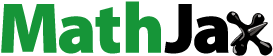
ABSTRACT
Herein are reported the syntheses of poly(9,9-di-n-octylfluorenyl-2,7-diyl) (PF), poly(9,9-dioctylfluorene-alt-benzothiadiazole) (PFBT), and poly[(9,9-bis(3′-(N,N-dimethylamino)propyl)-2,7-fluorene)-alt-2,7-(9,9-dioctylfluorene)] (PFN) by employing catalytic mechanochemical Suzuki polymerization in a ball mill. This mechanochemical synthesis technique can yield soluble functionalized polyfluorenes and its derivatives in 30 min or less. Furthermore, the polymer light emitting diode and photovoltaic interfacial electrolyte, poly(9,9-bis(3′-(N,N-dimethyl)-N-ethylammoinium-propyl-2,7-fluorene)-alt-2,7-(9,9-dioctylfluprene))dibromide (PFN-Br), was obtained via a facile mechanochemical quaternization reaction at the terminal amino groups of PFN.
GRAPHICAL ABSTRACT

Introduction
Conjugated polymers have been a staple for the organic electronic community due to their excellent optoelectronics (Citation1, Citation2). More importantly, carbon–carbon cross coupling reactions, such as Kumada (Citation3, Citation4), Sonagashira (Citation5–7), Negishi (Citation8, Citation9), Mizoroki Heck (Citation10, Citation11), and Suzuki–Miyaura (Citation12, Citation13), have been essential to the synthesis of the several conjugated polymers (Citation14). In most cases, these cross-coupling polymerizations have occurred in a homogeneous solution or a biphase system (Citation15–17). Recently, a few conjugated polymers have been synthesized by solvent-free mechanochemical grinding in a ball mill (Citation18–24). These mechanochemical polymerizations have proved to be facile, consuming less energy, reducing waste produced and circumventing possible solubility issues from incompatible monomers. In particular, Borchardt and coworkers demonstrated the mechanochemical Suzuki polymerization of poly(para-phenylene) (Citation25, Citation26). However, most of the materials produced lacked sufficient solubility for traditional polymer characterization for determining number average molecular weight (Mn), weight average molecular weight (Mw), polydispersity (Ð) and yield. We thought it was timely to synthesize soluble conjugated polymers in a ball mill to assess how the reaction parameters, such as milling time, milling frequency and catalyst loading, influence the polymer molecular weights, polydispersity and yield for catalytic ball milled polymerization reactions.
Soluble polyfluorenes were chosen as the candidate for this mechanochemical Suzuki polymerization study. These compounds are considered as one of the most attractive blue-emissive materials due to their charge transport properties, high efficiency, high chemical and thermal stability, high solubility, film-forming properties, and excellent tunability through chemical modification and copolymerization (Citation27–30). In addition, polyfluorenes can be widely used as active materials in optoelectronic devices, particularly in PLEDs (Citation31, Citation32).
Results and discussion
To investigate the mechanochemical Suzuki polymerization, we selected the synthesis of poly(9,9-di-n-octylfluorenyl-2,7-diyl) (Scheme 1, PF). The initial catalytic mechanochemical polymerization conditions were modeled according to Borchardt’s conditions (Citation26). Thus, 9,9-dioctylfluorene-2,7-diboronic acid (0.105 mmol), 9,9-dioctyl-2,7-dibromofluorene (0.105 mmol), K2CO3 (0.832 mmol), and Pd(OAc)2 (0.005 mmol) were added to a stainless steel milling jar with four stainless steel balls (5 mm) and then subjected to high speed ball milling at 1800 RPM for a 30 min milling time. Initial results showed that PF could be synthesized with Mn, Mw, and Ð of 4.2, 8.3 kDa, and 1.9, respectively, and an isolated yield of 50%. However, a considerable amount of insoluble polymer remained in a cellulose thimble after Soxhlet extraction with methanol, acetone, and chloroform. For comparison of the polymer size, dispersity and yield, we applied literature conditions to synthesize PF in solution which afforded PF with a Mn of 9.26, Ð of 1.91 and an isolated yield of 53% (Citation33). The green metrics for the Suzuki polymerization reaction in the solution phase and ball mill were evaluated using the EcoScale calculator (Supplementary information, Figures S3 and S4) (Citation34). The total score on the EcoScale was 21 for solution phase and 59 for ball mill demonstrating that the ball-milled polymerization was a better procedure for the preparation of PF.
Inspired by these preliminary results, we conducted a series of mechanochemical polymerizations by varying the milling frequency, milling time, and catalyst loading in order to assess the influence of these parameters on the solid-state polymerization. An overview of the reaction optimization is given in . Since, there is strong evidence in the relevant literature concerning the influence of milling frequency on solid-state reactions; we chose to vary the milling frequency (, entry 1, 2, and 3). However, this reaction parameter only exhibited a minimum effect on polymer molecular weight, dispersity and yield. By varying the milling time (, entry 3, 4, and 5), the molecular weights decreased significantly. The isolated yields remained constant for milling times of 30 and 15 min, whereas the milling time of 5 min resulted in a dramatic decrease in the isolated yield. Furthermore, the Pd loading was reduced from 10 mol% to 2.5 mol% (, entry 5, 6, and 7) which significantly reduced the yield, Mn and Mw values. Controlled reactions were carried out to determine the catalytic nature and base requirement for the polymerization reaction. In the absence of both variables, no polymer formation was observed, confirming the requirement of the base and the catalyst in this reaction.
Table 1. Reaction optimization for the mechanochemical synthesis of PF.
In the field of the organic semiconductors, the most representative and widely used conjugated polymers are donor–acceptor polymers. As a result, we thought it is noteworthy to extend our mechanochemical Suzuki polymerization for the synthesis of a donor–acceptor polymer. We selected the donor–acceptor polymer, poly(9,9-dioctylfluorene-alt-benzo-thiadiazole) (PFBT) which is a commercially available compound, for the synthesis of OLEDs, OFETs and OPVs (Scheme 1, PFBT) (Citation35). Optimized mechanochemical Suzuki polymerization conditions (, entry 3) were applied for the synthesis of PFBT. The resulting polymer had a Mn of 5.6 kDa, Ð of 1.4 (Supplementary information, Figure S2) with an isolated yield of 25%. Surprisingly, the reduction of the milling time (, Entry 1-4) displayed a significant increase in polymer molecular weight. It was observed that longer milling time (>5 min) resulted in more insoluble polymeric materials. We believe this could be due to high molecular weight polymers forming and/or crosslinking (Citation18). At 5 min milling time, the isolated PFBT had a Mn of 7.4 kDa, Ð of 1.7 and an isolated yield of 40%. This data suggest that this methodology can be applied for the synthesis of electronic donor–acceptor materials.
Table 2. Impact of milling time of the mechanochemical synthesis of PFBT.
To showcase the utility of this mechanochemical Suzuki polymerization, we elected to synthesize the conjugated polyelectrolyte, poly(9,9-bis(3′-(N,N-dimethyl)-N-ethylammoinium-propyl-2,7-fluorene)-alt-2,7-(9,9-dioctylfluorene))dibromide (Scheme 2, PFN-Br). With hydrophobic and hydrophilic monomer units, PFN-Br serves as an electron-interface layer material in OFETs, OLEDs, OPVs and perovskite solar cells to improve the interfacial properties (Citation36). The mechanochemical synthesis of PFN-Br was achieved in two steps. First, poly[(9,9-bis(3′-(N,N-dimethylamino)propyl)-2,7-fluorene)-alt-2,7-(9,9-dioctylfluorene)] (PFN) was synthesized in a ball mill using our optimized mechanochemical conditions (Scheme 1, PFN). By increasing the milling frequency from 600 to 1000 rpm, the maximum polymer molecular weight of 10.2 kDa (Supplementary information, Figure S2) was afforded with an isolated yield of 35%. The second step towards the desired polyelectrolyte PFN-Br would be a mechanochemical quaternization of the amino groups on the fluorene moiety with bromoethane. However, the mechanochemical quaternization reaction in the ball mill resulted in no reaction. It has been reported that temperature can be key factor for promoting ball-milled reaction (Citation38–40). Therefore, we were motivated to conduct the mechanochemical quaternization process in our FlackTek speedmixer since it has an average milling jar temperature of 41 °C compare the 23 °C in ball mill when measured with Etekcity infrared thermometer. However, the process still resulted in no reaction. The previously reported quaternization reaction for PFN in solution required a mixture of DMSO in THF (1:4 ratio) (Citation37). Therefore, we conducted the mechanochemical quaternization reaction under these liquid-assisted grinding conditions which afforded the desired polyelectrolyte, PFN-Br, in a good, isolated yield of 70%. The polymeric structures of PFN and PFN-Br were confirmed with 1H NMR analyses. The optical properties of PFN and PFN-Br were assessed and displayed similar spectral responses as previously reported (Supplementary information, Figure S1) (Citation37).
Conclusions
Herein, we presented the catalytic mechanochemical synthesis of soluble fluorene-based conjugated polymers PF, PFBT and PFN. This mechanochemical Suzuki polymerization offers a facile, simple, solvent-free synthetic route to soluble functionalized polyfluorenes and its derivatives. We conducted a series of mechanochemical polymerization reactions for the synthesis of PF to evaluate the effect of reaction parameters such as milling frequency, milling time and catalyst loading on the polymer molecular weights, dispersity and yield. The milling frequency and time did not dramatically affect the polymer molecular weight, dispersity and yield. However, Pd catalyst loadings had the more dramatic effect on the molecular weights, dispersity, and yield. Moreover, green metrics were evaluated for the synthesis of PF in the solution phase and ball mill using EcoScale calculator, and demonstrate that the ball-milled polymerization was a better procedure for the preparation of PF. Moreover, the conjugated polyelectrolyte, PFN-Br, was synthesized via a facile mechanochemical quaternization of PFN at the terminal amino groups. Furthermore, this methodology opens an opportunity for the synthesis of new polymeric materials where the monomers may not be compatible with the solvent system.
Experimental section
Materials
9,9-Dioctylfluorene-2,7-diboronic acid, 9,9-dioctyl-2,7-dibromofluorene, 4,7-dibromobenzo[c]-1,2,5-thiadiazole, and Pd(OAc)2 were purchased from Sigma Aldrich. 2,7-Dibromo-9,9-bis[3-(dimethyl amino)propyl]fluorene and bromoethane was purchased from TCI America, and K2CO3 was purchased from Acros. All reagents were used as received. THF was obtained from an Innovative Technologies solvent purification system under ultra-pure argon. Water was degassed under argon. All the other solvents were used as received.
Instrumentation
The milling experiments were carried out in FORM-TECH Scientific (FTS-1000) instrument and FlackTek speedmixer with SmartSnap stainless-steel grinding jars and stainless-steel balls (5 mm). 1H NMR spectra were measured on a Bruker Avance 400 MHz instrument. Number average molecular weight (Mn), weight average molecular weight (Mw) and, polydispersity (Ð) were determined by gel permeation chromatography (GPC) using a Waters pump with a Waters 2410 refractive index detector. THF was used as an eluent at 35○C with a flow rate of 1.0 mLmin−1. The GPC instrument was calibrated with polystyrene standards, and data were analyzed using Breeze software.
Synthesis
General experimental procedure for the synthesis of PF via mechanochemical Suzuki polymerization: To a stainless steel grinding jar (5 mL) were added 9,9-dioctylfluorene-2,7-diboronic acid (100 mg, 0.209 mmol), 9,9-dioctyl-2,7-dibromofluorene (115 mg, 0.209 mmol), Pd(OAc)2 (4.69 mg, 0.010 mmol) and K2CO3 (231 mg, 1.67 mmol), followed by stainless-steel balls (5 mm, 4 balls). The grinding jar (5 mL) was sealed and placed in the vibrational ball mill and subjected to milling for 30 min at 1800rpm. After the allotted time, the product was precipitated in methanol and refrigerated overnight. Then the solid was washed in a Soxhlet extraction apparatus using methanol and acetone to remove oligomers and catalyst residues. The chloroform extracted resulted in a dark green-yellow film, yield (83 mg, 51%). 1H NMR (400 MHz, CDCl3) δ 7.87–7.79 (d, 2H), 7.72–7.64 (m, 4H), 2.20–2.01 (m, 4H), 1.22–1.07 (m, 24H), 0.83–0.79 (t, 6H). Mn = 8.3 kDa, Ð = 2.1.
General experimental procedure for the synthesis of PF via Suzuki polymerization in solution: To a 10-mL Schlenk flask were added 9,9-dioctylfluorene-2,7-diboronic acid (100 mg, 0.209 mmol), 9,9-dioctyl-2,7-dibromofluorene (115 mg, 0.209 mmol), Pd(OAc)2 (4.69 mg, 0.010 mmol) and K2CO3 (231 mg, 1.672 mmol) inside a glove box. THF (4 mL) and degassed water (4 mL) was added to the reaction mixture. The system was sealed and refluxed for 48 h. The reaction mixture was poured into 6 N HCl (15 mL), extracted with CHCl3, and the solvent was evaporated. The crude product obtained was dissolved in a minimum amount of THF and precipitated in methanol (40 mL). Then the precipitate was poured into a cellulose thimble and subjected to Soxhlet extraction with methanol, acetone, and chloroform. The chloroform extract was evaporated to obtain a dark brownish, orange film, yield (86 mg, 53%). 1H NMR (400 MHz, CDCl3) δ 7.90–7.50 (d, 6H), 2.20–1.98 (m, 4H), 1.27–0.98 (m, 24H), 0.94–0.75 (t, 6H). Mn = 9.3 kDa, Ð = 1.9.
General experimental procedure for the synthesis of PFBT via mechanochemical Suzuki polymerization: To a stainless-steel grinding jar (5 mL) were added 9,9-dioctylfluorene-2,7-diboronic acid (100 mg, 0.209 mmol), 4,7-dibromobenzo[c]-1,2,5-thiadiazole (61.5 mg, 0.209 mmol), Pd(OAc)2 (4.70 mg, 0.021 mmol) and K2CO3 (231 mg, 1.672 mmol), followed by stainless-steel balls (5 mm, 4 balls). The vial (5 mL) was sealed and placed in the vibrational ball mill and subjected to milling for 5 min at 600 rpm. After the allotted time, methanol (40 mL) was added to remove the reaction mixture from the milling jar, followed by overnight refrigeration. The reaction mixture was poured into a cellulose thimble, and subjected to Soxhlet extraction with methanol, acetone, and chloroform. The chloroform extract was evaporated to obtain a dark yellowish-brown film, yield (48 mg, 45%). 1H NMR (400 MHz, CDCl3) δ 8.14–7.83 (d, 4H), 7.81–7.59 (d, 6H), 2.26–1.95 (t, 4H) 1.35–1.01 (m, 20H) 0.99–0.65 (m, 10H). Mn = 5.8 kDa, Ð = 1.8.
General experimental procedure for the synthesis of PFN via mechanochemical Suzuki polymerization. To a stainless-steel grinding jar (5 mL) were added 9,9-dioctylfluorene-2,7-diboronic acid (50 mg, 0.105 mmol), 2,7-dibromo-9,9-bis[3-(dimethyl amino)propyl]fluorene (50 mg, 0.105 mmol), Pd(OAc)2 (2.35 mg, 0.010 mmol) and K2CO3 (115.58 mg, 0.836 mmol) followed by stainless-steel balls (5 mm, 4 balls). The vial (5 mL) was sealed and placed in a vibrational ball mill and subjected to milling for 30 min at 1000 rpm. After the allotted time, the product was dissolved in CHCl3 (1 mL) and precipitated in methanol (80 mL). The mixture was refrigerated overnight, and then poured into a cellulose thimble and subjected to Soxhlet extraction with acetone and chloroform. The chloroform extract was evaporated to obtain a yellow film, yield (23.3 mg, 31%). 1H NMR (400 MHz, CDCl3) δ 7.45.7.95 (d, 12H), 2.96–2.77 (m, 4H), 2.76–2.53 (m, 8H), 2.47–1.97 (m, 12H), 1.30–1.00 (m, 24H), 0.88–0.74 (m, 10H). Mn = 8.6 kDa, Ð = 2.5.
General experimental procedure for the mechanochemical synthesis of PFN-Br: To a stainless-steel grinding jar (15 mL) was added the PFN polymer (50 mg) dissolved in THF (1 mL). To this mixture were added bromoethane (858 mg, 7.9 mmol) and DMSO (0.25 mL), followed by stainless-steel balls (5 mm, 10 balls). The vial (15 mL) was sealed and placed in the speedmixer and subjected to mixing for 60 min at 2500 rpm. After the allotted time, the reaction mixture was removed and placed in a vial, and then THF and excess bromoethane were evaporated. Acetone (20 mL) was added to the polymer mixture and then was centrifuged, washed with DCM, and dried overnight under vacuum, yield (46 mg, 70%). 1H NMR (400 MHz, CDCl3) δ 7.68–8.09 (m, 12H), 2.07–3.25 (m, 30H), 0.95–1.35 (m, 32H), 0.78–0.87 (m, 6H).
Supplemental Material
Download MS Word (977.6 KB)Acknowledgements
Dr. Nelson gratefully acknowledges the Sloan Scholars Mentoring Network of the Social Science Research Council with funds provided by the Alfred P. Sloan Foundation. The manuscript was written through contributions of all authors. All authors have given approval to the final version of the manuscript.
Data availability statement
NMR, UV-vis absorption and emission spectra for PF, PFBT, PFN and PFN-Br are available in supplementary information. GPC traces for PF, PFBT, and PFN and The EcoScale calculations are also available.
Disclosure statement
No potential conflict of interest was reported by the author(s).
Additional information
Funding
References
- Salaneck, W.; Sato, N.; Lazzaroni, R.; Lögdlund, M. Electronic and Chemical Structure of Conjugated Polymer ‘Surfaces’. Vacuum 1990, 41 (7-9), 1648–1650.
- Cacialli, F. Organic Semiconductors for the New Millennium. Philos. Trans. A. Math. Phys. Eng. Sci. 2000, 358 (1765), 173–192.
- Tamao, K.; Sumitani, K.; Kumada, M. Selective Carbon-carbon Bond Formation by Cross-coupling of Grignard Reagents with Organic Halides. Catalysis by Nickel-Phosphine Complexes. J. Am. Chem. Soc. 1972, 94 (12), 4374–4376.
- Limmert, M.E.; Roy, A.H.; Hartwig, J.F. Kumada Coupling of Aryl and Vinyl Tosylates Under Mild Conditions. J. Org Chem. 2005, 70 (23), 9364–9370.
- Elangovan, A.; Wang, Y.-H.; Ho, T.-I. Sonogashira Coupling Reaction with Diminished Homocoupling. Org. Lett. 2003, 5 (11), 1841–1844.
- Sonogashira, K.; Tohda, Y.; Hagihara, N. A Convenient Synthesis of Acetylenes: Catalytic Substitutions of Acetylenic Hydrogen with Bromoalkenes, Iodoarenes and Bromopyridines. Tetrahedron Lett. 1975, 16 (50), 4467–4470.
- Karpov, A.S.; Müller, T.J. New Entry to a Three-component Pyrimidine Synthesis by TMS−Ynones via Sonogashira Coupling. Org. Lett. 2003, 5 (19), 3451–3454.
- Negishi, E.I. Magical Power of Transition Metals: Past, Present, and Future (Nobel Lecture). Angew. Chem., Int. Ed. 2011, 50 (30), 6738–6764.
- Negishi, E. Palladium- or Nickel-Catalyzed Cross Coupling. A New Selective Method for Carbon-Carbon Bond Formation. Acc. Chem. Res. 1982, 15 (11), 340–348.
- Beletskaya, I.P.; Cheprakov, A.V.J.C.R. The Heck Reaction as a Sharpening Stone of Palladium Catalysis. Chem. Rev. 2000, 100 (8), 3009–3066.
- Nicolaou, K.; Bulger, P.G.; Sarlah, D. Palladium-catalyzed Cross-coupling Reactions in Total Synthesis. Angew. Chem., Int. Ed. 2005, 44 (29), 4442–4489.
- Miyaura, N.; Suzuki, A.J.C.R. Palladium-catalyzed Cross-coupling Reactions of Organoboron Compounds. Chem. Rev. 1995, 95 (7), 2457–2483.
- Schlüter, A.D. The Tenth Anniversary of Suzuki Polycondensation (SPC). J. Polym. Sci., Part A: Polym. Chem. 2001, 39 (10), 1533–1556.
- Cheng, Y.-J.; Luh, T.-Y. Synthesizing Optoelectronic Heteroaromatic Conjugated Polymers by Cross-coupling Reactions. J. Organomet. Chem. 2004, 689 (24), 4137–4148.
- Kim, Y.-H.; Shin, D.-C.; Kwon, S.-K. Synthesis and Characterization of Poly (Terphenylenevinylene) Derivative with Electron Withdrawing CN Group and an Electron Donating Alkoxy Group. Polymer 2005, 46 (13), 4647–4653.
- Lopez, L.C.; Strohriegl, P.; Stübinger, T. Synthesis of Poly (Fluorenevinylene-co- Phenylenevinylene) by Suzuki Coupling. Macromol. Chem. Phys. 2002, 203 (13), 1926–1930.
- Babudri, F.; Cardone, A.; Farinola, G.M.; Naso, F.; Cassano, T.; Chiavarone, L.; Tommasi, R. Synthesis and Optical Properties of a Copolymer of Tetrafluoro-and Dialkoxy-Substituted Poly (p-Phenylenevinylene) with a High Percentage of Fluorinated Units. Macromol. Chem. Phys. 2003, 204 (13), 1621–1627.
- Ravnsbæk, J.B.; Swager, T.M. Mechanochemical Synthesis of Poly(Phenylene Vinylenes). ACS Macro Lett. 2014, 3 (4), 305–309.
- Huang, J.; Moore, J.A.; Acquaye, J.H.; Kaner, R.B. Mechanochemical Route to the Conducting Polymer Polyaniline. Macromolecules 2005, 38 (2), 317–321.
- Mamtimin, X.; Matsidik, R.; Obulda, A.; Sidik, S.; Nurulla, I. Polymers, Solid-state Polymerization of EDOT Derivatives Containing Acenaphthenequinoxaline Ring. Fibers Polym. 2013, 14 (7), 1066–1072.
- Posudievsky, O.Y.; Goncharuk, O.; Pokhodenko, V. Mechanochemical Preparation of Conducting Polymers and Oligomers. Synth. Met. 2010, 160 (1-2), 47–51.
- Posudievsky, O.Y.; Goncharuk, O.; Barille, R.; Pokhodenko, V. Structure–Property Relationship in Mechanochemically Prepared Polyaniline. Synth. Met. 2010, 160 (5- 6), 462–467.
- Thorwirth, R.; Stolle, A.; Ondruschka, B. Fast Copper-, Ligand- and Solvent-Free Sonogashira Coupling in a Ball Mill. Green Chem. 2010, 12 (6), 985–991.
- Tullberg, E.; Peters, D.; Frejd, T. The Heck Reaction Under Ball-Milling Conditions. J. Organomet. Chem. 2004, 689 (23), 3778–3781.
- Grätz, S.; Wolfrum, B.; Borchardt, L. Mechanochemical Suzuki Polycondensation – from Linear to Hyperbranched Polyphenylenes. Green Chem. 2017, 19 (13), 2973–2979.
- Vogt, C.G.; Grätz, S.; Lukin, S.; Halasz, I.; Etter, M.; Evans, J.D.; Borchardt, L. Direct Mechanocatalysis: Palladium as Milling Media and Catalyst in the Mechanochemical Suzuki Polymerization. Angew. Chem., Int. Ed. 2019, 58 (52), 18942–18947.
- Da Como, E.; Becker, K.; Lupton, J.M. Single Molecule Spectroscopy of Polyfluorenes. Polyfluorenes 2008, 212, 293–318.
- Scherf, U.; List, E.J. Semiconducting Polyfluorenes—Towards Reliable Structure-Property Relationships. Adv. Mater. 2002, 14 (7), 477–487.
- Grimsdale, A.C.; Müllen, K. Polyphenylene-type Emissive Materials: Poly (Para- Phenylene) s, Polyfluorenes, and Ladder Polymers. Adv Polym Sci. 2006, 199, 1–82.
- Liu, B.; Yu, W.-L.; Lai, Y.-H.; Huang, W. Blue-light-emitting Fluorene-Based Polymers with Tunable Electronic Properties. Chem. Mater. 2001, 13 (6), 1984–1991.
- Abbel, R.; Schenning, A.P.; Meijer, E. Fluorene-based Materials and their Supramolecular Properties. J. Polym. Sci., Part A: Polym. Chem. 2009, 47 (17), 4215–4233.
- Chaudhry, M.U.; Muhieddine, K.; Wawrzinek, R.; Sobus, J.; Tandy, K.; Lo, S.C.; Namdas, E.B. Organic Light-emitting Transistors: Advances and Perspectives. Adv. Funct. Mater. 2020, 30 (20), 1905282.
- Leclerc, M. Polyfluorenes: Twenty Years of Progress. J. Polym. Sci., Part A: Polym. Chem. 2001, 39 (17), 2867–2873.
- Van Aken, K.; Strekowski, L.; Patiny, L. EcoScale, a Semi-quantitative Tool to Select an Organic Preparation Based on Economical and Ecological Parameters. Beilstein J. Org. Chem. 2006, 2 (1), 3.
- Herguth, P.; Jiang, X.; Liu, M.S.; Jen, A.K.-Y. Highly Efficient Fluorene-and Benzothiadiazole-Based Conjugated Copolymers for Polymer Light-Emitting Diodes. Macromolecules 2002, 35 (16), 6094–6100.
- Wu, H.; Huang, F.; Mo, Y.; Yang, W.; Wang, D.; Peng, J.; Cao, Y. Efficient Electron Injection from a Bilayer Cathode Consisting of Aluminum and Alcohol-/Water-Soluble Conjugated Polymers. Adv. Mater. 2004, 16 (20), 1826–1830.
- Huang, F.; Wu, H.; Wang, D.; Yang, W.; Cao, Y. Novel Electroluminescent Conjugated Polyelectrolytes Based on Polyfluorene. Chem. Mater. 2004, 16 (4), 708–716.
- Andersen, J.M.; Mack, J. Decoupling the Arrhenius Equation via Mechanochemistry. Chem. Sci. 2017, 8, 5447–5453.
- Seo, T.; Toyoshima, N.; Kubota, K.; Ito, H. Tackling Solubility Issues Inorganic Synthesis: Solid-State Cross-coupling of Insoluble Aryl Halides. J. Am. Chem. Soc. 2021, 143, 6165–6175.
- Takahashi, R.; Hu, A.; Gao, P.; Gao, Y.; Pang, Y.; Seo1, T.; Jiang, J.; Maeda, S.; Takaya, H.; Kubota, K.; Ito, H. Mechanochemical Synthesis of Magnesium-Basedcarbon Nucleophiles in Air and their Use in Organic Synthesis. Nat. Commun. 2021, 12, 1–10.