ABSTRACT
The overexpression of Rab proteins was linked to cancer development, making this family of proteins an attractive drug target for the identification of novel inhibitors against tumor diseases. In this study, novel triazole-based azomethine/thiazolidin-4-one hybrid analogs were designed, prepared and structurally confirmed by using elemental and spectral techniques, these include FT-IR, MS, and NMR spectra. In addition, in vitro cytotoxic activity was assessed against NCI-60 cancer cell lines. To understand the possible mode of action and drugability, molecular docking studies and drug-likeness were achieved. The docking simulations were performed against various Rab family proteins Rab2a, Rab25, Rab5, and Rab35; promising targets for cancer medication to support the cytotoxicity findings and to further validate the action of new molecules. Furthermore, in silico ADMET screening of the molecules was within the recommended values stated by Lipinski's rule of five (Ro5), indicating their oral bioavailability and therapeutic potentials. Among the newly prepared analogs tested, compounds 4d and 3d exhibited significant antitumor activity against breast, ovarian, lung, and leukemia cancer cell lines. Our findings suggested that azomethine/thiazolidin-4-one moieties incorporated triazole analogs are a promising class of molecular entities for the development of new anticancer therapies, through targeting of some Rab proteins.
GRAPHICAL ABSTRACT

Introduction
Cancer is characterized by uncontrolled cell growth with metastasizes to other organs inside the body (Citation1). Rab proteins are widely expressed in various human cancers (Citation2–4). They are essential regulators of cell cycle progression and are responsible for gene expression (Citation5). Rab proteins work as sensitive molecular switch existing either in an active GDP-bound form or an active GTP-bound form. Exchange from GDP to GTP is catalyzed by the guanidine exchange factor (GEF), leading to activation in response to various upstream signals. On the other hand, GTPase-activating protein (GAP) increases the intrinsic GTPase activity, resulting in the inactivation of the protein (Citation3,Citation4,Citation6). The upregulation of the selected Rab proteins namely Rab2a, Rab25, Rab5, and Rab35 is linked with various human cancers such as breast, ovarian, lung, and leukemia, respectively (Citation5,Citation7,Citation8). Therefore, targeting Rab proteins is an important strategy for the prevention and treatment of cancer.
Molecular hybridization is considered a pivotal approach to developing novel compounds with anticancer activity (Citation9). A series of new triazole bisphosphonic acids were tested for their ability to inhibit GGTase II and induce cytotoxicity in human myeloma cells. Also, another research study reported the introduction of N-((1-benzyl-1H-1,2,3-triazol-4-yl) methyl)arylamide a new moiety that inhibits tubulin polymerization and tumor cell growth. Triazole-based azomethine/thiazolidin-4-one hybrid molecules have received considerable attention by their diverse biological activities and a wide range of therapeutic properties. There are numerous reports available in the literature highlighting the potential of triazole moiety as an anticancer candidate (Citation10,Citation11). Additionally, triazole moiety and N-bridged heterocyclic derivatives have a wide spectrum of biological activities and used in medicinal chemistry as drug candidates for the treatment of various diseases including cancer (Citation12–18).
Further, thiazolidin-4-one nucleus is of great interest in medicinal and pharmaceutical chemistry (Citation19). It is used as a precursor for the design of new bioactive molecules with pharmacological properties, such as anti-tumor, anti-inflammatory, and anti-microbial agents (Citation20–22). Moreover, azomethine compounds with imino fragment (–CH=N–) exhibited medicinal and biological importance and are used in drug design (Citation23), as represented in .
Based on these findings and in continuation of our research to prepare bioactive triazole analogs (Citation24–26) and to develop potent anticancer agents (Citation27–32), the present study was designed to develop green method to synthesize new series of triazole-based azomethine/thiazolidin-4-one hybrid analogs as promising lead compounds with high efficacy as anticancer drug candidates through targeting the selected Rab proteins. To get insight into the intermolecular interactions between the synthesized compounds and the target proteins, in silico molecular docking studies were performed (Citation33–35,Citation6,Citation36,Citation37). In addition, in silico ADMET screening of new molecules was conducted to determine their drug-likeness properties.
Results and discussion
Chemistry
Our aim in this study is to utilize ultrasonic irradiation as an ecofriendly and green synthetic methodology for the preparation of new series of Schiff bases and thiazolidinone derivatives, by using highly efficient and simple methods with moderate to very good yields. Moreover, the activity of the newly prepared Schiff bases as anticancer drug candidates was assessed in comparison with the synthesized thiazolidine-4-ones.
Usually, Schiff bases are prepared by refluxing in an appropriate solvent with acid or base as a catalyst. Herein, the comparison between the efficiency of this method with different techniques like-sonication (sonochemistry) and Dean–Stark apparatus will be discussed. Compared with traditional methods, these methods are more convenient, due to shorter reaction time, higher yields, milder conditions, and safety. Condensation of 2-[3-Amino- 5-(2-hydroxy-phenyl)-5-methyl-1,5-dihydro-[1,2,4]triazol-4-yl]-propionic acid 1a and 2-[3-Amino-5-(2-hydroxy- phenyl)-5-methyl-1,5-di hydro-[1,2,4]triazol-4-yl]-3-(1H-indol-3-yl)-propionic acid 1b with various aromatic aldehydes 2 under ultrasound irradiation (USI) at 60–65°C and/or under reflux using Dean–Stark apparatus afforded the desired Schiff bases 3a–d and 4a–f (Scheme 1).
Table
The data incorporated in illustrated the comparison of time and yield of Schiff bases under different methods. The obtained results showed that in the absence of USI, the times of reaction increased while the yields of the product slightly decreased. This finding exhibited a successful impact of USI on the synthesis of Schiff bases.
Table 1. Comparison of the yields of Schiff bases under ultrasonic irradiation, Dean-Stark apparatus and conventional method.
According to the previous results, our study was extended to examine the reactivity of compounds 3c and 4c which are believed to be a starting material for the preparation of 4-thiazolidinones 5c and 6c. Treatment of compounds 3c and 4c with ethyl thioglycolate in ethanol/TEA under ‘silent’ conditions yielded the cyclocondensation product, providing newly thiazolidinone analogs 5c and 6c. Upon repeating the reaction using ultrasonic irradiation, the formation of the product was achieved in a shorter time (monitored by TLC) with a good improvement in yield. However, a catalyzed ultrasound irradiation process using a molecular sieve (4 Å) yielded an excellent amount for 5c and 6c with an even shorter time. The time of reactions and the yield of products are tabulated in , indicating that the catalyzed ultrasound technique both reduced the reaction time and improved the yield of products ranging from 47% to 49% (under conventional conditions) to 91–93% (under catalyzed ultrasound technique).
Table 2. Comparison of the yields of thiazolidin-4-ones under catalyzed and uncatalyzed ultrasonic methods and conventional method.
In search of the best experimental reaction conditions for the preparation of 4-thiazolidinones 5c and 6c; multicomponent reaction (MCR) method was applied to compare the efficiency of the catalyzed ultrasound method with catalyzed one pot (silent reaction).
The utility of the silent reaction procedure depends on using β-cyclodextrin which known as a preferred catalyst in MCR reactions, and satisfies the requirements for green chemistry. The results have better performance than other reported catalysts (in terms of yield and rate). The desired thiazolidine-4-one analogs 5c and 6c were synthesized by mixing ArCHO and triazole derivatives 1a and 1b with ethyl mercaptoacetate in the presence of a catalytic amount of β-cyclodextrin (15%) under free solvent condition at 110°C (Scheme 2).
Upon repeating the reaction using catalyzed sonochemical one-pot, three-component condensation reaction against catalyzed one pot-MCR method, the data presented in also showed the formation of the desired products by catalyzed USI in a shorter time (monitored by TLC) without yield improvement at RT which save the energy required to operate the reaction.
Table 3. Comparison of catalyzed ultrasonic and one-pot MCR method.
The plausible reaction pathway for the synthesis of thiazolidin-4-ones is depicted in Scheme 3. β-cyclodextrin activates the lone pair of the carbonyl group of ArCHO by increasing the electrophilicity to accelerate the formation of the imine intermediate [A]. Then, the anion of β-cyclodextrin activates the nucleophilicity of the mercapto group of ethyl thioglycolate and enhances the addition to the formed imine intermediate. Moreover, the carbonyl group of ester activated by β-cyclodextrin to generate an intermediate [B]. Finally, rearrangement and cyclodehydration give the target molecule thiazolidin-4-ones.
Biological screening
This study investigates the effect of novel prepared molecules on different cancer cells. MG_MID mean graph midpoint generated the percent growth of the treated cells when compared to the untreated control cells for each cell line to facilitate visual scanning of data for potential NCI patterns of selectivity, with bars depicting the deviation of the individual tumor cell lines from the overall mean value for all the cells tested. The selected compounds were added at a single concentration (10ˉ5 M). Results of in vitro anticancer screening of the newly prepared compounds are illustrated in the Supplementary file section as Tables S1–12.
The results tabulated in Tables S1 and S2 (Supplementary file section) indicated that compounds 1a and 1b exhibited moderate to highly cytotoxic and/or growth inhibitory effects toward all tested cancer cell lines.
Compound 1a revealed highly growth inhibition of 51.72% and 70.71% against leukemia cell line RPMI-8226 and non-small cell lung cancer cell line EKVX, respectively. On the other hand, compound 1b recorded moderate growth inhibition of 20.69%, 53.97% and 55.86% against leukemia cell line RPMI-8226; non-small cell lung cancer cell lines EKVX and breast cancer cell line T-47D.
A survey of Tables S3–S10 (Supplementary file section) for Schiff base scaffolds 3a–d and 4a–d illustrated that the highest cytotoxic activities on most of the panel cell lines were achieved by compounds 3d and 4d as shown in Tables S6 and S10 (Supplementary file section). The results of compound 3d revealed high growth inhibition against leukemia cell line CCRF-CEM; breast cancer cell line T-47D at 62.55%, 50.41%. On the other hand, the results of compounds 4d at 47.85%, 40.85%. Moreover, compounds 3d and 4d revealed moderate growth inhibition of 39.22% and 33.49% against leukemia cell lines SR; 42.66% and 33.19% against non-small cell lung cancer cell lines HOP62; 25.74% and 34.61% against ovarian cancer cell line SK-OV-3, respectively.
By moving to the prepared thiazolidinone derivatives, the results recorded in Tables S11 and S12 (Supplementary file section) showed that compounds 5c and 6c exhibited good growth inhibitory effects toward NCI-60 cancer cell lines. Compound 5c recorded growth inhibition of 31.12%, 27.96%, 34.15%, 24.21% and 31.94% against leukemia cell lines CCRF-CEM; non-small cell lung cancer cell line HOP62; ovarian cancer cell line IGROV1; SK-OV-3 and breast cancer T-47D, respectively. Furthermore, compound 6c recorded high growth inhibition of 55.94%, 61.06%, 44.54%, and 43.00% against non-small cell lung cancer cell line HOP-62, ovarian cancer cell line SK-OV-3, breast cancer cell line T-47D, and leukemia cell line CCRF-CEM respectively.
In silico docking approach
Considering the importance of Rab proteins as drug targets in various human carcinomas, molecular docking studies were carried out to prove their anticancer activity mechanism. The molecular database of 14 compounds was docked to the selected proteins by using PyRx–irtual screening tool. The obtained data showed that the studied ligand molecules were found to be successful on the basis that they interacted with the active site pockets of the proteins. Moreover, the relative affinity of the compounds towards the target proteins was selected based on binding energy (ΔGbind) in kcal/mol, as illustrated in .
Table 4. The calculated (ΔGbind) binding energy of best docked molecules 4d and 3d and co-crystalized ligand with the target proteins.
The ligands interacted with proteins Rab2a, Rab25, Rab5, and Rab35 in the ranges of −9.0 to −5.8, −9.9 to −6.3, −10.9 to −6.8 and −10.4 to −7.7 kcal/mol, respectively. The top-ranked ligand–protein complexes of the top scoring molecules were visualized. The 2D and 3D representations of interaction maps of the top-ranked protein–ligand complexes are illustrated in . The 2D structures of the top-ranked docked compounds, docking energies (ΔGbind) in kcal/mol, and intermolecular bond lengths are tabulated in .
Figure 2. Molecular docking of best compounds 4d and 3d and Guanosine-5'-diphosphate with Rab2a. (Left side): 3D representations; the docked compounds are shown in grey stick model, while the docked residues are represented in blue stick model. (Right side): 2D representations; the docked compounds are shown in grey stick model, while the docked residues are represented in ball model. H-bonds are shown in green dotted lines, while π-stacking interactions are given in orange line.
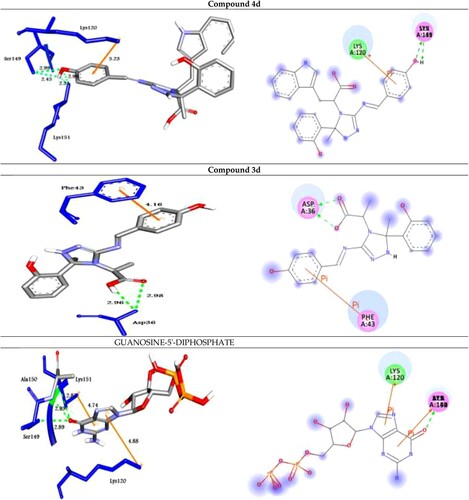
Figure 3. Molecular docking of best compounds 4d and 3d and Guanosine-5'-diphosphate with Rab25. (Left side): 3D representations; the docked compounds are shown in grey stick model, while the docked residues are represented in blue stick model. (Right side): 2D representations; the docked compounds are shown in grey stick model, while the docked residues are represented in ball model. H-bonds are shown in green dotted lines, while π-stacking interactions are given in orange line.
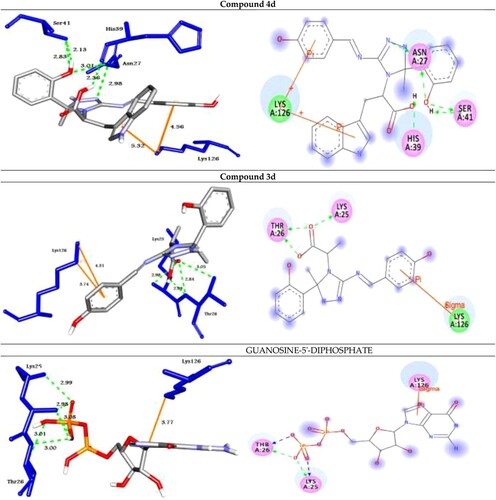
Figure 4. Molecular docking of best compounds 4d and 3d and Guanosine-5'-diphosphate with Rab5. (Left Side): 3D representations; the docked compounds are shown in grey stick model, while the docked residues are represented in blue stick model. (Right side): 2D representations; the docked compounds are shown in grey stick model, while the docked residues are represented in ball model. H-bonds are shown in green dotted lines, while π-stacking interactions are given in orange line.
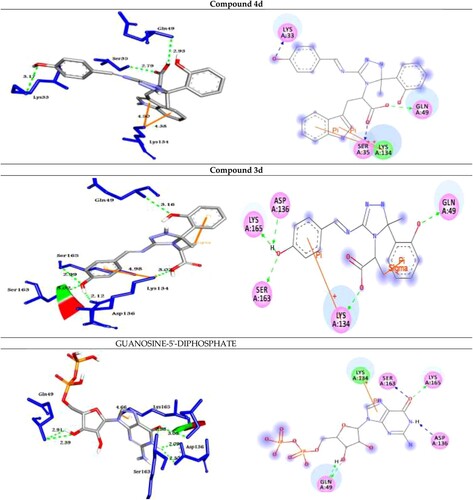
Figure 5. Molecular docking of best compounds 4d and 3d with Rab35. (Left side): 3D representations; the docked compounds are shown in grey stick model, while the docked residues are represented in blue stick model. (Right side): 2D representations; the docked compounds are shown in grey stick model, while the docked residues are represented in ball model. H-bonds are shown in green dotted lines, while π-stacking interactions are given in orange line
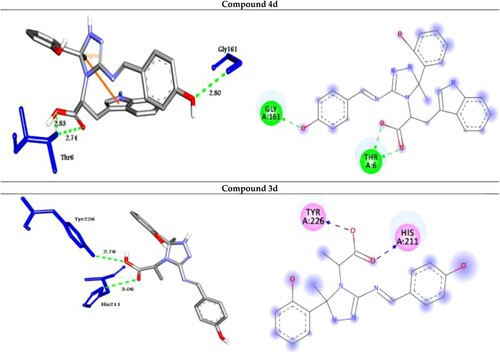
The analog 4d (with OH group at the para position of phenyl ring) was successfully docked into Rab2a, Rab25, Rab5, and Rab35 with the highest binding affinity of −9.0, −9.9, −10.9 and −10.4 kcal/mol, respectively. It docked with the Rab2a protein through H-bond and π-cation interactions with the residues Ser149, Lys151, and Lys120 at distances of 2.99, 2.45, 2.98, 2.31, and 5.23 Å, respectively. While it exhibited five H-bond and two π-cation interactions with the residues Asn27, Ser41, His39, and Lys126 of the target protein Rab25. Further, the compound 4d docked to the target Rab5 through three H-bond and two π-cation interactions with the amino acid residues Lys33, Ser35, Gln49, and Lys134 at 3.17, 2.79, 2.93, 4.38, and 4.50 Å, respectively. Finally, it formed four H-bond interactions with the target Rab35 as shown in .
On the other hand, the analog 3d (with OH group at the para position of phenyl ring) was docked to the target proteins Rab2a, Rab25, Rab5, and Rab35 with the binding energies of −8.8, −9.7, −10.5, and −9.8 kcal/mol, respectively. It exhibited two H-bonds and one π-cation with the protein Rab2a through the residues Asp36 and Phe43 at 2.98, 2.95, and 4.16 Å, respectively. Moreover, compound 3d showed three types of intermolecular interactions such as H-bond, π-cation, and π-sigma with the residues Lys25, Thr26, and Lys126 of the target Rab25. Moreover, it exhibited H-bond and π-cation interactions with Rab5 through Gln49, Lys134, Asp136, Ser163, and Lys165. Finally, the compound 3d showed two H-bond interactions with Rab35 through His211 and Tyr226 at 3.06, and 2.76 Å, respectively as illustrated in .
From the obtained data, we can conclude that introducing electron donating group (OH) at the para-position of the phenyl ring exhibited the highest activity as illustrated in compounds 4d and 3d.
In addition, to validate the docking process; GUANOSINE-5'-DIPHOSPHATE (a co-crystalized ligand) was removed from the target proteins and re-docked with their active sites (RMSD < 2 Å) and the results showed similar fitness to the docked molecules (, ).
To predict the drugability of the prepared molecules, ADMET and pharmacokinetics properties have been determined using admetSAR, mol inspiration, and SwissADME tools. It was noted that all molecules have zero violations of RO5 except compounds 4c and 6c. For all ligand molecules, they have good absorption properties in the range of 56.16–100%. The compounds except 4b, 4c, 5c, and 6c have good bioavailability scores as all have TPSA ≤ 140 Å. All compounds have no BBB permeability, providing safety on CNS (Citation38–40). None of the compounds are mutagenic nor carcinogenic and low toxicity level, indicating that the compounds are expected to be safe ().
Table 5. ADMET and drug-likeness of the prepared compounds 1a–6c.
Materials and methods
Chemistry
All the chemicals were purchased from El-Gomhouria Company for Drugs, Egypt, and used without further purification. Melting points were determined in open-glass capillaries using a Griffin melting point apparatus and are all uncorrected. IR spectra were recorded on Perkin Elmer1430 infrared spectrophotometer. NMR spectra were investigated on Jeol-400 MHz NMR-spectrometer (DMSO-d6) at Sohag University, Egypt. The chemical shifts are given in δ (ppm). Tetramethylsilane (TMS) was used as an internal standard. Micro analyses were performed on Vario El Fab-Nr elemental analyzer. Mass spectra were determined on a Hewlett Packard 5988 spectrometer (Microanalysis Center, Cairo University, Egypt). Thin-layer chromatography (TLC) was used to follow up on the reactions.
General procedure for synthesis of Schiff bases 3a–d
Method A: Silent reactions.
To a solution of compound 1 (10 mmol, 3.79 g) in DMF (10 mL), an appropriate aromatic aldehyde (10 mmol) was added. The mixture was allowed to reflux. Then the reaction mixture was poured onto ice bath and the formed precipitate was collected by vacuum filtration and recrystallized from ethanol (Citation41).
Method B: Dean–Stark apparatus.
A mixture of compound 1 (10 mmol, 3.79 g) and appropriate aromatic aldehyde (10 mmol) in toluene (30 mL) was refluxed using Dean–Stark apparatus. After completion of the reaction, about 60% of the solvent was stripped off and reaction mixture was cooled. Then the product formed was collected by vacuum filtration and recrystallized from a suitable solvent.
Method C: Sonicated reactions.
In a 100-mL Erlenmeyer flask, a solution of compound 1 (10 mmol, 3.79 g) and appropriate aromatic aldehyde (10 mmol) in ethanol (10 mL) was subjected to ultrasound irradiation at 60–65°C for the appropriate time () till disappearance of starting material (monitored by TLC). The precipitate formed was collected by vacuum filtration and recrystallized from ethanol to afford the corresponding Schiff bases.
(E)-2-(5-(2-hydroxyphenyl)-5-methyl-3-((naphthalen-2-ylmethylene)amino)-1H-1,2,4-triazol-4(5H)-yl)propanoic acid (3a)
Pale yellow crystals, m.p. 218°C. Anal. Calcd. for C23H22N4O3 (MW = 402.45): C, 68.64; H, 5.51; N, 13.92. Found: C, 68.55; H, 5.61; N, 13.89%. IR (υmax, cm−1): 3388 (OH), 3239 (OH), 3152 (NH), 3023 (CH)aromatic, 2982 (CH)aliphatic, 1641 (CH=N), 1591 (COOasy), 1532 (COOsy). 1H NMR (DMSO-d6), δ ppm: 1.97 (d, 3H, CH3), 2.59 (s, 3H, CH3), 3.83 (q, 1H, CH–COOH), 6.82–7.89 (m, 11H, 3ArH), 8.39 (s, 1H, NH, exchangeable by D2O), 8.64 (s, 1H, CH=N), 10.84 (s, 1H, OH, exchangeable by D2O), 11.31 (s, 1H, OH, exchangeable by D2O). 13C NMR (DMSO-d6), δ ppm: 19.47, 29.19 (2CH3), 37.12 (CH–COOH), 55.74 (Ctriazole), 114.57 (CH=N), 116.57, 119.81, 127.29, 128.85, 129.19, 129.80, 131.14, 131.65, 132.22, 134.15, 140.63 (m, ArC), 156.90 (C = N), 170.49 (C–OH), 178.18 (C = O).
(E)-2-(3-((4-hydroxy-3-methoxybenzylidene)amino)-5-(2-hydroxyphenyl)-5-methyl-1H-1,2,4-triazol-4(5H)-yl)propanoic acid (3b)
Pale yellow crystals, m.p. 188°C. Anal. Calcd. for C20H22N4O5 (MW = 398.41): C, 60.29; H, 5.57; N, 14.06. Found: C, 60.25; H, 5.67; N, 14.00%. IR (υmax, cm−1): 3504 (OH), 3412 (OH), 3295 (OH), 3215 (NH), 3030 (CH)aromatic, 2982 (CH)aliphatic, 1685 (CH=N), 1604 (COOasy), 1589 (COOsy), 1238 (C–O). 1H NMR (DMSO-d6), δ ppm: 2.22 (d, 3H, CH3), 2.65 (s, 3H, CH3), 3.46 (q, 1H, CH–COOH), 3.83 (s, 3H, OCH3), 4.35 (s, 1H, OH, exchangeable by D2O), 6.94 (s, 1H, NH, exchangeable by D2O), 6.96–8.11 (m, 7H, 2ArH), 8.41 (s, 1H, OH, exchangeable by D2O), 8.41 (s, 1H, CH=N), 11.42 (s, 1H, OH, exchangeable by D2O). 13C NMR (DMSO-d6), δ ppm: 17.87, 27.09 (2CH3), 33.76 (CH–COOH), 44.82 (C-triazole), 114.51 (CH=N), 117.38, 119.06, 129.82, 130.18, 130.53, 130.00, 132.60 (m, ArC), 139.52 (C=N), 160.56 (C–OH), 171.64 (C=O).
(E)-2-(5-(2-hydroxyphenyl)-5-methyl-3-((4-nitrobenzylidene)amino)-1H-1,2,4-triazol-4(5H)-yl)propanoic acid (3c)
Pale yellow crystals, m.p. 158°C. Anal. Calcd. for C19H19N5O5 (MW = 397.38): C, 57.43; H, 4.82; N, 17.62%. Found: C, 57.37; H, 4.85; N, 17.65%. IR (υmax, cm−1): 3388 (2OH), 3199 (NH), 3047 (CH)aromatic, 2995 (CH)aliphatic, 1668 (CH=N), 1615 (COOasy), 1590 (COOsy), 1347–1526 (NO2), 1229 (C–O). 1H NMR (DMSO-d6), δ ppm: 2.22 (d, 3H, CH3), 2.33 (s, 3H, CH3), 3.43 (q, 1H, CH-COOH), 6.87 (s, 1H, NH, exchangeable by D2O), 6.89–7.54 (m, 8H, 2ArH), 7.92 (s, 1H, OH, exchangeable by D2O), 8.70 (s, 1H, CH=N), 10.46 (s, 1H, OH, exchangeable by D2O). 13C NMR (DMSO-d6), δ ppm: 14.69, 22.68 (2CH3), 45.48 (CH-COOH), 55.38 (C-triazole), 113.64 (CH=N), 117.36, 119.09, 120.54, 126.51, 129.58, 130.50, 131.02, 136.51, 138.79 (m, ArC), 149.39 (C=N), 157.57 (C–OH), 161.08 (C–Cl), 180.72 (C=O).
(E)-2-(3-((4-hydroxybenzylidene)amino)-5-(2-hydroxyphenyl)-5-methyl-1H-1,2,4-triazol-4(5H)-yl)propanoic acid (3d)
Pale yellow crystals, m.p. 166°C. Anal. Calcd. for C19H20N4O4 (MW = 368.39): C, 61.95; H, 5.47; N, 15.21. Found: C, 61.90; H, 5.61; N, 15.17%. IR (υmax, cm−1): 3422 (OH), 3388 (OH), 3239 (OH), 3152 (NH), 3023 (CH)aromatic, 2982 (CH)aliphatic, 1641 (CH=N), 1591 (COOasy), 1532 (COOsy). 1H NMR (DMSO-d6), δ ppm: 2.21 (d, 3H, CH3), 2.31 (s, 3H, CH3), 2.72 (q, 1H, CH-COOH), 5.26 (s, 1H, OH, exchangeable by D2O), 6.81–7.52 (m, 7H, 2ArH), 7.67 (s, 1H, NH, exchangeable by D2O), 7.84 (s, 1H, OH, exchangeable by D2O), 8.39 (s, 1H, CH = N), 10.22 (s, 1H, OH, exchangeable by D2O). 13C NMR (DMSO-d6), δ ppm: 16.59, 20.90 (2CH3), 55.55 (CH-COOH), 63.04 (C-triazole), 114.11 (CH=N), 117.09, 117.23, 119.06, 122.69, 129.61, 129.82, 130.18, 130.53, 130.84, 131.00, 131.58, 132.60, 132.96, 147.40 (m, ArC), 166.83 (C = N), 169.66 (C–OH), 192.82 (C=O).
2. -[3-[(4-Dimethylamino-benzylidene)-amino]-5-(2-hydroxy-phenyl)-5-methyl-1,5-dihydro-[1,2,4]triazol-4-yl]-3-(1H-indol-3-yl)-propionic acid (4a)
Pale brown crystals, m.p. 143°C. Anal. Calcd. for C29H30N6O3 (MW = 510.59): C, 68.22; H, 5.92; N, 16.46. Found: C, 68.60; H, 5.99; N, 16.77%. IR (υmax, cm–1): 3340 (2 OH), 3270 (NH), 3208 (NH), 3058 (CH) aromatic, 2956 (CH) aliphatic, 1666 (CH = N), 1603 (COOasy), 1558 (COOsy), 1239 (C–O). 1H NMR (DMSO-d6), δ ppm: 2.11 (s, 3H, CH3), 2.36, 3.38 (s,s, 6H, 2CH3), 2.46, 2.66 (dd, 2H, CH2), 3.46 (q, 1H, CH-COOH), 6.95 (s, 1H, NH, exchangeable by D2O), 6.97–7.95 (m, 13H, 3ArH), 7.76 (s, 1H, NH, exchangeable by D2O), 8.70 (s, 1H, CH = N), 11.93 (s, 1H, OH, exchangeable by D2O), 12.86 (s, 1H, OH, exchangeable by D2O). 13C NMR (DMSO-d6), δ ppm: 27.03 (CH3), 28.19, 29.44 (2CH3), 32.24 (CH2), 50.56 (CH–COOH), 56.53 (C-triazole), 114.51 (CH=N), 117.36, 117.69, 118.02, 119.40, 119.93, 127.93, 128.24, 129.52, 130.04, 130.33, 130.59, 130.78, 131.79, 133.32, 136.66, 149.97 (m, ArC), 158.44 (C–NH), 159.98 (=C–N), 163.54 (C=N), 168.30 (C–OH), 181.36 (C=O).
2. -[3-[(4-Hydroxy-3-methoxy-benzylidene)-amino]-5-(2-hydroxy-phenyl)-5-methyl-1,5-dihydro-[1,2,4]triazol-4-yl]-3-(1H-indol-3-yl)-propionic acid (4b)
Pale yellow crystals, m.p. 131°C. Anal. Calcd. for C28H27N5O5 (MW = 513.55): C, 65.49; H, 5.30; N, 13.64. Found: C, 65.53; H, 5.28; N, 13.62%. IR (υmax, cm−1): 3404 (2OH), 3322 (OH), 3201 (NH), 3144 (NH), 3085 (CH)aromatic, 2965 (CH)aliphatic, 1666 (CH = N), 1601 (COOasy), 1547 (COOsy), 1212 (C–O). 1H NMR (DMSO-d6), δ ppm: 2.35 (s, 3H, CH3), 2.41, 2.57 (dd, 2H, CH2), 3.27 (q, 1H, CH-COOH), 3.97 (s, 3H, OCH3), 6.91 (s, 1H, NH, exchangeable by D2O), 6.93–8.39 (m, 12H, 3ArH), 8.64 (s, 1H, CH = N), 9.87 (s, 1H, OH, exchangeable by D2O), 10.84 (s, 1H, NH, exchangeable by D2O), 11.31 (s, 1H, OH, exchangeable by D2O), 12.02 (s, 1H, OH, exchangeable by D2O), 13C NMR (DMSO-d6), δ ppm: 15.57 (CH3), 18.87 (CH2), 24.61 (OCH3), 46.23 (CH–COOH), 56.54 (C-triazole), 111.81 (CH = N), 116.83, 117.38, 119.08, 120.60, 129.02, 129.14, 129.36, 130.32, 131.02, 132.22, 133.63, 134.74, 135.17, 141.38, 142.89 (m, ArC), 149.31 (C–NH), 153.61 (C = N), 157.74 (C–OCH3), 167.59 (C–OH), 178.57 (C–OH), 181.09 (C=O).
2. -[3-[(4-Nitro-benzylidene)-amino]-5-(2-hydroxy-phenyl)-5-methyl-1,5-dihydro-[1,2,4]triazol-4-yl]-3-(1H-indol-3-yl)-propionic acid (4c)
Pale yellow crystals, m.p. 114°C. Anal. Calcd. For C27H24N6O5 (MW = 512.52): C, 63.27; H, 4.72; N, 16.40. Found: C, 63.29; H, 4.64; N, 16.46%. IR (υmax, cm−1): 3378 (2OH), 3211 (NH), 3171 (NH), 3095 (CH)aromatic, 2935 (CH)aliphatic, 160..44 (CH=N), 1598 (COOasy), 1532 (COOsy), 1530–1320 (NO2), 1230 (C–O). 1H NMR (DMSO-d6), δ ppm: 1.79 (s, 3H, CH3), 2.92, 3.32 (dd, 2H, CH2), 3.48 (q, 1H, CH-COOH), 4.49 (s, 2H, 2NH, exchangeable by D2O), 6.83-8.27 (m, 13H, 3ArH), 8.57 (s, 1H, CH = N), 10.14 (s, 1H, OH, exchangeable by D2O), 10.93 (s, 1H, OH, exchangeable by D2O), 13C NMR (DMSO-d6), δ ppm: 24.63 (CH3), 27.43 (CH2), 44.26 (CH-COOH), 55.03 (C-triazole), 109.40 (CH=N), 111.82, 117.26, 117.42, 118.85, 119.00, 119.22, 121.46, 123.78, 124.70, 127.70, 127.84, 128.69, 128.73, 130.96, 136.80, 149.10, 149.42 (m, ArC), 152.70 (C-NH), 153.82 (C–OH), 157.90 (C=N), 172.30 (C–NO2), 181.82 (C=O).
2. -[3-[(4-Hydroxy-benzylidene)-amino]-5-(2-hydroxy-phenyl)-5-methyl-1,5-dihydro-[1,2,4]triazol-4-yl]-3-(1H-indol-3-yl)-propionic acid (4d)
Yellow crystals, m.p. 155°C. Anal. Calcd. for C27H25N5O4 (MW = 483.52): C, 67.07; H, 5.21; N, 14.48. Found: C, 67.12; H, 5.23; N, 14.41%. IR (υmax, cm−1): 3404 (2OH), 3352 (OH), 3266 (NH), 3114 (NH), 3055 (CH)aromatic, 2935 (CH)aliphatic, 1657 (CH = N), 1601 (COOasy), 1549 (COOsy), 1233 (C–O). 1H NMR (DMSO-d6), δ ppm: 1.89 (s, 3H, CH3), 2.35, 2.54 (dd, 2H, CH2), 3.26 (q, 1H, CH-COOH), 6.98 (s, 1H, NH, exchangeable by D2O), 6.99–8.13 (m, 13H, 3ArH), 7.96 (s, 1H, OH, exchangeable by D2O), 8.61 (s, 1H, CH=N), 10.09 (s, 1H, NH, exchangeable by D2O), 10.84 (s, 1H, OH, exchangeable by D2O), 12.81 (s, 1H, OH, exchangeable by D2O). 13C NMR (DMSO-d6), δ ppm: 17.87 (CH3), 27.23 (CH2), 54.26 (CH-COOH), 61.44 (C-triazole), 111.87 (CH = N), 117.69, 118.81, 123.78, 126.73, 128.07, 128.73, 129.13, 129.33, 129.65, 130.22, 130.34, 131.56, 132.14, 133.05, 136.62, 139.63 (m, ArC), 147.37 (C–NH), 148.33 (C–OH), 159.98 (C=N), 164.31 (C–OH), 179.76 (C=O).
2. -[3-[(4-Fluoro-benzylidene)-amino]-5-(2-hydroxyphenyl)-5-methyl1,5-dihydro[1,2,4]triazol-4-yl]-3-(1H-indol-2-yl)propionic acid (4e)
Pale yellow crystals, m.p. 108°C. Anal. Calcd. for C27H24FN5O3 (MW = 485.51): C, 66.79; H, 4.98; F, 3.91; N, 14.42. Found: C, 66.75; H, 4.77; F, 3.99; N, 14.63%. IR (υmax, cm−1): 3386 (OH), 3230 (OH), 3148 (NH), 3122 (NH), 3040 (CH)aromatic, 2982 (CH)aliphatic, 1596 (COOasy), 1668 (CH = N), 1530 (COOsy), 1225 (C–O), 514 (C–F). 1H NMR (CDCl3), δ ppm: 1.19 (s, 3H, CH3), 1.97, 2.03 (dd, 2H, CH2), 2.56 (q, 1H, CH–COOH), 6.31 (s, 2H, NH, OH, exchangeable by D2O), 6.83–7.80 (m, 13H, 3ArH),), 7.80 (s, 1H, CH=N), 9.70 (s, 2H, NH, OH, exchangeable by D2O). 13C NMR (CDCl3), δ ppm: 22.95 (CH3), 27.23 (CH2), 54.26 (CH-COOH), 61.44 (Ctriazole), 111.87 (CH = N), 117.69, 118.81, 123.78, 126.73, 128.73, 129.33, 130.22, 131.37, 132.14, 133.05, 136.62, 139.63 (m, ArC), 147.37 (C-NH), 148.33 (C = N), 160.22 (C–F), 164.31 (C–OH), 179.76 (C = O).
2. -[3-[(4-bromo-benzylidene)-amino]-5-(2-hydroxyphenyl)-5-methyl1,5-dihydro[1,2,4]triazol-4-yl]-3-(1H-indol-2- yl)propionic acid (4f)
Yellow crystals, m.p. 124°C. Anal. Calcd. for C27H24BrN5O3 (MW = 546.42): C, 59.35; H, 4.43; Br, 14.62; N, 12.82. Found: C, 59.38; H, 4.41; Br, 14.64; N, 12.79%. IR (υmax, cm−1): 3339 (2 OH), 3168 (NH), 3101(NH), 3075 (CH)aromatic, 2976 (CH)aliphatic, 1656 (CH = N), 1604 (COOasy), 1580 (COOsy), 1237 (C–O), 640 (C–Br). 1H NMR (DMSO-d6), δ ppm: 1.57 (s, 3H, CH3), 2.41, 2.63 (dd, 2H, CH2), 3.06 (q, 1H, CH–COOH), 6.81 (s, 1H, NH, exchangeable by D2O), 6.83-8.21 (m, 13H, 3ArH),), 8.70 (s, 1H, CH = N), 9.70 (s, 2H, NH, OH, exchangeable by D2O), 10.81 (s, 1H, OH, exchangeable by D2O). 13C NMR (DMSO-d6), δ ppm: 24.15 (CH3), 27.88 (CH2), 51.26 (CH-COOH), 59.23 (C-triazole), 111.77 (CH=N), 117.12, 118.32, 119.44, 121.08, 123.73, 126.33, 128.73, 129.33, 130.41, 133.54, 133.74, 133.95, 136.47, 139.61 (m, ArC), 148.55 (C–NH), 149.53 (C=N), 159.22 (C–Br), 166.51 (C–OH), 179.91 (C=O).
General procedure for synthesis of thiazolidin-4-ones (5c) and (6c)
Method A: Silent reaction.
A mixture of Schiff bases 3c and/or 4c (1 mmol) and ethyl thioglycolate (1 mmol, 0.1 mL) in EtOH/TEA, was heated under reflux for 5–7 hours (until the disappearance of the starting materials as examined by TLC) (cf. ). The reaction mixture was cooled at RT and the precipitate formed was collected, filtered, and recrystallized from ethanol.
Method B: Sonicated reaction.
A solution of Schiff bases 3c and/or 4c (1 mmol) in absolute ethanol and ethyl thioglycolate (1 mmol, 0.1 mL), was subjected to ultrasound irradiation for a suitable time (cf. ) until the starting material was no longer detectable by TLC. The reaction temperature was kept at 80–85°C inside the reaction vessel was 80°C. The reaction mixture was cooled at RT and the precipitate formed was collected, filtered, and recrystallized from ethanol.
Method C: Catalyzed sonicated reaction.
To a mixture of Schiff bases 3c and/or 4c (1 mmol) and ethyl thioglycolate (1 mmol, 0.1 mL) in ethanol, a molecular sieve (1 g, 4 Å) was added. The reaction mixture was subjected to ultrasound irradiation for a suitable time (cf. ). The reactants were kept at 80–85°C which was attained by the addition or removal of water in the ultrasonic bath (the temperature inside the reaction vessel was 80°C). The reaction mixture was decanted and cooled. The formed precipitate was collected, filtered, and recrystallized from ethanol.
General procedure for one-pot synthesis of thiazolidin-4-ones
Method A: Catalyzed one-pot, three-component condensation reaction.
The starting compound 1a and/or 1b (1 mmol), ethyl thioglycolate (1 mmol, 0.1 mL) and p-nitrobenzaldehyde (1 mmol, 0.12 g) in presence of (15% mol) of β-cyclodextrin, was magnetically stirred, then gradually the temperature was increased, and refluxed at 110°C under free solvent conditions. After completion of the reaction, the mixture was allowed to pour into 20 mL of H2O. The precipitated was filtered off and recrystallized with ethanol/H2O to yield the target product. The filtered aqueous layer was cooled at 5°C to recovery of β-cyclodextrin by filtration. The recovered β-cyclodextrin was reused for 2–3 consecutive runs in this reaction without any significant loss in yield and activity.
Method B: Catalyzed sonochemical one-pot, three component condensation reaction.
A mixture of compound 1a and/or 1b (1 mmol), ethyl thioglycolate (1 mmol, 0.1 mL), p-nitrobenzaldehyde (1 mmol, 0.12 g) and molecular sieve (1 g, 4 Å) in absolute ethanol, was subjected to ultrasound irradiation for a suitable time (cf. ) at RT 25–30°C which attained by addition or removal of water in ultrasonic bath (the temperature inside reaction vessel was 28–30°C). The sonochemical reactions were continued until the starting materials were no longer detectable by TLC. The products were obtained and purified as described above in reaction procedures.
2.-(5-(2-hydroxyphenyl)-5-methyl-3-(2-(4-nitrophenyl)-4-oxothiazolidin-3-yl)-1H-1,2,4-triazol-4(5H)-yl)propanoic acid 5c.
Pale brown crystals, m.p. 265°C. Anal. Calcd. for C21H21N5O6S (MW = 471.49): C, 53.50; H, 4.49; N, 14.85; S, 6.80. Found: C, 53.39; H, 4.52; N, 14.89; S, 6.84%. IR (υmax, cm−1): 3363 (OH), 3245 (OH), 3212 (NH), 3069 (CH)aromatic, 2993 (CH)aliphatic, 1666 (C = O), 1597 (COOasy), 1509 (COOsy), 1346–1555 (NO2), 1243 (C–O). 1H NMR (DMSO-d6), δ ppm: 2.33 (s, 3H, CH3), 3.07 (d, 3H, CH3), 3.47 (q, 1H, CH-COOH), 4.21 (s, 2H, CH2 thiazolidine), 6.09 (s, 1H, CH–Ar–NO2), 7.74 (s, 1H, NH, exchangeable by D2O), 7.84 (s, 1H, OH, exchangeable by D2O), 7.68–8.73 (m, 8H, 2ArH), 10.85 (s, 1H, NH, exchangeable by D2O), 11.02 (s, 1H, OH, exchangeable by D2O). 13C NMR (DMSO-d6), δ ppm: 17.43 (CH3), 20.21 (CH2), 28.53 (CH2), 49.46 (CH-COOH), 62.25 (CH–Ar), 62.92 (C-triazole), 113.04, 115.52, 116.19, 120.08, 121.09, 122.01, 122.30, 124.22, 126.03, 129.62, 130.04, 133.32, 134.16, 135.27, 136.15 (m, ArC), 145.54 (C-NH), 148.27 (C=N), 152.28 (C–NO2), 161.08 (C-OH), 178.68 (C=O), 181.70 (C = O).
2. -(5-(2-hydroxyphenyl)-5-methyl-3-(2-(4-nitrophenyl)-4-oxothiazolidin-3-yl)-1H-1,2,4-triazol-4(5H)-yl)-3-(1H-indol-3-yl)propanoic acid 6c.
Yellow crystals, m.p. 232°C. Anal. Calcd. for C29H26N6O6S (MW = 586.61): C, 59.38; H, 4.47; N, 14.33; S, 5.47. Found: C, 59.45; H, 4.49; N, 14.30; S, 5.41%. IR (υmax, cm−1): 3429 (2 OH), 3256 (NH), 3153 (NH), 3028 (CH)aromatic, 2989 (CH)aliphatic, 1650 (C = O), 1598 (COOasy), 1521 (COOsy), 1320–1511 (NO2). 1H NMR (DMSO-d6), δ ppm: 1.92 (s, 3H, CH3), 2.10, 2.20 (dd, 2H, CH2), 3.14 (q, 1H, CH–COOH), 4.86 (s, 2H, CH2thiazolidinone), 5.24 (s, 1H, NH, exchangeable by D2O), 5.93 (s, 2H, NH, CH, exchangeable by D2O, CH–Ar–NO2), 7.49–8.73 (m, 13H, 3ArH),), 8.14 (s, 1H, OH, exchangeable by D2O), 10.16 (s, 1H, OH, exchangeable by D2O). 13C NMR (DMSO-d6), δ ppm: 21.33 (CH3), 32.50 (CH2), 46.59 (CH2 thiazolidinone), 62.92 (CH–COOH), 68.38 (CH–Ar–NO2), 69.83 (C-triazole), 121.77, 122.52, 123.32, 124.27, 125.72, 129.25, 129.69, 130.50, 130.76, 133.76, 134.58, 135.33, 137.81, 140.56 (m, 3ArC), 143.40 (C–NH), 144.74 (C=N), 147.88 (C–OH), 148.81 (C–NO2), 161.43 (C=N), 161.62 (C=O), 179.34 (C=O).
All the chemical structures and spectral data of the repaired compounds are incorporated in the Supplementary file section as Figures S1–S36.
Anticancer screening
Twelve compounds 1a, 1b, 3a-3d, 4a–4d, 5c, and 6c were selected by National Cancer Institute (NCI) according to the protocol of the Drug Evaluation Branch of the National Cancer Institute, Bethesda, USA, for in vitro anticancer screening (Citation42,Citation43). The prepared analogs were tested at single concentration of 10−5 M and the culture was incubated for 48 hours. End-point determinations were made with a protein binding dye, Sulforhodamine B (SRB). All the screened compounds reduced the growth of most cancer cell lines to 32% or less. Additionally, they are called active and subsequently passed for further evaluation toward NCI-60 human tumor cell lines derived from nine different cancers: leukemia, melanoma, lung, colon, CNS, ovarian, renal, prostate, and breast.
Computational study
3D structures of proteins Rab2a, Rab25, Rab5, and Rab35 were identified from Protein data bank (PDB IDs: 1Z0A, 2OIL, 1N6H and 3TW8), as illustrated in . The files were improved by removing the water molecules, incorrect bond orders, and co-crystalized ligands. CHARMm Force Field (Citation44) in Discovery Studio 3.5 Visualizer was used to minimize their energies. Moreover, the generated SMILES were used as input to obtain the 3D structures of new molecules by using Open Babel GUI 2.4.1 tool (Citation45). In total, a library of novel triazole-based azomethine/thiazolidin-4-one derivatives was generated. UFF force field (Citation46) in PyRx tool was utilized in minimizing the energies of compounds. Subsequently, an in-house dataset of 14 compounds was performed for the docking process. Grid maps of 25*25*25 Å3 were allocated around the active site regions of the selected proteins.
Table 6. The selected Rab proteins with PDB ID.
Docking simulations were carried out using PyRx –virtual screening tool (Citation47). All synthesized analogs were successfully interacted with the active sites of the targets through docking simulations using the Lamarckian genetic algorithm (LGA) (Citation48). The best protein–ligand complexes were ranked based on the binding energy of the docked complex. Nine conformers were resulted for each docked molecule, and the conformer with lowest binding affinity was selected for further study (Citation49).
Discovery Studio 3.5 was used to generate the 2D and 3D representations of docking results. Further, in silico ADMET screening of the ligand molecules was achieved to estimate the physicochemical and pharmacokinetics, using free webservers namely, admetSAR, mol inspiration, and SwissADME. Lipinski's rule of five (Ro5) (Citation50) states that a molecule can act as a drug if the physicochemical properties such as Molecular Weight (MW) ≤ 500, polar Solvent Accessibility (TPSA) ≤ 140 Å2, Octane/water partition coefficient (MLogP) < 5, Hydrogen Bond Donors (HBD) in range of 0.0–6.0, and Hydrogen Bond Acceptors (HBA) in range of 0.2–20.0.
Conclusion
In this work, under both sonication and classical conditions, new class of novel Schiff bases and thiazolidine analogues were successfully synthesized. Ultrasonic irradiation resulted in significant enhancement in both rates and yields of reactions. Using solid catalysts both improves the efficacy of sonication and leads to improve yield and lessen reaction time. Moreover, various Rab proteins were selected as targets for the molecular docking simulation to gain insight about anti-cancer activity mechanism for the prepared compounds. The obtained results were highly correlated with the biological screening finding. The results suggested that these azomethine/thiazolidin-4-one moieties incorporated triazole analogs are a promising class of molecular entities for the development of novel candidates as Rab proteins inhibitors for treating cancer.
Author contributions
All authors have contribution to the reported work, whether that is in study design, data analysis, and interpretation. In addition they took part in drafting, revising or reviewing the manuscript; have agreed on the journal to which the article has been submitted.
Availability of data and materials
The original contributions presented in the study are included in the article/Supplementary Material; further inquiries can be directed to the corresponding author.
Supplemental Material
Download MS Word (3.7 MB)Disclosure statement
No potential conflict of interest was reported by the author(s).
References
- Popat, K.; McQueen, K.; Feeley, T.W. The Global Burden of Cancer. Best Pract. Res. Clin. Anaesthesiol. 2013, 27 (4), 399–408. doi:10.1016/j.bpa.2013.10.010.
- Chen, Y.; Ng, F.; Tang, B.L. Rab23 Activities and Human Cancer—Emerging Connections and Mechanisms. Tumor Biol. 2016, 37 (10), 12959–12967. doi:10.1007/s13277-016-5207-7.
- Ha, A.; Sp, L. Human Rab8b Protein as a Cancer Target – An In Silico Study. J. Comput. Sci. Syst. Biol. 2016, 9 (4), 132–149. doi:10.4172/jcsb.1000231.
- Abdelmonsef, A.H.; Dulapalli, R.; Dasari, T.; Padmarao, L.S.; Mukkera, T.; Vuruputuri, U. Identification of Novel Antagonists for Rab38 Protein by Homology Modeling and Virtual Screening. Comb. Chem. High Throughput Screen. 2016, 19 (10), 1–18. doi:10.2174/1386207319666161026153237.
- Tzeng, H.T.; Wang, Y.C. Rab-Mediated Vesicle Trafficking in Cancer. J. Biomed. Sci. 2016, 23 (1), 1–7. doi:10.1186/s12929-016-0287-7.
- Dasari, T.; Kondagari, B.; Dulapalli, R.; Abdelmonsef, A.H.; Mukkera, T.; Padmarao, L.S.; Malkhed, V.; Vuruputuri, U. Design of Novel Lead Molecules Against RhoG Protein as Cancer Target – a Computational Study. J. Biomol. Struct. Dyn. Oct. 2017, 35 (14), 3119–3139. doi:10.1080/07391102.2016.1244492.
- Krishnan, P.D.G.; Golden, E.; Woodward, E.A.; Pavlos, N.J.; Blancafort, P. Rab Gtpases: Emerging Oncogenes and Tumor Suppressive Regulators for the Editing of Survival Pathways in Cancer. Cancers (Basel). 2020, 12 (2), doi:10.3390/cancers12020259.
- Jin, H.; Tang, Y.; Yang, L.; Peng, X.; Li, B.; Fan, Q.; Wei, S.; Yang, S.; Li, X.; Wu, B.; Huang, M. Rab GTPases: Central Coordinators of Membrane Trafficking in Cancer. Front. Cell Dev. Biol. 2021, 9 (June), 1–13. doi:10.3389/fcell.2021.648384.
- dos Santos, L.T.D.; Teixeira, M.L.; Carneiro, J. Synthesis of a New Drug Candidate Trough Molecular Hybridization and Application As a Learning Strategy / Síntese De Um Novo Candidato À Fármaco Através De Hibridação Molecular E Aplicação Como Estratégia De Aprendizagem. Brazilian J. Dev. 2021, 7 (3), 22223–22233. doi:10.34117/bjdv7n3-096.
- Hamid, A.M.A.; El-Sayed, H.A.; Mohammed, S.M.; Moustafa, A.H.; Morsy, H.A. Functionalization of 1,2,3-Triazole to Pyrimidine, Pyridine, Pyrazole, and Isoxazole Fluorophores with Antimicrobial Activity. Russ. J. Gen. Chem. 2020, 90 (3), 476–482. doi:10.1134/S1070363220030226.
- Yakantham, T.; Sreenivasulu, R.; Alluraiah, G.; Tej, M.B.; Ramesh Raju, R. Design, Synthesis, and Anticancer Activity of 1,2,3-Triazole Linked Thiazole-1,2-Isoxazole Derivatives. Russ. J. Gen. Chem. 2019, 89 (12), 2522–2527. doi:10.1134/S1070363219120314.
- Ghanaat, J.; Khalilzadeh, M.A.; Zareyee, D. Molecular Docking Studies, Biological Evaluation and Synthesis of Novel 3-Mercapto-1,2,4-Triazole Derivatives. Mol. Divers. 2021, 25 (1), 223–232. doi:10.1007/s11030-020-10050-0.
- Ghanaat, J.; Khalilzadeh, M.A.; Zareyee, D.; Shokouhimehr, M.; Varma, R.S. Cell Cycle Inhibition, Apoptosis, and Molecular Docking Studies of the Novel Anticancer Bioactive 1,2,4-Triazole Derivatives. Struct. Chem. 2020, 31 (2), 691–699. doi:10.1007/s11224-019-01453-3.
- Poor Heravi, M.R.; Hemmati, S.; Nami, N.; Khalilzadeh, M.A. Synthesis of Novel Biologically Important 5-Amino-2-Oxo-7-Aryl-3,7-Dihydro-2H-Pyrano[2,3-d]Thiazole-6-Carbonitriles in Trifluoroethanol (TFE) Under Ultrasound Irradiation Condition and Their Antimicrobial Activity. Polycycl. Aromat. Compd. 2021, 41 (10), 2263–2273. doi:10.1080/10406638.2019.1711432.
- Hosseinzadeh, M.; Mohamad, J.; Khalilzadeh, M.A.; Zardoost, M.R.; Haak, J.; Rajabi, M. Isolation and Characterization of Bioactive Compounds from the Bark of Litsea Costalis. J. Photochem. Photobiol. B Biol. 2013, 128, 85–91. doi:10.1016/j.jphotobiol.2013.08.002.
- Khaleghi, F.; Din, L.B.; Charati, F.R.; Yaacob, W.A.; Khalilzadeh, M.A.; Skelton, B.; Makha, M. A New Bioactive Compound from the Roots of Petasites Hybridus. Phytochem. Lett. 2011, 4 (3), 254–258. doi:10.1016/j.phytol.2011.04.009.
- Tavakolinia, F.; Baghipour, T.; Hossaini, Z.; Zareyee, D.; Khalilzadeh, M.A.; Rajabi, M. Antiproliferative Activity of Novel Thiopyran Analogs on MCF-7 Breast and HCT-15 Colon Cancer Cells: Synthesis, Cytotoxicity, Cell Cycle Analysis, and DNA-Binding. Nucleic Acid Ther. 2012, 22 (4), 265–270. doi:10.1089/nat.2012.0346.
- Rajabi, M.; Khalilzadeh, M.A.; Tavakolinia, F.; Signorelli, P.; Ghidoni, R.; Santaniello, E. Naphthalene-fused (α-Alkoxycarbonyl)Methylene-γ-Butyrolactones: Antiproliferative Activity and Binding to Bovine Serum Albumin and DNA. DNA Cell Biol. 2012, 31 (5), 783–789. doi:10.1089/dna.2011.1433.
- Gomha, S.M.; Badrey, M.G. A Convenient Synthesis of Some New Thiazole and Pyrimidine Derivatives Incorporating a Naphthalene Moiety. J. Chem. Res. 2013, 37 (2), 86–90. doi:10.3184/174751912X13567137687630.
- Raza, S.; Srivastava, S.P.; Srivastava, D.S.; Srivastava, A.K.; Haq, W.; Katti, S.B. Thiazolidin-4-One and Thiazinan-4-One Derivatives Analogous to Rosiglitazone as Potential Antihyperglycemic and Antidyslipidemic Agents. Eur. J. Med. Chem. 2013, 63, 611–620. doi:10.1016/j.ejmech.2013.01.054.
- Kendre, M.M.; Baseer, M.A. Synthesis and Evaluation of Some new Pyrazoline Derivatives as Antimicrobial Agents. Orient. J. Chem. 2013, 29 (1), 253–256. doi:10.13005/ojc/290140.
- Gomha, S.M.; Abdelhady, H.A.; Hassain, D.Z.; Abdelmonsef, A.H.; El-Naggar, M.; Elaasser, M.M.; Mahmoud, H.K. Thiazole-Based Thiosemicarbazones: Synthesis, Cytotoxicity Evaluation and Molecular Docking Study. Drug Des. Devel. Ther. Feb. 2021, 15, 659–677. doi:10.2147/DDDT.S291579.
- Kajal, A.; Bala, S.; Kamboj, S.; Sharma, N.; Saini, V. Schiff Bases: A Versatile Pharmacophore. J. Catal. 2013, 2013 (Mic), 1–14. doi:10.1155/2013/893512.
- Mohamed, M.A.A.; Abd Allah, O.A.; Bekhit, A.A.; Kadry, A.M.; El-Saghier, A.M.M. Synthesis and Antidiabetic Activity of Novel Triazole Derivatives Containing Amino Acids. J. Heterocycl. Chem. 2020, 57 (6), 2365–2378. doi:10.1002/jhet.3951.
- El-Saghier, A.M.; Mohamed, M.A.; Abd-Allah, O.A.; Kadry, A.M.; Ibrahim, T.M.; Bekhit, A.A. Green Synthesis, Antileishmanial Activity Evaluation, and in Silico Studies of New Amino Acid-Coupled 1,2,4-Triazoles. Med. Chem. Res. 2019, 28 (2), 169–181. doi:10.1007/s00044-018-2274-x.
- Mohamed, M.A.A.; Bekhit, A.A.; Abd Allah, O.A.; Kadry, A.M.; Ibrahim, T.M.; Bekhit, S.A.; Amagase, K.; El-Saghier, A.M. Synthesis and Antimicrobial Activity of Some Novel 1,2-Dihydro-[1,2,4]Triazolo[1,5-: A] Pyrimidines Bearing Amino Acid Moiety. RSC Adv. 2021, 11 (5), 2905–2916. doi:10.1039/d0ra08189b.
- Rondla, R.; PadmaRao, L.S.; Ramatenki, V.; Haredi-Abdel-Monsef, A.; Potlapally, S.R.; Vuruputuri, U. Selective ATP Competitive Leads of CDK4: Discovery by 3D-QSAR Pharmacophore Mapping and Molecular Docking Approach. Comput. Biol. Chem. Dec. 2017, 71, 224–229. doi:10.1016/j.compbiolchem.2017.11.005.
- Haredi Abdelmonsef, A. Computer-aided Identification of Lung Cancer Inhibitors Through Homology Modeling and Virtual Screening. Egypt. J. Med. Hum. Genet. 2019, 20 (1), 1–14. doi:10.1186/s43042-019-0008-3.
- El-Maghraby, A.M.; Abdelmonsef, A.H. Synthesis, Characterization and in Silico Molecular Docking Studies of Novel Chromene Derivatives as Rab23 Inhibitors. Egypt. J. Chem. 2020, 63 (4), 1341–1358. doi:10.21608/ejchem.2019.15013.1911.
- Abdelmonsef, A.H.; Mosallam, A.M. Synthesis, in Vitro Biological Evaluation and in Silico Docking Studies of new Quinazolin-2,4-Dione Analogues as Possible Anticarcinoma Agents. J. Heterocycl. Chem. Apr. 2020, 57 (4), 1637–1654. doi:10.1002/jhet.3889.
- Noser, A.A.; El-Naggar, M.; Donia, T.; Abdelmonsef, A.H. Synthesis, In Silico and In Vitro Assessment of New Quinazolinones as Anticancer Agents via Potential AKT Inhibition. Molecules Oct. 2020, 25 (20), 4780. doi:10.3390/molecules25204780.
- Noser, A.A.; Abdelmonsef, A.H.; El-naggar, M.; Salem, M.M. New Amino Acid Schiff Bases as Anticancer Agents via Potential Mitochondrial Complex I-Associated Hexokinase Inhibition and Targeting AMP-Protein Kinases/mTOR Signaling Pathway. Molecules 2021, 26 (5332), 1–27. https://doi.org/10.3390/molecules26175332.
- Haredi Abdelmonsef, A.; Eldeeb Mohamed, M.; El-Naggar, M.; Temairk, H.; Mohamed Mosallam, A. Novel Quinazolin-2,4-Dione Hybrid Molecules as Possible Inhibitors Against Malaria: Synthesis and in Silico Molecular Docking Studies. Front. Mol. Biosci. Jun. 2020, 7 (105), 1–19. doi:10.3389/fmolb.2020.00105.
- El-Saghier, A.M.; El-Naggar, M.; Hussein, A.H.M.; El-Adasy, A.B.A.; Olish, M.; Abdelmonsef, A.H. Eco-Friendly Synthesis, Biological Evaluation, and In Silico Molecular Docking Approach of Some New Quinoline Derivatives as Potential Antioxidant and Antibacterial Agents. Front. Chem. 2021, 9 (679967), 1–14. doi:10.3389/fchem.2021.679967.
- El-Naggar, M.; Mohamed, M.E.; Mosallam, A.M.; Salem, W.; Rashdan, H.R.; Abdelmonsef, A.H. Synthesis, Characterization, Antibacterial Activity, and Computer-Aided Design of Novel Quinazolin-2,4-Dione Derivatives as Potential Inhibitors Against Vibrio Cholerae. Evol. Bioinforma. Jan. 2020, 16, 1–13. doi:10.1177/1176934319897596.
- Shehadi, I.A.; Rashdan, H.R.M.; Abdelmonsef, A.H. Homology Modeling and Virtual Screening Studies of Antigen MLAA-42Protein: Identification of Novel Drug Candidates Against Leukemia-an In Silico Approach. Comput. Math. Methods Med. 2020, 2020, 1–12. doi:10.1155/2020/8196147.
- Rashdan, H.R.M.; Abdelmonsef, A.H.; Shehadi, I.A.; Gomha, S.M.; Soliman, A.M.M.; Mahmoud, H.K. Synthesis, Molecular Docking Screening and Anti-Proliferative Potency Evaluation of Some New Imidazo[2,1-b]Thiazole Linked Thiadiazole Conjugates. Molecules 2020, 25 (21), 4997. doi:10.3390/molecules25214997.
- Rashdan, H.R.M.; Shehadi, I.A.; Abdelmonsef, A.H. Synthesis, Anticancer Evaluation, Computer-Aided Docking Studies, and ADMET Prediction of 1,2,3-Triazolyl-Pyridine Hybrids as Human Aurora B Kinase Inhibitors. ACS Omega 2021, 6, 1445–1455. doi:10.1021/acsomega.0c05116.
- Rashdan, H.R.M.; Abdelrahman, M.T.; Shehadi, I.A.; El-Tanany, S.S.; Hemdan, B.A. Novel Thiadiazole-Based Molecules as Promising Inhibitors of Black Fungi and Pathogenic Bacteria: In Vitro Antimicrobial Evaluation and Molecular Docking Studies. Molecules 2022, 27 (11), 3613.
- Shehadi, I.A.; Abdelrahman, M.T.; Abdelraof, M.; Rashdan, H.R.M. Solvent-Free Synthesis, In Vitro and In Silico Studies of Novel Potential 1, 3, 4-Thiadiazole-Based Molecules Against Microbial Pathogens. Molecules 2022, 27 (2), 342.
- El-Saghier, A.M.; Abd El-Halim, H.F.; Abdel-Rahman, L.H.; Kadry, A. Green Synthesis of new Trizole Based Heterocyclic Amino Acids Ligands and Their Transition Metal Complexes. Characterization, Kinetics, Antimicrobial and Docking Studies. Appl. Organomet. Chem. 2019, 33 (1), 1–18. doi:10.1002/aoc.4641.
- Chabner, B.A. NCI-60 Cell Line Screening: A Radical Departure in Its Time. J. Natl. Cancer Inst. 2016, 108 (5), 1–7. doi:10.1093/jnci/djv388.
- Holbeck, S.L. Analysis of FDA-Approved Anti-Cancer Agents in the NCI60 Panel of Human Tumor Cell Lines. Mol. Cancer 2011, 9 (5), 1451–1460. doi:10.1158/1535-7163.MCT-10-0106.Analysis.
- Brooks, B.R.; Brooks, C.L.; Mackerell, A.D.; Nilsson, L.; Petrella, R.J.; Roux, B.; Won, Y.; Archontis, G.; Bartels, C.; Boresch, S.; Caflisch, A.; Caves, L.; Cui, Q.; Dinner, A.R.; Feig, M.; Fischer, S.; Gao, J.; Hodoscek, M.; Im, W.; Kuczera, K.; Lazaridis, T.; Ma, J.; Ovchinnikov, V.; Paci, E.; Pastor, R.W.; Post, C.B.; Pu, J.Z.; Schaefer, M.; Tidor, B.; Venable, R.M.; Woodcock, H.L.; Wu, X.; Yang, W.; York, D.M.; Karplus, M. CHARMM: Molecular Dynamics Simulation Package. J. Comput. Chem. 2009, 30 (10), 1545–1614. doi:10.1002/jcc.21287.
- O’Boyle, N.M.; Banck, M.; James, C.A.; Morley, C.; Vandermeersch, T.; Hutchison, G.R. Open Babel: An Open Chemical Toolbox. J. Cheminform. 2011, 3 (10), 33. doi:10.1186/1758-2946-3-33.
- Rappé, A.K.; Casewit, C.J.; Colwell, K.S.; Goddard, W.A.; Skiff, W.M. UFF, a Full Periodic Table Force Field for Molecular Mechanics and Molecular Dynamics Simulations. J. Am. Chem. Soc. 1992, 114 (25), 10024–10035. doi:10.1021/ja00051a040.
- Dallakyan, S.; Olson, A.J. Small-Molecule Library Screening by Docking with PyRx. Methods Mol Biol. 2015, 1263, 243–250. doi:10.1007/978-1-4939-2269-7_19.
- Morris, G.M.; Goodsell, D.S.; Halliday, R.S.; Huey, R.; Hart, W.E.; Belew, R.K.; Olson, A.J. Automated Docking Using a Lamarckian Genetic Algorithm and an Empirical Binding Free Energy Function. J.: Comput. Chem. 1639, 19 (14), 1639–1662.
- Rashdan, H.R.M.; El-Naggar, M.; Abdelmonsef, A.H. Synthesis, Molecular Docking Studies and in Silico Admet Screening of new Heterocycles Linked Thiazole Conjugates as Potent Anti-Hepatic Cancer Agents. Molecules 2021, 26 (6), 1–17. doi:10.3390/molecules26061705.
- Lipinski, C.A.; Lombardo, F.; Dominy, B.W.; Feeney, P.J. Experimental and Computational Approaches to Estimate Solubility and Permeability in Drug Discovery and Development Settings. Adv. Drug Deliv. Rev. 1997, 23 (August), 3–25. doi:10.1016/S0169-409X(00)00129-0.