ABSTRACT
To help students address problems related to climate change, chemistry fundamentals are taught using sustainable principles. The principles of green chemistry and United Nations Sustainable Development Goals (SDGs) are guides to help us reach a sustainable future. Educators use these to create resources to connect green principles and sustainability goals. Systems thinking provides the method for creating relevant lectures, meaningful activities, and cohesive assessments in an educational module. A week-long stoichiometry module for introductory chemistry is described. Students tackle multiple learning outcomes to answer complex questions such as ‘what makes an reaction efficient?’. This module relates SDGs #7 and #13, clean energy and climate action, to the green principle of atom economy, which evaluates the efficiency of chemical transformations. The process of backward design is used with systems thinking to map learning outcomes across the module. Students demonstrate skills related to individual outcomes and use their knowledge to evaluate chemical systems from multiple perspectives across outcomes. Incorporating real-world examples the module explores how incomplete combustion impacts human health and the environment while exploring the material efficiency of making different fuels. The context and practice of sustainable science can be used to teach chemistry in a systematic way.
GRAPHICAL ABSTRACT
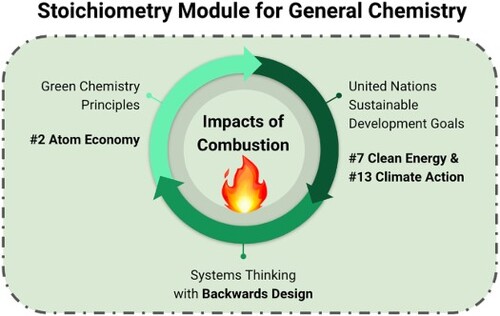
Introduction
Educators and researchers have worked to meaningfully incorporate sustainable practices and goals in their teaching and research (Citation1–3). Towards this end, educators, scientists, and engineers have connected theory and practice by incorporating the United Nations Sustainable Development Goals (SDGs) and Green Chemistry Principles (GCPs) into coursework (Citation4–6). The SDGs are a system of ideals from which sustainability emerges (Citation7). They provide the context for fostering a safe and sustainable society on earth. The twelve principles of green chemistry are a system to classify the nature of chemicals and their transformations (Citation8, Citation9). Green chemistry describes practices focused on efficiency, safety, and sustainability. (Citation10) Though distinctive, the GCPs and SDGs share the core focus of addressing both human and environmental well-being from related perspectives (Citation11, Citation12).
Green chemistry principles and the UN SDGs have been used together to guide chemistry through lecture, laboratory, and research experiences (Citation13, Citation14). These efforts are well documented by the scientific and educational communities and organized across primary, secondary, and higher education (Citation6, Citation7, Citation12, Citation13, Citation14). Connecting GCPs and SDGs is useful when holistically addressing chemicals and their reactions from different perspectives. Each principle and goal focuses on distinctive perspectives such as material or energy efficiency, but certain principles and goals are closely aligned. For example, green chemistry principle #2 Atom Economy is related to SDG goal #7, addressing affordable and clean energy, and goal #13, taking urgent action to combat climate change and its impacts () (Citation11). To teach chemistry in a meaningful context with relevant content a systems thinking approach can be used to relate and teach the GCPs and the SDGs.
Figure 1. Relating the United Nations Sustainable Development Goals and Principles of Green Chemistry.
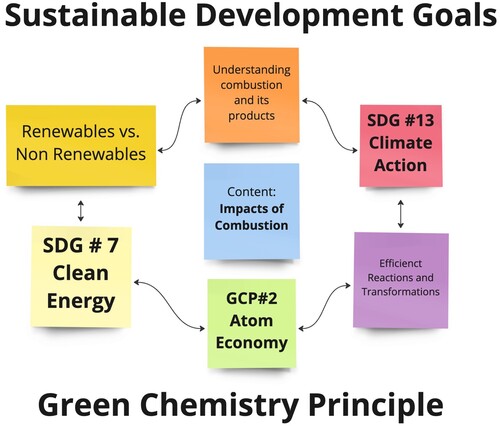
A reaction’s efficiency can be evaluated using different efficiency metrics which are complementary to percent yield. While the yield of a reaction relates the theoretical and actual mass of the product, reaction mass efficiency and process mass efficiency respectively incorporate excess reactants and other reagents to help us broaden our understanding of efficiency (Citation15). Using these metrics in addition to the yield of a reaction, students, educators and chemists have different and more descriptive tools to evaluate the safety and sustainability of chemical systems (Citation16).
Systems thinking is a metacognitive practice where one identifies the parts of a defined system, maps their interactions, and evaluates the system’s changes over time (Citation17, Citation18). This allows one to assess a system before and after these changes, and identify properties that emerge from the interconnected parts. Take for instance the heat and light from fire, the chemical reaction which emerges from a system of methane fuel, oxygen, and a spark (). Without any one of these components, combustion will not occur, and by evaluating the system, we can understand and safely control the reaction. Systems thinking is distinctive but complementary to the scientific method (Citation19). Systems thinking has been used to create curricular materials for teaching sustainability and it is explicitly taught in student activities within these materials (Citation20).
Discussing, using, and comparing the diverse set of green chemistry principles and sustainable development goals when teaching introductory chemistry is a challenging task. Systems thinking can be used to create a comprehensive set of curricular materials including individual and group activities, lecture content, and assessments that effectively relate GCPs and SDGs. Just as emergent properties come from a specific system of interconnected parts, a cohesive, meaningful, and relevant set of curricular materials allows educators to systematically teach fundamental chemistry concepts using green chemistry principles in the context of sustainability. Adding to the systems thinking framework taught in the classroom, systems thinking may serve as a framework for creating curricular materials.
To evaluate products and processes such as a chemical system or educational module a S.W.O.T. analysis, or analysis of the strengths, weaknesses, opportunities, and threats may be performed (Citation21). A S.W.O.T. analysis is typically a qualitative evaluation and snapshot that classifies features of a system based on their positive or negative implications stemming from internal and external factors. It is not used to describe the functioning or piloting of a system. S.W.O.T. analyzes have been used to investigate the real-world implications of green technologies by highlighting important features of systems to inform decision makers and their policies (Citation22, Citation23).
Energy generation from fossil fuels has environmental impacts that are a result of the complete and incomplete combustion of hydrocarbons. The efficiency of a combustible fuel is determined by considering both material and energetic factors of chemical reactions (Citation24). To understand fuel efficiency students must understand both how we evaluate material efficiency, using stoichiometry and ratios of amounts of reactants and products, and energy efficiency related to thermochemical considerations.
Herein we describe the design of an educational module for an introductory chemistry class focused on teaching students the skills to evaluate the material efficiency of a chemical reaction using stoichiometry principles. It comprises roughly three hours of in-class instruction, activities, and assessments. The examples in the module explore the topics of fire safety, the products of combustion reactions, and their environmental impacts. Students practice calculating yields and comparing them to reaction and process mass efficiencies. The content includes explicit discussions of GCP #2, SDGs #7 and #13 and systems thinking such that students are learning the fundamentals of chemistry in a meaningful context and manner. A S.W.O.T. analysis was performed to evaluate how systems thinking effectively incorporates green principles, SDGs and core chemistry content into a set of curricular materials designed after identifying learning outcomes using a backward design approach. By identifying the core chemistry learning outcomes for teaching stoichiometry first, and then developing these in the context of sustainability with content created using real-world examples, chemists and science educators do not have to choose between required and desired material.
Methods and materials
When asking students to broaden the boundaries of the systems they explore it is important that educators have clear boundaries for their curricular materials. When tackling complex issues surrounding sustainability we must not overload students with too much, lest they become overwhelmed and unable to assess things systematically. To help us define what is and is not in a module, a Systems-Oriented Concept Map Extension, or SOCME, can be used to identify and connect the components of an educational module (). (Citation25, Citation26)
Figure 3. Example SOCME relating the principles of green chemistry, sustainable development goals, and learning objectives before, during, and after a module on stoichiometry. The dotted line represents the scope of the module.
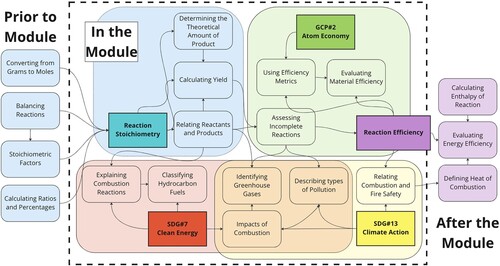
A SOCME for content related to combustion reactions, stoichiometry, GCPs, and SDGs is shown in . While this map is not exhaustive nor is all of this content included in the module, the SOCME helps us classify what will and will not be covered. Note the previous skills, knowledge, and abilities, and the future content which resides outside the scope of the module. This helps visualize how a separate module on energy efficiency is distinctive but will complement our module as part of a larger system, for example an entire course with multiple modules. Once we have defined the borders of our module we can identify the goals of the module.
Backward design is a process used by curriculum developers to craft a structured set of activities and assessments focused on identified learning outcomes (Citation27, Citation28). The five learning outcomes (LOs) for our module were defined by adapting learning objectives from the OpenStax Chemistry Textbook Second Edition () and organizing them with the UN SDGs and GCPs (Citation29). Learning outcomes are higher-order goals, which themselves are broken down into component parts or learning objectives that students will practice to achieve the desired outcome. Just as completing the objectives can allow students to master the outcome, when all five of these outcomes are met, students are able to address complex questions that relate fundamental concepts in chemistry, GCPs, and SDGs. For example, to fully evaluate the impacts of combustion (SDG #13) students must be able to both describe the products of combustion (SDG #7) and assess the completion of a reaction (GCP #2) using stoichiometric principles. The system of learning objectives comprise the learning outcomes which are the throughlines of the module.
A blend of different types of instruction, resources, and activities allows for different types of learning across the module. Inspired by distinctive GCPs and SDGs, the module and its outcomes are split across two-units. Show in the module outline, unit one focuses on the impacts of incomplete combustion to explore SDG #13 and SDG #7 across the first three LOs (A). Unit two focuses on the final two LOs by exploring yield, reaction efficiency metrics, and atom economy, GCP #2. Each of these units, comprising one and a half hours of in-class instruction and activities and three hours of written work outside of class, includes individual and group work, graded and ungraded activities (B). This provides students with multiple opportunities and settings to practice learning objectives as they work towards achieving the learning outcomes. The LOs are mapped across the units and modules to make sure that learning objectives are each addressed multiple times, and learning outcomes are addressed across the module. Now with the LOs as throughlines and the module outline as the scaffold, we can develop assessments that capture evidence of student learning across the module.
Figure 5. A) Mapping learning outcomes and learning objectives across the two-unit module B) Descriptions of module lectures, activities and assessments
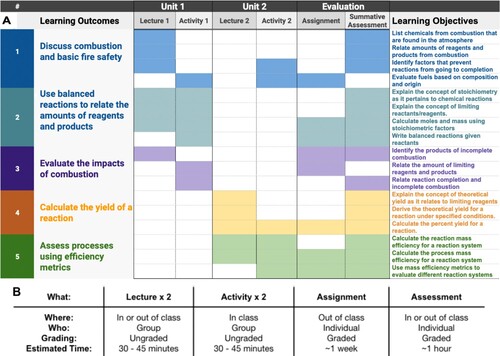
For this module, a formative take-home assignment and summative exam serve as assessments of student learning. These are structured such that all of the learning objectives are individually assessed and certain pairs of outcomes are assessed together (A). Having identified the curricular content, methods of instruction, and assessment practices, a comprehensive module emerges from the individual resources provided in the Supplementary Information including handouts, lecture notes, group activities, assignments and assessments.
Relating different learning outcomes
Let us take two different learning outcomes and explore how they evolve and connect across the module. Learning outcome #3, evaluate the impacts of incomplete combustion, is addressed in the first unit, and in the assignment and assessment. Learning objective #5, assess chemical reactions using efficiency metrics, is addressed in the second unit, and the same assignment and assessment. After reaching both of these outcomes, student’s skills may be used synergistically to answer complex questions considering both environmental impacts and the efficiency of fuels.
Following LO #3, first students are introduced to vocabulary and they practice distinguishing between complete and incomplete combustion. This includes classifying the products of combustion and relating their human health and environmental impacts (). Then in the activity, they practice identifying the products and impacts of combustion reactions. In the assignment they determine and suggest when specific chemical systems exhibit different types of combustion. Finally, in the assessment, their understanding of these ideas is evaluated.
Following LO #5, students compare yield and efficiency metrics including reaction and process mass efficiency in lecture (). They then practice calculating and comparing these metrics for different routes toward hydrogen fuel in the group activity. In the assignment, they work to compare and suggest a potential fuel based on its material efficiency, and in the assessment, they are presented with a complex scenario where they evaluate which fuel would best suit a specific system and work to explain their choice.
Learning outcomes #3 and #5 are both individually assessed in the formative and summative assessments, and connected in activities and assessments (). To fully answer a short answer question in the summative assessment about choosing a fuel for a given system in the assignment, they must draw on the skills practiced while working toward LOs #3 and #5. Due to the systematic design of the module, as students assess the emergent property of efficiency, they are both learning about and aware of the different complex parts of chemical systems.
Results and discussion
This module was developed as part of the American Chemical Society's Green Chemistry Institute Module Development program and piloted at Manhattan College, a primarily undergraduate institution in New York City in the spring of 2022. The 20-student General Chemistry section consisted of science and engineering majors in their first year of study. As part of the module development program, it was evaluated by chemical education researchers and practitioners.
A group of eight module reviewers, including program managers, education researchers, and other module developers evaluated a draft of the module before it was piloted. Their evaluation involved an inventory of core ideas related to both green chemistry and systems thinking and suggestions for improving the module. The main suggestions focused on making the discussion of systems thinking concepts, here defining boundaries of systems, more explicit, and identifying when and where green principles were being used. These are incorporated into the final draft of the module’s materials (Supporting Information). It was noted that the module seemed both comprehensive, yet flexible enough for different educators to add or subtract learning objectives to help make the module fit their student’s needs.
A qualitative S.W.O.T. analysis of the module’s strengths, weaknesses, opportunities, and threats was performed for the piloted module (Citation30). This serves to identify and distinguish internal and external factors that impact the success of the module based on whether they originate from the module, or external aspects, such as the course adopting the module or the influence of institutional learning outcomes (). The core learning outcomes and their connection to the module's component parts are a key strength of the module, while a more comprehensive and explicit instruction of systems thinking ideas, GCPs and SDGs may still be desired by some instructors. It is possible that in upper-division courses the GCPs or SDGs themselves would serve as the basis for each of the learning objectives, while in an introductory course there is prescribed or necessary material to first cover. External factors will always match the environment the module is used in, though the flexibility of the module may serve a variety of educators and students, allowing for activities outside of class, and low or high-stakes assessments based on the instructor’s preference. Of note are the potential threats to using the module, including satisfactory prior instruction and abilities related to stoichiometry and calculations, an ongoing focus of chemical educators (Citation31, Citation32).
Conclusion
While the process of backward design may seem linear, just like the scientific method it allows for flexibility when something isn’t working or something must be addressed. There were many times during the design of the module when we went back and added or removed content, changed an example, or reevaluated the learning objectives. At first we struggled with the decision not to include energy efficiency, realizing this was important and complementary but a different principle of green chemistry. We realized this due to the systems approach to our module, that the module itself is one component of a larger system, here a full chemistry course. The learning outcomes always remained at the core of the module serving as a guide, but even these were reworded or reordered for clarity and the cohesion of the module.
Backward design served as a guide to crafting the module and including systems thinking practices, green chemistry principles as the context, and the SDGs as the real-world context. These three components were mapped and linked using learning outcomes and their component learning objectives. These outcomes and objectives were introduced, practiced, and assessed over the course of a two-unit module. This curriculum focuses on basic stoichiometric principles and connects them through the property of yield to examine GCP #2, atom economy, and incorporates efficiency metrics allowing students to evaluate more complex and realistic chemical systems. These ideas are then used to explore human and environmental health through the lens of clean energy and climate action, SDGs #7 and #13.
To connect and relate core chemistry concepts, relevant content related to sustainability, and practice evaluating chemistry via multiple perspectives systems thinking can be used to create curricular materials. By first relating component parts such as GCPs and SDGs and then linking these to fundamental chemistry learning objectives modular curricula do not have to sacrifice meaningful content or context. Mapping learning outcomes across the semester makes sure that all of the material taught can be assessed and by completing learning across different individual and group activities. Beyond this at the end of a structured module students have the tools and resources to tackle questions connecting to multiple learning objectives to evaluate complex chemical systems. This model may be used by educators looks to explore science disciplines in the context of sustainability.
Systems thinking can be used in chemistry both to organize chemical systems and to solve problems across science and engineering. Further, it can be used to create curricular materials, and as a manner in which students can practice and learn fundamental chemistry topics such as stoichiometry. As we prepare our students for the future we must hand them the tools to tackle complex problems around sustainability. Here the principles of green chemistry and Sustainable Development Goals work together to make chemistry education instruction, practice, and assessment both engaging and meaningful.
Supplemental Material
Download MS Word (12.3 MB)Acknowledgments
This work was supported by the American Chemical Society’s Green Chemistry Institute as part of the Green & Sustainable Chemistry Education Module Development Project. The authors would like to thank their subject matter expert, Grace Lasker, and assessment consultant, Elizabeth Day in addition to the program coordinators, David Laviska, David Constable, Jennifer MacKellar and Aurora Ginzberg. They would also like to thank Manhattan College’s CHEM 101 General Chemistry 1 Section 05 from Spring 2022 and the organizers of the ‘Integrating Green Chemistry and Sustainability into Chemistry Education’ session at BCCE 2022 at Purdue University.
Disclosure statement
No potential conflict of interest was reported by the author(s).
Additional information
Funding
References
- Brundiers, K.; Wiek, A.; Redman, C.L. Real-world Learning Opportunities in Sustainability: From Classroom Into the Real World. Intern. J. Sustain. Higher Educ. 2010.
- DuPuis, E.M.; Ball, T. How Not What: Teaching Sustainability as Process. Sustain. Sci. Practice Policy 2013, 9 (1), 64–75. doi:10.1080/15487733.2013.11908108.
- Anastasiadis, S., et al. Teaching Sustainability: Complexity and Compromises. J. Appl. Res. Higher Educ. 2020, 13 (1), 272–286. doi:10.1108/JARHE-02-2020-0029.
- Axon, S.; James, D. The UN Sustainable Development Goals: How Can Sustainable Chemistry Contribute? A View from the Chemical Industry. Curr. Opin. Green Sustain. Chem. 2018, 13, 140–145. doi:10.1016/j.cogsc.2018.04.010.
- Chen, T.-L., et al. Implementation of Green Chemistry Principles in Circular Economy System Towards Sustainable Development Goals: Challenges and Perspectives. Sci. Total Environ. 2020, 716, 136998.
- Petillion, R.J.; Freeman, T.K.; McNeil, W.S. United Nations Sustainable Development Goals as a Thematic Framework for an Introductory Chemistry Curriculum. J. Chem. Educ. 2019, 96 (12), 2845–2851.
- Anastas, P., et al. The Power of the United Nations Sustainable Development Goals in Sustainable Chemistry and Engineering Research. ACS. Sustain. Chem. Eng. 2021, 9 (24), 8015–8017.
- Anastas, P.T.; Warner, J.C. Principles of Green Chemistry. Green Chem. Theory Practice 1998, 29.
- Jessop, Philip G., Sofia Trakhtenberg, and John Warner. “The Twelve Principles of Green Chemistry.” 2009. 401–436.
- Kaur, G.; Uisan, K.; Ong, K.L.; Ki Lin, C.S. Recent Trends in Green and Sustainable Chemistry & Waste Valorisation: Rethinking Plastics in a Circular Economy. Curr. Opin. Green Sustain. Chem. 2018, 9, 30–39. doi:10.1016/j.cogsc.2017.11.003.
- Poliakoff, M.; Licence, P.; George, M.W. UN Sustainable Development Goals: How Can Sustainable/Green Chemistry Contribute? By Doing Things Differently. Curr. Opin. Green Sustain. Chem. 2018, 13, 146–149.
- Chen, T.-L., et al. Implementation of Green Chemistry Principles in Circular Economy System Towards Sustainable Development Goals: Challenges and Perspectives. Sci. Total Environ. 2020, 716, 136998.
- Ivanković, A., et al. Review of 12 Principles of Green Chemistry in Practice. Intern. J. Sustain. Green Energy 2017, 6 (3), 39–48.
- Tarasova, Natalia P., Anna A. Dodonova, and Alexey A. Zanin. “The Concept of Sustainable Development and the Principles of Green Chemistry as an Integral Part of the Modern Chemical Education System.” Chemistry Education for a Sustainable Society Volume 2: Innovations in Undergraduate Curricula. American Chemical Society, 2020. 137–145.
- Silverman, J.R. Exploring Sustainability Metrics in General Chemistry Using Intensive and Extensive Properties of Matter. J. Chem. Educ. 2021, 98 (9), 2741–2745. doi:10.1021/acs.jchemed.1c00113.
- Silverman, J.R.; Hudson, R. Evaluating Feedstocks, Processes, and Products in the Teaching Laboratory: A Framework for Students to use Metrics to Design Greener Chemistry Experiments. J. Chem. Educ. 2020, 97 (2), 390–401. doi:10.1021/acs.jchemed.9b00292.
- York, S., et al. Applications of Systems Thinking in STEM Education. J. Chem. Educ. 2019, 96 (12), 2742–2751.
- Orgill, M.; York, S.; MacKellar, J. Introduction to Systems Thinking for the Chemistry Education Community. J. Chem. Educ. 2019, 96 (12), 2720–2729.
- Hurst, G.A. Systems Thinking Approaches for International Green Chemistry Education. Curr. Opin. Green Sustain. Chem. 2020, 21, 93–97.
- York, S.; Orgill, M. CheMIST Table: A Tool for Designing or Modifying Instruction for a Systems Thinking Approach in Chemistry Education. J. Chem. Educ. 2020, 97 (8), 2114–2129.
- Pernaa, J. Possibilities and Challenges of Using Educational Cheminformatics for STEM Education: A SWOT Analysis of a Molecular Visualization Engineering Project. J. Chem. Educ. 2022, 99 (3), 1190–1200.
- Falcone, P.M.; Tani, A.; Tartiu, V.E.; Imbriani, C. Towards a Sustainable Forest-Based Bioeconomy in Italy: Findings from a SWOT Analysis. Forest Pol.Econ. 2020, 110, 101910. doi:10.1016/j.forpol.2019.04.014.
- D’Adamo, I.; Falcone, P.M.; Gastaldi, M.; Morone, P. RES-T Trajectories and an Integrated SWOT-AHP Analysis for Biomethane. Policy Implications to Support a Green Revolution in European Transport. Energy. Policy. 2020, 138, 111220. doi:10.1016/j.enpol.2019.111220.
- McMillan, Colin A. Material and Energy Efficiency: A Framework for Broader Analysis of Energy Use in the US Economy. No. NREL/TP-6A20-70609. National Renewable Energy Lab. (NREL), Golden, CO (United States), 2018.
- Constable, D.J.; Jiménez-González, C.; Matlin, S.A. Navigating Complexity Using Systems Thinking in Chemistry, with Implications for Chemistry Education. J. Chem. Educ. 2019, 96 (12), 2689–2699.
- Mahaffy, P.G., et al. Integrating the Molecular Basis of Sustainability Into General Chemistry Through Systems Thinking. J. Chem. Educ. 2019, 96 (12), 2730–2741.
- Reynolds, H.L.; Kearns, K.D. A Planning Tool for Incorporating Backward Design, Active Learning, and Authentic Assessment in the College Classroom. Coll. Teach. 2017, 65 (1), 17–27.
- Neiles, K.Y.; Arnett, K. Backward Design of Chemistry Laboratories: A Primer. J. Chem. Educ. 2021, 98 (9), 2829–2839.
- Flowers P., et al. Chemistry 2e OpenStax Rice University https://openstax.org/details/books/chemistry-2e.
- AlMarwani, M. Pedagogical Potential of SWOT Analysis: An Approach to Teaching Critical Thinking. Think. Skills Creat. 2020, 38, 100741.
- Kauffman, G.B. A Schematic Summary of General Chemistry Stoichiometry. J. Chem. Educ. 1976, 53 (8), 509. doi:10.1021/ed053p509.
- Cai, S. Harry Potter Themed Digital Escape Room for Addressing Misconceptions in Stoichiometry. J. Chem. Educ. 2022, 99 (7), 2747–2753. doi:10.1021/acs.jchemed.2c00178.