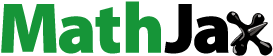
ABSTRACT
Ionic liquids (ILs) begin to be applied in more broad scopes and even in medicine steadily, so it is very necessary to study their biocompatibility, bioactivity, and influence on blood. For the first time, the main effects of popular imidazolium ILs with common anions and alkylated cations on rabbit blood were explored and compared comprehensively. A series of key indices and characteristic methods were applied in this systematic study. The thrombolytic properties in vitro of these ILs and their interactions with two kinds of serum albumins were also studied. This study provides key data and lays foundation for further applications of ILs and risk assessments in related fields.
GRAPHICAL ABSTRACT
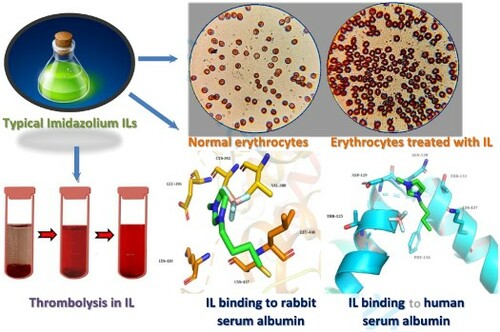
Introduction
Classified as sustainable and green media, ionic liquids (ILs) are the hot topic in current green chemistry during this decade (Citation1, Citation2). With the merits of wide solubility, low vapour pressure, flexible applied forms, etc., ILs are widely used in various fields such as extraction, separation, and catalysis, and even for medical purposes (Citation3–5). Moreover, due to their high designability, various functional ILs can be synthesized according to specific requirements, which also greatly expands their applied scopes (Citation6). With the development of their large-scale application, the exposure chance to this new chemical medium is becoming higher and carries some risks. Especially, the introduction of ILs in biomedicine has attracted great attention from many researchers in recent years, and the studies on their biocompatibility and safety assessment have become an important topic before they are fully accredited in related fields.
Currently, some alkyl imidazolium ILs with common anions were applied as neat solvents, surfactants, or penetration enhancers in drug encapsulation and delivery, functional dressing, and so on (Citation7–9). As penetration enhancers and vehicles, it is completely possible for ionic liquids to penetrate through skin and come into contact with blood. Therefore, the potential interactions between the IL and the blood together with the influence of the former on the latter need to be clarified from macro and micro perspectives. However, it is not very clear whether common ionic liquids can cause adverse effects on blood up to now.
Besides, when ILs are widely used in large scale for industrial purposes, their intrusion into the body due to inadvertent direct exposure can also exist, which still involves their impact on blood. There are some studies investigating the toxicity of ILs to cells, enzymes, and animals (Citation10, Citation11), and those with fluorinated anions exhibit the risk of releasing HF (Citation12); similar results have triggered further reflection on their green essence and safety. In addition, in recent studies, ILs have caused a lot of interest in them as active pharmaceutical ingredient-based ionic liquids (API-ILs) (Citation13, Citation14). Whether there are suitable candidates among them with specific bioactivity for blood diseases is also worth exploring. Here a model needs to be established to guide researchers to carry out relevant explorations. In the following study, a series of representative imidazolium ILs were mixed with fresh blood to explore their impacts; the latter was quantitatively obtained from rabbits in the experiment, and the blood without adding IL was used as the control group. In addition, thrombolytic properties in vitro of ILs and the specific interactions between the IL and serum albumin were studied at the molecular level.
Result and discussion
Firstly, the influences of 1-butyl-3-methylimidazolium ([Bmim]+) salts with different anions including Br−, Cl−, BF4− and PF6− on fresh rabbit blood were investigated by blood clotting index, hemolysis rate, the content of hemoglobin, erythrocyte morphology, erythrocyte sedimentation rate and the content of serum protein of the mixture. Based on the reported method (Citation15), blood clotting index (BCI) and hemolysis rate of these ionic liquids were determined (See Supplemental Data, SD for the test method). Blood clotting i.e. coagulation and thrombosis mechanisms can be affected by the blood contacting substances, and a high blood clotting index of a substance mean that the blood contacting substance is not changing the normal clotting mechanisms. However, a low blood clotting index means that this substance can promote clotting, which is a hemostatic effect. The lower the blood clotting index, the better the hemostatic effect. It can be found from (a) that clotting indices of [Bmim]Br, [Bmim]Cl, [Bmim]BF4 and [Bmim]PF6 are 71.79%, 76.81%, 69.11% and 87.74%, respectively. Compared with that of the control group, all of them decrease. Besides, compared with clotting indexes 75.6–91.57% of polymeric ionic liquid materials reported in previous study (Citation15), those of ILs are lower, indicating that ILs can interfere with clotting and have hemostatic performance. Compared with other ILs in this paper, [Bmim]BF4 shows the lowest blood clotting index and the most significant hemostatic performance. On the contrary, that of [Bmim]PF6 is very poor. Moreover, hemolysis rates of [Bmim]Br, [Bmim]Cl, [Bmim]BF4 and [Bmim]PF6 are 0.15%, 0.07%, 0.26% and 0.82%, respectively (see (b)). The experimental results show that hemolysis rates of the four ILs are all lower than 2%, indicating that they are non-hemolytic at the experimental concentration 10 mg/mL (Citation15). Furthermore, the hemolysis rate of [Bmim]PF6 is the highest of the four, indicating the best hemolytic properties among them, which is consistent with the findings about the hemostatic performance mentioned above.
Figure 1. (a) Blood clotting index, (b) hemolysis rate, (c) hemoglobin concentration, (d) conductivity and (e) serum protein concentration of blood with [Bmim]Br, [Bmim]Cl, [Bmim]BF4 and [Bmim]PF6.
![Figure 1. (a) Blood clotting index, (b) hemolysis rate, (c) hemoglobin concentration, (d) conductivity and (e) serum protein concentration of blood with [Bmim]Br, [Bmim]Cl, [Bmim]BF4 and [Bmim]PF6.](/cms/asset/3bc2617e-87f2-48ed-8b52-f375ab45c2e5/tgcl_a_2102439_f0001_oc.jpg)
In order to further verify the hemolytic properties of IL, 1 mg or 10 mg of the four ILs was directly mixed with 1 mL of fresh rabbit blood, respectively. After fully mixing, the mixture was centrifuged (3000 rpm) at 4°C for 5 min. Then the ultraviolet spectrum of the supernatant was obtained as shown in Figure S1(a,b) (see SD). Moreover, the concentration of free hemoglobin in plasma was measured by assay kit, and the results are shown in (c). In Figure S1, the hemoglobin peaks at 415 nm and oxygenated hemoglobin peaks at 540 and 576 nm can be observed as before (Citation16). The hemoglobin absorbance has been enhanced after the treatment of ILs, and it increases with the increasing concentration of ILs. According to (c), the free hemoglobin content in plasma treated with [Bmim]PF6 is the highest, which are 632.57 and 864.34 mg/L for two dosages of this IL, respectively. The presence of hemoglobin in plasma usually indicates some breakage of erythrocyte membranes or changes in the permeability of the membranes. Thus, the ILs can destroy erythrocytes to some extent (Citation17), and [Bmim]PF6 exhibits the most obvious destruction of red blood cells and the strongest hemolysis ability. When the concentration of ILs is 10 mg/mL, the free hemoglobin content in plasma reaches the lowest level after treatment with [Bmim]BF4, indicating that it results in less damage to red blood cells than others.
The change of blood rheological properties relates closely to the physiological state of organisms. For example, atherosclerosis, thrombosis, and coronary slow flow will change the blood flow velocity, viscosity, hematokrit (HCT), and so on (Citation18, Citation19). The change of blood rheological properties usually causes the change of its dielectric properties. For the study of its conductivity, blood can be equivalent to a three-element circuit model with plasma resistance, erythrocyte fluid resistance, and cytomembrane capacitance (Citation20). The above results prove that some ILs affect the integrity and permeability of erythrocyte membranes. In addition, cytomembrane capacitance is an important factor affecting blood conductivity. Therefore, ILs would also have a certain impact on blood conductivity. Based on (d), the presence of 0.05 mmol/L IL makes blood conductivity (37°C) increased to different degrees. In order to rule out the influence of ionic liquid itself as an electrolyte on blood conductivity, here the sample of mixed IL and the supernatant after erythrocyte sedimentation (SAES) was applied as a comparison. As a result, the greatest difference between IL + blood and IL + SAES indicates [Bmim]PF6 has the most obvious effect on erythrocyte membranes, which accords with the former finding among the tested ILs. As for the investigation of the level of serum protein in blood, 1 and 10 mg/mL ILs were applied and the results are shown in (e), the serum protein content of control group is 6.0 g/100 mL (5.6–7.6 g/100 mL for human generally) and that of [Bmim]PF6 remains unchanged. The other three ILs show a basic trend that the higher concentration of ILs, the higher the serum protein level. However, all the changes are small, indicating ILs have no obvious effects on serum protein levels.
In consequence, four ILs with various lengths of cationic chain were investigated while the anion was unchanged as PF6−. Therefore, 1-ethyl-3-methylimidazolium hexafluorophosphate ([Emim]PF6), 1-butyl-3-methylimidazolium hexafluorophosphate ([Bmim]PF6), and 1-octyl-3-methylimidazolium hexafluorophosphate ([Omim]PF6) were selected to compare their influence on the same indicators above. When the blood clotting index of the control was regarded as 100%, it would decrease and become 73.35% ([Emim]PF6), 87.74% ([Bmim]PF6) and 97.85% ([Omim]PF6) after the blood was mixed with corresponding ILs with same concentration (1.5 mmol/L), respectively (see (a)). Besides, the blood clotting index rises with the increase of carbon chain length, indicating that the longer the carbon chain, the worse the hemostatic performance. According to (b), the hemolysis rate of [Emim]PF6, [Bmim]PF6 and [Omim]PF6 is 0.45%, 0.82% and 13.30%, respectively. As a result, [Emim]PF6 and [Bmim]PF6 have minimal hemolytic ability at the experimental concentration, while [Omim]PF6 should be marked as a hemolytic substance wrecking the integrity of the cell membrane because the hemolysis rate is higher than 5% (Citation21). Thus, the blood clotting index and hemolysis rate are obviously changed by this kind of IL (Citation20). At the same time, the longer the alkyl chain of ILs, the stronger the destructive effect. Many studies have shown that the hydrophobic interaction of ILs is stronger with the increase of alkyl chain (Citation17, Citation22–24), which means the stronger binding affinity of ILs toward the lipid bilayer. Therefore, the destruction of erythrocyte membranes and changes in permeability are more obvious.
Figure 2. (a) Blood clotting index, (b) hemolysis rate, (c) hemoglobin concentration, (d) conductivity and (e) serum protein concentration of blood with [Emim]PF6, [Bmim]PF6 and [Omim]PF6.
![Figure 2. (a) Blood clotting index, (b) hemolysis rate, (c) hemoglobin concentration, (d) conductivity and (e) serum protein concentration of blood with [Emim]PF6, [Bmim]PF6 and [Omim]PF6.](/cms/asset/654f88f6-581d-424c-bd3c-77d5c7f942cb/tgcl_a_2102439_f0002_oc.jpg)
In detail, Figure S1(c) in SD shows the ultraviolet (UV) spectra of rabbit plasma treated with 1 mg/mL [Emim]PF6, [Bmim]PF6, and [Omim]PF6, respectively. The absorbance of hemoglobin and oxygenated hemoglobin mixed with [Omim]PF6 are both at the maximum level. On the basis of (c), when the concentration of ILs increases, the content of hemoglobin in plasma becomes higher, and that of [Omim]PF6 reaches the highest. Both above indicate that with the increase in the number of carbon atoms, the damage to red blood cells is more serious. Besides that, these three ionic liquids are hydrophobic, and the conductivity of the system increases little or even decreases slightly after they are mixed with SAES. As shown in (d), the conductivity at 37°C of blood treated with [Bmim]PF6 is the highest among the three at the same concentration (0.05 mmol/L). Especially, the conductivity value of blood + [Omim]PF6 is the lowest, which is also lower than that of blank control. In this kind of IL, the blood turns into a jelly state more easily, thus the ion movement in the blood is inhabited and the blood conductivity becomes lower. In terms of (e), there is no significant difference in serum protein content between various ILs and different concentrations of the same IL, which is also near to 6 g/100 mL. Among them, [Emim]PF6 and [Omim]PF6 result in a slight increase in the serum protein content, and that resulting from [Bmim]PF6 at both concentrations is the same as that of the control group basically.
In order to observe related phenomena and support the above findings from macrosystem level, the microscopic morphology and classic erythrocyte sedimentation were considered in the following experiments. As shown in (a), normal red blood cells are double-sided concave cakes with a regular shape. However, when they contact with the IL (contact time: 10 min), their shape changes, and the cells shrink, wrinkled walls make their edge look thicker and darker, and some irregular cells appear. In (b), some erythrocytes in contact with [Bmim]PF6 shrivel, and their membranes are destroyed to different degrees. Furthermore, as can be seen from (c), [Omim]PF6 is relatively more highly toxic to red blood cells, which affects their morphology more obviously. Therefore, it can be concluded that these ILs destroy the phospholipid bilayer of erythrocytes to a certain degree (Citation17). Then, erythrocytes shrink, even rupture, and hemoglobin is released from red blood cells, which is manifested as the increase of hemoglobin concentration in plasma. Thus, the selected ILs show definite hemolytic properties. Erythrocyte sedimentation rate (ESR) is an important index in routine blood testing, which is associated with many kinds of diseases (e.g. inflammation, (Citation25)). In addition, it represents the suspension stability and aggregation rate of erythrocytes (Citation26). As shown in (d), the ESR of blank control is very near to 1 mm/h, which is regarded as the normal level. After mixed with the 10 mg/mL ILs and sedimented for 1 h, ESR decreased with the increase of alkyl chain length on IL cations, which indicates a certain number of the red blood cells are broken and their specific gravity decreases. It is obvious that this result accords with the above findings. Especially, the erythrocyte sedimentation resulting from [Omim]PF6 cannot be observed, owing to its severe destructiveness for blood cells and lowered density of the whole system. Moreover, Figure S2 indicates PF6− has the most obvious effect than other tested anions when the cation is [Bmim]+, which accords with the aforementioned finding. This method does not rely on any special instruments, which can provide a simple and intuitive way to observe the blood change from a macro perspective and then guide further explorations.
Figure 3. Erythrocyte morphology (a) without the IL and that treated with (b) [Bmim]PF6 and (c) [Omim]PF6; (d) erythrocyte sedimentation (±0.01 mm) of blood with ionic liquids ① [Emim]PF6, ② [Bmim]PF6, ③ [Omim]PF6 and ④ without IL; thrombolytic rate of (e) ILs (10 mg/mL) at different time, and (f) different [Emim]PF6 dose in 2 mL PBS at 3 h.
![Figure 3. Erythrocyte morphology (a) without the IL and that treated with (b) [Bmim]PF6 and (c) [Omim]PF6; (d) erythrocyte sedimentation (±0.01 mm) of blood with ionic liquids ① [Emim]PF6, ② [Bmim]PF6, ③ [Omim]PF6 and ④ without IL; thrombolytic rate of (e) ILs (10 mg/mL) at different time, and (f) different [Emim]PF6 dose in 2 mL PBS at 3 h.](/cms/asset/36f5c0b2-e21f-43c8-8569-19f16102b9e8/tgcl_a_2102439_f0003_oc.jpg)
Thirdly, the thrombolytic properties of ILs were investigated based on previous study (Citation27). Here 4 mL of fresh rabbit blood was collected for the following experimental operation. After its complete natural coagulation (0.5 h), blood clots and serum were separated. Then blood clots were cut into small pieces and weighed to be 0.16 ± 0.03 g. Next, 2 mL physiological saline and 20 mg of different ILs and clots were mixed in a water bath at 37°C for 9 h. At the different time points in the range of 1–9 h, related blood clots were dried and weighed at 30 min accordingly. The thrombolytic rate was calculated according to the following equation:
(1)
(1) where R is thrombolytic rate, W0 is the mass of the original blood clot, and W is the mass of the blood clot after a period of dissolution.
According to the results in (e), thrombolytic rate of various ILs increases with the increase of time and is gradually stabilized after 7 h. Under the same dose of ILs in 2 mL PBS, thrombolytic rate of [Emim]PF6 is higher than other ILs at each time point and nearer to that of the control at the end, and nearly half of the blood clot was dissolved by the effect of this kind of IL; while the level of growth on that of [Omim]PF6 was the highest within the investigated duration. Before 9 h, the trend is obviously time-dependent. For comparison, the residual blood clots resulting from [Bmim]Cl, [Bmim]Br, [Bmim]BF4, [Bmim]PF4, [Bmim]PF6 and [Omim]PF6 are more that of the control and [Emim]PF6, indicating they can prevent thrombolysis. Then different concentrations of [Emim]PF6 were selected to explore the influence on the thrombolytic rate. (f) shows the stages of thrombolytic process and the thrombolytic rate varied with IL concentration after contacting for 3 h. It can be obviously seen the thrombolytic rate decreases with the increase of concentration, indicating that the thrombolysis can be inhibited by a high concentration of the IL more easily. When the dose of IL is high, the viscosity of the system is increased and the intermolecular interaction of IL itself is significantly strengthened.
Finally, various spectral techniques were used to explore the interactions between the ionic liquid and serum albumin qualitatively and quantitatively as in a previous study (Citation28) on the premise that the latter is not destroyed or seriously affected by IL. On the basis of obtained results, here [Bmim]BF4 was selected due to its less impacts on blood for the involved indexes. A series of solutions with 10 μM rabbit serum albumin (RSA) mixed with 0–10 μM [Bmim]BF4 was used as the investigated objects firstly. After blank deduction, their ultraviolet spectra in the range of 240–300 nm at different IL concentrations are shown in (a). The maximum absorbance wavelength (λmax) of the protein and its mixture with IL is found at 278 nm, similar to that reported in the literature (Citation29). With the increase of IL concentration, the absorbance at λmax increases, indicating that the IL and RSA are associated. The association constant can be calculated according to the following equation (Citation30).
(2)
(2) where A0 and A are the absorbance of RSA with the absence and presence of [Bmim]BF4, respectively; ϵSA and ϵIL-SA are the absorbance coefficient of RSA and IL-RSA complex, respectively; kb is the association constant, and [IL] is the concentration of [Bmim]BF4. According to Equation (2), the linear regression curve can be developed as y = 2.9750 × 10−5x+4.4358 (R2 = 0.9898) in the range of 1 × 105–5 × 105 L/mol with
as y and 1/[IL] as x, and R2 is 0.9898. Then the association constant kb corresponds to the ratio of the intercept to slope, which can be calculated as 147,860. This value is of the same order of magnitude as the value reported in current literature (Citation30), indicating the fairly strong connection between [Bmim]BF4 and RSA. Obviously, the results of qualitative and quantitative analysis show that [Bmim]BF4 has been associated with RSA. Moreover, both the whole UV profile and λmax exhibit no obvious difference in (a) before and after mixed with IL, suggesting that RSA can remain its original properties and structures under the effect of the latter. Moreover, (b) shows the fluorescence spectra of the mixture composed by [Bmim]BF4 and RSA. The fluorescence emission spectrum of each objective solution with excitation at 280 nm is similar and the fluorescence emission wavelength remains at 330 nm. Different concentrations of [Bmim]BF4 have an impact on fluorescence intensity at 330 nm, but it is not significant. Here the IL influence can also be reflected from the IR spectrum in (c). The characteristic peaks of IL-RSA complex solutions are similar under different IL concentrations. Among them, the peak at 3440 cm−1 belongs to O–H; the peaks at 2920 and 2850 cm−1 are attributed to aliphatic C–H stretching vibration of protein and [Bmim]BF4. The typical signal at 1640 cm−1 is the combination of amide I and II bands of RSA, attributed to C=O and N–H. Besides, the peak at 1475 cm−1 is the amide III band of RSA, resulting from C–N; and the peaks at 1120 and 1068 cm−1 belong to C–O and B–F, respectively. The above results indicate there is no noticeable structural change of RSA after bound with the IL.
Figure 4. (a) UV spectra with different [Bmim]BF4 concentrations; (b) fluorescence spectra with different [Bmim]BF4 concentrations and the fluorescence of the combined solution without or with 10 μM [Bmim]BF4 under 280 nm; (c) IR spectra of RSA with different [Bmim]BF4 concentrations; (d) the absorbance of the complex formed by the mixture of [Bmim]BF4 and two kinds of serum albumin at 278 nm; molecular docking diagram of the interactions between [Bmim]BF4 (green, blue, pink and cyanic sticks) and (e) RSA amino acid residues (yellow fragments) or (f) HSA amino acid residues (cyanic fragments).
![Figure 4. (a) UV spectra with different [Bmim]BF4 concentrations; (b) fluorescence spectra with different [Bmim]BF4 concentrations and the fluorescence of the combined solution without or with 10 μM [Bmim]BF4 under 280 nm; (c) IR spectra of RSA with different [Bmim]BF4 concentrations; (d) the absorbance of the complex formed by the mixture of [Bmim]BF4 and two kinds of serum albumin at 278 nm; molecular docking diagram of the interactions between [Bmim]BF4 (green, blue, pink and cyanic sticks) and (e) RSA amino acid residues (yellow fragments) or (f) HSA amino acid residues (cyanic fragments).](/cms/asset/4a9b16b3-d7e0-427f-bff1-c7c0e993c319/tgcl_a_2102439_f0004_oc.jpg)
On the basis of the above findings, human serum albumin (HSA) was also studied spectroscopically with [Bmim]BF4 for the following comparison. The ultraviolet, infrared and fluorescence spectra for the mixture of [Bmim]BF4 and HSA are shown in Figure S3. As same as above, the maximum absorbance wavelength is still 278 nm in UV analysis and the maximum fluorescence emission wavelength is 330 nm. With the increase of IL concentration, ultraviolet and fluorescence intensity slightly increases and decreases, respectively. For comparison, the absorbance of the complex formed by [Bmim]BF4 and two kinds of serum albumins at 278 nm is shown in (d). As a whole, the increase of IL concentration exerts more obvious influence on RSA than HSA. In order to further demonstrate the interactions between them, molecular docking was employed (see SD for details) and the simulations of [Bmim]BF4 binding to the different sites on RSA and HSA are shown in (e,f). It can be seen that [Bmim]BF4 exhibits binding affinity with RSA via hydrophobic interactions with Cys-392, Val-388, Leu-446, Sys-437, Lys-439 and Glu-396. Besides, the IL molecule interacts with HSA, occupying the positions of Asn-130, Thr-133, Lys-137, Phe-134, Thr-125 and Asp-129.
Experimental section
All of the ionic liquids, reagents and solvents used in the present study were of analytical-reagent grade or higher. The experimental animals were three Japanese white rabbits (male, younger than three months, 1.8–2 kg) and their blood was collected in tubes with ethylene diamine tetraacetic acid-dipotassium salt (EDTA-K2) and sodium citrate or heparin sodium. Based on the reported methods (e.g. 15), blood clotting index (BCI), hemolysis rate, hemoglobin content, erythrocyte morphology, blood conductivity, erythrocyte sedimentation rate, and serum protein content were all systematically studied, and the experimental details can be found in SD.
Conclusions
In summary, ILs will have certain effects on blood after entering the blood, so it is necessary to be cautious when using them in the field of biomedicine, especially those with long alkyl-substituted cations and fluorinated anions. When the cations are the same, the effect of PF6− on the blood system is more obvious than other anions; when the anion is fixed to PF6−, the effect of substituted imidazolium ILs with long alkyl chain on the blood system is more significant. Among the tested ILs, [Bmim]BF4 shows less damage on rabbit blood, and it should be continuously focused on some ILs for their significant destruction of erythrocytes (e.g. [Omim]PF6). Moreover, the thrombolytic ability of [Emim]PF6 can be paid more attention by researchers, meanwhile, a high concentration of ILs will inhibit thrombolysis. Finally, it is concluded that [Bmim]BF4 associates with serum albumin in different binding sites through specific interactions and this kind of association does not change the spectral characteristics and structure of the latter. This is the first time that ILs have been investigated for their obvious and potential effects on blood, and related results provide a reference for their safety assessment. When using them in daily work, it is recommended to wear protective clothing together with gloves (considering their good solubility and permeability, plastic coated gloves are suggested which are not breathable and uneasy to break) for preventing transdermal absorption into blood (Citation31). Especially when there are wounds on the body’s surface, special attention should be paid to isolating the wounds and strengthening protection for prevention. When hydrophilic ILs contact with skin, it is only necessary to wipe the excess ILs with a cleaning cloth first, and then rinse the contact part thoroughly with water immediately (if penetration has occurred, it should be immediately treated by immersion); on the contrary, if hydrophobic ILs are in contact with skin, it is recommended to apply lipid to fully dissolve them, and then thoroughly wash them with hand sanitizer.
Supplemental Material
Download PDF (447.3 KB)Acknowledgements
Special thanks to the following affiliations in no particular order: School of Chemical Engineering, Sichuan University for the FT-IR analysis. Experimental and Research Animal Institute of Sichuan University for providing standardized research site and technical assistance.
Disclosure statement
No potential conflict of interest was reported by the author(s).
Additional information
Funding
References
- Suresh; Sandhu, J.S. Green Chem. Lett. Rev. 2011, 4, 289–310.
- Suresh; Sandhu, J.S. Green Chem. Lett. Rev. 2011, 4, 311–320.
- Lv, G.; Li, Z.; Jiang, W.T.; Xu, S.; Larson, T.E. Green Chem. Lett. Rev. 2014, 7, 191–198.
- Zhao, S.; Meng, D.; Wei, L.; Qiao, Y.; Xi, F. Green Chem. Lett. Rev. 2019, 12, 26–32.
- Gao, Y.R.; Hao, Y.N.; Zhang, W.X.; Wei, Y.N.; Shu, Y.; Wang, J.H. Chem. Eng. J. 2022, 429, 131590.
- Rykowska, I.; Ziemblińska, J.; Nowak, I. J. Mol. Liq. 2018, 259, 319–339.
- Mizuuchi, H.; Jaitely, V.; Murdan, S. Eur. J. Pharm. Sci. 2008, 33, 326–331.
- Paul, B.K.; Moulik, S.P. Ionic Liquids in Colloidal Regime; John Wiley & Sons: New York, 2015; pp 228–231.
- Zhang, D.; Wang, H.J.; Cui, X.M.; Wang, C.X. Pharm. Dev. Technol 2017, 22, 511–520.
- Errazquin, D.; Mohamadou, A.; Dupont, L.; Gaetano, Y.D.; García, C.B.; Lomba, L.; Giner, B. Environ. Sci. Pollut. Res. 2021, 28, 65374–65384.
- Montalbán, M.G.; Víllora, G.; Licence, P. Ecotoxicol. Environ. Safe 2018, 150, 129–135.
- Freire, M.G.; Neves, C.; Marrucho, I.M.; Coutinho, J.; Fernandes, A.M. J. Phys. Chem. A 2010, 114, 3744–3749.
- Cole, M.R.; Li, M.; El-Zahab, B.; Janes, M.E.; Hayes, D.; Warner, I.M. Chem. Biol. Drug Des. 2011, 78, 33–41.
- Balk, A.; Holzgrabe, U.; Meinel, L. Eur. J. Pharm. Biopharm. 2015, 94, 291–304.
- Sahiner, N.; Sagbas, S. Sep. Purif. Technol. 2018, 196, 208–216.
- Zijlstra, W.G.; Buursma, A.; Meeuwsen-van der Roest, W.P. Clin. Chem. 1991, 37, 1633–1638.
- Radošević, K.; Cvjetko, M.; Kopjar, N.; Novak, R.; Dumić, J.; Srček, V.G. Ecotoxicol. Environ. Safe 2013, 92, 112–118.
- Baskurt, O.K.; Meiselman, H.J. Semin. Thromb. Hemost. 2003, 29, 435–450.
- Ergun-Cagli, K.; Ileri- Gurel, E. Clin. Hemorheol. Microcirc. 2011, 47, 27–35.
- Chang, Z.Y.; Pop, G.A.M.; Meijer, G.C.M. IEEE. Trans. Biomed. Eng. 2008, 55, 1247–1249.
- Ruokonen, S.K.; Sanwald, C.; Robciuc, A.; Hietala, S.; Rantamaki, A.H.; Witos, J.; King, A.W.T.; Lammerhofer, M.; Wiedmer, S.K. Chem. Eur. J. 2018, 24, 2669–2680.
- Nicola, W.; Jamie, L.F.; H.Q. Nimal, G.; Kenneth, R.S.; Royston, G.; Gillian, M.S. Green Chem. 2011, 13, 1843–1851.
- Marija, P.; Kenneth, R.S.; Luís, P.N.R.; Cristina, S.P. Chem. Soc. Rev. 2011, 40, 1383–1403.
- Navleen, K.; Sandeep, K.; Shiksha; Gagandeep, K.G.; Venus, S.M. J. Phys. Chem. B 2021, 125, 3613–3621.
- Harrison, M. Aust. Prescr. 2015, 38, 93–94.
- Naumov, A.A.; Dubrovskii, A.V.; Potselueva, M.M.; Tikhonenko, S.A. Bull. Exp. Biol. Med. 2017, 163, 42–45.
- Chazalviel, L.; Haelewyn, B.; Degoulet, M.; Blatteau, J.E.; Vallée, N.; Risso, J.J.; Besnard, S.; Abraini, J.H. Med. Gas. Res. 2016, 6, 64–69.
- Chanphai, P.; Tajmir-Riahi, H.A. J. Mol. Liq. 2020, 299, 112178.
- Kooravand, M.; Asadpour, S.; Haddadi, H.; Farhadian, S. J. Hazard. Mater. 2021, 407, 124878.
- Zhong, W.Y.; Wang, Y.C.; Yu, J.S.; Liang, Y.Q.; Ni, K.Y.; Tu, S.Z. J. Pharm. Sci. 2004, 93, 1039–1046.
- Zhang, T.H.; Peng, X.; Li, F.L.; Toufouki, S.; Yao, S. J. Mol. Liq. 2022, 353, 118770.