ABSTRACT
Nanoparticles of copper oxide were synthesized from the Spirodela polyrrhiza (greater duckweed) plant. Ultraviolet spectroscopy absorbance of around 400 nm was used for nanoparticle characterization. The presence of copper and oxygen in the biogenic nanoparticles was analyzed by the EDX technique. Based on scanning, and transmission electron microscopy (SEM and TEM) results, copper oxide nanoparticles (CuO NPs) are evenly distributed and spherical, and their sizes are in the 100 nm range. The synthesized nanoparticles of copper oxide were tested for antibacterial, antifungal, and nematicidal effectiveness against plants pest and pathogens. Antifungal and nematicidal activity against pests and plant pathogens was observed, with fungal mycelium reduced by 45–50% and eggs hatching. The findings suggest that plant-based copper oxide nanoparticles could be a potential source of plant pathogen inhibition. From this study, we concluded that synthesized CuO NPs have the potential to serve as a safer alternative for plant disease management.
GRAPHICAL ABSTRACT

Introduction
Nanotechnology has transformed agriculture and has the potential to control plant diseases (Citation1). Nano-agrochemicals are an emerging promising tool. They could be used to improve agriculture production and fill the global food demand (Citation2,Citation3). In nanotechnology, we could produce nanoparticles of desired shape and size using various methods (Citation4–6). The nanoparticles’ properties are based on size, morphology, and large surface area compared to bulk materials, making them highly sought after and widely used in recent years in numerous industries, such as agriculture and the environment (Citation3, Citation7–9), and even in the preparation of the nano steel synthesis (Citation10). In contrast to other methods, green manufacturing of nanoparticles (NPs) from plants obviates the need for sophisticated procedures. All over the world, medicinal plants are frequently used as complementary therapies for both physical and mental illnesses. Recently, nanoparticles have been synthesized using chemical, physical, and arc methods requiring direct current (DC) power supplies and commercially available copper rods (Citation11). However, biogenic synthesis, a well-accepted method of utilizing medicinal plant parts, has been used to produce biocompatible nanoparticles (Citation12–14) that improve biological functions. There has been an increasing interest in metal oxide nanoparticles for their antimicrobial and biocidal properties, such as copper oxide (CuO). They have multiple biomedical applications (Citation15,Citation16), as well as the development of sensors, catalysis, and semiconductors (Citation17–19). Green synthesis of nanomaterials is eco-friendly and plant materials are easily available, easy to handle, and economical and do not require a large number of chemicals or organic solvents. Green synthetic methods utilize a variety of waste materials such as dried leaves, fruit peels, seed coats, lemon rind, medicinal plants, algae, etc (Citation8, Citation20–22). Previously, CuO nanoparticles were produced using a variety of plant parts, including Ocimum sanctum (Citation23), Calotropisprocera (Citation15), Citrus limon, Turmeric curcumin (Citation16), Leucasaspera, Leucaschinensis (Citation17), Urtica and Matricariachamomill (Citation18).
Among all metal nanoparticles, CuO NPs are drawing significant attention recently due to their unique qualities compared to bulk copper and nano-copper have applications in agriculture, plant disease management, antibacterial and antifungal activities healthcare sectors, gas sensors, and high electrical conductivity (Citation18, Citation19, Citation24). Copper is an essential micronutrient and important for many enzyme activities in plants and a high amount of copper is occur in the chloroplast (Citation25, Citation26). The main disadvantage of using CuO nanoparticles (CuO NPs) in medicine is that they are potentially toxic to both vertebrate and invertebrate cells, as well as those of mammals. Even though CuO NPs have been used in biomedical applications, however, they can enhance cells’ production of reactive oxygen species of living organisms. Copper can be used in fertilizers, fungicides, and pesticides, and it is cheap material than silver or gold (Citation27–29). Chemical pesticides are primarily used in commercial agriculture to safeguard crops from pests and pathogens. We need to increase agriculture production without harming the environment because food demand increasing rapidly in an economized way is a global challenge. The field of nanobiotechnology may pave the new way to inhibit the growth of pests and pathogens. In the agriculture sector, nanotechnology has wide applications; it could improve crop productivity and could mitigate biotic and abiotic stresses (Citation26).
In this experiment, an attempt has been made to develop safer agrochemicals that could inhibit the attack of pests and pathogens. We evaluate the potential of copper oxide nanoparticles on the pathogenic fungus P. vexans and pathogenic gram negative bacterium Pectobacterium carotovorum (formerly Erwinia) and nematode Meloidogyne incognita. These test pathogens are highly destructive for important crops.
Experimental
Plant extract preparation
In this study, fresh 25 g of Spirodella polyrhiza leaves were cleaned with double distilled water. With 100 ml of distilled water, washed leaves were cooked for 10 min at 60°C. The mixtures were allowed to cool and filtered after boiling to collect an aqueous leaf extract. For later use, the extract was kept at 4°C. Many phytochemicals including proteins, alkaloids, carbohydrates, flavonoids, and polyphenols are present in the extract of Spirodela polyrrhiza (Citation30).
Biosynthesis of CuO NPs nanoparticle
Copper oxide nanoparticles were made by mixing Leaf extract (10 mL) with 90 mL of a 10 mM cupric sulfate solution (CuSO4.5H2O) (Sigma Aldrich, U.S.A.). At room temperature, the mixture was swirled until it turned black and indicated CuO NPs formation.
Characterization of synthesized nanoparticles
The size and morphology of the biosynthesized nanoparticles were studied using transmission and scanning electron microscopy (TEM and SEM). The elemental composition of biosynthesized CuO NPs was determined through an energy dispersion spectrum (EDS). Fourier transform infrared spectroscopy (FTIR) was used to investigate the phytonutrient involved in nanoparticle formation. UV-Vis spectroscopic analysis was used to characterize the nano copper surface plasmon resonance band. X-ray diffraction (XRD) was used to figure out the crystal structure of synthesized copper oxide nanoparticles.
Toxic effect of CuO NPs on Phomopsis vexans fungus
The fungus grew in PDA-medium Petri dishes at 25 °C for 15 days. CuO NPs were tested in vitro on P. vexans. After autoclaving, 10 mL of 100 g/mL CuO NPs solution was added to 500 mL of potato dextrose agar (PDA) medium and mixed. PDA with NPs and without NPs was inoculated with P. vexans. After 10 days in NPs mixed and unmixed medium, P. vexans grew differently.
Antibacterial activity of CuO NPs against Pectobacterium carotovorum bacteria
The disc diffusion method was used to test the antibacterial activity of synthesized CuO NPs against Pectobacterium carotovorum bacteria. Fresh bacterial cultures were plated on individual Nutrient Agar plates that had been sterile and softened. Then, sterile discs of 6 mm diameter were placed in a solution of copper oxide nanoparticles at a concentration of 100 μg/ml. The saturated discs were placed on the plates and incubated for 24 h at 30°C. One disc was used as a control and did not contain any CuO NPs. The inhibition zone developed in the discs was evaluated after incubation. To compare the results obtained from nanoparticles, streptomycin was used as a positive control.
Toxic effect on root-knot nematode bioassay
To ascertain the impact of CuO NPs on M. incognita mortality, we take a 20 ml suspension in which 5 ml 100μg/ml, suspension of CuO NPs and each Petri-plate was filled with 15 mL of distilled water. In each Petri-plate, 20 newly hatched J2 were placed. At 25 ± 1°C, the plates having a solution of nematodes and CuO NPs were allowed to incubate for 24 and 48 h intervals during this time, and a change in mortality was observed.
Egg hatching assay
A root-knot nematode known as M. incognita was found to be isolated from afflicted roots. Forceps were used to pick egg masses, which were then placed in sieves at 25 ± 2°C for hatching. For inoculation, second-stage juveniles (J2) were selected.
To perform the hatching assay, each Petri plate had a volume of 20 ml and was filled with a 5 ml suspension containing 100 μg/ml, which was combined with 15 ml of distilled water. Each petri dish contained five egg masses. As a control, a Petri dish containing five eggs along with 20 mL of double-distilled water. Effect on nematode hatching was determined for 24 and 48 h. Under the microscope, Juveniles that hatch from eggs was counted.
Results and discussion
UV-Vis spectroscopic analysis of CuO NPs
shows the UV-Vis absorption spectrum of the green synthesized CuO nanoparticles at wavelengths ranging from 200 to 600 nm. CuO nanoparticles were generated by using greater duckweed extract of leaves as a potential reducing agent, which has a peak of absorption at a wavelength of 400 nm. This peak can be attributed to CuO nanoparticles biosynthesized from plant extract (Citation31). In the UV-Vis spectrum, there was a broadened SPR peak, indicating the presence of polydisperse nanoparticles (Citation32).
Cu-NPs with diameters of 50–250 nm derived from Magnolia kobus leaf extract at room temperature (Citation33). These NPs were spherical, as evidenced by the peak in their UV-Vis spectrum at 400 nm. Kulkarni et al. (Citation34) used Eucalyptus sp. leaf extract to make CuO NPs from copper sulfate at room temperature and found UV-Vis absorbance at 572 nm.
EDX analysis of copper oxide nanoparticles
An EDX spectrum () confirms the presence of copper elements with carbon and oxygen. Leaf extract could contain secondary metabolites that cause aggregation.
SEM of copper oxide nanoparticles
shows SEM images of produced copper oxide nanoparticles. This environmentally benign approach revealed a nearly monodisperse distribution of particle sizes and surface morphology of CuO NPs. It mostly shows spherical CuO NPs and a large number of aggregates containing synthesized nanoparticles, with some nanoparticles having an unclear shape.
TEM analysis of CuO nanoparticles
Spherical dispersed nanoparticles of size 100 nm were discovered using transmission electron microscopy (TEM). The most common tool for determining the structure, size, morphology, dispersion, and orientation of biological and physical samples is the transmission electron microscope (TEM). The nano copper particles were found to have an average particle size of roughly 100 nm. depicts typical TEM images of nano copper, which have a spherical shape and a wide size dispersion.
FT-IR analysis of copper oxide nanoparticles
FT-IR spectrum of biogenic copper oxide nanoparticles revealed that phytochemicals, particularly phenolic compounds, as well as other functional groups found in plant extract, were the primary contributors to the formation of nanoparticles. The functional groups present in the extract act as reducing and limiting agents in the synthesis of stable nanoparticles. As shown in (a), asymmetric and symmetric alkanes, C–O ethers or esters, and hydrogen-bonded O–H stretching associated with alcohols and phenols have peaks at 1038, 1096, 1640, 2965, and 3291 cm−1, respectively. Furthermore, it depicts the FT-IR spectrum of CuO nanoparticles synthesized with greater duckweed. Finally, capping agents are used to regulate the size and diameter of nano copper particles.
The formation of copper oxide nanoparticles through the involvement of bioactive molecules present in extracts, as well as their role in the bioreduction of copper and the isolation of phytochemical compounds have been documented in the literature (Citation9,Citation22,Citation35). Based on the literature and IR spectrum, the proposed mechanism explains the three phases of activation, growth, and termination in the presence of biomolecules to support the production and stabilization of CuO NPs during process biosynthesis. The tentative mechanism was sketched out to explain the formation of CuO NPs as shown in . HPLC-DAD/MS-MS was used to confirm the presence of biomolecules in the extract as well as to identify some phenolic compounds (Citation36) that have been documented in the literature and may be involved in the production of nanoparticles.
XRD spectrum
XRD analysis was used to determine the crystallinity of the biosynthesized CuO NPs, which produced a variety of peaks patterns in the XRD spectrum ((b)). Diffraction sharp peaks were found at 2θ = 33.01, 35.25, 38.75, 48.09, 61.37, and 66.01, with miller indices planes of 110, -111, 111, -202, 113, and 311, respectively, corresponding to JCPDS-01-080-0076 and ICSD-087122 with monoclinic phase and face-centered cubic CuO NPs. XRD patterns of copper nanoparticles show sharp and high-intensity peaks at de Bragg reflection angles associated with two lattice planes (−111) and (111). These distinctive peaks indicate that the synthesized nanoparticles of copper are crystalline. Using the Debye-Scherrer formula, the crystallite size of biogenic CuO NPs with a sharp peak was determined to be 7.44 nm, indicating its crystalline nature. There were a few unidentified peaks in this sample, which may imply fewer biochemicals. As well, Nasrollahzadeh et al. (Citation37) produced crystalline copper nanoparticles from Euphorbia L leaf extracts (aqueous extract). The biosynthesized nanoparticles were analyzed with XRD, and the results showed that a significant amount of nano copper crystals were present in the sample.
Antibacterial activity of CuO NPs
The antibacterial action of nanoparticles against pathogens was studied, and the inhibition zone surrounding the loaded disc with CuO NPs was examined, and calculated (,). From the results, CuO NPs were found to be active against the bacterium, exhibiting an inhibition zone but not efficacious compared to the reference drug (streptomycin, 10 µg/disc, zone of inhibition 8.5 mm) (Citation38). The particle size of nanoparticles would be thought to affect their antibacterial activity. In our study, particles with a diameter of 100 nm were used to evaluate the bacterial study. However, the pharmaceutical industry is now placing more and more emphasis on research into unconventional antioxidants and antibacterial agents. Biosynthesized CuO NPs generated from the flower extract of Millettia pinnata were tested against several pathogenic bacteria. Thiruvengadam et al. (Citation39) noticed that these particles were more effective at killing Staphylococcus aureus and Bacillus subtilis than Pseudomonas aeruginosa and E. coli. CuO NPs derived from gum karaya demonstrated extremely potent antibacterial activity against E. coli and S. aureus (Citation40,Citation41). Efficiency is believed to depend on the size of the NPs (Citation38). CuO NPs obtained from an aquatic noxious weed, Eichhorniacrassipes, were found to act as a growth inhibitor of Fusarium culmorum and Aspergillus niger (Citation42). In a similar study, Sivaraj et al. (Citation43) proved that CuO NPs derived from Tabernaemontana divaricate leaves were highly effective against E. coli. E. coli, Enterococcus sp., Proteus sp., and Klebsiella sp. are all inhibited by CuO NPs ().
Figure 7. Antibacterial activity; A-Inhibition zone is absent (Untreated) B-Inhibition zone is present (Treated with CuO NPs).
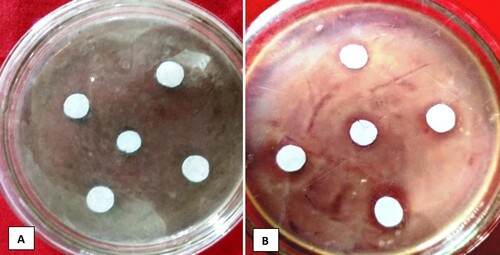
Table 1. Antimicrobial activity of CuO NPs.
Table 2. Recent research on plant-mediated CuO NPs synthesis.
Cuo NPs effect on egg hatching and mortality under in vitro
Direct contact with CuO NPs caused M. incognita in water to exhibit toxic effects that were fatal ( and ). The effect gets stronger as exposure time and concentration increase. CuO NPs also prevent egg hatching. Maximum hatching took place in double-distilled water at 24 and 48 h (DDW). After 48 h, DD Water contained the fewest dead nematodes. The egg hatching was observed to be diminishing with increasing time-based on CuO NPs applications.
Table 3. Effect of CuO NPs on Meloidogyne incognita.
Effect of CuO NPs on fungi
In an in-vitro test, P. vexans growth was significantly reduced in potato dextrose medium with 100μg/ml CuO NPs compared to the control (without NPs) (,). The antifungal effect of CuO NPs on P. vexans mycelium was investigated using agar diffusion. depicts the in vitro effects of CuO NPs on mycelia growth ((B)) in comparison to the control (without CuO NPs) ((A)). P. vexans growth was reduced by 85% in the PDA medium with 54 ppm CuO NPs compared to the control (without NPs) (). The fungal mycelium was disturbed by the synthesized CuO NPs, according to microscopic and SEM studies (). The prior work provided an explanation of the calculation for the inhibition of fungal growth (Citation1,Citation7,Citation47).
Conclusion
The morphology, size, and bioactive molecules involved in NP formation were confirmed by various spectroscopic techniques after the successful production of approximately 100 nm CuO NPs using Spirodella polyrhiza leaves. The biogenic CuO NPs inhibited plant pathogenic bacterium P. carotovorum, fungus, P. vexans, and root-knot nematode M. incognita with a 2–3 mm inhibition zone, 45-50% inhibition of fungal mycelium growth, and significantly reduced hatching at 24 and 48 h, respectively. As a result of this study, it was determined that green synthesized CuO nanoparticles mediated by Spirodella polyrhiza leaf extract exhibit strong pathogen inhibitory properties. Biosynthesized CuO NPs need to be tested against various pathogenic strains at various concentrations to conclude the finding of copper oxide nanoparticles and their biocompatibility with living organisms. However, biosynthesis of CuO NPs is a green, inexpensive, eco-friendly, reliable, and safe method that can be used for human benefit.
Disclosure statement
No potential conflict of interest was reported by the author(s).
Additional information
Funding
References
- Khan, A.U.; Malik, N.; Khan, M.; Cho, M.H.; Khan, M.M. Fungi assisted silver nanoparticles synthesis and their applications. Bioprocess Biosyst. Eng. 2018, 41, 1–20.
- El-Shetehy, M.; Moradi, A.; Maceroni, M.; Reinhardt, D.; Petri-Fink, A.; Rothen-Rutishauser, B.; Mauch, F.; Schwab, F. Silica nanoparticles enhance disease resistance in Arabidopsis plants. Nat. Nanotechnol. 2021, 16, 344–53.
- Buazar, F. Impact of biocompatible nanosilica on green stabilization of subgrade soil. Sci. Rep. 2019, 9, 15147.
- Grigore, M.E.; Biscu, E.R.; Holban, A.M.; Gestal, M.C.; Grumezescu, A.M. Methods of synthesis, properties and biomedical applications of CuO nanoparticles. Pharmaceuticals 2016, 9, 75.
- Buazar, F.; Bavi, M.; Kroushawi, F.; Halvani, M.; Khaledi-Nasab, A.; Hossieni, S.A. Potato extract as reducing agent and stabiliser in a facile green one-step synthesis of ZnO nanoparticles. J. Exp. Nanosci. 2016, 11, 175–184.
- Buazar, F.; Baghlani-Nejazd, M.H.; Badri, M.; Kashisaz, M.; Khaledi-Nasab, A.; Kroushawi, F. Facile one-pot phytosynthesis of magnetic nanoparticles using potato extract and their catalytic activity. Starch-Starke 2016, 68, 796–804.
- Khan, A.U.; Khan, M.; Malik, N.; Cho, H.M.; Khan, M.M. Recent progress of algae and blue–green algae-assisted synthesis of gold nanoparticles for various applications. Bioprocess Biosyst. Eng. 2019, 42, 1–15.
- Moavi, J.; Buazar, F.; Sayahi, M.H. Algal magnetic nickel oxide nanocatalyst in accelerated synthesis of pyridopyrimidine derivatives. Sci. Rep. 2021, 11, 6296.
- Buazar, F.; Sweidi, S.; Badri, M.; Kroushawi, F. Biofabrication of highly pure copper oxide nanoparticles using wheat seed extract and their catalytic activity: a mechanistic approach. Green Process. Synth. 2019, 8, 691–702.
- Buazar, F.; Cheshmehkani, A.; Kassaee, M. Nanosteel synthesis via arc discharge: media and current effects. J. Iran. Chem. Soc. 2012, 9, 151–156.
- Kassaee, M.Z.; Buazar, F.; Motamedi, E. Effects of current on arc fabrication of Cu nanoparticles. J. Nanomater 2010, 2010, 1–5.
- Alavi, M.; Thomas, S.; Sreedharan, M. Modification of silica nanoparticles for antibacterial activities: mechanism of action. Micro. Nano Bio. Aspects 2022, 1, 49–58.
- Alavi, M.; Kowalski, R.; Capasso, R.; Coutinho, H.D.M.; de Menezes, A. Various novel strategies for functionalization of gold and silver nanoparticles to hinder drug-resistant bacteria and cancer cells. Micro. Nano Bio. Aspects 2022, 1, 38–48.
- Ahmadi, S.; Ahmadi, G.; Ahmadi, H. A review on antifungal and antibacterial activities of some medicinal plants. Micro Nano Bio. Aspects 2022, 1, 10–17.
- Harne, S.; Sharma, A.; Dhaygude, M.; Joglekar, S.; Kodam, K.; Hudlikar, M. Novel route for rapid biosynthesis of copper nanoparticles using aqueous extract of Calotropisprocera L. latex and their cytotoxicity on tumor cells. Colloid Surf. B 2012, 95, 284–8.
- Jayandran, M.; Haneefa, M.M.; Balasubramanian, V. Green synthesis of copper nanoparticles using natural reducer and stabilizer and an evaluation of antimicrobial activity. J. Chem. Pharm. Res. 2015, 7, 251–9.
- Hase, G.J.; Bharati, K.T.; Deshmukh, K.K.; Phatangre, N.D.; Rahane, A.; Dokhe, S. Synthesis and characterization of Cu nanoparticles of Leucas chinensis L. plant. Eur. J. Pharm. Med. Res. 2016, 3, 241–2.
- Mane, A.; Patil, N.; Gaikwad, S.S. Extracellular synthesis of copper nanoparticles using different plant extract. Int. J. Appl. Nat. Sci. 2016, 5, 33–38.
- Jain, S.; Jain, A.; Kachhawah, P.; Devra, V. Synthesis and size control of copper nanoparticles and their catalytic application. Trans. Nonferrous Met. Soc. China 2015, 25, 3995–4000.
- Siddiqi, K.S.; Husen, A. Current status of plant metabolite-based fabrication of copper/copper oxide nanoparticles and their applications: a review. Biomater. Res. 2020, 24, 1–5.
- Khan, A.U.; Khan, M.; Malik, N.; Cho, H.M.; Khan, M.M. Recent progress of algae and blue–green algae-assisted synthesis of gold nanoparticles for various applications. Bioprocess Biosyst. Eng. 2019, 42, 1–15.
- Sepahvand, M.; Buazar, F.; Sayahi, M.H. Novel marine-based gold nanocatalyst in solvent-free synthesis of polyhydroquinoline derivatives: green and sustainable protocol. Appl. Organomet. Chem. 2020, 34, e6000.
- Shende, S.; Gaikwad, N.; Bansod, S. Synthesis and evaluation of antimicrobial potential of copper nanoparticle against agriculturally important Phytopathogens. Synthesis 2016, 1, 41–47.
- Din, M.I.; Arshad, F.; Hussain, Z.; Mukhtar, M. Green adeptness in the synthesis and stabilization of copper nanoparticles: catalytic, antibacterial, cytotoxicity, and antioxidant activities. Nano Res. Lett. 2017, 12, 638.
- Rai, M.; Ingle, A.P.; Pandit, R.; Paralikar, P.; Shende, S.; Gupta, I.; Biswas, J.K.; da Silva, S.S. Copper and copper nanoparticles: Role in management of insect-pests and pathogenic microbes. Nanotechnol. Rev. 2018, 7, 303–15.
- Kasana, R.C.; Panwar, N.R.; Kaul, R.K.; Kumar, P. Biosynthesis and effects of copper nanoparticles on plants. Environ. Chem. Lett. 2017, 15, 233–40.
- Borkow, G.; Gabbay, J. Copper, an ancient remedy returning to fight microbial, fungal and viral infections. Cur. Chem. Biol. 2009, 3, 272–8.
- Zheng, X.G.; Xu, C.N.; Tomokiyo, Y.; Tanaka, E.; Yamada, H.; Soejima, Y. Observation of charge stripes in cupric oxide. Phys. Rev. Lett. 2000, 85, 5170–3.
- Ren, G.; Hu, D.; Cheng, E.W.; Vargas-Reus, M.A.; Reip, P.; Allaker, R.P. Characterization of copper oxide nanoparticles for antimicrobial applications. Int. J. Antimicrob. Agent 2009, 33, 587–90.
- Das, B.K.; Das, D.P.; Pradhan, J.Y.; Priyadarshinee, B.A.; Sahu, I.P.; Roy, P.R.; Mishra, B.K. Evaluation of antimicrobial activity and phytochemical screening of ethanolic extract of greater duckweed, Spirodelapolyrrhiza. Int. J. Pharma Bio Sci. 2012, 3, 822–33.
- Ashtaputrey, S.D.; Ashtaputrey, P.D.; Yelane, N. Green synthesis and characterization of copper nanoparticles derived from Murrayakoenigii leaves extract. Int. J. Chem. Pharm. Sci. 2017, 10, 1288–1291.
- Ramyadevi, J.; Jeyasubramanian, K.; Marikani, A.; Rajakumar, G.; Rahuman, A.A. Synthesis and antimicrobial activity of copper nanoparticles. Mater. Lett. 2012, 71, 114–116.
- Lee, H.J.; Song, J.Y.; Kim, B.S. Biological synthesis of copper nanoparticles using Magnolia kobus leaf extract and their antibacterial activity. J. Chem. Technol. Biotechnol. 2013, 88, 1971–7.
- Kulkarni, V.; Suryawanshi, S.; Kulkarni, P. Biosynthesis of copper nanoparticles using aqueous extract of Eucalyptus sp. plant leaves. Curr. Sci. 2015, 109, 255–27.
- Koopi, H.; Buazar, F. A novel one-pot biosynthesis of pure alpha aluminum oxide nanoparticles using the macroalgae Sargassum ilicifolium: A green marine approach. Ceram. Int. 2018, 44, 8940–8945.
- Pagliuso, D.; Palacios Jara, C.E.; Grandis, A.; Lam, E.; Pena Ferreira, M.J.; Buckeridge, M.S. Flavonoids from duckweeds: potential applications in the human diet. RSC Adv. 2020, 10, 44981–44988.
- Nasrollahzadeh, M.; Sajadi, S.M.; Khalaj, M. Green synthesis of copper nanoparticles using aqueous extract of the leaves of Euphorbia esula L and their catalytic activity for ligand-free Ullmanncoupling reaction and reduction of 4-nitrophenol. RSC Adv. 2014, 4, 47313–47318.
- Nezhad, M.H.; Alamshahi, L.; Panjehkeh, N. Biocontrol efficiency of medicinal plants against Pectobacterium carotovorum. Ralstonia solanacearum and Escherichia coli. Open. Conf. Proc. J. 2012, 3, 46–51.
- Thiruvengadam, M.; Chung, I.M.; Gomathi, T.; Ansari, M.A.; Khanna, V.G.; Babu, V.; Rajakumar, G. Synthesis, characterization and pharmacological potential of green synthesized copper nanoparticles. Bioprocess Biosyst. Eng. 2019, 42, 1769–77.
- Khani, R.; Roostaei, B.; Bagherzade, G.; Moudi, M. Green synthesis of copper nanoparticles by fruit extract of Ziziphusspina-christi (L.) Willd: application for adsorption of triphenylmethane dye and antibacterial assay. J. Mol. Liq. 2018, 255, 541–9.
- Azam, A.; Ahmed, A.S.; Oves, M.; Khan, M.S.; Memic, A. Size-dependent antimicrobial properties of CuO nanoparticles against gram-positive and -negative bacterial strains. Int. J. Nanomed. 2012, 7, 3527–35.
- Vanathi, P.; Rajiv, P.; Sivaraj, R. Synthesis and characterization of Eichhornia mediated copper oxide nanoparticles and assessing their antifungal activity against plant pathogens. Bull. Mater. Sci. 2016, 39, 1165–70.
- Sivaraj, R.R.; Rajiv, P.K.; Salam, P.; A, H.; Venckatesh, R. Biogenic copper oxide nano-particles synthesis using Tabernaemontana divaricate leaf extractand its antibacterial activity against urinary tract pathogen. Spectrochim. Acta A Mol. Biomol. Spectrosc. 2014, 133, 178–181.
- Jadhav, M.S.; Kulkarni, S.; Raikar, P.; Barretto, D.A.; Vootla, S.K.; Raikar, U.S. Green biosynthesis of CuO & Ag–CuO nanoparticles from Malus domestica leaf extract and evaluation of antibacterial, antioxidant and DNA cleavage activities. New J. Chem. 2018, 42, 204–13.
- Khatami, M.; Varma, R.S.; Heydari, M.; Peydayesh, M.; Sedighi, A.; Askari, H.A.; Rohani, M.; Baniasadi, M.; Arkia, S.; Seyedi, F.; Khatami, S. Copper oxide nanoparticles greener synthesis using tea and its antifungal efficiency on Fusariumsolani. Geomicrobiol. J. 2019, 36, 777–81.
- Akhter, S.M.H.; Mohammad, F.; Ahmad, S. Terminalia belerica mediated green synthesis of nanoparticles of copper, iron and zinc metal oxides as the alternate antibacterial agents against some common pathogens. BioNanoSci 2019, 9, 365–72.
- Mani, V.M.; Kalaivani, S.; Sabarathinam, S.; Vasuki, M.; Soundari, A.J.P.G.; Das, M.A.; Elfasakhany, A.; Pugazhendhi, A. ; Copper oxide nanoparticles synthesized from an endophytic fungus aspergillus terreus: bioactivity and anti-cancer evaluations. Environ. Res. 2021, 201, 111502.