ABSTRACT
A convenient and efficient [3 + 2] cycloaddition reaction of alkenes and alkynes with the in situ generated nitrile oxides from aldoximes in the presence of NaCl, Oxone and Na2CO3 has been developed to afford a series of isoxazoles and isoxazolines at room temperature in yields up to 85% and 86%, respectively. The current methodology exhibits a wide substrate scope, (hetero)aromatic and aliphatic aldoximes, alkenes including acrylate esters, acrylonitrile, chalcone, styrene, N-methylmaleimide and [60]fullerene, phenylacetylene, and alkynes containing CH2OH, SiMe3, COCH3 or CO2CH3 group can be employed. The present protocol features good functional group tolerance, short reaction time, mild reaction conditions and high atom economy, providing an efficient and environmentally friendly access to isoxazoles and isoxazolines.
1. Introduction
As a consequence of the huge potential applications in environmental protection and waste reduction, mechanochemistry has continued to capture the interest of chemists worldwide (Citation1–8). The ball-milling technique as an attractive and efficient mechanochemical methodology has advantages in increasing reaction yields, shortening reaction time and improving reaction selectivity. In addition, the mechanochemical protocols are suitable for substrates with poor solubility and can even alter the chemical selectivity, providing products which are difficult or impossible to access in the liquid-phase reactions (Citation9–14). Therefore, the ball-milling technique has been extensively utilized in organic synthesis (Citation15–19).
Isoxazoles and isoxazolines are important structures in organic chemistry and pharmaceutical chemistry, which widely exist in various natural compounds and synthetic drugs (Citation20–22). They often display versatile pharmacological activities, such as anti-cancer and anti-tumor activities (Citation23–24), antibacterial activities (Citation25), analgesic and anti-inflammatory activities (Citation26), and so on (Citation27–28). On the other hand, they are also important intermediates in organic synthesis and important precursors for the synthesis of β-hydroxyl ketones, β-amino acids and γ-amino alcohols (Citation29–34). Given the widespread utility of isoxazoles and isoxazolines, it is not surprising that a variety of methods have been developed for their synthesis. The [3 + 2] cycloaddition of nitrile oxides with various alkenes and alkynes is an important strategy to synthesize isoxazoles and isoxazolines. The formation of nitrile oxides relies heavily on oxidation of aldoximes. In the past decades, quite a number of oxidants have been reported (Citation35–42). However, most oxidants either require complex preparation or produce toxic byproducts. Therefore, the development of convenient and green strategies toward isoxazoles and isoxazolines is highly desirable.
Oxone (2KHSO5·KHSO4·K2SO4) is a stable, environmentally friendly, non-toxic oxidant, which has been used in mechanochemical reactions (Citation43–47). In our previous work, we reported that the dimerization of nitrile oxides generated in situ from aldoximes in the presence of Oxone, NaCl and base under ball-milling conditions could afford furoxans in high yields (a) (Citation47). Inspired by this result, we envisioned that the addition of alkenes and alkynes to the in situ generated nitrile oxides may compete with the dimerization process and lead to isoxazoles and isoxazolines. Herein, we report the details for the mechanosynthesis of these [3 + 2] cycloaddition products under ball-milling conditions (b). We have found that various (hetero)aromatic and aliphatic aldoximes, diverse alkenes such as acrylate esters, acrylonitrile, chalcone, styrene, N-methylmaleimide and [60]fullerene, different alkynes including simple phenylacetylene and those containing functional group CH2OH, SiMe3, COCH3 or CO2CH3 can be utilized to generate the [3 + 2] cycloadducts in good yields.
2. Results and discussion
Initially, we chose (E)-4-methylbenzaldehyde oxime (1a) and methyl acrylate (2a) as the model substrates to screen the optimal reaction conditions. At first, a mixture of 1a (0.1 mmol), 2a (0.1 mmol), NaCl (0.1 mmol), Oxone (0.1 mmol) and NEt3 (0.1 mmol) together with four stainless-steel balls (5 mm in diameter) was introduced into a stainless-steel jar (5 mL) and milled vigorously (30 Hz) in a Retsch MM400 mixer mill (Retsch GmbH, Haan, Germany) at room temperature for 30 min. As a result, the desired product, i.e. methyl 3-(p-tolyl)-4,5-dihydroisoxazole-5-carboxylate 3aa, was obtained in 28% yield along with the undesired furoxan product in 33% yield (, entry 1). Encouraged by this initial result, we tried to replace the base NEt3. To our delight, when Na2CO3 was employed, 3aa could be obtained in 54% yield along with the minor byproduct furoxan in 7% yield (, entry 2), indicating that Na2CO3 was more productive for this [3 + 2] cycloaddition reaction than NEt3. Increasing the amount of 2a from 1.0 to 1.2 equiv. resulted in a slightly higher yield of 59%, yet further increasing to 1.5 equiv. could not give a better product yield although the byproduct furoxan was formed in a trace amount (, entries 3 and 4). Similarly, when the amount of Na2CO3 was increased from 1.0 to 1.2 equiv. and 1.5 equiv., 3aa was obtained in 62% and 69% yields, respectively, whereas further increasing to 2.0 equiv. could not provide a higher yield (Table 1, entries 5−7). The amount of NaCl and Oxone was subsequently optimized, and it was found that when the dosage of both NaCl and Oxone was increased from 1.0 to 1.1 equiv., the yield could be increased to 75% (, entries 8−11). Then, the reaction time was investigated. As the reaction time was extended from 30 min to 60 min, product 3aa was isolated in 85% yield (, entry 12). Further extending the reaction time to 90 min led to a slightly decreased yield of 84% (, entry 13). The liquid-assisted grinding (LAG) protocol has acted as a power tool to promote mechanochemical reactions (Citation48–51). Accordingly, several liquids such as ethyl alcohol (EtOH), dichloromethane (DCM), ethyl acetate (EtOAc) and acetonitrile (CH3CN) were added to the reaction mixture. However, it was found that the addition of LAG was detrimental to this reaction (, entries 14−17). Therefore, the optimized reaction conditions of the [3 + 2] cycloaddition reaction were established as follows: 0.1 mmol 1a, 1.2 equiv. 2a, 1.1 equiv. NaCl, 1.1 equiv. Oxone and 1.5 equiv. Na2CO3 at 30 Hz for 60 min (, entry 12).
Table 1. Optimization of the reaction conditions Table Footnotea
Having established the optimal reaction conditions, the substrate scope of alkenes and alkynes were investigated, and the results are shown in . Firstly, tert-butyl acrylate, acrylonitrile and chalcone, of which alkenes bearing electron-withdrawing groups, reacted smoothly with 1a to provide products 3ab, 3ac and 3ad in 82%, 72% and 66% yields, respectively. It should be pointed out that 3ad consisted of two regioisomers 3ad’ and 3ad'’ with isolated yields of 37% and 29%, respectively. Styrene 2e also worked well in this reaction system, and afforded the corresponding product 3ae in 67% yield. To our satisfaction, N-methylmaleimide 2f was also compatible under the standard reaction conditions, and the desired product 3af was isolated in 77% yield. What is more, the cycloaddition reaction could also be expanded to alkynes and provide the corresponding isoxazoles in moderate to good yields. Phenylacetylene 2g gave the corresponding product 3ag in 83% yield. Alkynes bearing CH2OH and SiMe3 groups 2h and 2i proceeded efficiently to provide products 3ah and 3ai in 65% and 70% yields, respectively. The keto-substituted alkyne 2j could be used to give 3aj in 78% yield. The diester-substituted alkyne 2k proved to be feasible for this procedure and afforded product 3ak in 79% yield. Gratifyingly, [60]fullerene (C60), which behaves as an electron-deficient polyolefin, could also be employed and provided 3al in 36% yield (the yield based on consumed C60 was 45%). The structures of products were unambiguously confirmed by single-crystal X-ray diffraction analysis with 3aa as an example (see the Supplementary Materials for details).
Scheme 2. Reaction scope of alkenes and alkynes 2a–l.a,b a Unless otherwise stated, the reactions were performed in a stainless-steel jar (5 mL) with 1a (0.1 mmol), NaCl (0.11 mmol), Oxone (0.11 mmol), Na2CO3 (0.15 mmol) and 2a–l (0.12 mmol) together with 4 stainless-steel balls (5 mm in diameter) by using a Retsch MM400 mixer mill at 30 Hz for 60 min. b Isolated yields based on 1a. c 1a (0.1 mmol), NaCl (0.11 mmol), Oxone (0.11 mmol), Na2CO3 (0.15 mmol), 2l (0.05 mmol) and isolated yield based on C60. d Isolated yield based on consumed C60.
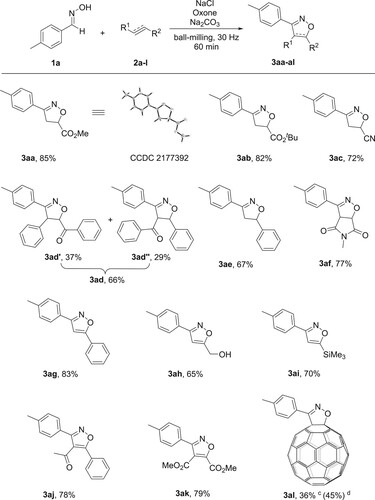
To further investigate the substrate generality, we explored the substrate scope of aldoximes. As shown in , different kinds of aldoximes were well tolerated under the optimal reaction conditions. For instance, when aldoxime 1b with the para-substituted electron-donating methoxy group was used, product 3ba was obtained in 76% yield. The substrates 1c and 1d with para-substituted electron-withdrawing groups (Br, CO2Me) gave the corresponding products 3ca and 3da in 80% and 83% yields, respectively. Similarly, 1-naphthaldehyde oxime also proved to be feasible for this procedure and provided product 3ea in 76% yield. Satisfactorily, 3-phenylpropanal oxime 1f and conjugate aldoxime 1g were also suitable substrates for this transformation, affording the corresponding products 3fa and 3ga in 82% and 69% yields. Moreover, the heteroaromatic aldoximes 3h−j could be used under our oxidation conditions for the [3 + 2] cycloaddition. Nicotinaldehyde oxime 1h, thiophene-2-carbaldehyde oxime 1i and benzofuran-2-carbaldehyde oxime 1j afforded products 3ha, 3ia and 3ja in 75%, 70% and 86% yields, respectively.
Scheme 3. Reaction scope of aldoximes 1b–j.a,b a Unless otherwise stated, the reactions were performed in a stainless-steel jar (5 mL) with 1b–j (0.1 mmol), NaCl (0.11 mmol), Oxone (0.11 mmol), Na2CO3 (0.15 mmol) and 2a (0.12 mmol) together with 4 stainless-steel balls (5 mm in diameter) by using a Retsch MM400 mixer mill at 30 Hz for 60 min. b Isolated yields based on 1b–j.
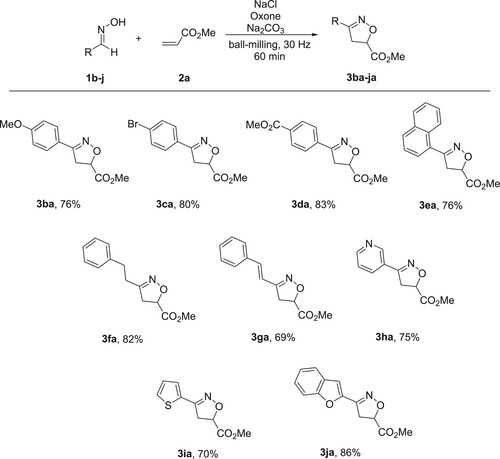
To further evaluate the practicability of the present mechanochemical protocol, a scale-up reaction of aldoxime 1a (6 mmol) with 2a (7.2 mmol) was carried out under the optimal reaction conditions (). To our delight, product 3aa was obtained in a satisfactory yield of 74% (0.97 g), which indicated that the present method could be easily adopted for a larger-scale preparation.
In order to assess the greenness of this mechanochemical process, complete and simple environmental factors (cEF and sEF), atom economy (AE) and reaction mass efficiency (RME) for the mechanochemical synthesis of 3aa were quantified, showing advantages in greenness compared with the liquid-phase counterpart (see the Supplementary Materials for details).
3. Conclusions
In summary, we have successfully developed the mechanochemical solvent-free reactions of alkenes and alkynes with the in situ generated nitrile oxides from aldoximes NaCl, Oxone and Na2CO3, affording isoxazoles and isoxazolines at room temperature in yields up to 85% and 86%, respectively. In the current protocol, (hetero)aromatic and aliphatic aldoximes, alkenes including acrylate esters, acrylonitrile, chalcone, styrene, N-methylmaleimide and [60]fullerene, phenylacetylene, and alkynes containing CH2OH, SiMe3, COCH3 or CO2CH3 group can be utilized. This process provides an efficient and environmentally friendly access to a variety of isoxazole and isoxazoline derivatives with remarkable functional group compatibility, short reaction time and good yields. We expect that this efficient method will be of great interest to organic and drug synthesis.
4. Materials and methods
4.1. General information
All reagents were obtained from commercial sources and used without further purification. NMR spectra were recorded on a Bruker Advance III HD 400 NMR spectrometer (Bruker BioSpin AG, Fällanden, Switzerland; 400 MHz for 1H NMR; 101 MHz for 13C NMR) and a Bruker Advance III HD 500 NMR spectrometer (Bruker BioSpin AG, Fällanden, Switzerland; 500 MHz for 1H NMR; 126 MHz for 13C NMR). 1H NMR chemical shifts were determined relative to TMS at 0.00 ppm or CDCl3 at δ 7.26 ppm. 13C NMR chemical shifts were determined relative to TMS at 0.00 ppm or CDCl3 at δ 77.08 ppm. Data for 1H NMR and 13C NMR are reported as follows: chemical shift (δ, ppm), multiplicity (s = singlet, d = doublet, t = triplet, m = multiplet). High-resolution mass spectra (HRMS) were taken on a Waters Acquity UPLC-Xevo G2 QTof mass spectrometer (Waters, Milford, MA, USA) with FTMS-ESI in positive mode. Ball-milling reactions were performed in a MM400 mixer mill (Retsch GmbH, Haan, Germany), using a 5 mL stainless-steel jar with four 5 mm diameter stainless-steel balls and were milled at a frequency of 1800 rounds per minute (30 Hz) at room temperature. Aldoximes 1 were prepared according to the reported protocol (Citation52).
4.2 Mechanochemical synthesis and characterization of products 3aa–al and 3ba–ja
A mixture of aldoximes 1a–l (0.1 mmol), NaCl (0.11 mmol), Oxone (0.11 mmol), Na2CO3 (0.15 mmol) and alkenes or alkynes (0.12 mmol) together with four stainless-steel balls (5 mm in diameter) was introduced into a stainless-steel jar (5 mL). The reaction vessel along with another identical vessel was closed and fixed on the vibration arms of a Retsch MM400 mixer mill, and was vibrated at a rate of 1800 rounds per minute (30 Hz) at room temperature for 60 min. After completion of the reaction, the resulting mixtures from the two runs were combined by washing with ethyl acetate. Then, the reaction mixture was filtered through a silica gel plug with ethyl acetate as the eluent and evaporated in a vacuum. The residue was separated by flash column chromatography on silica gel with ethyl acetate/petroleum ether as the eluent to afford products 3aa–al and 3ba–ja.
Methyl 3-(p-tolyl)-4,5-dihydroisoxazole-5-carboxylate (3aa). By following the general procedure, the reaction of 1a (27.2 mg, 0.2 mmol) with NaCl (14.1 mg, 0.22 mmol), Oxone (140.3 mg, 0.22 mmol), Na2CO3 (31.9 mg, 0.3 mmol) and 2a (22 μL, 0.24 mmol) afforded 3aa (37.5 mg, 85% yield). White solid; 1H NMR (500 MHz, CDCl3) δ 7.57 (d, J = 7.8 Hz, 2H), 7.22 (d, J = 7.8 Hz, 2H), 5.18 (t, J = 9.1 Hz, 1H), 3.81 (s, 3H), 3.70−3.57 (m, 2H), 2.38 (s, 3H); 13C NMR (126 MHz, CDCl3) δ 170.9, 156.1, 140.9, 129.5 (2C), 126.9 (2C), 125.7, 77.8, 52.9, 39.1, 21.5. The NMR data agreed with those in a literature report (Citation53).
Tert-butyl 3-(p-tolyl)-4,5-dihydroisoxazole-5-carboxylate (3ab). By following the general procedure, the reaction of 1a (27.5 mg, 0.2 mmol) with NaCl (14.2 mg, 0.22 mmol), Oxone (139.3 mg, 0.22 mmol), Na2CO3 (33.2 mg, 0.3 mmol) and 2b (36 μL, 0.24 mmol) afforded 3ab (43.8 mg, 82% yield). White solid; 1H NMR (400 MHz, CDCl3) δ 7.57 (d, J = 8.0 Hz, 2H), 7.21 (d, J = 8.0 Hz, 2H), 5.03 (t, J = 9.3 Hz, 1H), 3.57 (d, J = 9.3 Hz, 2H), 2.38 (s, 3H), 1.50 (s, 9H); 13C NMR (101 MHz, CDCl3) δ 169.3, 155.8, 140.7, 129.5 (2C), 126.9 (2C), 126.0, 82.7, 78.6, 38.9, 28.0 (3C), 21.5. The NMR data agreed with those in a literature report (Citation54).
3-(p-Tolyl)-4,5-dihydroisoxazole-5-carbonitrile (3ac). By following the general procedure, the reaction of 1a (27.2 mg, 0.2 mmol) with NaCl (14.3 mg, 0.22 mmol), Oxone (140.5 mg, 0.22 mmol), Na2CO3 (35.3 mg, 0.3 mmol) and 2c (16 μL, 0.24 mmol) afforded 3ac (27.0 mg, 72% yield). White solid; 1H NMR (400 MHz, CDCl3) δ 7.55 (d, J = 8.2 Hz, 2H), 7.25 (d, J = 8.2 Hz, 2H), 5.35 (dd, J = 10.5, 6.4 Hz, 1H), 3.76 (dd, J = 16.7, 10.5 Hz, 1H), 3.69 (dd, J = 16.7, 6.4 Hz, 1H), 2.40 (s, 3H); 13C NMR (101 MHz, CDCl3) δ 156.3, 141.8, 129.8 (2C), 127.0 (2C), 124.5, 117.2, 66.4, 41.3, 21.6. The NMR data agreed with those in a literature report (Citation55).
Phenyl(4-phenyl-3-(p-tolyl)-4,5-dihydroisoxazol-5-yl)methanone (3ad). By following the general procedure, the reaction of 1a (27.5 mg, 0.2 mmol) with NaCl (13.1 mg, 0.22 mmol), Oxone (136.4 mg, 0.22 mmol), Na2CO3 (32.6 mg, 0.3 mmol) and 2d (50.9 mg, 0.24 mmol) afforded regioisomers 3ad’ (25.7 mg, 37% yield) and 3ad'’ (20.1 mg, 29% yield). 3ad': White solid; 1H NMR (400 MHz, CDCl3) δ 8.05 (d, J = 8.0 Hz, 2H), 7.61 (t, J = 7.4 Hz, 1H), 7.55−7.45 (m, 4H), 7.39−7.26 (m, 5H), 7.07 (d, J = 8.0 Hz, 2H), 5.67 (d, J = 4.8 Hz, 1H), 5.42 (d, J = 4.8 Hz, 1H), 2.29 (s, 3H); 13C NMR (101 MHz, CDCl3) δ 193.6, 158.5, 140.5, 138.4, 134.2, 134.0, 129.7 (2C), 129.5 (2C), 129.4 (2C), 128.8 (2C), 128.0, 127.9 (2C), 127.6 (2C), 125.2, 89.9, 55.3, 21.5; HRMS (FTMS-ESI) Calcd for C23H20NO2 [M + H]+ 342.1489; found 342.1487. 3ad'': White solid; 1H NMR (400 MHz, CDCl3) δ 7.91 (dd, J = 8.0, 1.3 Hz, 2H), 7.64 (t, J = 7.4 Hz, 1H), 7.53−7.44 (m, 4H), 7.43−7.34 (m, 5H), 7.12 (d, J = 8.0 Hz, 2H), 5.74 (d, J = 6.7 Hz, 1H), 5.37 (d, J = 6.7 Hz, 1H), 2.32 (s, 3H); 13C NMR (101 MHz, CDCl3) δ 195.5, 155.4, 140.6, 139.8, 135.2, 134.4, 129.6 (2C), 129.2 (2C), 129.1 (2C), 129.02 (2C), 128.95, 127.0 (2C), 125.9 (2C), 125.7, 87.5, 65.0, 21.5; HRMS (FTMS-ESI) Calcd for C23H20NO2 [M + H]+ 342.1489; found 342.1488.
5-Phenyl-3-(p-tolyl)-4,5-dihydroisoxazole (3ae). By following the general procedure, the reaction of 1a (27.1 mg, 0.2 mmol) with NaCl (14.1 mg, 0.22 mmol), Oxone (140.7 mg, 0.22 mmol), Na2CO3 (33.3 mg, 0.3 mmol) and 2e (28 μL, 0.24 mmol) afforded 3ae (31.7 mg, 67% yield). White solid; 1H NMR (400 MHz, CDCl3) δ 7.58 (d, J = 8.2 Hz, 2H), 7.41−7.28 (m, 5H), 7.21 (d, J = 8.2 Hz, 2H), 5.71 (dd, J = 11.0, 8.2 Hz, 1H), 3.75 (dd, J = 16.6, 11.0 Hz, 1H), 3.32 (dd, J = 16.6, 8.2 Hz, 1H), 2.37 (s, 3H); 13C NMR (101 MHz, CDCl3) δ 156.1, 141.1, 140.4, 129.5 (2C), 128.8 (2C), 128.2, 126.73 (2C), 126.68, 125.9 (2C), 82.4, 43.3, 21.5. The NMR data agreed with those in a literature report (Citation56).
5-Methyl-3-(p-tolyl)-3a,6a-dihydro-4H-pyrrolo[3,4-d]isoxazole-4,6(5H)-dione (3af). By following the general procedure, the reaction of 1a (27.3 mg, 0.2 mmol) with NaCl (14.5 mg, 0.22 mmol), Oxone (141.7 mg, 0.22 mmol), Na2CO3 (33.5 mg, 0.3 mmol) and 2f (27.8 mg, 0.24 mmol) afforded 3af (38.0 mg, 77% yield). White solid; 1H NMR (400 MHz, CDCl3) δ 7.85 (d, J = 8.3 Hz, 2H), 7.25 (d, J = 8.3 Hz, 2H), 5.49 (d, J = 9.5 Hz, 1H), 4.80 (d, J = 9.5 Hz, 1H), 3.00 (s, 3H), 2.39 (s, 3H); 13C NMR (101 MHz, CDCl3) δ 172.1, 171.0, 152.7, 141.7, 129.5 (2C), 127.9 (2C), 123.9, 80.3, 55.0, 25.6, 21.5; HRMS (FTMS-ESI) Calcd for C13H13N2O3 [M + H]+ 245.0921; found 245.0931.
5-Phenyl-3-(p-tolyl)isoxazole (3ag). By following the general procedure, the reaction of 1a (27.2 mg, 0.2 mmol) with NaCl (14.6 mg, 0.22 mmol), Oxone (141.8 mg, 0.22 mmol), Na2CO3 (31.8 mg, 0.3 mmol) and 2g (26 µL, 0.24 mmol) afforded 3ag (39.3 mg, 83% yield). White solid; 1H NMR (400 MHz, CDCl3) δ 7.83 (dd, J = 8.2, 1.8 Hz, 2H), 7.76 (d, J = 8.2 Hz, 2H), 7.51−7.42 (m, 3H), 7.28 (d, J = 8.2 Hz, 2H), 6.80 (s, 1H), 2.41 (s, 3H); 13C NMR (101 MHz, CDCl3) δ 170.3, 163.0, 140.2, 130.2, 129.7 (2C), 129.0 (2C), 127.6, 126.7 (2C), 126.3, 125.9 (2C), 97.5, 21.5. The NMR data agreed with those in a literature report (Citation57).
(3-(p-Tolyl)isoxazol-5-yl)methanol (3ah). By following the general procedure, the reaction of 1a (27.1 mg, 0.2 mmol) with NaCl (15.4 mg, 0.22 mmol), Oxone (140.5 mg, 0.22 mmol), Na2CO3 (33.6 mg, 0.3 mmol) and 2h (14 µL, 0.24 mmol) afforded 3ah (24.6 mg, 65% yield). White solid; 1H NMR (400 MHz, CDCl3) δ 7.67 (d, J = 8.2 Hz, 2H), 7.25 (d, J = 8.2 Hz, 2H), 6.53 (s, 1H), 4.80 (s, 2H), 2.39 (s, 3H); 13C NMR (101 MHz, CDCl3) δ 171.7, 162.5, 140.3, 129.6 (2C), 126.7 (2C), 125.9, 100.0, 56.6, 21.4. The NMR data agreed with those in a literature report (Citation58).
3-(p-Tolyl)-5-(trimethylsilyl)isoxazole (3ai). By following the general procedure, the reaction of 1a (27.4 mg, 0.2 mmol) with NaCl (14.7 mg, 0.22 mmol), Oxone (139.4 mg, 0.22 mmol), Na2CO3 (33.8 mg, 0.3 mmol) and 2i (34 µL, 0.24 mmol) afforded 3ai (32.8 mg, 70% yield). White solid; 1H NMR (500 MHz, CDCl3) δ 7.72 (d, J = 8.1 Hz, 2H), 7.25 (d, J = 8.1 Hz, 2H), 6.72 (s, 1H), 2.39 (s, 3H), 0.38 (s, 9H); 13C NMR (126 MHz, CDCl3) δ 178.6, 160.8, 139.8, 129.6 (2C), 127.0 (2C), 126.4, 110.7, 21.5, −1.8 (3C); HRMS (FTMS-ESI) Calcd for C13H18NOSi [M + H]+ 232.1152; found 232.1160.
1-(5-Phenyl-3-(p-tolyl)isoxazol-4-yl)ethan-1-one (3aj). By following the general procedure, the reaction of 1a (27.3 mg, 0.2 mmol) with NaCl (13.9 mg, 0.22 mmol), Oxone (137.5 mg, 0.22 mmol), Na2CO3 (32.2 mg, 0.3 mmol) and 2j (36 µL, 0.24 mmol) afforded 3aj (43.6 mg, 78% yield). White solid; 1H NMR (500 MHz, CDCl3) δ 7.85 (dd, J = 8.0, 1.6 Hz, 2H), 7.59−7.48 (m, 5H), 7.31 (d, J = 8.0 Hz, 2H), 2.43 (s, 3H), 2.20 (s, 3H); 13C NMR (126 MHz, CDCl3) δ 195.7, 170.2, 162.2, 140.5, 131.4, 129.6 (2C), 128.9 (2C), 128.7 (2C), 128.5 (2C), 127.0, 125.5, 117.3, 31.7, 21.5. HRMS (FTMS-ESI) Calcd for C18H16NO2 [M + H]+ 278.1176; found 278.1182.Dimethyl 3-(p-tolyl)isoxazole-4,5-dicarboxylate (3ak). By following the general procedure, the reaction of 1a (27.3 mg, 0.2 mmol) with NaCl (14.2 mg, 0.22 mmol), Oxone (140.7 mg, 0.22 mmol), Na2CO3 (34.9 mg, 0.3 mmol) and 2k (30 µL, 0.24 mmol) afforded 3ak (43.9 mg, 79% yield). Yellow solid; 1H NMR (400 MHz, CDCl3) δ 7.58 (d, J = 8.2 Hz, 2H), 7.27 (d, J = 8.2 Hz, 2H), 4.01 (s, 3H), 3.90 (s, 3H), 2.41 (s, 3H); 13C NMR (101 MHz, CDCl3) δ 162.0, 161.3, 159.2, 156.6, 141.1, 129.7 (2C), 128.0 (2C), 124.0, 116.1, 53.4, 53.2, 21.5. The NMR data agreed with those in a literature report (Citation59).
3-(p-Tolyl)-[60]fullereno[1′,2′:4,5]isoxazoline (3al). By following the general procedure, the reaction of 1a (27.1 mg, 0.2 mmol) with NaCl (15.1 mg, 0.22 mmol), Oxone (139.9 mg, 0.22 mmol), Na2CO3 (34.2 mg, 0.3 mmol) and 2l (72.5 mg, 0.1 mmol) afforded recovered C60 (14.4 mg, 20%) and 3al (31.0 mg, 36% yield). Amorphous brown solid; 1H NMR (400 MHz, 1/1 CS2/CDCl3) δ 8.02 (d, J = 8.1 Hz, 2H), 7.29 (d, J = 8.1 Hz, 2H), 2.43 (s, 3H); 13C NMR (101 MHz, 1/1 CS2/CDCl3) δ 152.9 (C = N), 147.6, 147.1, 146.2 (2C), 146.07 (2C), 146.05 (2C), 145.8 (2C), 145.7 (4C), 145.4 (2C), 145.2 (2C), 145.0 (2C), 144.9 (2C), 144.7 (2C), 144.6 (2C), 144.4 (2C), 144.2 (2C), 143.9 (2C), 142.8 (2C), 142.7 (4C), 142.3 (4C), 142.2 (2C), 142.1 (2C), 141.9 (2C), 141.5 (2C), 140.6 (aryl C), 140.12 (2C), 140.11 (2C), 136.8 (2C), 136.5 (2C), 129.6 (aryl C, 2C), 128.7 (aryl C, 2C), 126.1 (aryl C), 103.8 (sp3-C of C60), 79.1 (sp3-C of C60), 21.6; MALDI-TOF MS m/z calcd for C68H7NO [M]- 853.0522, found 853.0526.
Methyl 3-(4-methoxyphenyl)-4,5-dihydroisoxazole-5-carboxylate (3ba). By following the general procedure, the reaction of 1b (30.4 mg, 0.2 mmol) with NaCl (14.6 mg, 0.22 mmol), Oxone (140.4 mg, 0.22 mmol), Na2CO3 (31.4 mg, 0.3 mmol) and 2a (22 µL, 0.24 mmol) afforded 3ba (36.0 mg, 76% yield). Yellow solid; 1H NMR (400 MHz, CDCl3) δ 7.62 (d, J = 8.9 Hz, 2H), 6.92 (d, J = 8.9 Hz, 2H), 5.17 (dd, J = 10.5, 7.6 Hz, 1H), 3.84 (s, 3H), 3.82 (s, 3H), 3.69−3.55 (m, 2H); 13C NMR (101 MHz, CDCl3) δ 171.0, 161.4, 155.7, 128.6 (2C), 121.0, 114.2 (2C), 77.8, 55.4, 52.9, 39.2. The NMR data agreed with those in a literature report (Citation53).
Methyl 3-(4-bromophenyl)-4,5-dihydroisoxazole-5-carboxylate (3ca). By following the general procedure, the reaction of 1c (40.7 mg, 0.2 mmol) with NaCl (14.1 mg, 0.22 mmol), Oxone (141.4 mg, 0.22 mmol), Na2CO3 (33.5 mg, 0.3 mmol) and 2a (22 µL, 0.24 mmol) afforded 3ca (46.2 mg, 80% yield). White solid; 1H NMR (500 MHz, CDCl3) δ 7.54 (s, 4H), 5.21 (t, J = 9.1 Hz, 1H), 3.82 (s, 3H), 3.71−3.54 (m, 2H); 13C NMR (126 MHz, CDCl3) δ 170.5, 155.3, 132.1 (2C), 128.4 (2C), 127.4, 124.9, 78.2, 53.0, 38.6; HRMS (FTMS-ESI) Calcd for C11H1079BrNO3Na [M + Na]+ 305.9736; found 305.9733.
Methyl 3-(4-(methoxycarbonyl)phenyl)-4,5-dihydroisoxazole-5-carboxylate (3da). By following the general procedure, the reaction of 1d (36.2 mg, 0.2 mmol) with NaCl (14.2 mg, 0.22 mmol), Oxone (141.5 mg, 0.22 mmol), Na2CO3 (32.8 mg, 0.3 mmol) and 2a (22 µL, 0.24 mmol) afforded 3da (44.1 mg, 83% yield). White solid; 1H NMR (500 MHz, CDCl3) δ 8.08 (d, J = 6.6 Hz, 2H), 7.75 (d, J = 6.6 Hz, 2H), 5.24 (dd, J = 10.4, 8.0 Hz, 1H), 3.94 (s, 3H), 3.83 (s, 3H), 3.74−3.62 (m, 2H); 13C NMR (126 MHz, CDCl3) δ 170.5, 166.4, 155.5, 132.6, 131.8, 130.0 (2C), 126.9 (2C), 78.4, 53.0, 52.4, 38.6; HRMS (FTMS-ESI) Calcd for C13H14NO5 [M + H]+ 264.0866; found 264.0863.
Methyl 3-(naphthalen-1-yl)-4,5-dihydroisoxazole-5-carboxylate (3ea). By following the general procedure, the reaction of 1e (35.0 mg, 0.2 mmol) with NaCl (14.8 mg, 0.22 mmol), Oxone (141.3 mg, 0.22 mmol), Na2CO3 (32.1 mg, 0.3 mmol) and 2a (22 µL, 0.24 mmol) afforded 3ea (38.6 mg, 76% yield). Yellow solid; 1H NMR (500 MHz, CDCl3) δ 7.97 (d, J = 8.6 Hz, 1H), 7.92 (s, 1H), 7.90−7.79 (m, 3H), 7.61−7.45 (m, 2H), 5.25 (dd, J = 10.3, 7.5 Hz, 1H), 3.83 (s, 3H), 3.82−3.69 (m, 2H); 13C NMR (126 MHz, CDCl3) δ 170.8, 156.3, 134.2, 132.9, 128.7, 128.5, 127.9, 127.42, 127.41, 126.8, 126.1, 123.6, 78.1, 53.0, 38.9; HRMS (FTMS-ESI) Calcd for C15H13NO3Na [M + Na]+ 278.0788; found 278.0795.
Methyl 3-phenethyl-4,5-dihydroisoxazole-5-carboxylate (3fa). By following the general procedure, the reaction of 1f (30.1 mg, 0.2 mmol) with NaCl (15.7 mg, 0.22 mmol), Oxone (140.5 mg, 0.22 mmol), Na2CO3 (32.6 mg, 0.3 mmol) and 2a (22 µL, 0.24 mmol) afforded 3fa (38.6 mg, 82% yield). Yellow solid; 1H NMR (500 MHz, CDCl3) δ 7.31 (t, J = 7.3 Hz, 2H), 7.26−7.14 (m, 3H), 4.97 (t, J = 9.0 Hz, 1H), 3.78 (s, 3H), 3.16 (d, J = 8.9 Hz, 2H), 2.93 (t, J = 8.1 Hz, 2H), 2.69 (t, J = 8.0 Hz, 2H); 13C NMR (126 MHz, CDCl3) δ 171.0, 157.9, 140.3, 128.7 (2C), 128.3 (2C), 126.5, 76.9, 52.8, 41.3, 32.6, 29.1; HRMS (FTMS-ESI) Calcd for C13H15NO3Na [M + Na]+ 256.0944; found 256.0947.
Methyl (E)-3-styryl-4,5-dihydroisoxazole-5-carboxylate (3ga). By following the general procedure, the reaction of 1g (30.2 mg, 0.2 mmol) with NaCl (14.4 mg, 0.22 mmol), Oxone (139.7 mg, 0.22 mmol), Na2CO3 (32.3 mg, 0.3 mmol) and 2a (22 µL, 0.24 mmol) afforded 3ga (32.7 mg, 69% yield). White solid; 1H NMR (500 MHz, CDCl3) δ 7.47 (d, J = 7.5 Hz, 2H), 7.41−7.30 (m, 3H), 7.07 (d, J = 16.5 Hz, 1H), 6.79 (d, J = 16.5 Hz, 1H), 5.15 (dd, J = 10.8, 7.4 Hz, 1H), 3.82 (s, 3H), 3.61−3.42 (m, 2H); 13C NMR (126 MHz, CDCl3) δ 170.6, 157.1, 137.7, 135.4, 129.3, 128.9 (2C), 127.1 (2C), 116.8, 78.0, 52.9, 37.5; HRMS (FTMS-ESI) Calcd for C13H14NO3 [M + H]+ 232.0968; found 232.0977.
Methyl 3-(pyridin-3-yl)-4,5-dihydroisoxazole-5-carboxylate (3ha). By following the general procedure, the reaction of 1h (24.9 mg, 0.2 mmol) with NaCl (15.0 mg, 0.22 mmol), Oxone (138.1 mg, 0.22 mmol), Na2CO3 (33.1 mg, 0.3 mmol) and 2a (22 µL, 0.24 mmol) afforded 3ha (31.5 mg, 75% yield). Yellow solid; 1H NMR (500 MHz, CDCl3) δ 8.84 (s, 1H), 8.68 (d, J = 4.8 Hz, 1H), 8.08 (d, J = 9.8 Hz, 1H), 7.38 (t, J = 6.1 Hz, 1H), 5.25 (dd, J = 11.2, 6.8 Hz, 1H), 3.84 (s, 3H), 3.75−3.61 (m, 2H); 13C NMR (126 MHz, CDCl3) δ 170.4, 153.9, 151.5, 148.0, 134.0, 124.8, 123.8, 78.2, 53.1, 38.3; HRMS (FTMS-ESI) Calcd for C10H11N2O3 [M + H]+ 207.0764; found 207.0768.
Methyl 3-(thiophen-2-yl)-4,5-dihydroisoxazole-5-carboxylate (3ia). By following the general procedure, the reaction of 1i (25.8 mg, 0.2 mmol) with NaCl (13.7 mg, 0.22 mmol), Oxone (142.1 mg, 0.22 mmol), Na2CO3 (32.3 mg, 0.3 mmol) and 2a (22 µL, 0.24 mmol) afforded 3ia (30.0 mg, 70% yield). Yellow oil; 1H NMR (500 MHz, CDCl3) δ 7.43 (d, J = 5.2 Hz, 1H), 7.24 (d, J = 3.7 Hz, 1H), 7.08 (t, J = 4.2 Hz, 1H), 5.19 (dd, J = 11.0, 6.9 Hz, 1H), 3.82 (s, 3H), 3.72−3.63 (m, 2H); 13C NMR (126 MHz, CDCl3) δ 170.6, 151.8, 130.8, 129.1, 129.0, 127.4, 78.1, 53.0, 39.7; HRMS (FTMS-ESI) Calcd for C9H10NO3S [M + H]+ 212.0376; found 212.0371.
Methyl 3-(2,3-dihydrobenzofuran-2-yl)-4,5-dihydroisoxazole-5-carboxylate (3ja). By following the general procedure, the reaction of 1j (32.3 mg, 0.2 mmol) with NaCl (15.0 mg, 0.22 mmol), Oxone (139.5 mg, 0.22 mmol), Na2CO3 (33.2 mg, 0.3 mmol) and 2a (22 µL, 0.24 mmol) afforded 3ja (42.1 mg, 86% yield). Yellow solid; 1H NMR (500 MHz, CDCl3) δ 7.62 (d, J = 7.8 Hz, 1H), 7.54 (d, J = 8.3 Hz, 1H), 7.38 (t, J = 7.8 Hz, 1H), 7.28 (t, J = 7.0 Hz, 1H), 7.09 (s, 1H), 5.24 (dd, J = 11.2, 6.9 Hz, 1H), 3.83 (s, 3H), 3.79−3.65 (m, 2H); 13C NMR (126 MHz, CDCl3) δ 170.2, 155.5, 148.8, 145.3, 127.6, 126.5, 123.7, 121.8, 111.8, 109.2, 78.2, 53.0, 38.5; HRMS (FTMS-ESI) Calcd for C13H11NO4Na [M + Na]+ 268.0580; found 268.0588.
4.3. Mechanochemical gram-scale synthesis of 3aa
A mixture of 1a (0.41 g, 3 mmol), NaCl (0.19 g, 3.3 mmol), Oxone (2.03 g, 3.3 mmol), Na2CO3 (0.48 g, 4.5 mmol) and 2a (0.33 mL, 3.6 mmol) together with four stainless-steel balls (7.5 mm in diameter) was introduced into a stainless-steel jar (10 mL). The reaction vessel along with another identical vessel was closed and fixed on the vibration arms of a Retsch MM400 mixer mill, and was vibrated at a rate of 1800 rounds per minute (30 Hz) at room temperature for 60 min. After completion of the reaction, the resulting mixtures from the two runs were combined by washing with ethyl acetate. Then, the reaction mixture was filtered through a silica gel plug with ethyl acetate as the eluent and evaporated in a vacuum. The residue was separated by flash column chromatography on silica gel with ethyl acetate/petroleum ether as the eluent to afford products 3aa (0.97 g, 74%).
4.4. Synthesis of 3aa in liquid phase
To a stirred solution of 1a (27.4 mg, 0.2 mmol) in CH3CN (2 mL) were added NaCl (13.9 mg, 0.22 mmol), Oxone (138.9 mg, 0.22 mmol), Na2CO3 (32.3 mg, 0.3 mmol) and 2a (22 µL, 0.24 mmol). The reaction mixture was allowed to stir at room temperature for 2 h. Then, the reaction mixture was filtered through a silica gel plug with ethyl acetate as the eluent, the solvent was subsequently removed under reduced pressure. The residue was purified by flash chromatography on silica gel with petroleum ether/ethyl acetate as eluent to give product 3aa (8.4 mg, 19% yield).
Supplemental Material
Download MS Word (9.7 MB)Disclosure statement
No potential conflict of interest was reported by the author(s).
Additional information
Funding
References
- Stolle, A.; Szuppa, T.; Leonhardt, S.E.S.; Ondruschka, B. Ball Milling in Organic Synthesis: Solutions and Challenges. Chem. Soc. Rev. 2011, 40, 2317–2329.
- James, S.L.; Adams, C.J.; Bolm, C.; Braga, D.; Collier, P.; Friščić, T.; Grepioni, F.; Harris, K.D.M.; Hyett, G.; Jones, W.; Krebs, A.; Mack, J.; Maini, L.; Orpen, A.G.; Parkin, I.P.; Shearouse, W.C.; Steed, J.W.; Waddell, D.C. Mechanochemistry: Opportunities for New and Cleaner Synthesis. Chem. Soc. Rev. 2012, 41, 413–447.
- Friščić, T. Supramolecular Concepts and New Techniques in Mechanochemistry: Cocrystals, Cages, Rotaxanes, Open Metal–Organic Frameworks. Chem. Soc. Rev. 2012, 41, 3493–3510.
- Zhu, S.-E.; Li, F.; Wang, G.-W. Mechanochemistry of Fullerenes and Related Materials. Chem. Soc. Rev. 2013, 42, 7535–7570.
- Wang, G.-W. Mechanochemical Organic Synthesis. Chem. Soc. Rev. 2013, 42, 7668–7700.
- Hernández, J.G.; Bolm, C. Altering Product Selectivity by Mechanochemistry. J. Org. Chem. 2017, 82, 4007–4019.
- Bolm, C.; Hernándezm, J.G. Mechanochemistry of Gaseous Reactants. Angew. Chem. Int. Ed. 2019, 58, 3285–3299.
- Wang, N.-N.; Wang, G.-W. Investigation Into Condensed-Matter Organic Synthesis Under Mechanical Milling Conditions. Prog. Chem. 2020, 32, 1076–1085.
- Wang, G.-W.; Komatsu, K.; Murata, Y.; Shiro, M. Synthesis and X-Ray Structure of Dumb-Bell-Shaped C120. Nature 1997, 387, 583–586.
- Wang, G.-W. Fullerene Mechanochemistry: Serendipitous Discovery of Dumb-Bell-Shaped C120 and Beyond. Chin. J. Chem. 2021, 39, 1797–1803.
- Su, Y.-T.; Wang, G.-W. FeCl3-Mediated Cyclization of [60] Fullerene with N-Benzhydryl Sulfonamides Under High-Speed Vibration Milling Conditions. Org. Lett. 2013, 15, 3408–3411.
- Zhao, Y.; Rocha, S.V.; Swager, T.M. Mechanochemical Synthesis of Extended Iptycenes. J. Am. Chem. Soc. 2016, 138, 13834–13837.
- Turberg, M.; Ardila-Fierro, K.J.; Bolm, C.; Hernández, J.G. Altering Copper-Catalyzed A3 Couplings by Mechanochemistry: One-Pot Synthesis of 1,4-Diamino-2-Butynes from Aldehydes, Amines, and Calcium Carbide. Angew. Chem. Int. Ed. 2018, 57, 10718–10722.
- Seo, T.; Ishiyama, T.; Kubota, K.; Ito, H. Solid-State Suzuki–Miyaura Cross-Coupling Reactions: Olefin-Accelerated C–C Coupling Using Mechanochemistry. Chem. Sci. 2019, 10, 8202–8210.
- Kubota, K.; Pang, Y.; Miura, A.; Ito, H. Redox Reactions of Small Organic Molecules Using Ball Milling and Piezoelectric Materials. Science 2019, 366, 1500–1504.
- Li, L.; Wang, G.-W. Mechanochemical Solvent-Free Synthesis of Indenones from Aromatic Carboxylic Acids and Alkynes. J. Org. Chem. 2021, 86, 14102–14112.
- Ni, S.; Hribersek, M.; Baddigam, S.K.; Ingner, F.J.L.; Orthaber, A.; Gates, P.J. Mechanochemical Solvent-Free Catalytic C–H Methylation. Angew. Chem. Int. Ed. 2021, 60, 6660–6666.
- Zhang, J.; Zhang, P.; Ma, Y.; Szostak, M. Mechanochemical Synthesis of Ketones via Chemoselective Suzuki–Miyaura Cross-Coupling of Acyl Chlorides. Org. Lett. 2022, 24, 2338–2343.
- Kong, D.; Amer, M.M.; Bolm, C. Stainless Steel-Initiated Chloro Sulfoximidations of Allenes Under Solvent-Free Conditions in a Ball Mill. Green Chem. 2022, 24, 3125–3129.
- Groutas, W.C.; Venkataraman, R.; Chong, L.S.; Yoder, J.E.; Epp, J.B.; Stanga, M.A.; Kim, E.-H. Isoxazoline Derivatives as Potential Inhibitors of the Proteolytic Enzymes Human Leukocyte Elastase, Cathepsin G and Proteinase 3: A Structure-Activity Relationship Study. Bioorg. Med. Chem. 1995, 3, 125–128.
- Wityak, J.; Sielecki, T.M.; Pinto, D.J.; Emmett, G.; Sze, J.Y.; Liu, J.; Tobin, A.E.; Wang, S.; Jiang, B.; Ma, P.; Mousa, S.A.; Wexler, R.R.; Olson, R.E. Discovery of Potent Isoxazoline Glycoprotein IIb/IIIa Receptor Antagonists. J. Med. Chem. 1997, 40, 50–60.
- Antczak, C.; Bauvois, B.; Monneret, C.; Florent, J.-C. A New Acivicin Prodrug Designed for Tumor-Targeted Delivery. Bioorg. Med. Chem. 2001, 9, 2843–2848.
- Kumbhare, R.M.; Kosurkar, U.B.; Ramaiah, M.J.; Dadmal, T.L.; Pushpavalli, S.N.C.V.L.; Pal-Bhadra, M. Synthesis and Biological Evaluation of Novel Triazoles and Isoxazoles Linked 2-Phenyl Benzothiazole as Potential Anticancer Agents. Bioorg. Med. Chem. Lett. 2012, 22, 5424–5427.
- Hamama, W.S.; Ibrahim, M.E.; Zoorob, H.H. Synthesis and Biological Evaluation of Some Novel Isoxazole Derivatives. J. Heterocycl. Chem. 2017, 54, 341–346.
- Changtam, C.; Hongmanee, P.; Suksamrarn, A. Isoxazole Analogs of Curcuminoids with Highly Potent Multidrug-Resistant Antimycobacterial Activity. Eur. J. Med. Chem. 2010, 45, 4446–4457.
- Silva, N.M.; Tributino, J.L.M.; Miranda, A.L.P.; Barreiro, E.J.; Fraga, C.A.M. New Isoxazole Derivatives Designed as Nicotinic Acetylcholine Receptor Ligand Candidates. Eur. J. Med. Chem. 2002, 37, 163–170.
- Kumar, J.; Chawla, G.; Akhtar, M.; Sahu, K.; Rathore, V.; Sahu, S. Design, Synthesis and Pharmacological Evaluation of Some Novel Derivatives of 1-{[3-(Furan-2-yl)-5-Phenyl-4,5-Dihydro-1,2-Oxazol-4-yl]Methyl}-4-Methyl Piperazine. Arab. J. Chem. 2017, 10, 141–149.
- Buoli, M.; Grassi, S.; Ciappolino, V.; Serati, M.; Altamura, A.C. The Use of Zonisamide for the Treatment of Psychiatric Disorders: A Systematic Review. Clin. Neuropharmacol. 2017, 40, 85–92.
- Bode, J.W.; Fraefel, N.; Muri, D.; Carreira, E.M. A General Solution to the Modular Synthesis of Polyketide Building Blocks by Kanemasa Hydroxy-Directed Nitrile Oxide Cycloadditions. Angew. Chem. Int. Ed. 2001, 40, 2082–2085.
- Minter, A.R.; Fuller, A.A.; Mapp, A.K. A Concise Approach to Structurally Diverse β-Amino Acids. J. Am. Chem. Soc. 2003, 125, 6846–6847.
- Maimone, T.J.; Shi, J.; Ashida, S.; Baran, P.S. Total Synthesis of Vinigrol. J. Am. Chem. Soc. 2009, 131, 17066–17067.
- Tang, S.; He, J.; Sun, Y.; He, L.; She, X. Efficient and Regioselective Synthesis of 5-Hydroxy-2-Isoxazolines: Versatile Synthons for Isoxazoles, β-Lactams, and γ-Amino Alcohols. J. Org. Chem. 2010, 75, 1961–1966.
- Choe, H.; Cho, H.; Ko, H.-J.; Lee, J. Total Synthesis of (+)-Pochonin D and (+)-Monocillin II via Chemo- and Regioselective Intramolecular Nitrile Oxide Cycloaddition. Org. Lett. 2017, 19, 6004–6007.
- Nomura, T.; Yokoshima, S.; Fukuyama, T. Total Synthesis of Huperzine R. Org. Lett. 2018, 20, 119–121.
- Moriya, O.; Nakamura, H.; Kageyama, T.; Urata, Y. Synthesis of Isoxazolines and Isoxazoles from Aldoximes by the Use of Sodium Bromite with Organotin Halide. Tetrahedron Lett. 1989, 30, 3987–3990.
- Radhakrishna, A.S.; Sivaprakash, K.; Singh, B.B. Iodobenzene Dichloride-An Efficient Reagent for Preparation of Nitrile Oxides from Aldoximes. Synth. Commun. 1991, 21, 1625–1629.
- Kiegiel, J.; Popławska, M.; Jóźwik, J.; Kosior, M.; Jurczak, J. New Method of In Situ Generation of Nitrile Oxides by MnO2 Oxidation of Aldoximes. Tetrahedron Lett. 1999, 40, 5605–5608.
- Pandit, P.; Chatterjee, N.; Halder, S.; Hota, S.K.; Patra, A.; Maiti, D.K. PhIO as a Powerful Cyclizing Reagent: Regiospecific [3+2]-Tandem Oxidative Cyclization of Imine Toward Cofacially Self-Aggregated Low Molecular Mass Organic Materials. J Org. Chem. 2009, 74, 2581–2584.
- Mendelsohn, B.A.; Lee, S.; Kim, S.; Teyssier, F.; Aulakh, V.S.; Ciufolini, M.A. Oxidation of Oximes to Nitrile Oxides with Hypervalent Iodine Reagents. Org. Lett. 2009, 11, 1539–1542.
- Minakata, S.; Okumura, S.; Nagamachi, T.; Takeda, Y. Generation of Nitrile Oxides from Oximes Using t-BuOI and Their Cycloaddition. Org. Lett. 2011, 13, 2966–2969.
- Pal, G.; Paul, S.; Ghosh, P.P.; Das, A.R. PhIO Promoted Synthesis of Nitrile Imines and Nitrile Oxides Within a Micellar Core in Aqueous Media: A Regiocontrolled Approach to Synthesizing Densely Functionalized Pyrazole and Isoxazoline Derivatives. RSC Adv. 2014, 4, 8300–8307.
- Yoshimura, A.; Nguyen, K.C.; Rohde, G.T.; Saito, A.; Yusubov, M.S.; Zhdankin, V.V. Oxidative Cycloaddition of Aldoximes with Maleimides Using Catalytic Hydroxy(Aryl)Iodonium Species. Adv. Synth. Catal. 2016, 358, 2340–2344.
- Gao, J.; Wang, G.-W. Direct Oxidative Amidation of Aldehydes with Anilines Under Mechanical Milling Conditions. J. Org. Chem. 2008, 73, 2955–2958.
- Wang, G.-W.; Gao, J. Solvent-Free Bromination Reactions with Sodium Bromide and Oxone Promoted by Mechanical Milling. Green Chem. 2012, 14, 1125–1131.
- Schmidt, R.; Stolle, A.; Ondruschka, B. Aromatic Substitution in Ball Mills: Formation of Aryl Chlorides and Bromides Using Potassium Peroxomonosulfate and NaX. Green Chem. 2012, 14, 1673–1679.
- Chen, K.; Niu, C.; Wang, G.-W. Reaction of Aldoximes with Sodium Chloride and Oxone Under Ball-Milling Conditions. Molecules 2020, 25, 3719.
- Fang, R.-K.; Chen, K.; Niu, C.; Wang, G.-W. Mechanochemical Dimerization of Aldoximes to Furoxans. Molecules 2022, 27, 2604.
- Friščić, T.; Jones, W. Recent Advances in Understanding the Mechanism of Cocrystal Formation via Grinding. Cryst. Growth Des. 2009, 9, 1621–1637.
- Štrukil, V.; Margetić, D.; Igrc, M.D.; Eckert-Maksić, M.; Friščić, T. Desymmetrisation of Aromatic Diamines and Synthesis of Non-Symmetrical Thiourea Derivatives by Click-Mechanochemistry. Chem. Commun. 2012, 48, 9705–9707.
- Tan, D.; Štrukil, V.; Mottillo, C.; Friščić, T. Mechanosynthesis of Pharmaceutically Relevant Sulfonyl-(Thio)Ureas. Chem. Commun. 2014, 50, 5248–5250.
- Li, H.-G.; Wang, G.-W. Liquid-Assisted One-Pot Mechanosynthesis and Properties of Neutral Donor-Acceptor [2]Rotaxanes. J. Org. Chem. 2017, 82, 6341–6348.
- Zhao, G.; Liang, L.; Wen, C.H.E.; Tong, R. In Situ Generation of Nitrile Oxides from NaCl−Oxone Oxidation of Various Aldoximes and Their 1,3-Dipolar Cycloaddition. Org. Lett. 2019, 21, 315–319.
- Xiang, C.; Li, T.; Yan, J. Hypervalent Iodine–Catalyzed Cycloaddition of Nitrile Oxides to Alkenes. Synth. Commun. 2014, 44, 682–688.
- Li, C.; Deng, H.; Li, C.; Jia, X.; Li, J. Palladium-Catalyzed Synthesis of Δ2-Isoxazoline from Toluene Derivatives Enabled by the Triple Role of Silver Nitrate. Org. Lett. 2015, 17, 5718–5721.
- Wang, D.-J.; Chen, B.-Y.; Wang, Y.-Q.; Zhang, X.-W. Ruthenium-Catalyzed Direct Transformation of Alkenyl Oximes to 5-Cyanated Isoxazolines: A Cascade Approach Based on Non-Stabilized Radical Intermediate. Eur. J. Org. Chem. 2018, 1342–1346.
- Han, L.; Zhang, B.; Zhu, M.; Yan, J. An Environmentally Benign Synthesis of Isoxazolines and Isoxazoles Mediated by Potassium Chloride in Water. Tetrahedron Lett. 2014, 55, 2308–2311.
- Kung, K.K.-Y.; Lo, V.K.-Y.; Ko, H.-M.; Li, G.-L.; Chan, P.-Y.; Leung, K.-C.; Zhou, Z.; Wang, M.-Z.; Che, C.-M.; Wong, M.-K. Cyclometallated Gold(III) Complexes as Effective Catalysts for Synthesis of Propargylic Amines, Chiral Allenes and Isoxazoles. Adv. Synth. Catal. 2013, 355, 2055–2070.
- Cosimelli, B.; Simorini, F.; Taliani, S.; Motta, C.L.; Settimo, F.D.; Severi, E.; Greco, G.; Novellino, E.; Costa, B.; Pozzo, E.D.; Bendinelli, S.; Martini, C. Tertiary Amides with a Five-Membered Heteroaromatic Ring as New Probes for the Translocator Protein. Eur. J. Med. Chem. 2011, 46, 4506–4520.
- Kobayashi, E.; Togo, H. Facile One-Pot Transformation of Primary Alcohols Into 3-Aryland 3-Alkyl-Isoxazoles and -Pyrazoles. Synthesis 2019, 51, 3723–3735.