ABSTRACT
Quinolines are a prominent heterocyclic motif and crucial building blocks in creating physiologically active compounds. Due to the fast development of novel medicines with a quinoline nucleus, numerous research papers have been published in a short amount of time. Therefore, to comprehend the present state of the quinoline nucleus in medicinal chemistry science, it is necessary to combine new information with older data. So far, several traditional synthesis techniques have been reported in the literature to synthesize this scaffold. Pfitzinger, Gould–Jacob, Friedlander, Skraup, Doebner–von Miller, and Conrad–Limpach are examples of old synthetic methods. However, they need expensive and demanding conditions, such as high temperature, the use of non-biodegradable chemical compounds degrade the ecosystem, create irritation or harm as pollutants, and represent a threat to the environment. However, traditional synthesis processes need a difficult and time-consuming apparatus set-up, resulting in high costs and pollutants. As a result, scientists are presently developing new and innovative techniques to decrease the use of chemicals, solvents, and catalysts, which are detrimental to both humans and the environment. Therefore, we have attempted to shed light in this current review on various reactions to produce quinolines and their derivatives using various green synthetic methods.
GRAPHICAL ABSTRACT
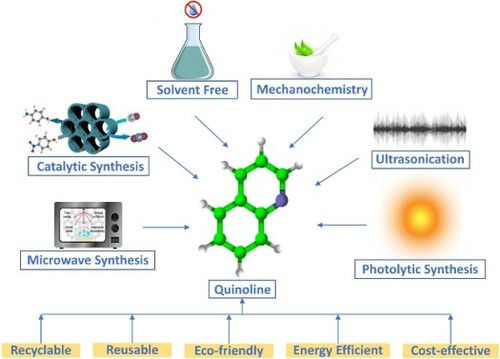
1. Introduction
Heterocyclic compounds have been extensively used in medicinal chemistry. Their uses are escalating by the day since they are being analyzed in multifold architectures of the bioactive compound. Quinoline and derivatives belong to the N-containing heterocycles family that has lately attracted the interest of researchers due to their wide variety of applications, such as the diverse spectrum of activities and their numerous uses in industrial and synthetic organic chemistry (Citation1–5). Quinoline is a weak tertiary base that can react with acids to produce salts and is widely recognized as 1-aza-naphthalene or benzo[b]pyridine (). Its reaction is analogous to pyridine, and benzones may participate in electrophilic and nucleophilic substitution processes (Citation6).
In 1834, Friedlieb Runge discovered quinoline as a colorless hygroscopic liquid by distillation of coal tar. Nevertheless, its basic heterocyclic core structure was first revealed in 1871 when Dewar noticed the chemical similarities between quinoline and pyridine (Citation7). Since it was first uncovered, coal tar has remained the primary source of commercial quinoline, despite the development of numerous processes for its synthesis (Citation8,Citation9). Moreover, several derivatives with noteworthy biological activity have been isolated and/or synthesized from plants (Citation10,Citation11). Due to their rapid tautomerism to 2-hydroxyquinolines and 4-hydroxyquinolines, the synthetic pathway for synthesis of quinoline-2(1H)-one and quinoline-4(1H)-one derivatives is reported in organic chemistry ()(Citation12).
Different biological functions have been discovered in quinoline derivatives like anti-cancer, anti-malarial (Citation13,Citation14), anti-analgesics (Citation15), anti-tubercular (Citation16), anti-bacterial (Citation16), anti-protozoal (Citation17), anti-glycemic (Citation18), anti-inflammatory (Citation19), anti-fungal (Citation20), anti-hypertensive (Citation21), anti-HIV (Citation22), and anti-helmentic (Citation23). There are a few promising compounds with the quinoline ring system like pamaquine (A), chloroquine (B), tafenoquine (C), bulaquine (D), quinine (E), mefloquine (F), and amodiaquine (G) as an antimalarial agent. Furthermore, the 2-arylquinoline derivatives (H) and (I) bind to the Estrogen receptor b with selectivity. In recent years, several quinoline derivatives have been developed as new Raf kinase inhibitors with more powerful and selective anticancer effects, based on structural modifications of Sorafenib (J) () (Citation24–28).
2. Conventional synthetic approach for quinolines and derivatives
Quinolines have been synthesized using a range of methodologies. The most widespread is the Skraup synthesis technique (Citation29), which includes heating anilines with glycerol in the presence of sulphuric acid, ferrous sulphate, and N-nitrobenzene (this is the most common and widely used method due to the wide range of available substituents). Moreover, Doebner-von Miller (Citation30), Conrad-Limpach-Knorr (Citation31), and Combes (Citation32) synthesis are alternative ways to synthesize quinoline derivatives, whereas Friedlander (Citation33), Pfitzinger (Citation34), and Niementowski synthesis employ ortho-substituted quinoline derivatives (). However, even though many of these techniques are quite successful, they are not environment-conscious since they create large amounts of detritus that must be disposed of and require a high level of operational complexity to isolate the output (Citation35).
Furthermore, many of these techniques produce significant quantities of unwanted side products, whose removal is time-consuming, invariably wasteful, and insufficient to isolate the yield, as the procedure is simple. As a result, it is evolved into critical to adopt a protocol that might be regarded as superior and more environmentally friendly feasible ‘green synthetic’ methods. This method can solve environmental pollution as global warming with reduction of chemical consumption and reaction time. This review highlights all the green synthetic methods devised to synthesize quinoline scaffolds that would help researchers in the future in organic and medicinal chemistry.
3. Green synthetic approach for the synthesis of quinolines and its derivatives
It is crucial to adapt and incorporate new green chemistry methodologies into our routine procedures to improve yield, selectivity, productivity, energy, life, and time (Citation36). The use of organocatalysts, pollution reduction, safer chemicals, less hazardous synthesis, waste prevention substances, and goods that are biodegradable was among the twelve principles of ‘Green Chemistry’ formulated in the 1990s to fulfill the current generation demands without jeopardizing the future generation needs (Citation37,Citation38).
3.1. Microwave-assisted quinoline synthesis
Many chemical and pharmaceutical industries are currently grappling with environmental issues such as large amounts of solvent waste, loss of solvents/reagents, the presence of a catalyst in the product, and so on. Green synthesis is the most efficient and preferred approach for synthesis that involves a microwave-assisted reaction to overcome these issues. Microwave transfers energy directly to the reactive species known as molecular heating by two mechanisms, (i) dipole rotation and (ii) ionic conduction. The use of microwave irradiation as an energy source, on the other hand, reduces the need for solvents in processes (refer ).
Table 1. Outline for Microwave-assisted quinolines synthesis (Citation39–57).
Saggadi et al. have developed a ‘one-pot eleven steps’ reaction by microwave-assisted regioselective modified Skraup reaction and Bamberger rearrangement reacting with glycerol, nitrobenzene, and p-amino/p-nitrophenol in water as a green solvent for synthesizing 6-hydroxyquinoline in 15–20 min (). The % yield of the target compound obtained through p-aminophenol and p-nitrophenol are respectively 27% and 55%, while nitrobenzene gave 77% (Citation39).
Scheme 1. Microwave-assisted green synthetic approach for quinoline synthesis in water as a green solvent.
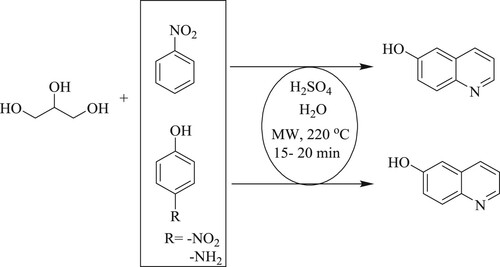
Yu et al. have developed one-pot synthetic protocol using a three-component reaction of aldehydes and 1-aryl ethylidene malononitriles in ethane-1,2-dione at 100°C in the presence of sodium hydroxide as a base catalyst to synthesize novel poly-functionalized dihydroquinoline derivatives with good yields (75–86%) (). This protocol has the following features: Mild condition, a cheaper catalyst, fast reaction timeframes of 8–20 min, and good regioselectivity (Citation40).
Li et al. have developed an eco-friendly synthetic approach for the synthesis of polyfunctionalized tetracyclicindolo[2,3-b]quinoline derivatives by cycloaddition reaction of 3-arylidene-2-oxindoles with enaminones using sodium ethoxide in a polar protic solvent like ethanol at 110°C for 12 min under microwave irradiation with excellent yields (73–86%) (). This tactic has the following features: optimum process efficiency, quick reaction, operational simplicity but irksome work-up, and intermediate separation (Citation41).
Saggadi et al.have developed an effective one-pot, green Skraup reaction in water using affordable, plentiful, and ecological-friendly glycerol with substituted anilines at 200°C in presence of catalytic H2SO4 under the influence of microwave irradiation for 15–20 min (). The % yield of the target compound obtained through p-aminophenol is 10–66%, whereas nitroaniline is 15–52% (Citation42).
Chidurala et al. have designed a simple, catalyst-free, greener, one-pot multi-component condensation reaction of benzene-1,3-diol, aldehyde, ammonium acetate, and acetoacetanilide in ethanol for the production of quinoline derivatives with an excellent yield of 88–96% under microwave for 8–10 min as compared to the classical method in which % yield was found to be 72–90% in 4–6 h reaction time () (Citation43).
Zangh et al. have developed a one-pot catalyst-free method for the synthesis of fused-quinoline and quinoline dicarboxylates using the best efficient microwave-assisted process involving a cascade of denitrogenationazide, benzisoxazole formation, aza-Diels–Alder cycloaddition, and dehydrative aromatization with a high yield between 75–93% (). This method has following features: catalyst-free synthesis and efficient methodology (Citation44).
Fedoseev et al. have developed a metal-free and Bronsted acid-promoted method for the production of 3,4-cyclopentane-quinoline-3-ones from indolyl-one in the presence of trifluoroacetic acid [TFA] in chloroform under microwave irradiation at 100°C for 30 min with a medium to excellent yields (68–99%) () (Citation45).
Scheme 7. Microwave-assisted protocol for quinoline synthesis using trifluoroacetic acid in chloroform.

Ma et al. have reported a neat and efficient method for the synthesis of quinoline derivatives by reaction of ferrocene carboxaldehyde with dimedone and ketone catalyzed by ammonium acetate using water as a green reaction media via microwave (100°C) for 10–15 min. Moreover, the % yield obtained using water was 75–93%, while, with glycol, it was 32% (). The advantages of this reaction are economical, potential, and covers a short reaction period (Citation46).
Scheme 8. Synthesis of quinoline derivatives using water as green solvent under the microwave method.
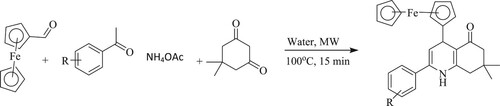
Malvacio et al. have reported a method for the synthesis of 3-carboethoxy-quinoline-4-one from substituted aniline and diethyl malonates via Gould–Jacobs (G–J) cyclization using the flash vacuum pyrolysis protocol at 330 °C for 1 h under a nitrogen atmosphere, yielding a moderate yield of 40–45% (). The tactic of this feature includes a short period of time, but the disadvantage of this method is its non-applicability for industrial use and its cost(Citation47).
Libertoet al. have developed a suitable and simple one-pot microwave synthesis of 2,4-disubstituted quinolines from a series of substituted anilines, benzaldehyde derivatives, and styrene under solvent-free conditions in the presence of p-sulfonic acid calix[4]arene [CX4SO3H] as a catalyst, yielding a good yield (38–78%) over 20-min. Recycling catalysts, tolerance to a wide range of functional groups, and solvent-free are all tactic features of these approaches () (Citation48).
Scheme 10. Microwave reported synthesis of substituted quinoline using p-sulfonic acid calix[Citation4]arene catalyst.
![Scheme 10. Microwave reported synthesis of substituted quinoline using p-sulfonic acid calix[Citation4]arene catalyst.](/cms/asset/954608d8-9dcf-406c-99b9-a62640fdae71/tgcl_a_2064194_f0014_ob.jpg)
Ojer et al. have reported an alluring and impactful metal-free Friedlander synthesis of trisubstituted quinolines by the reaction of substituted 2-amino benzaldehyde and ethyl acetoacetate using nano-carbon aerogels as a catalyst via microwave method for 4 h, resulting in a moderate yield (60–65%) () (Citation49).
Cravotto and coworkers have developed a solvent-free microwave-assisted method for the synthesis of polysubstituted quinoline derivatives from o-amino benzophenone derivatives and carbonyl compounds using functionalized propylsulfonic acid [SiO2-Pr-SO3H] as a recyclable and reusable catalyst with a low to high yield (22–93%) for 30–210 min at 80 °C () (Citation50).
Scheme 12. Friedlander synthesis of polysubstituted quinoline using propylsulfonic acid as recyclable catalyst.
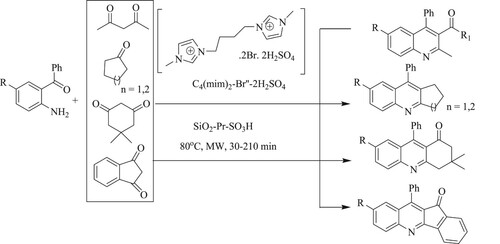
Anvar and his team have developed one-pot, three-component, solvent-free rapid synthesis of quinolines and bis-quinolines usingmicrowavebyreaction between aromatic amines, aromatic aldehydes, and phenylacetylene in the presence of potassium dodecatungstocobaltate trihydrate [K5CoW12O40·3H2O] as a recyclable catalyst that can be used five to six times without significant loss of catalytic activity, resulting in an excellent yield of 87–98% in 10 min () (Citation51).
Scheme 13. Microwave-assisted synthesis of quinolines using potassium dodecatungstocobaltate trihydrate as a catalyst.
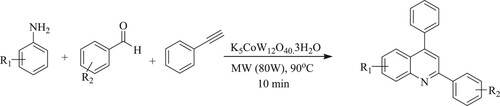
Jiang et al. have synthesized a highly diastereoselective three-component reaction of 4-hydroxy pyran-2-ones, aromatic aldehydes, and N-aryl enaminones to afford bicyclic hexahydro quinoline-2,5-diones via the Knoevenagel condensation reaction under microwave irradiation at 100 °C for 20 min, resulting in a yield varying between 65–67% (). This outlined method has the advantage of lenient conditions, pliability of structural modification, reliability, and scalability (Citation52).
Kulkarni and Torokhave developed a three-component reaction of anilines, aldehydes, and 4-substituted phenyl acetylenes to produce substituted quinolines quickly and efficiently using a powerful and ecologically friendly solid acid catalyst like montmorillonite K-10 using microwave technique to produce a yield with nearly 72–96% in 10 min () (Citation53).
Scheme 15. Microwave reported synthesis of substituted quinolines using montmorillonite K-10 catalyst.
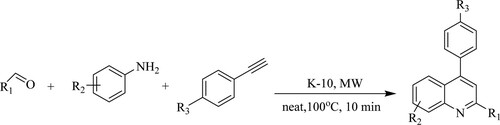
Kyung et al. have applied safe, straightforward, and ecologically friendly reactions for quinoline derivatives via microwave technique of 2-aminobenzophenone with heteroaromatic ketones through Friedlander condensation in the presence of diphenylphosphate [DPP] as a catalyst, resulting in high yields varying between 40–78% in 3–5 min (). Moreover, the % yield of side product 2-aminobenzophenone, i.e. dibenzo[b,f][1,5]diazocines, was only 9–16% (Citation54).
Peng et al. have synthesized a sequence of furo[3,4-b]indeno[2,1-f]quinolin-1-one derivatives by the condensation of an aromatic aldehyde, tetronic acid, and 9H-fluoren-2-amine in the presence of a weak acid as glacial acetic acid via microwave irradiation, forming a high product yield (79–88%) in 15 min () while, with other solvents systems such as water and glycol gave minimal yield (12–35%). The advantages of this method are: functional simplicity, neat reaction, and increased safety for small-scale fast synthesis (Citation55).
Albert-Soriano et al.have used a heterogeneous catalyst based on alkali earth metals like barium and calcium to manufacture quinoline derivatives by combining 2-amino aryl aldehydes or 2-amino aryl ketones via microwave technique at 80 °C without using solvent, resulting in a high yield of 67–99% () (Citation56).
Yun Li et al. have reported a microwave protocol for the production of a fused quinoline by reacting ortho-heteroaryl anilines and carbon disulfide[CS2] in the presence of green solvents like water through 6π-electrocyclization at 140 °C for 30 min () (Citation57).
3.2. Green solvent-based approach for quinoline synthesis.
Toxic solvents are used in large quantities during chemical synthesis for cleaning and degreasing. However, the use and discharge of chlorinated solvents are hazardous to human health and the environment. A contrario green solvents are non-toxic and environmentally friendly solvents. These solvents are biodegradable and easily generated from natural renewable resources. Therefore, for the synthesis of quinoline and its derivatives, water, ethanol, isopropanol, ionic liquids, deep eutectic solvents (DES), and choline azide are employed as green solvents (refer ).
Table 2. Outline for quinolines synthesis using green solvents (Citation58–83).
Ramesh et al. have developed a one-pot multi-component synthetic approach for tetrahydroquinoline by reacting ethyl acetoacetate, dimedone, and ammonium acetate with aromatic aldehydes using silica iodide [SiO2-I] as a catalyst and ethanol as a solvent under reflux for 2–3 h at 80°C to obtain excellent yield of 90% (). This protocol's features include high atom ability and recoverability of the catalyst (Citation58).
Meena et al. have developed a green strategy of spiro-quinoline through a one-pot three-component condensation of enaminones with substituted isatin derivatives and indane-1,3-dione in ethanol:water (green solvent) using ceric ammonium nitrate [CAN] catalyst, obtaining highly substituted spiro-quinolines with a yield between 84–94% in 10–40 min () (Citation59).
Teimouri et al. have designed a highly multifaceted, rapid, and straightforward synthetic route for poly-substituted quinolines via Friedlander condensation of 2-amino aryl ketones, carbonyl compounds, and β-keto esters catalyzed by Montmorillonite K-10 (MK-10), Zeolite, or nano-crystalline sulfated zirconia [NC-SZ] in green ethanol solvent under mild conditions with yields obtained as follows: MK-10(70–83%), Zeolite(81–92%), NC-SZ(88–93%) () (Citation60).
Mansoor et al. have used eco-friendly green solvent (ethanol) based simple four-component coupling reaction of poly-hydro quinoline derivatives using gadolinium triflate [Gd(OTf)3] as a reusable catalyst in a Hantzsch reaction involving aldehydes, 5,5-dimethyl-1,3-cyclohexanedione [dimedone], ethyl acetoacetate, and ammonium acetate at room temperature to obtain 83–89% yield in 5–6 h () (Citation61).
Karnakar et al. have reported green solvent and catalyst based efficient, and environmentally-friendly approach for the one-pot three-component reaction of aldehydes, aminopyrazole, and 1,3-cyclohexanediones in the presence of polyethylene glycol [PEG]−400 in water as a recyclable catalyst to obtain pyrazolo[3,4-b]quinoline derivatives affording an excellent yield between 76–92% at 100–110 °C for 4 h (). The tactical features include a recyclable solvent medium and less hazardous waste (Citation62).
Pasha et al. have developed an eco-friendly technique to synthesize quinolone derivatives through Povarov reaction between aromatic aldehydes, N-arylidene-1H-indazol-6-amines, and substituted indoles catalyzed by reusable Amberlyst-15 catalyst in ethanol solvent at room temperature, resulting in a yield between 65–70% () (Citation63).
Scheme 25. Green solvent-based synthesis of quinoline derivatives in reusable Amberlyst-15 catalyst.
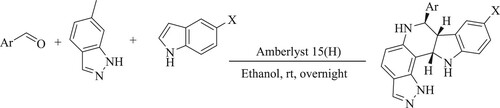
El-Remaily and his team have described one-pot multicomponent reactions of tetrahydrobenzo[h][1,3]thiazolo[4,5-b]quinolin-9-one with excellent yields (84–93%) from α-naphthylamine, aromatic aldehydes, and 2,4-thiazolidinedione in water: ethanol (3:1) green solvent system in the presence of cobalt-ferrite [CoFe2O4] magnetic nano-particles [MNPs] at 120 °C for 30–50 min (). The tactical features: environmentally benign and efficient, no need for surfactant and capping agents, and a catalyst to be reusable and recyclable (Citation64).
Jaydip Gosh et al.have reported a potent and green protocol for 5-aryl-3,3-dimethyl-2,3-dihydro-5aH-chromeno[2,3-b]quinoline-1,11(4H,5H)diones through athree-component reaction involving chromone-3-carbaldehydes, aromatic amines, and 5,5-dimethyl-1,3-cyclohexanedione [dimedone] using 1.8 M aqueous tetra-n-butylammonium bromide [TBAB] solution as a catalyst for 10 min at 70–80 °C to obtain excellent yields 85–95% () (Citation65).
Verma et al. have developed one-pot three-component technique for pyrimido[4,5-b]quinolones synthesis by reaction of 6-amino-1,3-dimethyluracil, aldehydes, and dimedone in an eco-friendly green solvent like water at 90°C for 2.5–3.5 h catalyzed by p-toluene sulfonic acid [p-TSA] catalyst with an afforded yield of 60–94% () (Citation66).
Shahrzad et al. have reported a highly efficient and eco-friendly green solvent-based one-pot four-component coupling method for the synthesis of indeno[1,2-b]quinolinone derivatives by the reaction of 1,3-indanedione, aromatic aldehydes, primary amines, and dimedone catalyzed by recyclable catalyst titanium dioxide nano-particles [TiO2-NPs] at 80°C in aqueous media for 2 h with an excellent yield varying between 95–98% () (Citation67).
Nemati and Saeedirad have developed a green, efficient one-pot synthetic route of pyrimido[4,5-b]quinolines from the reaction mixture of 6-amino-1,3-dimethyl uracil, aryl aldehydes, 1,3-diketonescatalyzed by a magnetic nano-particles silica sulfuric acid [nano-Fe3O4@SiO2–SO3H] in water under lenient conditions (70 °C) for 25–40 min to obtain moderate to excellent yields(71–92%) () (Citation68).
Scheme 30. Nano-catalyst and green solvent (water) based synthetic approach for substituted quinolines synthesis.

Arenas et al. have reported a new environmental technique to produce diastereospecific cis-4-amidyl-2-methyl-1,2,3,4-tetrahydroquinolines [THQs] in acidified mixtures through Diels–Alder reaction using a surfactant such as sodium dodecyl sulphate [SDS] in a green solvent like water at room temperature for 1–8 h to obtain moderate to excellent yields (70–99%) () (Citation69).
Anand et al. have developed a one-pot reaction for the synthesis of substituted quinolines through a modified Friedlander reaction of bromo aromatic benzaldehydes or ketone derivatives, sodium azide, and active methylene substrates in the presence of a water–ethanol mixture and copper sulphate [CuSO4–D-glucose] as a catalyst for 3–12 h to obtain moderate to excellent yields (76–95%) (). Moreover, the catalyst is air-stable, eco-efficient, and affordable (Citation70).
Scheme 32. CuSO4–D-glucose catalyzed modified Friedlander reaction for quinoline synthesis in a green solvent.
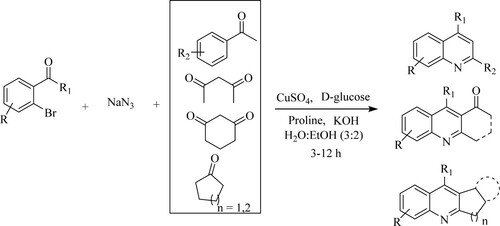
Mohammadi et al. have carried out an easy appropriate one-pot three-component condensation reaction for the synthesis of pyrimido[4,5-b]-quinoline derivatives with excellent yields (82–95%) by reacting 6-amino-1,3-dimethyluracil, aromatic aldehydes, and cyclic 1,3-diketones using 1,3-disulfonic acid imidazolium hydrogen sulphate{[dsim]HSO4} as a reusable and eco-friendly catalyst in the presence of a green solvent like ethanol at 70°C for 15–35 min () (Citation71).
Paplal et al. have reported the synthesis of functionalized poly-hydroquinolines by reacting substituted aromatic aldehydes, ethyl acetoacetate, dimedone, and ammonium acetate in an aqueous medium catalyzed by russellite [Bi2WO6] nano-particles for 10 min at room temperature with excellent yields (82–95%) () (Citation72).
Scheme 34. Green solvent-based reaction of functionalized poly-hydroquinolines using Bi2WO6 as heterogeneous catalyst.

Siddiqui et al.have reported the rapid and convenient synthesis of pyrimido[4,5-b]quinolines from substituted aryl aldehydes, 2-hydroxynaphthalene-1,4-dione and 6-aminouracil or 6-amino-1,3-dimethyluracil catalyzed by recyclable nano-catalyst Zinc Oxide [ZnO] using cetyltrimethylammonium bromide [CTAB-H2O] in water as reaction medium at 80°C for 4 h to obtain a high yield of product (>90%) () (Citation73).
Sadhupriya et al. have developed a one-pot synthesis for the formation of substituted quinolines via coupling reaction using Copper iodide (CuI) followed by reductive cyclization of 2-nitrobenzaldehydes, piperidine, and alkyne derivatives in the presence of ethanol as solvent and stannous chloride dihydrate [SnCl2·2H2O] as a catalyst at 80 °C for 2 h that provides good to moderate yields between 72–87% () (Citation74).
Abdelhamid et al.have reported a cautious, easy, and rapid method, a one-pot three component cyclo condensation reaction for the synthesis of hexahydro quinoline derivatives by reacting 1,3-cyclohexanedione with primary amines and arylidene malononitrile or salicylaldehyde derivatives under reflux conditions using ethanol as a solvent and a catalytic amount of triethylamine [TEA] as a base for 6–8 h providing low to moderate yields of 50–83% () (Citation75).
Mou et al. have developed the efficient green synthesis of 4-ferrocenyl quinoline derivatives with a good yield varying between 69 and 95% by the reaction of aromatic aldehydes, amines, and ferrocenyl acetylene utilizing water as a green solvent and p-toluene sulfonic acid [p-TSA] as a catalyst under reflux conditions (). The features’ tactic includes the use of a minimal amount of a metal catalyst and organic solvent (Citation76).
Luo et al. have developed an eco-friendly reaction for the synthesis of quinolines via a three-component cascade Mannich addition with C–C formative cyclization utilizing a zirconocene dichloride [Cp2ZrCl2], trimellitic acid as a synergic catalyst, and copper oxide as collaborative and relay catalysis system by the reaction of aniline derivatives, aldehydes, and ketones with isopropanol and water (3:1) as a green solvent system at 60 0C for 2 h to obtain an excellent yield of 90–96% () (Citation77).
Scheme 39. Cascade Mannich addition reaction for substituted quinoline synthesis using an eco-friendly catalyst and green solvent.

Khan et al. have developed an iodine[I2] catalyzed one-pot synthesis of functionalized chromeno[3,4-b]quinolines by reacting 3-aminocoumarins, cyclic 1,3-diketones, and aromatic aldehydes or isatins in ethanol under reflux conditions to obtain moderate to excellent yields of 80–85% (). The tactic of these features involves easy screening without the need for time-consuming chromatographic separation, a quicker reaction time, and a minimal, environment-conscious catalyst (Citation78).
Guangzhou et al. have developed the production of 1′,7′,8′,9′-tetrahydrospiro [indoline-3,4′-pyrazolo[3,4-b]quinoline]-2,5′(6′H)-dione derivatives using substituted isatins, 3-amino-1H-pyrazole (5-methyl-1H- pyrazol-3-amine) and 1,3-diketone as starting reactants at 90°C for 5–7 h utilizing the ratio of water and acetic acid (1:1) as a solvent to obtain high yields of 87–95% (). The tactic offers simple operation, moderate reaction conditions, broad substrate range, and is environmentally benign (Citation79).
Kumbar et al. have developed an easy, eco-friendly, highly competent, and neat reaction for novel7-aryl-10-thioxo-7,10,11,12-tetrahydro-9H-benzo[H]pyrimido[4,5-b]quinoline-8-one derivatives as antimicrobial agents by the Knoevenagel condensation reaction of thiobarbituric acid, aldehydes derivatives, and 1-naphthylamine followed by Michael addition and subsequent cyclization utilizing ethanol:acetic acid (8:2) catalyst system at 80 0C for 8–9 h to obtain high yields between 85–90% () (Citation80).
Yaragorla et al. have developed a single step regioselective electrophilic-cyclization of 1-(2-aminophenyl) ketones with substituted alkynes to produce 3-iodoquinoline derivatives utilizing potassium tert-butoxide [KOtBu] and water-ethyl acetate (1:1) medium at ambient temperature for 2 h with a yield varying between 73–93% (). The benefit of this method is that it does not need the use of chromatographic methods for purification (Citation81).
Shahabi and Tavakol have described a one-pot three component quinoline synthesis by employing choline chloride/tin(II) chloride [ChCl·2SnCl2] as a deep eutectic solvent [DES] from aniline derivatives, aromatic aldehydes, and enolizable aldehydes at 60°C for 2 h with moderate to excellent yields (68–96%) (). Moreover, this catalyst can be reusable, renewable four times without diminishing substantial catalytic potency (Citation82).
Scheme 44. Synthesis of quinolines utilizing choline chloride/tin(II) chloride [ChCl·2SnCl2] as a deep eutectic solvent.
![Scheme 44. Synthesis of quinolines utilizing choline chloride/tin(II) chloride [ChCl·2SnCl2] as a deep eutectic solvent.](/cms/asset/b88d7e35-0a54-4f7d-95d4-b2b964e4009e/tgcl_a_2064194_f0048_ob.jpg)
Esfandiary et al. have developed a highly potent, one-pot reaction for substituted quinoline synthesis by reacting 2-bromobenzoic acid, piperidine, and phenylacetylene derivatives using γ-Fe2O3@Cu-LDH@Cysteine-Pd as a nano-catalyst and choline azide as a green solvent at 80 °C for 4 h, to obtain moderate to high yields (76–93%) () (Citation83).
3.3. Solvent-free reaction for quinolines synthesis.
The importance of solvent systems in conventional synthetic protocols is well defined and critical for driving a reaction. However, the most commonly used solvents such as methylene chloride (DCM), chloroform, acetonitrile, dimethylformamide (DMF), N,N-dimethylsulfoxide (DMSO), and toluene are hazardous and toxic too. Chemical synthesis without a reaction medium (solvents) is an imaginary thought because the solvent is indispensable in a reaction system. Several neat protocols for synthesizing quinolines with or without catalyst are discussed below (refer ).
Table 3. Outline for solvent-free quinolines synthesis. (Citation84–102).
Sarode et al. have reported solvent-free, three-component coupling reactions for 2,4-disubstituted quinoline synthesis using alkynes, amine derivatives, and substituted aldehydes at 100°C for 5 h in the presence of Zinc(II) triflate [ZnO(Tf)2] as a catalyst, resulting in yields of 75–84% (). The tactic of these features is: eliminate the use of valuable metals, caustic solvents, and intense reaction conditions (Citation84).
Scheme 46. One-pot solvent-free approach for substituted quinoline synthesis using ZnO(Tf)2 as a catalyst.
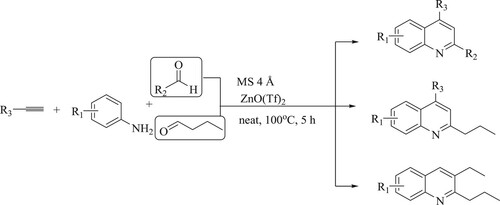
Smuszkiewicz et al. have described bifunctional and multifunctional mesoporous MCF silicates that catalyze the Friedlander reaction of 2-amino aryl aldehydes and ethyl acetoacetate at 49.5 °C for 160 min to synthesize corresponding quinoline derivatives to obtain high yields () (Citation85).
Scheme 47. Solvent-free synthesis of quinoline derivatives using mesoporous MCF silicates as a catalyst.

Jin et al. have developed a continuous Skraup synthesis for mono- and bis-quinolines by reacting a ketal with substituted anilines using niobium phosphate[NbP]as a catalyst at 250°C under 10 MPa pressure to obtain a moderate yield of 60% () (Citation86).
Scheme 48. NbP catalyzed Skraup synthesis for the quinoline derivatives under solvent-free conditions.

Singh and Yaragorla have reported highly potent and one-pot regioselective Friedlander synthesis of 2-methyl-3-acyl quinolines by the reaction of aminobenzophenone derivatives, β-ketoesters, chalcones, or malononitrile via in situ chemoselective Csp3–H functionalization using calcium triflate [Ca(OTf)2] as a catalyst in solvent-free conditions at 110 °C for 5–10 h, resulting in medium to high yields (45–98%) () (Citation87).
Li et al. have developed a one-pot synthesis of 3-aryl quinolines by reacting o-nitrobenzaldehyde derivatives with β-nitrostyrene using glacial acetic acid under conventional heating (100 °C) for 30 min, resulting in a good yield varying between 72–95% (). Moreover, iron is used as a mediator to initiate the cyclization by reducing o-nitrobenzaldehyde and lowering the hydrolysis of nitrostyrene (Citation88).
Ahmed et al. have discovered a technique for quinolines synthesis using bronsted acid-catalyzed cyclization reactions of N-alkyl anilines with alkynes or alkenes catalyzed by triflic acid [TfOH] in the presence of oxygen gas as an oxidant in metal-free and solvent-free conditions at 120 °C for 24 h to obtain a high yield of 83% with metals. In contrast, a moderate yield was observed with different solvents such as DCE, toluene, 1,4-dioxane, or DMF (40–57%) () (Citation89).
Koley et al. have reported easy, effective, solvent-free one-pot synthesis of 2-mercapto quinolines (11–77% yield) using highly functionalized indium chloride [InCl3]as a catalyst by reacting o-amino aryl ketones with α-enolic thioesters derivatives at 80°C for 2–3 h () (Citation90).
Zare et al. have developed a one-pot multi-component synthesis of pyrimido[4,5-b]quinolines by reaction of 6-amino-1,3-dimethyluracil with 4-chloroaldehyde anddimedone using nano-[Fe3O4@-SiO2@R-NHMe2][H2PO4] as organic–inorganic hybrid nano-magnetic catalyst under the solvent-free conditions at 120 °C to obtain yields between 79–96%. The tactic of these features includes short reaction time simple set-up () (Citation91).
Zahra et al. have synthesized thiazole fused quinolines through a four-component reaction of α-enolized thioesters, cysteamine, aromatic aldehydes, and dimedone catalyzed by superparamagnetic silica-encapsulated γ-Fe2O3 supported L-Leucinenanoparticles in solvent-free conditions at 80°C for 1 h, resulting in excellent yields (83–93%) (). Moreover, the catalyst can be recoverable and reusable at least five times without intense loss of catalytic activity (Citation92).
G.M Ziarani et al. have discovered a one-pot four-component reaction of different aromatic aldehydes, Meldrum’s acid, dimedone, and ammonium acetate in the presence of silica-based sulfonic acid [SiO2–Pr–SO3H] as a heterogeneous catalyst at 140 °C for 10–150 min under the solvent-free conditions to form 2,5-dioxo-1,2,3,4,5,6,7,8-tetrahydroquinoline derivatives with good to excellent yields (35–96%) (). The tactic of these features includes operational simplicity, simple procedure, without an organic solvent, and a short reaction time (25 min) (Citation93).
Vinayaka et al. have developed a transition metal-free synthetic technique for 1,2-disubstituted 4-quinoline-2-one synthesis through the regiospecific synthesis of different types of enaminones using an alumina-supported anhydrous potassium carbonate [K2CO3] solid-phase reaction, leading to moderate yields (48–83%) (). The following are the implications of this method: transition metal-free reaction is less risky, requires no solvent (solid phase), and is simple to implement (Citation94).
Sanap and Shankarling have reported a greener synthesis of 4-aminoquinoline-2-ones by intramolecular cyclization of 2-cyano phenyl amide derivatives utilizing biodegradable and reusable green catalyst choline hydroxide [ChOH] for 15–20 min, resulting in moderate yields (69–83%) (). The tactic behind these features includes easy work-up and environmentally benign methodology (Citation95).
Scheme 57. ChOH catalyzed reaction to synthesized quinoline derivatives under solvent-free conditions.
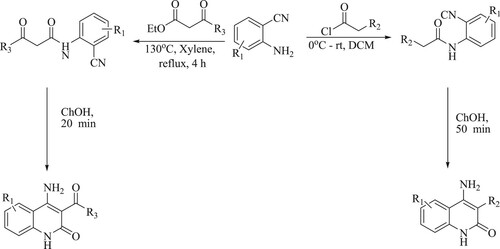
Kumar et al. have synthesized 2-steryl quinolones via Friedlander annulation-Knoevenagel condensation of 2-aminobenzophenone derivatives, ethyl acetoacetate, and benzaldehyde catalyzed by indium triflate [In(OTf)3] as a recoverable and reusable catalyst in solvent-free conditions at 100°C for 5 h resulting in good yields (70–84%) () (Citation96).
Anand et al. have discovered metal-free, simple operation, 100% carbon economy, highly potent one-pot aerobic process for the synthesis of functionalized quinolines from 2-aminobenzyl alcohol/2-aminobenzophenone and alkyl/aryl alcohols using potassium hydroxide [KOH] as a base at 80°C for 5–7 h, resulting in moderate to high yields (62–95%) () (Citation97).
Bandyopadhyay et al. have synthesized poly-substituted quinolines via Friedlander hetero-annulation of 2-aminobenzophenone with normal ketones or β-keto esters using titanium nano-particle [TiO2NP] under solvent-free conditions at 80 0C for 12–45 min to achieve maximum yields of 78–94%. The tactic of the following features includes solvent-free reaction, higher selectivity, affordable, neat reaction profiles, and an easy work-up () (Citation98).
Avula et al. have reported one-pot, two-component cascade synthesis of N,4-diaryl-2,3-dihydro pyrrolo[3,4-c]quinoline-1,3-diones and their 3-thioxo-analogs in clean conditions catalyzed by 4-N,N-dimethylaminopyridine [DMAP] at 120°C for 1.5 h to obtain excellent yields of 72–90% (). Furthermore, this technique eliminates the use of expensive catalysts, hazardous chemicals, solvents, column chromatography purification, and challenging reaction conditions (Citation99).
L. Yang et al. have developed high efficient three-component reaction of ethyl/methyl lactate, anilines, and aldehydes catalyzed by simple iron(III) chloride [FeCl3] without utilizing additional organic medium at 110 °C to form 2,4-disubstituted quinolines with good yields() (Citation100).
Hui et al. have developed in situ reduction and cyclization reaction for the synthesis of pyrazolo[4,3-f]quinoline, pyrazolo[3,4-f]quinoline and pyrazolo[3,2-f]quinoline derivatives by the reaction of 5-nitroindazole, 6-nitroindazole and 5-nitroindole by utilizing tin chloride [SnCl2·2H2O] at 80 °C for 5–10 h with obtained yields varying between 81–92% () (Citation101).
Xiao et al. have developed a one-pot three-component coupling reaction of aromatic amine, aldehyde, and alkyne to synthesize 2,4 disubstituted quinolone derivatives catalyzed by MOF-5 at 110 °C for 3 h to obtain excellent yields of 87–91% () (Citation102). Moreover, this protocol has easy recovery and reusability of catalyst, broad substrate scopes, and cost-effective.
In addition to that, quinoline was also developed through a catalyst-free approach by Patil et al. via a one-pot four-component green synthesis of hexahydroquinoline through enaminone intermediate by the reaction of dimedone, ammonium acetate (acting as reagent as well as a catalyst), aryl aldehydes, and malononitrile in a green solvent like water for 1–1.5 h without using an external catalyst, resulting in yields varying between 57–85% (). The tactic has features like rapid reaction, excellent atom economy, quick set-up, and purification of products by the non-chromatographic method (Citation103).
3.4. Biocatalyst and photo-catalyst based green approach for quinolines synthesis.
Green chemistry's primary goals are to improve process selectivity, maximize the use of starting materials, and replace toxic and stoichiometric reagents with environmentally friendly catalysts to make it easier to separate final reaction blends with catalyst recovery. Biodegradable, non toxic by-products from numerous industries utilized green catalysts in diverse processes. For example, enzymes are biological catalysts that lower the activation energy of a reaction when used in organic synthesis, allowing it to proceed at room temperature or moderate temperatures. The biocatalysts are substrate-specific and environmentally beneficial, as well as biodegradable (refer ).
Table 4. Outline for synthesizing quinoline derivatives using Bio and Photo-catalysts(Citation104–109).
Tufail et al. have developed a novel Friedlander method for synthesis of several multisubstituted quinolines using organo-promotor malic acid by the reaction of substituted 2-amino benzophenone or o-acyl anilines and carbonyl compounds with an active methylene group at 55–80 0C for 3.5–7 h, resulting ingoodyields (72–95%) (). The tactical's features include broad substrate scope, solvent-free reaction at room temperature, fast reaction, easily operated, affordable, high atom economy, excellent yield (Citation104).
Godino-Ojer et al. have reported the reusable and solvent-free synthesis of quinoline by the reaction of 2-amino-5-chlorobenzaldehyde derivatives and ethyl acetoacetate in the presence of metal nanoparticles of cobalt Co(0) and copper Cu(0) doped aerogels catalyst via Friedlander reaction, resulting in yields of 90–97% in 2 h under mild reaction conditions (50 °C) () (Citation105).
Fei et al. have developed a novel, efficient one-pot copper-catalyzed sequential approach for the synthesis of functionalized quinolines through substituted enamino esters and ortho-halogen aromatic carbonyl derivatives catalyzed by Copper iodide [CuI] and L-proline at 120 °C for 18 h to obtain yields varying between 45–86% () (Citation106).
Scheme 68. Copper and L-proline catalyzed tandem reactions for the synthesis of functionalized quinolines.

Dekamin et al. have synthesized poly-hydroquinolines via four-component Hantzsch synthesis of substituted ethyl acetoacetate, aryl aldehydes, ammonium acetate,1,3-cyclohexanediones, or dimedone in ethanol for 10–40 min under reflux conditions to obtain good to excellent yields (75–97%) utilizing alginic acid catalyst (). Moreover, the catalyst is highly active, affordable, commercially accessible, and recyclable for at least six consecutive cycles without losing its catalytic activity (Citation107).
Zohu et al. have developed 10-methyl-9,10-dihydroacridine [AcrH2] as a photo-redox catalyst for developing disubstituted quinolines and tetrahydroquinolines from styrenes or phenyl acetylenes with numerous diazonium salts in acetonitrile utilizing ultraviolet light at ambient temperature with yields of 54–93% (). Moreover, sodium borohydride was also employed to minimize and consolidate the imine product formed (Citation108).
Gangu et al. have described affordable, eco-friendly Knovenagel condensation synthesis by four-component organic reaction of aromatic aldehyde, benzyl-3-oxobutanoate, 1,3-cyclohexanedione, and ammonium acetate with the help of a three-dimensional coordination complex catalyst [Cd(2,5-Pydc)2(H2O)2].H2O or [Sm(2,5-Pydc)(NO3)(H2O)] for the formation of quinoline-3-carboxylate utilizing ethanol as a solvent for 15–20 min, resulting in excellent yields of 92–96% at ambient temperature () (Citation109).
3.5. Nano-catalyst based green approach for quinolines synthesis.
Nanoparticles have gained much attention from researchers because of their high catalytic activity, reusability, and benign nature in green chemistry. Below are several promising nanocatalyst-based approaches for quinolines synthesis (refer ).
Table 5. Outline for the synthesis of quinoline derivatives using nano-catalysts(Citation110–117).
J. Safari et al. have developed an easy, rapid, and eco-friendly method for the synthesis of pyrimido[4,5-b]quinoline-tetraones using effective, robust, recyclable magnetic nano-composites containing 12-phospho-tungstic acid-functionalized chitosan@Nickel-Cobaltite Nanoparticles[PWA/CS/NiCo2O4] by reacting aromatic aldehydes, 6-aminouracil or 6-amino-1,3-dimethyluracil with 2-hydroxy-1,4-naphthoquinone in reflux conditions for 60–90 min to obtain excellent yields (85–96%) (). In addition, this tactic requires simple purification without the need for column chromatography (Citation110).
Scheme 72. Chitosan@NiCo2O4 NPs as a novel magnetic nano-composite for the synthesis of quinoline derivatives.
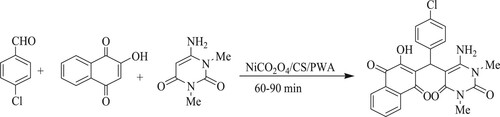
Xie et al. have synthesized functionalized quinolines by reductive annulation of 2-nitro aryl carbonyls with alkynoates and alkynones using newly nitrogen-doped Zirconium Dioxide [ZrO2@C] supported cobalt [Co/ N-ZrO2@C] nano-catalyst in the presence of formic acid at 110 °C for 18 h to produce 83% yield(). This protocol has the following advantages: a broad substrate range, strong functional compatibility, excellent transfer hydrogenation selectivity, repeatable earth-abundant metal catalyst, and easy operation (Citation111).
Angajala et al. have developed an efficient nano copper[CuNPs] catalyst for the Knoevenagel condensation, the reaction between 3,3-dimethyl-9-phenyl-3,4-dihydroacridine-1(2H) and 2-chloroquinoline-3-carbaldehydes for 2 h, resulting in the formation of acridino-quinoline derivatives with the yield of 90% (). Moreover, this method provides quicker reaction time, a straightforward work-up operation, neat reaction profiles, and catalyst recyclability for up to five cycles (Citation112).
Esmaeilpour and Javidi have developed poly-substituted quinoline derivatives via one-pot synthesis by using 2-aminobenzophenone and ethyl acetoacetate or ketones catalyzed by Fe3O4@SiO2-imid-PMAn and Fe3O4@SiO2-imid-PMAb nano-particles under solvent-free conditions at 70 °C for 35–70 min, leading to excellent yields of 90–95% (). Moreover, the nano-catalysts can be recycled and reused at least four times before losing catalytic activity (Citation113).
Chaurasia et al. have reported a simple, environmentally benign, and sustainable method for the synthesis of substituted quinolines by double dehydrogenation cyclization reaction of 2-aminobenzyl alcohol with 1-phenylethyl alcohols using nano Copper Nickel Iron(II) oxide[CuNiFeO]reusable catalyst under the optimized reaction conditions such as 130 °C and 24 h reaction time to obtain 83% yield () (Citation114).
Gajare et al. have developed graphene-oxide supported bronsted acidic ionic liquid [GrBenzImi]SO3H assisted synthetic approach for the production of 2,4-disubstituted quinolines by coupling reactions of aryl aldehydes, anilines with phenylacetylene using a ratio of water and ethanol system (1:1) as a green medium at 80 °C () (Citation115).
Scheme 77. Graphene-oxide supported ionic liquid catalyst-based approach for the synthesis of quinolines.
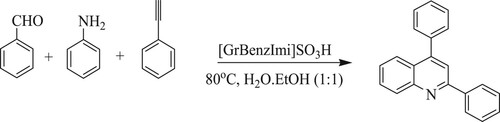
S.Elavarasann et al. have developed a one-pot sequential multi-step oxidative dehydrogenative coupling of 2-aminobenzylalcohol with aromatic ketones in the presence of efficient copper mesoporous organic nano-rod [Cu-HMOP] recyclable catalyst and t-BuOK as a base in a non-polar solvent like toluene at 130°C for 24 h, resulting in a yield of 96% without any trace of side product() (Citation116).
Chakraborty et al. have carried out a straightforward synthesis of quinolines, 2-aminoquinolines via dehydrogenative coupling reactions of 2-aminobenzyl alcohol with 2-phenylacetonitrile or acetophenone at 80–95 °C conditions using singlet diradicalNickel[Ni(II)] catalyst and afforded 85% and 65% yield, respectively (). Moreover, the catalyst used in this synthesis is inexpensive (Citation117).
Apart from nano-catalysts, Reddy et al. have discovered an effective, easy, fast reaction strategy towards the access of pyrimido[4, 5-b]quinoline-diones by the condensation of aldehydes, anilines, and barbituric acid mediated by a supramolecular green catalyzed like β-cyclodextrin in an aqueous medium at 80 °C for 4–5 h reaction time () (Citation118).
3.6. Miscellaneous green synthetic approaches for quinolines synthesis.
Many other green approaches for synthesizing quinoline have been reported, such as mechanochemistry, which deals with the chemical behavior of mechanically stressed solids and eliminates the use of solvents. Moreover, the ultrasound-assisted method also enhances the rate of the chemical reactions for quinoline and its derivatives (refer ).
Table 6. Miscellaneous approaches for synthesizing quinoline derivatives (Citation119–134).
Szlachcic et al. have developed solvent-free, simple synthesis of 1H-pyrazolo[3,4-b]quinolines through a regioselective condensation reaction of 2-fluorobenzaldehydes and 1H-pyrazole-5-amines in the presence of a base catalyst like 1,4-diazabicyclo[2.2.2]octane [DABCO] at 180 °C for 60 min, which results in yield values of 25–60% () (Citation119).
Thigullaet al. have developed one-pot synthesis of fused chromeno[4,3-b]pyrrolo[3,2-h]quinolin-7(1H)-one compound by the reaction of 4-hydroxy coumarin, 7-amino indole, and diverse aromatic aldehydes in the presence of FeCl3 as a catalyst and 1,4-dioxane as a polar aprotic solvent at 90 °C for 12 h with yields of 27–51% () (Citation120).
Wu et al. have discovered a one-pot synthesis of regioselective copper(II)-catalyzed annulation of anilines with two molecules of alkyne esters to form substituted quinolines using copper triflate [Cu(OTf)2] as a catalyst and triflic acid [HOTf] as an add-on system in acetonitrile at 120°C for 24 h to obtain 56–98% yields () (Citation121).
Scheme 83. Synthesis of substituted quinolines using triflic acid and copper triflate as a catalyst.
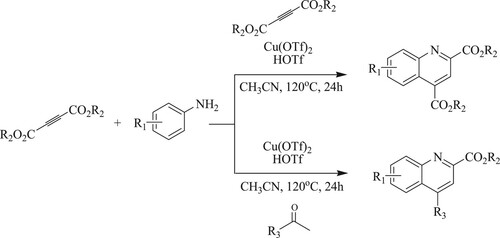
Long-Yi et al. have described an effective approach to synthesize substituted quinolines through C–N cleavage of amines followed by condensation with ketones and 2-amino benzylamines in the presence of copper triflate and toluene sulphonic acid [TsOH] utilizing toluene as a non-polar solvent at 100 °C for 36 h, to obtain yields of 43–99% () (Citation122).
Kadam and Tilvehave reported the simple, efficient one-pot synthesis of 6H-Indole[2,3-b]quinolines via sequential alkylation, dehydration followed by cyclization and aromatizationcatalyzed by pivalic acid (non-nucleophilic weak acid) under reflux conditions for 6 h using diphenyl ether as a solvent to obtain yields of 54–57% () (Citation123).
Andrade et al. used niobium pentachloride[NbCl5], a green catalyst as a Lewis acid in multi-component reactions between benzaldehyde, aniline derivatives, and phenylacetylene for the synthesis of quinoline derivatives under reflux for 24 h in acetonitrile to obtain yields varying from 67 to 96% () (Citation124).
Li et al. have developed a copper-catalyzed [CuBr] one-pot synthesis of substituted quinolines by the reactions of 2-bromobenzaldehyde with aryl methyl ketones and aqueous ammonia using cesium carbonate [CsCO3] and 1,10-phenanthroline hydrate ligand at 80 °C for 20 h, resulting in good yields from 81 to 92% (). The protocol has the following features: good commercial availability of starting materials, simple synthetic procedures, and lenient reaction conditions (Citation125).
Gao et al. have developed quinoline-4-carboxamide derivatives using iodine catalyzed conversion of isatin through cleavage of N-O/C–N bond and formation of C–C/C–N in the presence of triethylamine as a base in chlorobenzene, which results in 57–91% yields at 130 °C () (Citation126).
Xu et al. have developed a one-pot synthesis of a novel Co(III) derived [Cp(CO)I2] carbonyldiiodo(pentamethylcyclopentadienyl) cobalt catalyzed C–H activation/carbonylation/cyclization of anilines with ketones in the presence of paraformaldehyde as the carbonyl source under co-catalytic hexafluoroantimonate [AgSbF6] and triflic acid [HOTf] at 120 °C for 8 h to synthesize quinoline derivatives with yields varying from 54–91% (). The tactic of these features includes a waste-free, eco-friendly, and atom-step-economical (Citation127).
Zhang et al. have designed a one-pot synthesis of 2-aminoquinoline-3-carboxamides by the reaction of 2-bromobenzaldehydes, aqueous ammonia, and acetamide derivatives in the presence of copper(II) acetate[Cu(OAc)2] catalyst and potassium carbonate [K2CO3]as a base at 100 °C for 6 h, resulting in moderate yield values of 41–60% (). The main feature of this method is that it is a single catalyst system and atom economic strategy (Citation128).
Lee et al. have reported the one-pot synthesis of substituted 1,4-dihydroquinolines using trimethylsilyl[O-TMS] ether as an organocatalystvia aza-Michael/Michael cascade reaction of 2-(tosyl amino)phenyl α,β-unsaturated ketones with alkynyl aldehydes, and diphenylprolinol for 72–96 h, generating an excellent yield (up to 97 %) () (Citation129).
Sushi Zadeh et al. have synthesized 1,2-dihydro and 1,2,3,4-tetrahydro-quinolines via imino Diels–Alder reaction of N-aryl-substituted aldimines and numerous alkenes using 1,7-sigmatropic rearrangement with the help of efficient catalyst like a marine sponge/oxalic acid at room temperature in the presence of N,N-dimethylformamide (DMF) as a solvent under UV light for 36 h (). The tactic has the following features: lenient reaction conditions, easy experimental procedure, high yields of products(47–80%) (Citation130).
Chen et al. have reported a two-step reaction of ketones and primary alcohols via hydrogen transfer process catalyzed by silver–palladium loaded carbon [AgPd/C] which results in the formation of an α-alkylated ketone. On further reaction with 2-aminobenzyl alcohol at 125°C, it resulted in polysubstituted quinolines with low to moderate yields (15–71%) () (Citation131).
Karad et al. have designed a one-pot, three-component reaction of 3-methyl-5-substituted aryloxy-1-phenyl-pyrazole-4-carbaldehyde with malononitrile and different enhydrazinoketones for 15–30 min under ultrasound irradiation to synthesize biologically active fluorinated 5-aryloxy pyrazole-quinolines with good yields (76–91%)(). The tactic's features include short reaction time under ultrasonic waves, delivering functionalized molecules of biological importance (Citation132).
Ahmed et al. have developed a one-pot three component, solvent-free grinding method for green synthesis of 5-(4-methoxyphenyl)−7,7-dimethyl-10-phenyl-7,8-dihydro-5H-indeno[1,2-b]quinoline-9,11(6H,10H)-dione derivatives by the reaction of 1,3-indanedione, enaminone, and aryl-aldehyde using p-toluene sulfonic acid [p-TSA] as a catalyst for 15–30 min, resulting in good yields (70–86%) at room temperature () (Citation133).
Ma et al. have developed an efficient and green method for the synthesis of quinolone derivatives via the Friedlander heteroannulation reaction of substituted 2-aminoaryl ketones and α-methylene ketones using L-(+)-tartaric acid-DMU (30:70) as a catalytical reaction medium at 70 °C to obtain yields varying between 70–95% () (Citation134).
4. Conclusion
Quinoline and its derivatives possess a wide range of pharmacological activities and are also utilized as ligands in numerous biologically-modelled transition metal complexes. In this review, we have compiled and discussed all commonly used green methods, such as ultrasound-assisted, microwave-irradiation, heterogeneous acid-catalyzed methods, photo-catalyzed, solvent-free conditions with a critical presentation of data in a tabulated form so that the researcher can create a new environmentally sustainable, efficient, and cost-effective technique.
In addition, numerous quinoline derivatives play a significant role in the progress of organic synthesis and applications to medicinal chemistry. However, in the creation of novel techniques, it was possible to find that using Friedlander or multicomponent reactions (MCR) results in increased atom economy and using solvent-free conditions, ionic liquids assisted, and ultrasound irradiation synthetic methodologies meet the requirements of ‘green chemistry.’
In a nutshell, this type of recent review is necessary from today's point of view as we need an environmentally clean protocol for the large-scale production of such an essential biological moiety (Citation135, Citation136), which may be used further in many reactions to develop a potent pharmacophore for the future.
Acknowledgment
Declared none.
Disclosure statement
No potential conflict of interest was reported by the author(s).
References
- Zhang, X.; Shetty, A.S.; Jenekhe, S.A. Macromolecules 1999, 32, 7422–7429.
- Lam, P.L.; Kan, C.W.; Yuen, M.C.W.; Cheung, S.Y.; Gambari, R.; Lam, K.H.; Tang, C.O.; Chui, C.H. Color. Technol. 2012, 128, 192–198.
- Nolan, E.M.; Jaworski, J.; Okamoto, K.-I.; Hayashi, Y.; Sheng, M.; Lippard, S.; Lippard, J. J. Am. Chem. Soc. 2005, 127, 16812–16823.
- Rose, M.J.; Fry, N.L.; Marlow, R.; Hinck, L.; Mascharak, P.K. J. Am. Chem. Soc. 2008, 130, 8834–8846.
- Patel, A.; Shah, D.; Patel, N.; Patel, K.; Soni, N.; Nagani, A.; Parikh, V.; Shah, H.; Bambharoliya, T. Mini-Reviews in Organic Chemistry 2021, 18, 1–22.
- Marella, A.; Tanwar, O.P.; Saha, R.; Ali, M.R.; Srivastava, S.; Akhter, M.; Shaquiquzzaman, M.; Alam, M.M. Saudi. Pharm. J. 2013, 21, 1–12.
- Dobbin, L. J. Chem. Educ. 1934, 11, 596.
- Collin, G.; Hoke, H. Quinoline and Isoquinoline. In Ullmann’s Encyclopedia of Industrial Chemistry; 1–4, Wiley-VCH: Weinheim, 2000.
- Lawrence, S. Cambridge University Press: UK, 2004. 162–163.
- Boyd, D.R.; Sharma, N.D.; Loke, P.L.; Malone, J.F.; McRoberts, W.C.; Hamilton, J.T.G. Organicand Biomolecular Chemistry 2007, 52, 983–991.
- Cretton, S.; Breant, L.; Pourrez, L.; Ambuehl, C.; Marcourt, L.; Ebrahimi, S.N.; Hamburger, M.; Perozzo, R.; Karimou, S.; Kaiser, M.; Cuendet, M.; Christen, P. Journal of Natural Product 2014, 77, 2304–2311.
- Miller, L.M.; Mayer, S.C.; Berger, D.M.; Boschelli, D.H.; Boschelli, F.; Di, L.; Du, X.; Dutia, M.; Floyd, M.B.; Johnson, M.; Kenny, C.H.; Krishnamurthy, G.; Moy, F.; Petusky, S.; Tkach, D.; Torres, N.; Wu, B.; Xu, W. Bioorganic and Medicinal ChemistryLetters 2009, 19, 62–66.
- Raynes, K.; Foley, M.; Tilley, L.; Deady, L.; Deady, W. Biochem. Pharmacol. 1996, 52, 551–559.
- Travins, J.M.; Ali, F.; Huang, H.; Ballentine, S.K.; Khalil, E.; Hufnagel, H.R.; Pan, W.; Gushue, J.; Leonard, K.; Bone, R.F.; Soll, R.M.; DesJarlais, R.L.; Crysler, C.S.; Ninan, N.; Kirkpatrick, J.; Cummings, M.D.; Huebert, N.; Molloy, C.J.; Gaul, M.; Tomczuk, B.E.; Subasinghe, N.L. Bioorg. Med. Chem. Lett. 2008, 18, 1603–1606.
- Abadi, A.H.; Hegazy, G.H.; El-Zaher, A.A. Bioorg. Med. Chem. 2005, 13, 5759–5765.
- Eswaran, S.; Adhikari, A.V.; Chowdhury, I.H.; Pal, N.K.; Thomas, K.; Thomas, D. Eur. J. Med. Chem. 2010, 45, 3374–3383.
- Franck, X.; Fournet, A.; Prina, E.; Mahieux, R.; Hocquemiller, R.; Figadere, B. Bioorg. Med. Chem. Lett. 2004, 14, 3635–3638.
- Edmont, D.; Rocher, R.; Plisson, C.; Chenault, J. Bioorg. Med. Chem. Lett. 2000, 10, 1831–1834.
- Gilbert, A.M.; Bursavich, M.G.; Lombardi, S.; Georgiadis, K.E.; Reifenberg, E.; Flannery, C.R.; Morris, E.A. Bioorg. Med. Chem. Lett. 2008, 18, 6454–6457.
- Kumar, S.; Bawa, S.; Drabu, S.; Panda, B.P. Med. Chem. Res. 2010, 20, 1340–1348.
- Cai, Z.; Zhou, W.; Sun, L. Bioorganicand Medicinal Chemistry 2007, 15, 7809–7829.
- Fakhfakh, M.A.; Fournet, A.; Prina, E.; Mouscadet, J.-F.; Franck, X.; Hocquemiller, R.; Figadere, B. Bioorg. Med. Chem. 2003, 11, 5013–5023.
- Rossiter, S.; Peron, J.M.; Whitfield, P.J.; Jones, K. Bioorganicand Medicinal Chemistry Letters 2005, 15, 4806–4808.
- Bawa, S.; Kumar, S.; Drabu, S.; Kumar, R. Journal of Pharmacy and Bioallied Sciences 2010, 2, 64–71.
- M, O.; S, D.; H, B.; N, D.; A, D.; Sa, K. Turk. J. Chem. 2012, 36, 233–246.
- Graves, P.R.; Kwiek, J.J.; Fadden, P.; Ray, R.; Hardeman, K.; Coley, A.M.; Foley, M.; Haystead, T.A.J. MolecularPharmacology 2002, 62, 1364–1372.
- Vu, A.T.; Cohn, S.T.; Manas, E.S.; Harris, H.A.; Mewshaw, R.E. Bioorganicand Medicinal Chemistry Letters 2005, 15, 4520–4525.
- Li, Y.; Shi, X.; Xie, N.; Zhao, Y.; Li, S. Med Chem Comm 2013, 4, 367–370.
- Skraup, Z.H. Monatsh. Chem 1881, 2, 139–170.
- Matsugi, M.; Tabusa, F.; Minamikawa, J. Tetrahedron Lett. 2000, 41, 8523–8525.
- Schmittel, M.; Ammon, H. Eur. J. Org. Chem. 1998, 5, 785–792.
- Bergstrom, F.W. Chem. Rev. 1944, 35, 77–277.
- Cheng, C.; Yan, S. Org. React. 2004, 28, 37–201.
- Jones, G. In the chemistry of Heterocyclic compounds; Weissberger, A.; Taylor, E. C., Eds.; John Wiley and Sons: Chichester, 1977, pp 93–318.
- Gogoi, S.; Shekarrao, K.; Duarah, A.; Bora, T.C.; Gogoi, S.; Boruah, R.C. Steroids 2012, 77, 1438–1445.
- Nainwal, L.M.; Tasneem, S.; Akhtar, W.; Verma, G.; Khan, M.F.; Parvez, S.; Shaquiquzzaman, M.; Akhter, M.; Alam, M.M. Eur. J. Med. Chem. 2019, 164, 121–170.
- Tanaka, K.; Toda, F. Chemical Review 2000, 100, 1025–1074.
- Shaikh, I.R. Journal of Catalysts 2014. e402860.
- Saggadi, H.; Luart, D.; Thiebault, N.; Polaert, I.; Estel, L.; Len, C. Catal. Commun. 2014, 44, 15–18.
- Yu, Y.; Tu, M.S.; Jiang, B.; Wang, S.L.; Tu, S.J. Tetrahedron Lett. 2012, 53, 5071–5075.
- Li, M.Y.; Xu, H.W.; Fan, W.; Ye, Q.; Wang, X.; Jiang, B.; Wang, S.L.; Tu, S.J. Tetrahedron 2014, 70, 1004–1010.
- Saggadi, H.; Luart, D.; Thiebault, N.; Polaert, I.; Estel, L.; Len, C.L. RSC Advance 2014, 4, 21456–21464.
- Chidurala, P.; Jetti, V.; Pagadala, R.; Meshram, J.S.; Jonnalagadda, S.B. J. Heterocycl. Chem. 2015, 52, 1302–1307.
- Zhang, X.; Dhawan, G.; Muthengi, A.; Liu, S.; Wang, W.; Legris, M.; Zhang, W. Green Chem. 2017, 19, 3851–3855.
- Fedoseev, P.; Eycken, E.V.d. Chemical Communications Journal 2017, 53, 7732–7735.
- Ma, N.; Lu, H.; Wu, F.; Zhang, G.; Jiang, B.; Shi, F.; Gao, Y.; Tu, S. J. Heterocycl. Chem. 2011, 48, 803–807.
- Malvacio, I.; Moyano, E.L.; Vera, D.M.A.V. RSC Adv. 2016, 6, 83973–83981.
- Liberto, N.A.; Simoes, J.B.; de Paiva Silva, S.; da Silva, C.J.; Modolo, L.V.; de Fatima, A.; Silva, L.M.; Derita, M.; Zacchino, S.; Zuniga, O.M.P.; Romanelli, G.P.; Fernandes, S.; Fernandes, A. Bioorg. Med. Chem. 2017, 25, 1153–1162.
- Godino-Ojer, M.; Soriano, E.; Calvino-Casilda, V.; Maldonado-Hodar, F.J.; Perez-Mayoral, E. Chem. Eng. J. 2017, 314, 488–497.
- Garella, D.; Barge, A.; Upadhyaya, D.; Rodríguez, Z.; Palmisano, G.; Cravotto, G. Synth. Commun. 2009, 40, 120–128.
- Anvar, S.; Baltork, I.M.; Tangestaninejad, S.; Moghadam, M.; Mirkhani, V.; Khosropour, A.R.; Kia, R. RSC Adv. 2012, 2, 8713–8720.
- Jiang, B.; Liang, Y.B.; Kong, L.F.; Tu, X.J.; Hao, W.-J.; Ye, Q.; Tu, S.J. RSC Adv. 2014, 4, 54480–54486.
- Kulkarni, A.; Torok, B. Green Chem. 2010, 12, 875–878.
- Cho, S.K.; Song, J.H.; Lee, E.J.; Lee, D.-H.; Hahn, J.-T.; Jung, D.-I. Bull. Korean Chem. Soc. 2015, 36, 2746–2749.
- Peng, J.H.; Jia, R.H.; Ma, N.; Zhang, G.; Wu, F.Y.; Cheng, C.; Tu, S.J. J. Heterocycl. Chem. 2013, 50, 899–902.
- Albert-Soriano, M.; Trillo, P.; Soler, T.; Pastor, I.M. Eur. J. Org. Chem. 2017, 43, 6375–6381.
- Li, X.Y.; Liu, Y.; Chen, X.L.; Lu, X.Y.; Liang, X.X.; Zhu, S.S.; Wei, C.W.; Qu, L.B.; Yu, B. Green Chem. 2020, 22, 4445–4449.
- Ramesh, K.B.; Omkaramurthy, B.M.; Srinivas, M. J. Mol. Struct. 2020, 1222, 128790.
- Meena, K.; Kumari, S.; Khurana, J.M.; Malik, A.; Sharma, C.; Panwar, H. Chin. Chem. Lett. 2017, 28, 136–142.
- Teimouri, A.; Chermahini, A.N. Arabian Journal of Chemistry 2016, 9, S433–S439.
- Mansoor, S.S.; Aswin, K.; Logaiya, K.; Sudhan, S.P.N. Arabian Journal of Chemistry 2017, 10, S546–S553.
- Karnakar, K.; Narayana Murthy, S.; Ramesh, K.; Satish, G.; Nanubolu, J.B.; Nageswar, Y.V.D. Tetrahedron Lett. 2012, 53, 2897–2903.
- Pasha, J.; Kandagatla, B.; Sen, S.; Seerapu, G.P.K.; Bujji, S.; Haldar, D.; Nanduri, S.; Oruganti, S. Tetrahedron Lett. 2015, 56, 2289–2292.
- Abd El Aleem Ali Ali El-Remaily, M.; El-Dabea, T.; Alsawat, Hamad, H.A. J. Mol. Catal. A: Chem. 2015, 404–405, 148–155.
- Ghosh, J.; Biswas, P.; Sarkar, T.; Drew, M.G.B.; Bandyopadhyay, C. Tetrahedron Lett. 2014, 55, 2924–2928.
- Verma, G.K.; Raghuvanshi, K.; Kumar, R.; Singh, M.S. Tetrahedron Lett. 2012, 53, 399–402.
- Abdolmohammadi, S. Chin. Chem. Lett. 2013, 24, 318–320.
- Nemati, F.; Saeedirad, R. Chin. Chem. Lett. 2013, 24, 370–372.
- Arenas, D.R.M.; Bonilla, C.A.M.; Kouznetsov, V.V. Organic and Biomolecular Chemistry 2013, 11, 3655–3663.
- Anand, N.; Chanda, T.; Koley, S.; Chowdhury, S.; Singh, M.S. RSC Adv. 2015, 5, 7654–7660.
- Mohammadi, K.; Shirini, F.; Yahyazadeh, A. RSC Adv. 2015, 5, 23586–23590.
- Paplal, B.; Nagaraju, S.; Veerabhadraiah, P.; Sujatha, K.; Kanvah, S.; Kumar, B.V.; Kashinath, D. RSC Adv. 2014, 4, 54168–54174.
- Siddiqui, I.R.; Rai, P.; Rahila; Sagir, H.; Singh, P. RSC Adv. 2015, 5, 27603–27609.
- Sudhapriya, N.; Nandakumar, A.; Perumal, P.T. RSC Adv. 2014, 4, 58476–58480.
- Abdelhamid, A.A.; Allah, O.A.A.; Tamam, A.H.A. J. Heterocycl. Chem. 2017, 54, 2822–2829.
- Mou, R.Q.; Zhao, M.; Lv, X.X.; Zhang, S.Y.; Guo, D.S. RSC Adv. 2018, 8, 9555–9563.
- Luo, Y.; Sun, H.; Zhang, W.; Wang, X.; Xu, S.; Zhang, G.; Jian, Y.; Gao, Z. RSC Adv. 2017, 7, 28616–28625.
- Khan, M.M.; Saigal; Khan, S.; Shareef, S.; Hussain, S. Chemistry Select 2018, 3, 2261–2266.
- Zhu, G.; Gao, L.; Yu, Q.; Qin, Y.; Xi, J.; Rong, L. J. Heterocycl. Chem. 2018, 55, 871–878.
- Kumbhar, D.; Chandam, D.; Patil, R.; Jadhav, S.; Patil, D.; Patravale, A.; Deshmukh, M. J. Heterocycl. Chem. 2018, 55, 692–698.
- Yaragorla, S.; Pareek, A.; Dada, R.; Saini, P.L. Eur. J. Org. Chem. 2017, 2017, 4600–4608.
- Shahabi, D.; Tavakol, H. J. Mol. Liq. 2016, 220, 324–328.
- Naghmeh, E.; Akbar, H. Appl. Organomet. Chem. 2000, 9, e5760.
- Sarode, P.B.; Bahekar, S.P.; Chandak, H.S. Tetrahedron Lett. 2016, 57, 5753–5756.
- Smuszkiewicz, A.; Perez-Mayoral, E.; Soriano, E.; Sobczak, I.; Ziolek, M.; Martín-Aranda, R.M.; Lopez Peinado, A.J. Catal. Today 2013, 218–219, 70–75.
- Jin, J.; Guidi, S.; Abada, Z.; Amara, Z.; Selva, M.; George, M.W.; Poliakoff, M. Green Chem. 2017, 19, 2439–2447.
- Singh, G.; Yaragorla, S. RSC Adv. 2017, 7, 18874–18882.
- Li, D.K.; Cai, Q.; Zhou, R.R.; Wu, Y.D.; Wu, A.-X. ChemistrySelect 2017, 2, 1048–1051.
- Ahmed, W.; Zhang, S.; Yu, X.; Yamamoto, Y.; Bao, M. Green Chem. 2018, 20, 261–265.
- Koley, S.; Tiwari, N.; Neelabh; Singh, R.K.; Singh, M.S. ChemistrySelect 2018, 3, 1688–1692.
- Zare, A.; Lotfifar, N.; Dianat, M. J. Mol. Struct. 2020, 1211, 128030.
- Arabpoor, Z.; Shaterian, H.R. RSC Adv. 2016, 6, 44459–44468.
- Ziarani, G.M.; Asadi, S.; Badiei, A.; Mousavi, S.; Gholamzadeh, P. Res. Chem. Intermed. 2015, 41, 637–645.
- Vinayaka, A.C.; Swaroop, T.R.; Chikkade, P.K.; Rangappa, K.S.; Sadashiva, M.P. RSC Adv. 2016, 6, 11528–11535.
- Kailas Sanap, A.; Subray Shankarling, G. New J. Chem. 2015, 39, 206–212.
- Kumar, D.; Kumar, A.; Qadri, M.M.; Ansari, M.I.; Gautam, A.; Chakraborti, A.K. RSC Adv. 2015, 5, 2920–2927.
- Anand, N.; Koley, S.; Ramulu, B.J.; Singh, M.S. Organic and Biomolecular Chemistry 2015, 13, 9570–9574.
- Bandyopadhyay, P.; Prasad, G.K.; Sathe, M.; Sharma, P.; Kumar, A.; Kaushik, M.P. RSC Adv. 2014, 4, 6638–6645.
- Avula, S.; Koppireddi, S.; Komsani, J.R.; Nanubolu, J.B.; Yadla, R. RSC Adv. 2013, 3, 20990–20998.
- Yang, L.; Wan, J.P. Green Chem. 2020, 22, 3074–3078.
- Xu, H.; Li, L.; Dai, L.; Mao, K.; Kou, W.; Lin, C.; Rong, L. Appl. Organomet. Chem. 2018, 32, e4194.
- Xiao, S.T.; Ma, C.T.; Di, J.Q.; Zhang, Z.H. New J. Chem. 2020, 44, 8614–8620.
- Patil, D.; Chandam, D.; Mulik, A.; Jagdale, S.; Patil, P.; Deshmukh, M. J. Saudi Chem. Soc. 2017, 21, S329–S338.
- Tufail, F.; Saquib, M.; Singh, S.; Tiwari, J.; Singh, M.; Singh, J.; Singh, J. New J. Chem. 2017, 41, 1618–1624.
- Godino-Ojer, M.; Lopez-Peinado, A.J.; Maldonado-Hodar, F.J.; Perez-Mayoral, E. ChemCatChem. 2017, 9, 1422–1428.
- Peng, F.; Liu, J.; Li, L.; Chen, Z. Eur. J. Org. Chem. 2018, 2018, 666–672.
- Dekamin, M.G.; Karimi, Z.; Latifidoost, Z.; Ilkhanizadeh, S.; Daemi, H.; Naimi-Jamal, M.R.; Barikani, M. Int. J. Biol. Macromol. 2018, 108, 1273–1280.
- Zhou, X.-F.; Sun, Y.-Y.; Dai, J.-J.; Xu, J.; Xu, H.-J. Journal of Photochemistry and PhotobiologyA: Chemistry 2018, 355, 186–193.
- Gangu, K.K.; Pothala, V.; Tvspv, S.G.; Maddila, S.; Jonnalagadda, S.B. Inorg. Chem. Commun. 2020, 119, 1–25.
- Safari, J.; Tavakoli, M.; Ghasemzadeh, M.A. Polyhedron 2020, 182, 114459.
- Xie, R.; Lu, G.P.; Jiang, H.F.; Zhang, M. J. Catal. 2020, 383, 239–243.
- Angajala, G.; Aruna, V.; Subashini, R. J. Mol. Struct. 2020, 1220, 128601.
- Esmaeilpour, M.; Javidi, J. J. Chin. Chem. Soc. 2015, 62, 328–334.
- Chaurasia, S.R.; Tiwari, A.R.; Bhanage, B.M. Molecular Catalyst 2019, 478, 110565.
- Gajare, S.; Patil, A.; Hangirgekar, S.; Dhanmane, S.; Rashinkar, G. Res. Chem. Intermed. 2020, 46, 2417–2436.
- Elavarasan, S.; Bhaumik, A.; Sasidharan, M. ChemCatChem. 2019, 11, 4340–4350.
- Chakraborty, G.; Sikari, R.; Das, S.; Mondal, R.; Sinha, S.; Banerjee, S.; Paul, N.D. J. Org. Chem. 2019, 84, 2626–2641.
- Reddy, S.S.; Reddy, M.V.K.; Reddy, P.V.G. ChemistrySelect 2018, 3, 4283–4288.
- Szlachcic, P.; Kucharek, M.; Jarosz, B.; Danel, A.; Stadnicka, K. J. Heterocycl. Chem. 2017, 54, 1729–1745.
- Thigulla, Y.; Kumar, T.U.; Trivedi, P.; Ghosh, B.; Bhattacharya, A. ChemistrySelect 2017, 2, 2718–2721.
- Wu, W.; Guo, Y.; Xu, X.; Zhou, Z.; Zhang, X.; Wu, B.; Yi, W. Org. Chem. Front. 2018, 5, 1713–1718.
- Xi, L.Y.; Zhang, R.Y.; Zhang, L.; Chen, S.Y.; Yu, X.Q. OrganicandBiomolecular Chemistry 2015, 13, 3924–3930.
- Kadam, H.K.; Tilve, S.G. J. Heterocycl. Chem. 2016, 53, 2066–2069.
- Andrade, A.d.; Santos, G.C.d.; Silva-Filho, L.C.d. J. Heterocycl. Chem. 2015, 52, 273–277.
- Li, B.; Guo, C.; Fan, X.; Zhang, J.; Zhang, X. Tetrahedron Lett. 2014, 55, 5944–5948.
- Gao, Q.; Liu, Z.; Wang, Y.; Wu, X.; Zhang, J.; Wu, A. Adv. Synth. Catal. 2018, 360, 1364–1369.
- Xu, X.; Yang, Y.; Chen, X.; Zhang, X.; Yi, W. Organic andBiomolecular Chemistry 2017, 15, 9061–9065.
- Zhang, X.Y.; Guo, X.J.; Fan, X.S. Chemistry- An Asian Journal 2015, 10, 106–111.
- Lee, Y.; Heo, S.; Kim, S.G. Adv. Synth. Catal. 2015, 357, 1545–1550.
- Shushizadeh, M.R.; Mostoufi, A.; Behfar, A.; Heidary, M. Arabian Journal of Chemistry 2015, 8, 868–872.
- Chen, B.W.J.; Chng, L.L.; Yang, J.; Wei, Y.; Yang, J.; Ying, J.Y. ChemCatChem. 2013, 5, 277–283.
- Karad, S.C.; Purohit, V.B.; Raval, D.K.; Kalaria, P.N.; Avalani, J.R.; Thakor, P.; Thakkar, V.R. RSC Adv. 2015, 5, 16000–16009.
- Ahmed, K.; Dubey, B.; Nadeem, S.; Shrivastava, B.; Sharma, P. Chin. Chem. Lett. 2016, 27, 721–725.
- Ma, F.P.; Cheng, G.T.; He, Z.G.; Zhang, Z.H. Aust. J. Chem. 2012, 65, 409–416.
- Patel, A.; Shah, D.; Patel, N.; Patel, K.; Soni, N.; Nagani, A.; Parikh, V.; Shah, H.; Bambharoliya, T. Mini. Rev. Org. Chem. 2021, 18, 1064–1085.
- Patel, A.; Shah, D.; Patel, N.; Patel, K.; Soni, N.; Nagani, A.; Patel, M.; Bhimani, B.; Shah, U.; Bambharoliya, T. Curr. Org. Chem. 2021, 25, 3004–3016.