studies of Ruthenium(III) Complexes of Symmetric and Asymmetric Tetradentate Schiff Bases. J. Coord. Chem. 2015, 68, 2552–2564.
- Stefanowicz-Hajduk, J.; Ochocka, J.R. Real-time Cell Analysis System in Cytotoxicity Applications: Usefulness and Comparison with Tetrazolium Salt Assays. Toxicol. Rep. 2020, 7, 335–344.
- Zhang, L.; Ma, L.; Yan, D.; Zhang, C.; Gao, D.; Xiong, Y.; Sheng, F.; Dong, X.; Xiao, X. Chin. J. Nat. Med. 2014, 12, 0428–0435.
- Kaya, T.T.; Altun, A.; Turgut, N.H.; Ataseven, H.; Koyluoglu, G. Effects of a Multikinase Inhibitor Motesanib (AMG 706) Alone and Combined with the Selective DuP-697 COX-2 Inhibitor on Colorectal Cancer Cells. Asian Pac. J. Cancer Prev. 2016, 17, 1103–1110.
- Kikowska, M.A.; Chmielewska, M.; Włodarczyk, A.; Studzińska-Sroka, E.; Żuchowski, J.; Stochmal, A.; Kotwicka, M.; Thiem, B. Effect of Pentacyclic Triterpenoids-Rich Callus Extract of Chaenomeles Japonica (Thunb.) Lindl. ex Spach on Viability, Morphology, and Proliferation of Normal Human Skin Fibroblasts. Molecules 2018, 23, 3009.