ABSTRACT
The large-enrollment undergraduate organic chemistry laboratories at Michigan State University have been redesigned to incorporate green and sustainable chemistry and evidence-based instructional approaches to laboratory teaching. Students engage in scientific practices such as defining problems, designing and carrying out experiments, analyzing data, and designing solutions during in-person lab sessions. Principles of green and sustainable chemistry are introduced in the case studies, where students investigate a variety of approaches and discuss the relative merits of different approaches from a range of perspectives including efficiency, environmental impact, and cost. An in-depth summary of design pedagogy, instructional materials, implementation details, instructor’s notes and challenges will be discussed.
GRAPHICAL ABSTRACT
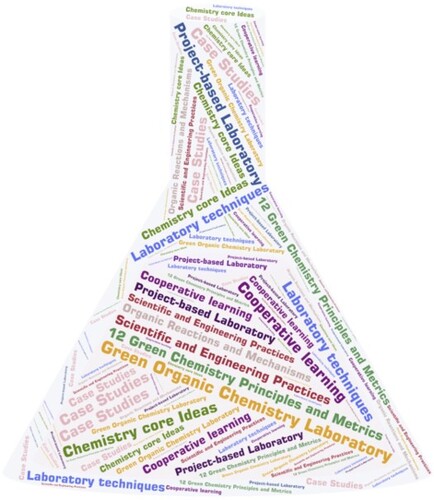
Introduction
For people in the United States in the mid-twentieth century, national crises related to the environment – such as the leaching of chemical waste at the Love Canal in Niagara Falls, NY or the burning of the Cuyahoga River in Ohio – spurred action from both governmental organizations in the form of prominent reports (Anastas & Warner, 1998 (Citation1)) and activism from grassroots organizations for environmental justice, such as the community outrage over the dumping of polychlorinated biphenyls (PCBs) in a predominantly African-American area of Warren County, North Carolina (Citation2). The public pressure and expense to governmental organizations for the remediation of these events led to legislation to regulate the manufacture, use, and disposal of chemical substances. In response to the Pollution Prevention Act of 1990, the field of Green Chemistry was developed by the U.S. Environmental Protection Agency (EPA) to design and produce cost-competitive chemical products and processes that attain the highest level of the pollution-prevention hierarchy by reducing pollution at its source instead of eliminating pollution after disposal (Citation3).
As outlined in , one consequence of this decade of innovation in the strategy of pollution prevention was the development of the 12 Principles of Green Chemistry, published by Anastas and Warner in 1998 (Citation1). These principles represent considerations and strategies to guide the practice of professional chemists, as well as suggestions of analytical tools that chemists should employ to analyze and optimize a chemical process.
Table 1. The 12 Principles of Green Chemistry proposed by Anastas and Warner (Citation1).
Green Chemistry is a paradigm shift in professional practice: it has the attention and support of academic chemists and is a desirable skillset for the industrial workforce as the United States aims to meet its goals for mitigation of climate change and pollution reduction. This pressing need for change also underscores the ambitious and critical Sustainable Development Goals (SDGs) adopted by the United Nations. To achieve collective action towards these SDGs, both green and sustainable chemistry education and chemical manufacturing practices are imperative. However, incorporation of these necessary knowledge performances into the United States higher education chemistry curriculum is slow to spread and centered on several nucleation sites of innovation. According to the American Chemical Society, only 47 higher-ed institutions offer green chemistry or green engineering programs in the U.S. (Citation4). Despite 105 higher-ed institutions having signed on to the Green Chemistry Commitment with Beyond Benign as of today (Citation5), an organization dedicated to fostering a green chemistry community that empowers educations to transform chemistry education for a sustainable future, and the proliferation of green chemistry related job markets and networks over the past decade. These offerings predominantly take the form of curricular add-ons, such as elective courses or select sections of certain laboratory courses. This approach implies that green chemistry is an add-on or supplement, rather than a necessary way of thinking to guide scientists in the twenty-first century. An in-class survey given to MSU STEM major students taking organic chemistry laboratory courses in the 2021 summer semester suggests that many students expressed interest in green chemistry related topics and were eager to learn more:
I know green chemistry has to do with environmental chemistry regarding the management and reduction of hazardous wastes. I think it is an interesting concept, aspiring to enter the medical field. I think it could apply to pharmaceutical production and would like to learn more.
Course design
Much of recent research on learning in STEM (Citation8, Citation9) has focused on the idea that simply knowing something is insufficient, and that we must support students to use knowledge in appropriate contexts, using the Scientific and Engineering Practices (SEPs) (Citation8). Green Cooperative Organic Laboratory was designed around A Framework for K-12 Science Education and the eight scientific and engineering practices which describes ‘behaviors that scientists engage in as they investigate and build models and theories about the natural world and the key set of engineering practices that engineers use as they design and build models and systems.’ (Citation8) The eight scientific and engineering practices are: Ask Questions; Develop and Use Models; Plan and Carry out Investigations; Analyze and Interpret Data; Use Mathematics and Computational Thinking; Construct Explanations; Engage in Argument from Evidence – including dialogue; Obtain, Evaluate, and Communicate Information.
To align with this approach, this project has positioned green and sustainable chemistry not as a set of principles that students must learn, but rather as a set of decisions that a scientist must make, guided by their understanding of core chemistry ideas and the particular context and goals within which they are working. From this perspective, there is a necessary balance to be achieved between the phenomenon of interest (the experiment or socio-scientific issue) and the elicitation of student knowledge and ability to use that knowledge to demonstrate their understanding of what is green or sustainable. ‘Green’ is a comparative concept since there is always a way to make it ‘greener,’ by using less energy, greener solvents, or achieving a higher atom economy; therefore, students must have a comparative baseline measurement of greenness. Often published curricula and laboratory (Citation10–13) activities designed to teach green chemistry feature greener reaction schemes, with the instructor making the final decision on trade-offs such as solvent selection and avoiding producing hazardous by-products. In some sense, the actual green chemistry – all the decisions and analysis – have been completed long before the students enrolled in the course set foot in the lab. Therefore, such activities are unlikely to promote students decision making. Just as with most verification-style ‘cookbook’ courses, laboratory activities are designed with little space for students to explore ideas or design and carry out investigations.
This curriculum is designed to promote a more flexible approach to green chemistry by supporting students as they use their chemistry knowledge to make green and sustainable chemistry decisions. The primary method of accomplishing this is through engaging students in Scientific and Engineering Practices, adopted from the A Framework for K-12 Science Education (Citation8). These SEPs are embedded in the scaffolded prompts throughout the curriculum, as demonstrated in .
Table 2. Scientific and engineering practices in Green Cooperative Organic Chemistry Laboratory.
Cooperative laboratory projects based on real-life scenarios
In the Green Cooperative Organic Chemistry Laboratory, students work in small teams of 4 on multi-week projects to investigate green chemistry related questions or to propose the best solution based on a real-life scenario provided to them. In each laboratory project, students are provided with procedural details of 3–4 synthetic routes towards the final goal, just like what would happen in a research laboratory or in the industry. Students usually follow the steps in while completing a project. Each lab project also provides students the opportunity to communicate the final conclusion using lab report, oral presentation or poster presentation format, as shown in the ‘Assessment’ column of .
Table 3. A summary of the weekly tasks, example prompt questions, laboratory techniques used, green chemistry concept involved, and assessment used in each lab project in Green Cooperative Organic Chemistry Laboratory.
Using project 2 as an example, the project scenario is framed such that the students are told that their research team accepted a task from a start-up company looking for a green alternative synthesis of aspirin to satisfy Environmental Protection Agency (EPA) requirements and shareholders. Their team is tasked with exploring ways to synthesize aspirin using four different catalysts. The best solution should strike a balance between the highest yield, the ‘greenest’ conditions, and being the most cost-effective synthesis. Students report their solution in an evidence-based argument in a formal lab report. Compared to the abstract theories delivered in the lecture, cooperative lab offers students a unique experience in developing laboratory and instrumental skills, working as part of a team, and more importantly, engaging in scientific practices and decision-making processes. This aligns with the course learning objectives: students will gain experience with the following activities that are central to the work of science, providing a foundation for future experimental work and scientific investigation. A summary of weekly tasks, example prompt questions, laboratory techniques used, green chemistry concept involved, and assessment used in each lab project is included in .
Cooperative case studies investigating real-world sustainability issues
While the cooperative project-based labs engage students in green and sustainable chemistry at the bench level, the case study sessions serve as the primary mode through which students grapple with green and sustainable chemistry issues in a broader context. Case studies are conducted outside of the laboratory, allowing students to focus on each task separately and mitigating safety concerns of the laboratory. Although case studies are completed asynchronously from the lab projects, efforts have been made to align lab project and case study topics. For example, case study one focuses on the synthesis of an amide-containing molecule while lab project two highlights a mechanistically similar ester synthesis reaction. The goal for these case studies is two-fold: (1) to present information about the relevant principles of green chemistry; and (2) to encourage students to use their chemistry knowledge to engage in green decision-making. Each case study presents students with a specifically designed problem that incorporates appropriately scaffolded chemistry knowledge, green chemistry tools, and experimental data to help students understand what it means for something to be ‘green.’ Through consideration of the relevant stakeholders, criteria, and constraints with each scenario, students actively engage in the use of green chemistry ideas by designing solutions to each real-world problem.
Case study development follows an evidence-centered design approach in which iterative changes are made to the prompt based on prior student responses. These changes are intended to improve scaffolding that helps support students as they use their chemistry knowledge to engineer solutions to sustainability issues (Citation14). Each case study was designed following the framework described in , with individual case studies described specifically in .
Table 4. Summary of weekly tasks, green chemistry involved, and assessment for each cooperative case study.
The first part of each case study was designed to describe the scenario and provide students with the prerequisite knowledge needed to define the problem and design an acceptable solution. Through purposeful scaffolding of conceptual resources, students construct explanations for the molecular-level interactions that underpin the sustainability issues being addressed in each case study. The second part of each case study highlights the specific sustainability issue and prompts students to define the problem to which they will propose a solution. By providing the relevant data and green chemistry tools, students are asked to evaluate potential solutions to the problem at hand. The final part of each case study culminates in students designing a solution to the problem and generating an evidence-based argument to formally communicate their solution. Each case study also provides students the opportunity to communicate this solution using a different modality as shown in the ‘Assessment’ column of .
Challenges for project-based labs and cooperative learning
The transformation from cookbook style labs to project-based labs featuring green chemistry has not been without its challenges. The Green Cooperative Organic Chemistry Laboratory course at MSU serves approximately 1200 students in STEM majors per year. Each semester, 15 graduate teaching assistants (GTAs) supervise 2 sections of as many as 20 students assigned to 5 teams at a time. Coordinating so many students and associated GTAs is a challenge in itself.
The second major challenge was that students were so used to following explicit instructions on the lab manual instead of designing their own experiment as a team towards the project goal. Cooperative General Chemistry Laboratory and Green Cooperative Organic Chemistry Laboratory are the only introductory laboratory courses based on project-based cooperative modules at MSU. Carrying out the experiment successfully required mastery of relevant laboratory techniques, in-depth understanding of the chemistry concepts behind it and lab practices, and ability to troubleshoot. Assessments of the laboratory activities included both written reports and oral/poster presentations. Presenting scientific evidence, making argumentation, and conveying experimental results in front of their peers takes courage and practice, especially for students who took online courses during the pandemic and were still adjusting to the in-person labs.
Implications for instructors
There have been many major changes since the Green Cooperative Organic Chemistry Laboratory course was first implemented in Fall 2021 semester. Weekly video & quiz bundles replaced the final exam to better prepare students. Smaller goals with prompt feedback are easier to achieve and less intimidating than a big final goal. The individual-to-group assignment ratio increased significantly to encourage individual participation and motivation. Students rotate between different roles for each project, including team leader, recorder, communicator, and safety officer. In this way, they get to develop well-rounded abilities in different positions and learn to work towards the same goal as a group. An example planning document is included in the supporting information. A peer evaluation system was built into each lab project and case study to provide more guidance to students for better communication and more efficient collaboration.
As cooperative learning requires more active engagement and decision-making for students, the bar is raised for instructors as well. Course instructors or GTAs are expected to offer appropriately scaffolded guidance to students’ planning new projects. Variations in students’ choices and decisions lead to individualized outcomes. Special GTA training sessions are offered to focus on:
Group planning and in-person experimental sessions. Project-based labs require GTAs to be more prepared and flexible. It is important that students ‘do science’ as a group rather than following instructions from the instructor. Time management ability is also necessary.
Resolving group conflicts. Instructors are not just mediators. What is more important is that students evolve into their own roles while working in a group and learn to contribute and work collaboratively under GTAs’ guidance.
Grading and assessments. How can grades reflect students’ contribution and participation in a more equitable manner? Adjusting and reinforcing the grading rubric is recommended. An example rubric is included in the supporting information.
Feedback from GTAs, students, and faculty suggests that both lab-specific training and weekly meetings have made a positive impact on course instruction and their professional development.
Implications for researchers
Researchers, in green and sustainable chemistry, who wish to incorporate their research and expertise into their teaching, may have an understandable desire to show students the newest and ‘coolest’ breakthroughs in green and sustainable chemistry. However, if these breakthroughs are to have the largest impact on the students, the curricular materials should be based on a sound theory of learning. A particularly accessible theory of learning, 3-Dimensional Learning (3DL) leads to the development of curricular materials that encourage students to think and act like experts (Citation15). Development of curricular resources is not a one and done process. Just as the initial standards for evaluating the ‘green-ness’ began with marginal improvements, further refinements of the standards have led to substantial improvements. The processes outlined here are the educational research equivalent to those iterative improvements. By comparing ideal student performances/outcomes to real-world student performances/outcomes, refinements to curricular materials and assessment prompts can help close the gap.
For discipline-based educational researchers, we encourage the framing of green and sustainable chemistry as a lens and set of values to aid in the decision-making process. This approach should ease the integration of new curricular materials with existing materials and avoid the appearance of add-ons or ancillary goals. While the authors advocate for the use of 3DL from the Next Generation Science Standards (NGSS) as a foundational theory of learning, the focus on green and sustainable chemistry in decision-making processes can be integrated with the researcher’s learning theory of choice (Citation16,Citation17).
Conclusions
The Green Cooperative Organic Chemistry Laboratory course was designed for STEM major students, emphasizing cooperative learning and green chemistry. We aim to educate students to think and make decisions using green chemistry knowledge as lenses and tools with the hope that this green chemistry seed we plant in students’ minds will flourish in their future decision making. It takes collaborative efforts to cultivate a culture towards a greener and more sustainable society.
Supplemental Material
Download MS Word (81.3 KB)Acknowledgements
Any opinions, findings, conclusions, or recommendations expressed here are those of the authors and do not necessarily reflect the views of both funding sources. The implementation of the reformed CEM 255 course would not have been possible without the support and feedback from teaching assistants and students in fall semester 2021 and spring semesters 2022. Special thanks go to Dr. Natalie O'Neil and Gustavo Casanova.
Disclosure statement
No potential conflict of interest was reported by the author(s).
Additional information
Funding
References
- Anastas, P.T.; Warner, J.C. Green Chemistry: Theory and Practice; Oxford University Press: Oxford, UK, 1998.
- Cole, L.W.; Foster, S.R. From the Ground Up: Environmental Racism and the Rise of the Environmental Justice Movement; NYU Press: New York City, NY, 2001.
- United States Environmental Protection Agency. Chemicals under the Toxic Substances Control Act (TSCA). https://www.epa.gov/chemicals-under-tsca (accessed Nov 29, 2022).
- Schools with Green Chemistry and Green Engineering Programs. American Chemical Society. https://www.acs.org/content/acs/en/greenchemistry/students-educators/academicprograms.html (accessed Nov 29, 2022).
- About Mission & Vision. https://www.beyondbenign.org/about-mission-vision/ (accessed Jan 1, 2023).
- Cooper, M.M. Cooperative Organic Laboratories. J. Chem. Educ 1995, 72 (6), 534. doi:10.1021/ed072p534.1.
- Bretz, S.L. Evidence for the Importance of Laboratory Courses. J. Chem. Educ. 2019, 96 (2), 193–195. doi:10.1021/acs.jchemed.8b00874.
- National Research Council. A Framework for K-12 Science Education: Practices, Crosscutting Concepts, and Core Ideas; 2011. doi:10.17226/13165.
- National Research Council. Discipline-Based Education Research: Understanding and Improving Learning in Undergraduate Science and Engineering; 2012. doi:10.17226/13362.
- Doxsee, K.M.; Hutchison, J.E. Green Organic Chemistry: Strategies, Tools, and Laboratory Experiments; Thomson-Brooks/Coles: New York City, NY, 2004.
- Roesky, H. W.; Kennepohl, D. K. Experiments in Green and Sustainable Chemistry; Wiley-VCH: Weinheim, 2009
- Henrie, S.A. Green Chemistry Laboratory Manual for General Chemistry; 1st ed. CRC Press: Boca Raton, 2015.
- Dicks, A.P. Green Organic Chemistry in Lecture and Laboratory; CRC Press: Boca Raton, FL, 2016.
- Mislevy, R.J.; Almond, R.G.; Lukas, J.F. A Brief Introduction to Evidence-Centered Design. ETS Research Report Series 2003, (1), i–29. doi:10.1002/j.2333-8504.2003.tb01908.x.
- Cooper, M.M.; Caballero, M.D.; Ebert-May, D.; Fata-Hartley, C.L.; Jardeleza, S.E.; Krajcik, J.S.; Laverty, J.T.; Matz, R.L.; Posey, L.A.; Underwood, S.M. Challenge Faculty to Transform STEM Learning. Science 2015, 350 (6258), 281–282. doi:10.1126/science.aab0933.
- National Research Council. Next Generation Science Standards: For States, by States; The National Academies Press: Washington, DC; 2013.
- Cooper, M.M. Chemistry and the Next Generation Science Standards. J. Chem. Educ. 2013, 90 (6), 679–680. doi:10.1021/ed400284c.