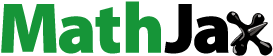
ABSTRACT
The anti-corrosive effect of the extract in ethanol of Justicia brandegeeana (Acanthaceae) against carbon steel AISI 1020 in sulfuric acid medium 1 mol L−1 was investigated. In addition to the effect of seasonality, several techniques were used to investigate the anti-corrosive activity, such as electrochemical impedance spectroscopy (EIS), potentiodynamic polarization (PP), linear polarization resistance (LPR), and weight loss. The chelating effect was analyzed by UV-Vis spectrophotometry and the surface was analyzed by scanning electron microscopy (SEM). The results showed that the inhibition efficiency is dependent on the concentration of the extract, reaching its maximum at 1500 ppm and despite having good thermal stability (82.41% inhibition at 70°C), it decreases with increasing temperature, indicating a physisorption behavior. The extract obeyed the Langmuir isotherm and the Tafel curves characterized it as a mixed inhibitor, with a tendency to cathodic region. It also presented a 52% chelating effect against Fe+2 ions, and the SEM analysis indicated a protection with the presence of plant extract, thus minimizing surface roughness. These results indicated the J. brangeeana ethanol extract as a green anti-corrosive agent.
Introduction
Corrosion is a crucial problem worldwide that heavily affects natural and industrial environments. Countless production areas, such as the chemical industry, civil construction, automotive sector, dentistry, and others, are directly impacted by the corrosive process, which mostly affects metals. It is estimated that in 2016 the global annual cost of corrosion consisted of 3.4% of GDP, equivalent to approximately US 2.5 trillion (Citation1).
Among the various materials that can corrode, carbon steel is the most used industrially due to its low cost and ease of welding and forming. Carbon steel pipes are responsible for transporting large volumes of oil, derivatives, and natural gas, but this material has limitations, such as low resistance to corrosion during prolonged exposure to high temperatures, which can cause serious problems in the industry (Citation2, Citation3).
Corrosion can be controlled by several means, from modifying the process or the corrosive medium to applying protective coatings. The use of corrosion inhibitors is one of the more effective ways to prevent or control corrosion in several materials (Citation4). The use of natural inhibitors has been intensified due to their renewable origin, biodegradability, low cost and not hazardous to human health, and the environment (Citation5). Furthermore, the inhibitors, to be considered green or eco-friendly need to meet three requirements: (i) they must not be bioaccumulative, (ii) they must be biodegradable, and (iii) they must have zero or very low marine toxicity (Citation6, Citation7).
Plant extracts have been widely studied as inhibitors due to their low cost, abundant availability, and biodegradability. The different parts of plants are useful, such as bark, stem, root, leaves, flowers, and seeds, or even waste and by-products of agroindustry; however, they may present different inhibition efficiencies due to the amounts of secondary metabolites and other organic substances (Citation8–12).
Considering the range of existing studies on vegetal extracts with corrosion-inhibitory characteristics, it is possible to exemplify with the citation of some works. The corrosion inhibition efficiency of 89.2% by the aqueous extract of coffee husks was obtained at 800 ppm in HCl 1.0 mol L−1 with 24 h of immersion (Citation4). In the same corrosive medium, Dehghani et al. (Citation13) evaluated the aqueous extract of the Chinese gooseberry (Kiwi) fruit peel and obtained 92% efficiency in 2.5 h of immersion at 1000 ppm (Citation13).
Hydroalcoholic extracts at 400 ppm of orange, mango, passion fruit, and cashew peels, in addition to papaya seed and grape pomace, were evaluated in two different media: neutral and HCl 1.0 mol L−1 medium. The extracts did not show good inhibition in a neutral medium, except for 2% concentrated grape pomace, which showed 74% efficiency in 72 h of immersion by the gravimetric technique. However, they obtained a variation of 93%–97% of inhibition efficiency in an acid medium (Citation14).
Santana et al. (Citation15) studied the aqueous extract of castor bean, also at 800 ppm and obtained 97.8% efficiency by the gravimetric method in HCl 1.0 mol L−1 medium, showing that castor bean is a mixed inhibitor, attributed to the existence of ricinoleic acid in its composition (Citation15).
The hydroalcoholic extract of Malvaviscus arboreus was tested against H2SO4 1.0 mol L−1 and it showed an inhibition efficiency of 97.5% at 3, 24, and 48 h at 500 ppm (Citation16). The aqueous extract of Coriandrum sativum seeds was evaluated in two different acid media (H2SO4 0.5 mol L−1 and HCl 1.0 mol L−1) and showed good inhibition efficiencies for both media at a concentration of 1000 ppm, being 96.7% and 93.7%, respectively (Citation17).
Alvarez et al. (Citation18) evaluated the methanolic extract of Rollinia occidentalis in 1.0 mol L−1 of the HCl medium and obtained 85.7% anti-corrosion efficiency with the extract at 1000 ppm (Citation18).
Recently, the aqueous extract of Brassica rapa was evaluated regarding the anti-corrosion effect in Q235 steel in a sulfuric acid medium. The observed results revealed an inhibition efficiency of 94.3% and 92.5% by polarization potentiodynamic (PP) and electrochemical impedance spectroscopy (EIS), respectively (Citation19). The review of natural plant extracts shows the performance of Fraxinus excelsior (FE), Zingiber zerumbet (ZZ), and Isatis tictoria (IT) as efficient anti-corrosion agents of the mild carbon steel in phosphoric acid (2 mol L−1). The observed inhibitory efficiencies by the weight loss method were 95.2%, 92.8% and 91.7%, respectively, to FE, ZZ, and IT aqueous extracts with the concentration of 3 g L−1 (Citation20).
Furthermore, Gu et al. (Citation21) evaluated the efficiency of copper protection in sulfuric acid (0.5 mol L−1) by the aqueous extract of Lycium barbarum leaves. The electrochemical methods showed corrosion inhibition efficiencies of 92.9%, 92.8%, and 94.1% at 298, 303, and 308 k, respectively (Citation21). Golshani et al. (Citation22) investigated the Dracocephalum extract as a green corrosion inhibitor for mild steel in sulfuric acid (0.5 mol L−1) and hydrochloric acid (1.0 mol L−1) media. Interestingly, the authors observed an inhibition efficiency of 94% at 75 ppm of concentration and 89% at 200 ppm in the H2SO4 solution, but in the HCl medium the value of 88% at 100 ppm and 90% at 400 ppm. The results indicated that extract acts in cathodic and anodic processes by the Langmuir adsorption isotherm (Citation22).
Because of the range and importance of studies with extracts of vegetal species, it is proposed, then, the analysis of Justicia brandegeeana, an ornamental plant, as the object of study of this work. J. brandegeeana shown in is popularly known as red shrimp, and belongs to the Acanthaceae family, originally from Mexico. It is a bushy plant, being used for ornamental purposes in tropical and subtropical climates (Citation23, Citation24).
The first phytochemical investigation of J. brandegeeana was carried out by Jiang et al. (25), where its essential oil was analyzed, identifying 32 compounds, with the main class being alcohols (68.42%), followed by aldehydes and ketones (9.92%), esters (6.59%), and alkanes (1.10%) (Citation25). Another study demonstrated the effectiveness of J. brandegeeana petroleum ether extract at 1200 ppm as a biocontrol agent against Rhynchophorus ferrugineus which is a pest of rapid dispersion in several species of palm tree. 98.47% of total fatty acids was detected by GC/MS, with 11.98% saturated fatty acids, mainly palmitic acid; 86.49% unsaturated fatty acids, the main component being linoleic acid with 37.4% (Citation26).
Thus, with the advancement of the search for new eco-friendly corrosion inhibitors, especially the use of plant extracts, the choice of J. brandegeeana for this work was outlined due to the existence of few biological and phytochemical studies related to it, and no studies on its use as an anti-corrosive agent, in addition to being an easy handling ornamental plant. The anti-corrosive effect of the ethanolic extract of J. brandegeeana was then evaluated in carbon steel in an H2SO4 medium at 1.0 mol L−1 because is a corrosive medium usually used in industrial processes. The analyses occurred by the gravimetric method, electrochemical analysis as electrochemical impedance spectroscopy (EIS), potentiodynamic polarization (PP) and linear polarization resistance (LPR), and surface analysis by scanning electron microscopy (SEM).
Experimental
Plant extract
The aerial parts (leaves, flowers and stems) of Justicia brandegeeana Wassh. & L. B. Sm. were collected in autumn and spring, to compare the effects of seasonality in the extracts. The collection took place in the garden of the Chemistry Institute and the samples were identified in the Herbarium of the Department of Botany which deposited a voucher specimen RBR 56243, both Federal Rural University of Rio de Janeiro
were collected 500.0 g of material that after drying at room temperature presented 70% and 69% of moisture for the June and October collections, respectively. The dry, crushed, and heavy mass was extracted by static maceration in 2.0 L of ethanol for 7 days, generating a fluid extract of J. brandegeeana with 3470 ppm that was stored in an amber container under refrigeration of approximately 4°C. The concentration of extract was obtained by the difference in mass the first weighing of the material dry before extraction and the material dry after extraction, obtaining the concentration of the material in the solvent. From the fluid extract were performed dilutions with ethanol to obtain concentrations in the range of 250–1500 ppm of the inhibitor.
Both extracts were analyzed by FTIR (ATR, Bruker – VERTEX 70), 1H NMR and 13C, obtained in DMSO-d6 (Bruker – AVANCE – 500 MHz) spectra to compare the seasonality effects on the main organic compound classes found in the ethanol extract of J. brandegeeana.
Acid solution
The acid solution used was prepared from concentrated sulfuric acid in analytical grade and Milli-Q water. A stock solution was prepared at the concentration of 2.0 mol L−1 and the required concentration of 1.0 mol L−1 was obtained by dilution.
Electrochemical assays
The AUTOLAB PGSTAT 302N potentiostat was used for the analyses, with an electrochemical cell composed of three electrodes: the reference electrode of Ag/AgCl (3 mol L−1 KCl), the counter electrode of platinum, the working electrode of carbon steel AISI 1020 inserted in epoxy resin, with an exposed surface area of 2.1 cm2. All measurements were performed in a Faraday cage and the results were analyzed in the software NOVA 2.1.5. The working electrode, before the analysis, was sanded with sandpaper of three different grades (400, 600, and 1200 mesh), washed with distilled water to remove impurities, defatted with ethanol and dried.
Initially, the blank solution contained only ethanol and H2SO4 1.0 mol L−1. Subsequently, the solutions containing the ethanol extract of J. brandegeeana at 250, 450, 750, 1000, and 1500 ppm were analyzed at a temperature of 32°C with a total volume of 50 mL in the cell for each reading. The analyses were performed in triplicate to examine the reliability and reproducibility of the measurements, and one of the rejoinders was selected to represent the data graphically.
The electrochemical analysis consisted in the stabilization of the open circuit potential (OCP) in 5400 s, thus obtaining the OCP corresponding to the corrosion potential (Ecorr) of the working electrode. Then, electrochemical impedance spectroscopy (EIS), linear polarization resistance (LPR), and finally potentiodynamic polarization (PP) were performed.
The EIS spectra were performed in a frequency range of 10 kHz–0.1 Hz with 10 mV peak-by-peak amplitude and the inhibitor efficiency (ηEIS) was calculated using EquationEquation 1(1)
(1) :
(1)
(1) where Rct is the charge transfer resistance in solution with inhibitor and R0ct is the charge transfer resistance in the blank solution.
The LPR assays were performed using a sweep rate of 1.0 mVs−1 in the potential range of ±10 mV around the open circuit potential (Eocp), and the inhibition efficiency (ηLPR) was calculated from EquationEquation 2(2)
(2) :
(2)
(2) where Rp and R0p are the polarization resistance in the solution with inhibitor and blank solution, respectively.
The PP assays were performed in the ±200 mV potential range around the open circuit potential (Eocp), with a sweep rate of 1.0 mVs−1, and the inhibition efficiency (ηPP) was calculated with EquationEquation 3(3)
(3) :
(3)
(3) where icorr,0 and icorr are the corrosion current densities in the solution with inhibitor and blank solution, respectively.
Weight loss measurements
The weight loss tests were performed with carbon steel coupons AISI 1020 with dimensions of 3.0 × 3.0 × 0.15 cm in 50 mL of H2SO4 1.0 mol L−1 solution and the ethanol extract of J. brandegeeana in a concentration range of 250–1500 ppm.
The coupons received the same treatment performed for the working electrode in electrochemistry, then were weighed in an analytical balance and immersed in the solution in the absence (blank) and in the presence of the extract under temperature control with the aid of a thermostatic bath. After immersion, the coupons were again cleaned with distilled water and ethanol, dried, and weighed.
The gravimetric assays were performed in triplicates with variations of immersion time (3, 6, 24, and 48 h), temperature (30°C, 40°C, 50°C, 60°C, and 70°C) and inhibitor concentration (250, 450, 750, 1000, and 1500 ppm).
The inhibition efficiency (η%) of the plant extract of J. brandegeeana for carbon steel AISI 1020 was calculated by EquationEquation 4(4)
(4) :
(4)
(4) where W0corr and Wcorr are the corrosion rates in the blank and the presence of the inhibitor, respectively.
Chelating effect
To determine the chelating effect of the ethanol extract of J. brandegeeana, the UV-VIS spectrophotometry method (Shimadzu, model UV-1800) was used. The spectra were obtained in quartz cuvette (1.5 mL) and to determine the interaction between the plant extract and the Fe+2 ions FeSO4 solution was used at the final concentration of 1.87 × 10−6 mol L−1, and solutions of the extract at 125 ppm, concentrations that provided the best curves.
The analysis was performed after a time-out for 10 min at room temperature. EDTA was used as a positive control for the chelating effect at the concentration of 2.32 × 10−4 mol L−1.
The analysis was performed after 10 min of rest at room temperature. EDTA was used as a positive control for the chelating effect at the concentration of 2.32 × 10−4 mol L−1. The chelating effect of the ethanol extract of J. brandageeana was calculated and expressed as a percentage from EquationEquation 5(5)
(5) :
(5)
(5) where As and Ac are the maximum absorbances of the sample and the control, respectively.
Surface analysis
The analysis of the metallic surface was performed by scanning electron microscopy (SEM) in the presence and absence of the ethanol extract of J. brandegeeana. Three coupons of carbon steel AISI 1020 were analyzed: metallic surface without immersion in any medium (Coupon I), metallic surface after immersion in the corrosive medium H2SO4 1.0 mol L−1 in the absence of the extract in ethanol of the plant species for 24 h at 30°C (Coupon II) and metal surface after immersion in the corrosive medium H2SO4 1.0 mol L−1 in the presence of the plant extract in ethanol species at 1000 ppm for 24 h at 30°C (Coupon III).
The treatments used in the coupons before and after the analysis were the same ones performed in the weight loss tests. The micrographs were obtained with an acceleration voltage of 15 kV and with 4000× magnification. The analysis was performed using the HITACHI TM 3000 Tabletop Microscope.
Results and discussion
Plant extract
The effects of seasonality for the ethanolic extracts of J. brandegeeana collected on two different occasions were evaluated for the presence of the main functional groups present in the metabolites through infrared spectroscopic analysis, 1H NMR, and 13C NMR for each of the samples (Citation27).
shows the infrared spectrum for the extract obtained from the June and October collects, presenting broadband at 3299 cm−1 relative to the presence of OH with intermolecular interactions derived from hydrogen bonds. Bands in 2923 and 2854 cm−1 were attributed to stretch asymmetric and symmetric strains of aliphatic C–H, respectively. The band at 1733 cm−1 can be attributed to carbonyl groups of ester or carboxylic acids (Citation28). The average intensity band at 1614 cm−1 may indicate vibrations of C = C conjugated to carbonyl. At 1400 cm−1, it was observed the absorption relative to the OH folding, and at 1051 cm−1 the stretch relative to a primary alcohol. The presence of these bands indicates the existence of heteroatoms such as oxygen, which are desirable in organic corrosion inhibitors. The infrared spectrum of the extract of the second collection (October) showed great similarity with that collected in June, indicating that the effect of seasonality showed change only in the intensity of the bands of the spectrum, being in this case less intense not having change like the classes of extracted substances.
Figure 2. Overlapping of infrared spectra, in ATR, for extracts of J. brandegeeana obtained in June 2021 and October 2021.
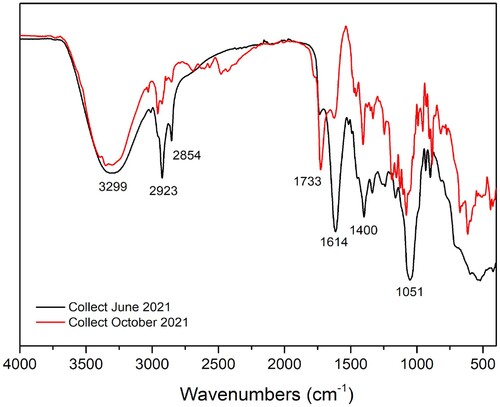
The 1H NMR spectra for the ethanol extracts of J. brandegeeana in the collects of June and October are presented in they were very similar, only with a difference in the intensity of the signals, being the extract collected in June the one that presented the highest intensity in the signals, as well as in the 13C-DEPTQ NMR spectra showing in .
Figure 3. Overlapping of 1H NMR spectra in DMSO-d6, at the power of 500 MHz for the extracts of J. brandegeeana obtained from the collections of June 2021 and October 2021.
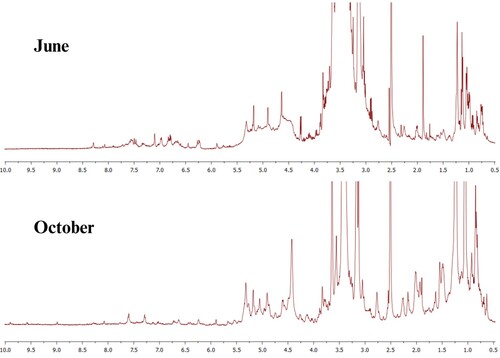
Figure 4. Overlapping of 13C-DEPTQ NMR spectra in DMSO-d6, at the power of 500 MHz for the extracts of J. brandegeeana obtained from the collections of June 2021 and October 2021.
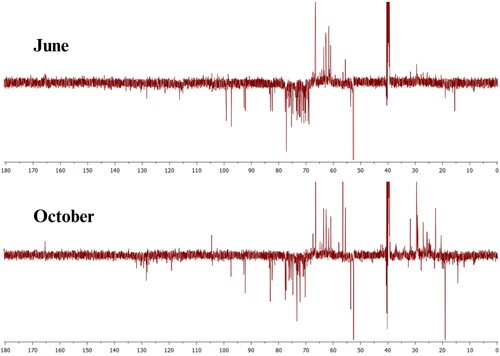
The ethanol extract prospection was performed also through the 1H and 13C-DEPTQ NMR spectra enabling the identification of the carbohydrate class presence through the signals in the range of 4.0–5.5 ppm (1H NMR) and 60–80 ppm (13C-DEPTQ NMR), with the presence of methoxy groups (3–4 ppm for 1H and 52.6 ppm for 13C). In addition, signals at 10.14 and 10.56 ppm in the 1H NMR spectra indicated the OH moieties’ presence of carboxylic acids and/or alcohols according to the bands observed in the infrared spectra. Chemical displacements in the 13C-DEPTQ NMR spectra in the range of 100–60 ppm suggested the presence of disaccharides or glycosylated metabolites according to the literature (Citation29, Citation30), highlighting the absorptions in 99.17 ppm: anomeric carbon of β-D-fructopyranose, 97.21 ppm: anomeric carbon of β-D-glucopyranose, 92.31 and 92.63 ppm: anomeric carbon of α-D-glycopyranose, 82.15 and 82.87 ppm: CH (C5) of α-D-fructofuranose, 68.77–77.60 ppm: -CHOH of gluco or fructopyranose, 60.88–63.50 ppm: CH2-OH of gluco or fructopyranose.
Electrochemical tests
Analysis of the OCP
shows the evolution of the open circuit potential for 5400 s, until its stabilization, in the presence and absence of the plant extract at different concentrations. Such stabilization is necessary so that there is no potential variation during measurements, thus, the blank stabilized at −0.427 V, while in the presence of the J. brandegeeana extract the OCP varied in the range of −0.438 to −0.432 V in the concentrations of 250–1000 ppm, with values more negative than the blank, as shown in . Already at 1500 ppm, stabilization occurred at −0.420 V, with potential more positive than blank.
Figure 5. OCP variation obtained in the absence and presence of J. brandegeeana ethanol extract at various concentrations.
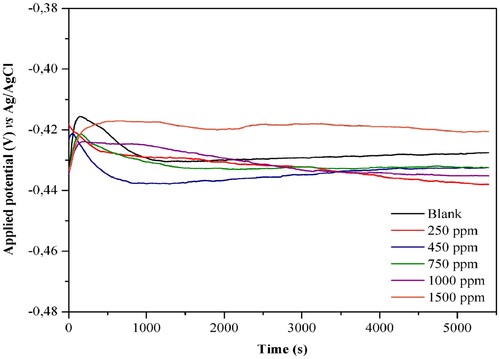
Table 1. EIS parameters for carbon steel AISI 1020 in H2SO4 1.0 mol L−1 solution in the absence and presence of various concentrations of the J. brandegeeana ethanol extract at 32°C.
Electrochemical impedance spectroscopy – EIS
The ethanol extract of J. brandegeeana was evaluated for its inhibitory activity at concentrations of 250, 450, 750, 1000, and 1500 ppm using EIS, against carbon steel AISI 1020 immersed in a solution of H2SO4 1,0 mol L−1 at 32°C.
After the potential stabilization, the Nyquist and Bode diagrams, shown in (A,B), respectively, were obtained. The Nyquist diagram is an alternative to represent the results in characteristic frequencies, using the charge transfer function, varying from zero to infinity (Citation31).
Figure 6. (A) Diagram of Nyquist and (B) Bode diagram, obtained in the absence and presence of the ethanol extract of J. brandegeeana for carbon steel AISI 1020 in H2SO4 1.0 mol L−1.
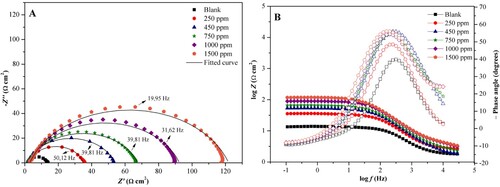
The graph obtained for the Nyquist diagram shows a single semicircle, which corresponds to a capacitive arc, with displacement along the real impedance axis (Z‵) for the blank and the variations of the concentration of the plant extract. The displacement for higher values of Z‵ indicates that the corrosion reaction is under the control of load transfer and double-layer capacitance, thus, the higher the concentration, the larger the diameter of the semicircle, indicating adsorption of plant extract substances to the metal surface promoting an inhibitory barrier to the charge transfer reactions occurring at the metal-inhibitor interface (Citation32).
There is no change in the shape of the curves in the presence and absence of the inhibitor, which, according to Carlos et al. (33), indicates that the adsorption of the extract on the surface occurs without altering the mechanism of corrosion of the metal. In addition, curves in the absence and presence of the inhibitor present a flattening that may be related to non-homogeneity and the presence of roughness on the metal surface, caused by the corrosive process itself and the formation of a protective layer, promoting the dispersion of the frequency (Citation33–35).
When analyzing the Bode diagrams, we have the first portion log f vs phase angle, in which we can observe the presence of a single constant phase for the corrosion process, represented by a single crest in the presence and absence of the inhibitor (Citation36).
With the increasing frequency there is an increase in phase angle in the absence and presence of the inhibitor; however, the blank has a maximum angle of 42.7°, while in the presence of the inhibitor, there is a variation in the range of 50.4°–57.1° in 250 and 1500 ppm, respectively, still displaying a single time constant (Citation37). This fact indicates that with the increase of the time of contact of the extract with the carbon steel, and the concentration of the inhibitor there is an increase of the phase angle and capacitance, suggesting a modification in the microstructure in the metallic surface, due to the formation of a protective film, whereas an ideal capacitor occurs with a maximum phase angle of 90° (Citation33, Citation38).
When observing the portion log Z vs log f it is noticed that, in low frequency there is an increase of impedance in the presence of the inhibitor, being demonstrated by progressive parallel lines according to the increase of the extract concentration, suggesting the protection of the metallic surface (Citation35). Both results observed in the Bode diagram corroborate the results observed in the Nyquist diagram.
The equivalent circuit model that fit all curves was Randle’s circuit, presented in , in which the resistance of the solution (Rs) is in series with the resistance to load transfer (Rct), and this is in parallel with the constant phase circuit (CPE) (Citation39).
Each component of the equivalent circuit provides information about what occurred at the metal/inhibitor interface, and, according to Ma et al. (38), the Rct value is influenced by the inhibitor concentration and by the formation of a film on the metal surface. This film generates a new layer at the metal/electrolyte interface which is rated by the electrical capacitance of the double layer (Cdl) (Citation38).
The constant phase circuit (CPE) model is inserted to justify the heterogeneous nature of the inhibitor coating, in which the capacitance is not ideal, thus seeking a more precise adjustment (Citation38). An n parameter is also inserted, which includes surface roughness, inhibitor adsorption, and/or desorption and formation of porous film, with the deviation of the ideal behavior between 1 and –1 (Citation34, Citation37).
The Cdl was calculated by EquationEquation 6(6)
(6) , through constant phase elements (Citation40).
(6)
(6) where Yo is the magnitude of the CPE and fmax is the frequency where the imaginary component of the impedance is the maximum.
The degree of surface coverage (θ) was obtained in EquationEquation 7(7)
(7) , followed by the calculation of the corrosion inhibition efficiency described in EquationEquation 1
(1)
(1) , where Rct is the load transfer resistance in the blank and R0ct is the load transfer resistance in the presence of the inhibitor. Thus, shows the results obtained by EIS.
(7)
(7) When observing the results obtained by EIS, it is noted that with the increase of the concentration of the J. brandegeeana extract, there is an increase in the efficiency of corrosion inhibition, reaching 88% in 1500 ppm. Together with this data there is an increase of Rct, indicating that the resistance to load transfer is dependent on the concentration of the inhibitor, being justified by the increase of θ and the resistance of the solution.
There is also a decrease in Cdl, which suggests a decrease in the local dielectric constant and/or increase in the viscosity of the electric double layer, demonstrating a slow substitution of water molecules and ions by inhibiting molecules, thus creating a protective film on the metal surface (Citation38, Citation41).
Potentiodynamic polarization – PP
The potentiodynamic polarization (PP) assays were performed under the same conditions as EIS for the ethanol extract of J. brandegeeana and the same concentrations. In the PP curves are obtained to identify the behavior of the plant extract, classifying it as a cathodic, anodic, or mixed inhibitor.
Figure 8. PP curves obtained in the presence and absence of the J. brandegeeana ethanol extract in varied concentrations for carbon steel AISI 1020 in H2SO4 1.0 mol L−1.
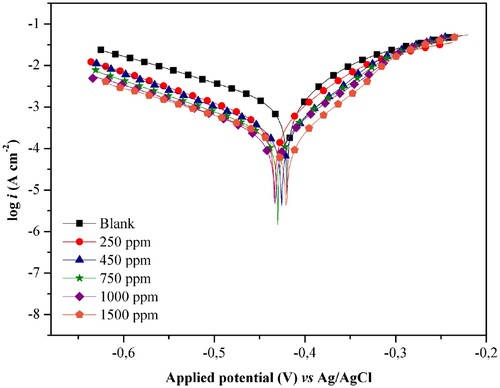
There was a decrease in current density with the presence of the extract in ethanol, both in the anodic and cathodic portions, suggesting the adsorption of inhibitors to the metal surface. The decrease was constant for all concentrations studied, being higher in the cathodic portion in relation to the blank.
There is a slight displacement of the Ecorr for more negative values, being more pronounced in 250 ppm with 13.85 mV of displacement, indicating a blockage of the cathodic areas of the metal surface. According to Fernandes et al. (36) and Ma et al. (38) to have a classification of the inhibitor as cathodic or anodic this displacement must reach values greater than 85 mV (Citation36, Citation38). In this case, the results indicate that the ethanol extract of J. brandegeeana can be considered as a mixed inhibitor, inhibiting both the dissolution of the metal in the anode and the reduction of hydrogen ions in the cathode, but with cathodic tendency (Citation41, Citation42).
In the anodic area, for a potential above −0.3 V, the formation of a plateau in high current density, which corresponds to a passivation process, which can be explained by the adsorption of inhibiting molecules to the metal surface simultaneously the desorption of these molecules, caused by the dissolution of the metal in the corrosive medium. In this plateau then, the desorption rate of the inhibitor is higher than the adsorption rate, being observed by the increase of the current density with the increase of the potential (Citation34, Citation43, Citation44).
describes the electrochemical parameters of corrosion potential (Ecorr), corrosion current density by surface area (jcorr), anodic (βa) and cathodic (βc) constants obtained by Tafel extrapolation. The inhibition efficiency ηPP was obtained from EquationEquation 3(3)
(3) .
Table 2. PP parameters for carbon steel AISI 1020 in H2SO4 1.0 mol L−1 solution in the absence and presence of the extract in ethanol of J. brandegeeana.
The results show that with the increase of the inhibitor concentration occurs the decrease of the corrosion current density and consequently the increase of the inhibition efficiency, presenting 84% to 1500 ppm, indicating inhibition of the metal surface by adsorption of the J. brandegeeana extract (Citation37). In addition, the cathodic and anodic Tafel lines show little variation in the presence of plant extract, and this fact linked to impedance results suggests the inhibition of corrosion without mechanism modification of the cathodic and anodic reactions (Citation45).
Linear polarization resistance – LPR
The linear polarization resistance (LPR) was also evaluated under the same conditions as the other electrochemical analysis, and the inhibition efficiency was calculated from EquationEquation 2(2)
(2) . The values of the polarization resistance in the presence (Rp) and absence (R0p) of the plant extract are described in .
Table 3. LPR parameters for carbon steel AISI 1020 in the solution of H2SO4 1.0 mol L−1 in the absence and presence of the ethanol extract of J. brandegeeana.
Good linear correlation coefficients were obtained for the blank and the other extract concentrations, that the Rp value is dependent on the concentration, complementing the results obtained in EIS and PP tests. At the highest concentration, 1500 ppm, the adsorption of the inhibitor to the metal surface was more efficient, with 88.62% inhibition, minimizing the corrosion intensity in the metal.
Weight loss measurements
The initial weight loss tests were performed in carbon steel coupons AISI 1020, with an area of 19.80 cm2, in the presence of the ethanol extract of J. brandegeeana with variation of extract concentration and immersion time at a fixed temperature of 30°C. shows the results of these tests with their respective standard deviations.
Table 4. Results of corrosion rates (Wcorr in mg cm2 h−1) in the absence and presence of ethanol extract of J. brandegeeana in H2SO4 1.0 mol L−1 against carbon steel AISI 1020, and inhibition efficiency η (%) at 30°C with variation of immersion time and inhibitor extract concentration.
When comparing the values of blank Wcorr and extracts, it was observed that there was a considerable decay of the values of the inhibition rates, indicating an inhibitory effect of the plant extract. It is also noted that with the increase of extract concentration occurred the increase of corrosion inhibition, which was more pronounced after 6 h of immersion, may thus be considered that the increased concentration of the extract caused the formation of a protective layer on the metal surface (Citation4).
When analyzing the values of η (%) in 24 h of immersion stability of the protective layer was formed, and at 48 h of immersion there was a decay in efficiency, which can be explained by a gradual desorption of the extract that interacted with the metallic surface (Citation46, Citation47). Thus, among the results, the time of 6 h stands out as the best time evaluated, presenting 93.54% inhibition efficiency at 1500 ppm.
Among the concentrations studied, those that presented better inhibitory results were 1000 and 1500 ppm and considering the small difference between the inhibition efficiencies of both, it was decided to follow with the concentration of 1000 ppm for the other evaluations.
Weight loss with temperature variation
The temperature effect was also studied for the ethanol extract of J. brandegeeana, maintaining the concentration of 1000 ppm, and varying the temperature at 30°C, 40°C, 50°C, 60°C and 70°C, as shown in .
Table 5. Results of corrosion rates (Wcorr in mg cm2 h−1) and efficiency of inhibition η (%) with 3 h of immersion in H2SO4 1.0 mol L−1 against carbon steel AISI 1020, and temperature variation in the absence and presence of the ethanol extract of J. brandegeeana.
With the increase in temperature, there was a decrease in inhibition efficiency, and consequently an increase in Wcorr; however, this decay can be considered mild, indicating thermal stability of the extract, with a variation of 6% considering if the increase in temperature from 30°C to 70°C, providing a good inhibition efficiency at 70°C (η = 82.43%). This result also indicates that the corrosion inhibition process occurs by physisorption, that is, the adsorption between the plant extract and the metal surface, occurs mainly through interactions by van der Waals forces, indicating a reversible adsorption mechanism.
From the results of the weight loss obtained, the physicochemical parameters involved in the corrosion process were calculated for the ethanol extract from the equations of Arrhenius (EquationEquation 8(8)
(8) ) and Eyring (EquationEquation 9
(9)
(9) ), as shown previously. All the results are described in and the graphs generated in .
(8)
(8) where Wcorr is the rate of corrosion in the presence of extract, Ea activation energy, R gas constant, T temperature in Kelvin, and A frequency factor.
(9)
(9) where Wcorr is the rate of corrosion in the presence of extract, T temperature in Kelvin, Kb Boltzmann constant, h Planck constant, R gas constant, ΔS≠ apparent entropy of transition, and ΔH≠ apparent enthalpy of transition.
Figure 9. Graphs of (A) Arrhenius and (B) Eyring for the corrosive process of carbon steel AISI 1020 in H2SO4 1.0 mol L−1 in the absence (blank) and in the presence of the ethanol extract of J. brandegeeana at 1000 ppm.
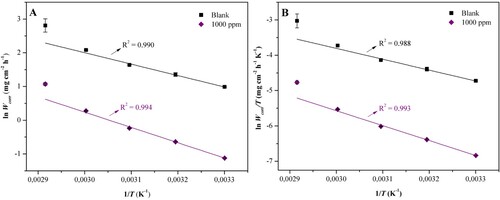
Table 6. Values of physicochemical parameters (Ea, ΔH≠, ΔS≠) associated with the corrosive process of AISI 1020 carbon steel in H2SO4 1.0 mol L−1 medium, in the absence and presence of the J. brandegeeana ethanol extract at 1000 ppm.
Higher values of Ea and ΔH≠ for the extract compared to blank indicated the need for a higher energy barrier to start the corrosive process, due to the formation of interactions between the extract and the metal surface (Citation13, Citation32). Regarding ΔS≠, a less negative value was observed with the addition of the plant extract, indicating a greater disorder in the system, due to the desorption of water molecules and sulfate ions of the metallic surface and adsorption of the plant extract (Citation4). This result characterizes a relevant mass transfer to the system, corroborating with the higher inhibition efficiency after 6 h of immersion, by the gravimetric technique of weight loss, suggesting a slow mass transfer, with a high induction time.
To verify if the efficiency obtained for Justicia brandegeeana is consistent with other plant extracts, a comparative study was carried out, shown in , with other vegetal species found in the literature, for carbon steel in H2SO4 in two different concentrations, being analyzed by the same method, weight loss. The maximum concentration used for J. brandegeeana is acceptablecompared to the other species studied in the same corrosive medium (H2SO4 1.0 mol L−1) because it showed higher inhibition efficiency by the weight loss method and same immersion time. In addition, plant species employed in a less corrosive medium (H2SO4 0.5 mol L−1) required longer immersion time to obtain inhibition efficiencies close to that found for J. brandegeeana.
Table 7. Comparison of the corrosion inhibition efficiency of Justicia brandegeeana with other vegetal inhibitors against carbon steel in H2SO4 by the weight loss method.
Adsorption isotherm
Corrosion inhibition is dependent on the adsorption capacity of the inhibitor on the metal surface, which can be evaluated by adsorption isotherms through different mathematical models, and it is necessary to calculate the degree of surface coverage by the inhibitor (θ). The values of θ can be obtained in a more reliable form using the gravimetric technique of weight loss through EquationEquation 10(10)
(10) (Citation16).
(10)
(10) where w0corr is the corrosion rate in the absence of an inhibitor and wcorr in the presence of an inhibitor.
The isothermal models of Frumkin, Temkin, Flory-Huggins, El-Awady, and Langmuir, Equations Equation11(11)
(11) –Equation15
(15)
(15) , respectively, were used to evaluate which would best suit the results obtained for the anti-corrosive effect of J. brandegeeana extract. The equations of the first four applied models did not show good linear correlation coefficients, as can be seen in .
(11)
(11)
(12)
(12)
(13)
(13)
(14)
(14)
(15)
(15) where θ is the degree of surface coverage by the inhibitor, C concentration, Kads adsorption constant, g is the degree of lateral interaction between adsorbed molecules, ηFH is the number of metal ions occupying the adsorption sites on two membranes, and y is the parameter of occupation of the active site.
Table 8. Adsorption isotherms for the anti-corrosive effect presented by the ethanol extract of J. brandegeeana in carbon steel AISI 1020 in the solution of H2SO4 1,0 mol L−1.
The best fit was obtained for the Langmuir isotherm, presenting a 0.99957 linear correlation coefficient and 1.06 slope, as shown in . The value of the slope near the unit confirms the suitability to the Langmuir isotherm, indicating the adsorption of the plant extract in monolayer, with each site containing only one adsorbed molecule, which does not suffer side interactions or stereo impediment between them (Citation16, Citation48).
Figure 10. Adsorption isotherm of Langmuir for the anti-corrosive effect presented by the ethanol extract of J. brandegeeana in carbon steel AISI 1020 in the solution of H2SO4 1.0 mol L−1.
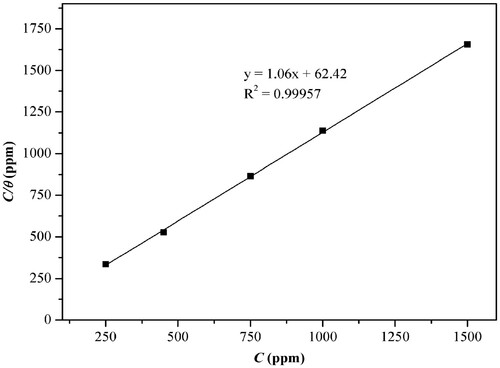
The adsorption constant (Kads) was calculated from the Langmuir isotherm, obtaining the value of 0.0160 L mg−1 that is comparable to founds in the literature for other plant extracts such as aqueous extract of coffee grounds (0.0571 L mg−1), the aqueous extract of Cumaru (0.0690 L mg−1) and the extract in a solution containing ethyl acetate, distilled water, and ethanol in the ratio 8:22:70 of Equisetum arvense (0.0166 L mg−1) (Citation42, Citation49, Citation50).
After obtaining the Kads, the adsorption standard free energy (ΔG0ads) was calculated by EquationEquation 16(16)
(16) , which assists in the evaluation of the interaction of the inhibitor with the metallic surface:
(16)
(16) where R is the universal constant of gases (J K−1 mol−1), T is the temperature in K, and
is the density of water (mg L−1) (Citation51, Citation52).
The value of −24.39 kJ mol−1 for ΔG0ads was obtained, indicating that the adsorption process of the inhibiting extract on the metallic surface is spontaneous. Moreover, according to the literature, values of ΔG0ads lower than −40 kJ mol−1 indicate that the corrosion inhibition process occurs by chemisorption, while values close to −20 kJ mol−1 or less negative indicate physisorption (Citation13, Citation53–56). Thus, for this system, the value of ΔG0ads indicates that the mechanism of corrosion inhibition must occur by physisorption, corroborating with the data found by the gravimetric method of weight loss with temperature variation, where it was observed a decrease in inhibitory efficiency with increasing temperature.
Chelating effect
The analysis of the chelating effect of the ethanol extract of Justicia brandegeeana against ions Fe+2 occurred in three modes: (I) only the extract in ethanol at 125 ppm; (II) extract solution in ethanol at 125 ppm and FeSO4 at 1.87 × 10−6 mol L−1 in the ratio 1:1 and (III) solution of the extract in ethanol at 125 ppm and FeSO4 at 1.87 × 10−6 mol L−1 in a 2:1 ratio.
The ultraviolet–visible spectrum with the three modes of analysis is presented in , where it is possible to observe in modes II and III a slight bathochromic displacement, for the red region and a hypochromic displacement, for lower absorbance values when compared to mode I, being more pronounced in the 1:1 ratio between the ethanol extract and Fe+2. This pronounced displacement demonstrates a change in the energy levels of the substances present in the extract, suggesting complexation with Fe+2.
Figure 11. Ultraviolet-visible spectra for the ethanol extract of Justicia brandegeeana (125 ppm) in the presence and absence of Fe+2 (1.87 × 10−6 mol L−1).
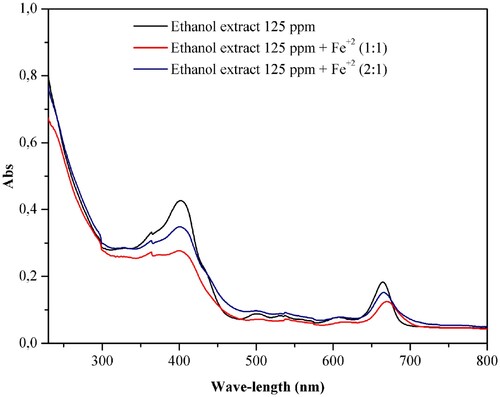
The bands are weakly displaced to longer wavelengths, the effect caused by the yellowish-green staining of the solution, which absorbs in violet at 400–435 nm, where it is possible to observe the wavelength of maximum absorption (Citation57). There is also the presence of two zones: (a) in the wavelength range of 399–403 nm that is related to the transitions π→π* of C = C; (b) connections, in the range of 664–670 nm related to n→π* transitions, assigned to groups C–O and OH that must be present in the substances that make up the plant extract (Citation58).
The overlays of the spectra quantified the chelating effect of the extract in ethanol of J. brandegeeana against Fe+2 ions. The positive control of EDTA at 2.32 × 10−4 mol L−1 showed an 83% chelating effect, while the plant extract in ethanol showed 54% for mode II, and 22% for mode III, confirming that the extract in ethanol forms complex with Fe+2, being more effective in the ratio 1:1. The chelating effect observed in the presence of Fe+2 ions may be related to the effect of corrosion inhibition by the extract in ethanol of J. brandegeeana.
SEM analysis
The micrographs of the three analyzed coupons are shown in . Coupon I, without immersion in any medium, has a smooth surface, visible with the marks of the treatment with the sandpapers.
Figure 12. Micrographs of SEM (2000× and 4000×) of carbon steel AISI 1020 without immersion in any medium (Coupon I); in corrosive medium H2SO4 1.0 mol L−1 in the absence of the extract in ethanol of the plant species for 24 h at 30°C (Coupon II); in corrosive medium H2SO4 1.0 mol L−1 in the presence of the plant extract in ethanol species at 1000 ppm for 24 h at 30°C (Coupon III).
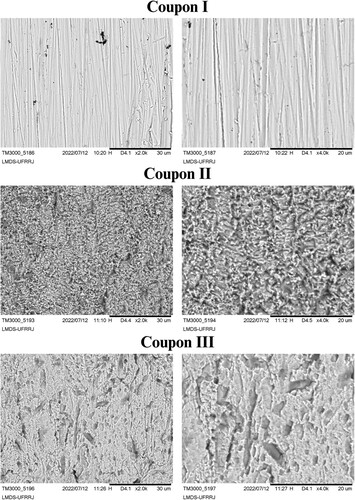
In coupon II, immersed in the blank solution, a roughness and irregular surface related to the acid attack is observed due to the metal deterioration caused by the presence of sulfuric acid, it is not possible to observe the sanding marks.
In coupon III, with the presence of the inhibitor at 1000 ppm, even not observed sanding marks and less rough, there is a significant improvement in the morphological aspect of the metal surface, thus being more preserved compared to coupon II, indicating a protection on the surface due to the formation of the protective layer, minimizing the attack of sulfuric acid.
The surface analysis ratifies the weight loss measurements and the electrochemical results showing the effectiveness of J. brandegeeana extract in the protection of carbon steel in the presence of a corrosive medium with sulfuric acid (1.0 mol L−1).
Conclusions
The aerial parts of Justicia brandegeeana presented high moisture contents, causing low yields. According to the infrared spectra performed for both collections, there is the presence of heteroatoms, such as oxygen. Likewise, the infrared spectra, together with the ultraviolet spectrum, confirmed the presence of classes containing electrons π, with their electronic transitions, as well as the NMR spectra that enabled the identification of the carbohydrate class.
The results of weight loss indicated that all concentrations studied for the extract in ethanol had good inhibitory efficiencies against carbon steel AISI 1020 in H2SO4 1.0 mol L−1 medium, being the best in the concentration of 1500 ppm (93.54% to 6 h of immersion) indicating that the increase in inhibition is dependent on the inhibitor concentration, as well as electrochemical analysis of EIS, PP, and LPR (88.49%, 84.47%, and 88.62%, respectively). It was also observed stability in the inhibition efficiency between 6 and 24 h of immersion, with decay at 48 h for all concentrations. It is noteworthy that the characteristics of the PP curves suggested that the extract behaves as a mixed inhibitor, with a tendency to the cathodic region. When evaluating the inhibition efficiency with temperature variation, the inhibitor showed good thermal stability in the wide range studied, presenting 82.41% inhibition efficiency at 70°C in 3 h of immersion, suggesting a physisorption process. The results were confirmed by the physicochemical parameters of Ea, ΔHǂ and ΔSǂ.
The adsorption of the extract in ethanol of J. brandegeeana to the metallic surface was observed according to the Langmuir isotherm, and the calculated Kads was adequate to other values for plant extracts in the literature, and the calculated value of ΔG0ads corroborated with the gravimetric results, confirming physical adsorption of the plant extract to the metallic surface. It was also observed that the ethanol extract showed chelating activity with Fe+2 ions, in a 1:1 ratio (54% chelating effect), supporting the results found in the analysis of anti-corrosive activity. In addition, the SEM provided the visualization of the morphology of the metallic surface in the presence and absence of the inhibitor, showing that in the presence of the plant extract, there is greater preservation and less roughness of the metallic surface, evidencing the formation of a protective film.
Disclosure statement
No potential conflict of interest was reported by the author(s).
References
- Economic Impact: Assessment of the global cost of corrosion. http://impact.nace.org/economic-impact.aspx Access in Dec 27, 2022.
- Kobzar, Y.L.; Fatyeyeva, K. Ionic Liquids as Green and Sustainable Steel Corrosion Inhibitors: Recent Developments. Chem. Eng. J. 2021, 425, 131480.
- Alamri, A.H. Localized Corrosion and Mitigation Approach of Steel Materials Used in Oil and Gas Pipelines – An Overview. Eng. Fail. Anal. 2020, 116, 104735.
- Cordeiro, R.F.B.; Belati, A.J.F.; Perrone, D.; D’Elia, D. Coffee Husk as Corrosion Inhibitor for Mild Steel in HCl Media. J. Electrochem. Sci. 2018, 13, 12188–12207.
- Hossain, N.; Chowdhury, M.A.; Kchaou, M. An Overview of Green Corrosion Inhibitors for Sustainable and Environment Friendly Industrial Development. J. Adhesion Sci. Technol. 2020, 35, 673–690.
- Umoren, S.A.; Solomon, M.M.; Obot, I.B.; Suleiman, R.K. A Critical Review on the Recent Studies on Plant Biomaterials as Corrosion Inhibitors for Industrial Metals. J. Ind. Eng. Chem. 2019, 76, 91–115.
- Verma, D.K.; Dewangan, Y.; Dewangan, A.K.; Asatkar, A. Heteroatom-Based Compounds as Sustainable Corrosion Inhibitors: An Overview. J. Bio-Tribo-Corros. 2021, 7, 1–18.
- Panchal, J.; Shah, D.; Patel, R.; Shah, S.; Prajapati, M.; Shah, M. Comprehensive Review and Critical Data Analysis on Corrosion and Emphasizing on Green Eco-Friendly Corrosion Inhibitors for Oil and Gas Industries. J. Bio Tribo-Corros. 2021, 7, 1–29.
- Thomas, A.; Prajila, M.; Shainy, K.M.; Joseph, A. A Green Approach to Corrosion Inhibition of Mild Steel in Hydrochloric Acid Using Fruit Rind Extract of Garcinia Indica (Binda). J. Mol. Liq. 2020, 312, 113369.
- Salleh, S.Z.; Yusoff, A.H.; Zakaria, S.K.; Taib, M.A.A.; Seman, A.A.; Masri, M.N.; Mohamad, M.; Mamat, S.; Sobri, S.A.; Ali, A.; Teo, P.T. Plant Extracts as Green Corrosion Inhibitor for Ferrous Metal Alloys: A Review. J. Cleaner Prod. 2021, 304, 127030.
- Sivakumar, P.R.; Srikanth, A.P. Sadhana - Acad. Proc. Eng. Sci. 2020, 45, 1–11.
- Wei, H.; Heidarshenas, B.; Zhou, L.; Hussain, G.; Li, Q.; Ostrikov, K. Green Inhibitors for Steel Corrosion in Acidic Environment: State of Art. Mater. Today Sustain. 2020, 10, 100044.
- Dehghani, A.; Bahlakeh, G.; Ramezanzadeh, B. A Detailed Electrochemical/Theoretical Exploration of the Aqueous Chinese Gooseberry Fruit Shell Extract as a Green and Cheap Corrosion Inhibitor for Mild Steel in Acidic Solution. J. Mol. Liq. 2019, 282, 366–384.
- Rocha, J.C.; Gomes, J.A.C.P. Inibidores de Corrosão Naturais – Proposta de Obtenção de Produtos Ecologicos de Baixo Custo a Partir de Resíduos Industriais. Matéria (Rio J.). 2018, 22, 11927.
- Santana, C.A.; Cunha, J.N.; Rodrigues, J.G.A.; Greco-Duarte, J.; Freire, D.M.G.; D’Elia, E. Aqueous Extracts of the Castor Beans as a Corrosion Inhibitor of Mild Steel in HCl Media. J. Braz. Chem. Soc. 2020, 31, 1225–1238.
- Valbon, A.; Ribeiro, B.F.; Soares, M.A.F.; Oliveira, M.C.C.; Neves, M.A.; Echevarria, A. Extrato de Hibisco-Colibri Como Inibidor Verde de Corrosão do aço-Carbono em ácido Sulfúrico. Quím. Nova. 2019, 42, 797–802.
- Kadiri, L.; Galai, M.; Ouakki, M.; Essaadaoui, Y.; Ouass, A.; Cherkaoui, M.; Rifi, E.; Lebkiri, A. Coriandrum Sativum. L Seeds Extract as a Novel Green Corrosion Inhibitor for Mild Steel in 1.0 M Hydrochloric and 0.5 M Sulfuric Solutions. Anal. Bioanal. Electrochem. 2018, 10, 249–268.
- Alvarez, P.E.; Fiori-Bimbi, M.V.; Neske, A.; Brandán, S.A.; Garvasi, C.A. Rollinia Occidentalis Extract as Green Corrosion Inhibitor for Carbon Steel in HCl Solution. J. Ind. Eng. Chem. 2018, 58, 92–99.
- Yao, X.; Qiang, Y.; Guo, L.; Xu, Q.; Wen, L.; Jin, Y. Renewable Low-Cost Brassica Rapa Subsp. Extract for Protection of Q235 Steel in H2SO4 Medium: Experimental and Modeling Studies. J. Ind. Eng. Chem. 2022, 114, 427–437.
- Al-Moubaraki, A.H.; Chaouiki, A.; Alahmari, J.M.; Al-Hammadi, W.A.; Noor, E.A.; Al-Ghamdi, A.A.; Ko, Y.G. Development of Natural Plant Extracts as Sustainable Inhibitors for Efficient Protection of Mild Steel: Experimental and First-Principles Multi-Level Computational Methods. Materials 2022, 15, 8688.
- Gu, T.; Xu, Z.; Zeng, X.; Fu, A.; Zhang, F.; Al-Zaqri, N.; Chen, J.; Tan, B.; Li, W. Lycium Barbarum Leaf Extract as Biodegradable Corrosion Inhibitor for Copper in Sulfuric Acid Medium. Ind. Crops Prod. 2023, 203, 117181.
- Golshani, Z.; Arjmand, F.; Amiri, M.; Hosseini, S.M.A.; Fatemi, S.J. Investigation of Dracocephalum Extract Based on Bulk and Nanometer Size as Green Corrosion Inhibitor for Mild Steel in Different Corrosive Media. Sci. Rep. 2023, 13, 913.
- O’Neill, C.S. Anatomy of the Shrimp Plant, Justicia Brandegeana (Acanthaceae). SURG J. 2010, 3, 41–47.
- Freidenreich, A.; Barraza, G.; Jayachandran, K.; Khoddamzadeh, A.A. Precision Agriculture Application for Sustainable Nitrogen Management of Justicia Brandegeana Using Optical Sensor Technology. Agriculture 2019, 9, 98.
- Jiang, X.; Xie, Y.; Li, J.; Ning, D. Essential Oil Composition of Justicia Brandegeeana. Chem. Nat. Comp. 2014, 50, 149–150.
- Shehawy, A.A.; Ibrahim, M.T.; Aboutaleb, E.S.; Qari, S.H. Bioactivity and Biochemical Efficacy of Chitinase and Justicia Brandegeana Extract Against Red Palm Weevil Rhynchophorus Ferrugineus Olivier (Coleoptera: Curculionidae). Food Sci. Nutr. 2020, 8, 4625–4636.
- Ribeiro, S.M.; Bonilla, O.H.; Lucena, E.M.P. Influência da Sazonalidade e do Ciclo Circadiano no Rendimento e Composição Química dos óleos Essenciais de Croton spp. da Caatinga. Iheringia Ser. Bot. 2018, 73, 31–38.
- Satapathy, A.K.; Gunasekaran, G.; Sahoo, S.C.; Amit, K.; Rodrigues, P.V. Corrosion Inhibition by Justicia Gendarussa Plant Extract in Hydrochloric Acid Solution. Corros. Sci. 2009, 51, 2848–2856.
- Duquesnoy, E.; Castola, V.; Casanova, J. Identification and Quantitative Determination of Carbohydrates in Ethanolic Extracts of Two Conifers Using 13C NMR Spectroscopy. Carbohydr Res. 2008, 343, 893–902.
- Shehawy, A.A.; Ibrahim, M.T.; Alrefaei, A.F.; Mohamed, H.A.-D.; Aboutaleb, E.S.; Kadasah, S. Assessment of Antitumor Activity of β-Sitosterol-β-D-Glucoside Isolated and Identified from Justicia Brandegeana. Int. J. Nutr. Food Sci. 2022, 11, 159–169.
- Frauches-Santos, C.; Bauerfeldt, G.F.; Echevarria, A. Corrosion Inhibition of Carbon Steel in HCl and H2SO4 Acids by Novel 1,3,4-Thiadiazolium-2-Phenylamine Salts. Int. J. Electrochem. Sci. 2018, 13, 6978–6995.
- Guruprasad, A.M.; Sachin, H.P. Novel Cost-Effective Aqueous Amorphophallus Paeoniifolius Leaves Extract as a Green Corrosion Inhibitor for Mild Steel Corrosion in Hydrochloric Acid Medium: A Detailed Experimental and Surface Characterization Studies. Chem. Data Collect. 2021, 34, 100734.
- Carlos, M.F.P.; Barboza, G.K.P.; Echevarria, A. Anticorrosive Effect of Halogenated Aniline Enaminoesters on Carbon Steel in HCl. Int. J. Corros. 2022, 2022, 7218063.
- Dzulkifli, N.N.; Razali, N.Z.K.; Sahani, N.I.; Ghazali, S.A.I.S.M.; Abidin, D.Z.; Abdullah, A.; Nor, N.M. A Brief Review on Corrosion Inhibition Study of Organic Ligand: Electrochemical, Morphology, and Isotherm Studies. Malaysian J. Anal. Sci. 2022, 26, 867–883.
- Olasunkanmi, L.O.; Ebenso, E.E. Experimental and Computational Studies on Propanone Derivatives of Quinoxalin-6-yl-4,5-Dihydropyrazole as Inhibitors of Mild Steel Corrosion in Hydrochloric Acid. J. Colloid Interface Sci. 2020, 561, 104–116.
- Fernandes, C.M.; Fagundes, T.D.F.; Santos, N.E.; Rocha, T.S.M.; Garrett, R.; Borges, R.M.; Muricy, G.; Valverde, A.L.; Ponzio, E.A. Ircinia Strobilina Crude Extract as Corrosion Inhibitor for Mild Steel in Acid Medium. Electrochim. Acta. 2019, 312, 137–148.
- Guimarães, T.A.S.; Cunha, J.N.; Oliveira, G.A.; Silva, T.U.; Oliveira, S.M.; Araújo, J.R.; Machado, S.P.; D’Elia, E.; Rezende, M.J.C. Nitrogenated Derivatives of Furfural as Green Corrosion Inhibitors for Mild Steel in HCl Solution. J. Mater. Res. Technol. 2020, 9, 7104–7122.
- Ma, I.A.W.; Ammar, S.; Kumar, S.S.A.; Ramesh, K.; Ramesh, S. A Concise Review on Corrosion Inhibitors: Types, Mechanisms and Electrochemical Evaluation Studies. J. Coat. Technol. Res. 2022, 19, 241–268.
- Murmu, M.; Saha, S.K.; Murmu, N.C.; Banerjee, P. Effect of Stereochemical Conformation into the Corrosion Inhibitive Behaviour of Double Azomethine Based Schiff Bases on Mild Steel Surface in 1 mol L−1 HCl Medium: An Experimental, Density Functional Theory and Molecular Dynamics Simulation Study. Corros. Sci. 2019, 146, 134–151.
- Souza, A.V.; Rocha, J.C.; Gomes, J.A.C.P.; Palermo, L.C.M.; Mansur, C.R.E. Development and Application of a Passion Fruit Seed Oil Microemulsion as Corrosion Inhibitor of P110 Carbon Steel in CO2-Saturated Brine. Colloids Surf. A: Physicochem. Eng. Asp. 2020, 599, 124934.
- Carlos, M.F.L.P.; Valbon, A.; Neves, M.A.; Santos, M.R.L.; Echevarria, A. Synergy Between Experimental and Theoretical Investigations Reveals the Anti-Corrosion Efficiency of Imine-Chalcones. J. Braz. Chem. Soc. 2021, 32, 1654–1669.
- Teixeira, V.M.; Oliveira, G.A.; Rezende, M.J.C.; D’Elia, E. Aqueous Extract of Cumaru (Dipteryx Odorata) Seeds as Corrosion Inhibitor for Mild Steel in Hydrochloric Acid Solution. J. Braz. Chem. Soc. 2021, 32, 413–428.
- Tachibana, M.; Ishida, K.; Wada, Y.; Aizawa, M.; Fuse, M. Study of Polarization Curve Measurement Method for Type 304 Stainless Steel in BWR High Temperature-High Purity Water. J. Nucl. Sci. Technol. 2009, 46, 132–141.
- Xu, B.; Yang, W.; Liu, Y.; Yin, X.; Gong, W.; Chen, Y. Experimental and Theoretical Evaluation of Two Pyridinecarboxaldehyde Thiosemicarbazone Compounds as Corrosion Inhibitors for Mild Steel in Hydrochloric Acid Solution. Corros. Sci. 2014, 78, 260–268.
- Haque, J.; Srivastava, V.; Quraishi, M.A.; Chauhan, D.S.; Lgaz, H.; Chung, I. Polar Group Substituted Imidazolium Zwitterions as Eco-Friendly Corrosion Inhibitors for Mild Steel in Acid Solution. Corros. Sci. 2020, 172, 108665.
- Karki, N.; Neupani, S.; Chauldhari, Y.; Gupta, D.K.; Yadav, A.P. Berberis Aristata: A Highly Efficient and Thermally Stable Green Corrosion Inhibitor for Mild Steel in Acidic Medium. Anal. Bioanal. Electrochem. 2020, 12, 970–988.
- Aziz, I.A.A.; Abdulkareem, I.A.; Anoon, I.A.; Hanoon, M.M.; Alkaabi, M.H.; Shaker, L.M.; Al-Amiery, A.A.; Kadhum, A.A.H. Corrosion Inhibition Potential of a New Corrosion Inhibitor for Mild Steel in 1 M Hydrochloric Acid Solution Determined by Weight Loss Technique, Complemented With Adsorption Studies and DFT Calculations. Int. J. Corros. Scale Inhib. 2022, 11, 64–81.
- Kokalj, A. Corrosion Inhibitors: Physisorbed or Chemisorbed?. Corros. Sci. 2022, 196, 109939.
- Li, H.; Zhang, S.; Qiang, Y. Corrosion Retardation Effect of a Green Cauliflower Extract on Copper in H2SO4 Solution: Electrochemical and Theoretical Explorations. J. Mol. Liq. 2021, 321, 114450.
- Victoria, S.N.; Prasad, R.; Manivannan, R. Psidium Guajava Leaf Extract as Green Corrosion Inhibitor for Mild Steel in Phosphoric Acid. Int. J. Electrochem. Sci. 2015, 10, 2220–2238.
- Verma, G.; Mishra, M. Development and Optimization of UV-Vis Spectroscopy-a Review. World J. Pharm. Res. 2018, 7, 1170–1180.
- Haldhar, R.; Prasad, D.; Bahadur, I.; Dagdag, O.; Kaya, S.; Verma, D.K.; Kim, S. Investigation of Plant Waste as a Renewable Biomass Source to Develop Efficient, Economical and Eco-Friendly Corrosion Inhibitor. J. Mol. Liq. 2021, 335, 116184.
- Saxena, A.; Prasad, D.; Haldhar, R.; Singh, G.; Kumar, A. Use of Sida Cordifolia Extract as Green Corrosion Inhibitor for Mild Steel in 0.5 M H2SO4. J. Environ. Chem. Eng. 2018, 6, 694–700.
- Rathod, M.R.; Minagalavar, R.L.; Rajappa, S.K. Effect of Artabotrys Odoratissimus Extract as an Environmentally Sustainable Inhibitor for Mild Steel Corrosion in 0.5 M H2SO4 Media. J. Indian Chem. Soc. 2022, 99, 100445.
- Muthukrishnan, P.; Prakash, P. Adsorption and Charge Transfer Resistance Behavior of Ficus Hispida Leaf Extract on Mild Steel Against Acid Attack. J. Fail. Anal. Prev. 2020, 20, 1803–1809.
- Karki, N.; Neupane, S.; Chauldhari, Y.; Gupta, D.K.; Yadav, A.P. Equisetum Hyemale: A New Candidate for Green Corrosion Inhibitor Family. Int. J. Corros. Scale Inhib. 2021, 10, 206–227.
- Odidika, C.C.; Ajiwe, V.I.E.; Eboagu, N.C.; Awuzie, C.H. Corrosion Inhibitive Action of Euphorbia Heterophylla Leaves Extract on Mild Steel in 1 M H2SO4 Solution. Sci. J. Anal. Chem. 2020, 5, 1–8.
- Deyab, M.A.; Mohsen, Q.; Guo, L. Theoretical, Chemical, and Electrochemical Studies of Equisetum Arvense Extract as an Impactful Inhibitor of Steel Corrosion in 2 M HCl Electrolyte. Sci. Rep. 2022, 12, 1–14.
- Ituen, E.; Akaranta, O.; James, A. Evaluation of Performance of Corrosion Inhibitors Using Adsorption Isotherm Models: An Overview. Chem. Sci. Int. J. 2017, 18, 1–34.
- Torres, V.V.; Amado, R.S.; Sá, C.F.; Fernandez, T.L.; Riehi, C.A.S.; Torres, A.G.; D’Elia, E. Inhibitory Action of Aqueous Coffee Ground Extracts on the Corrosion of Carbon Steel in HCl Solution. Corros. Sci. 2011, 53, 2385–2392.
- Golchinvafa, A.; Anijdan, S.H.M.; Sabzi, M.; Sadeghi, M. The Effect of Natural Inhibitor Concentration of Fumaria Officinalis and Temperature on Corrosion Protection Mechanism in API X80 Pipeline Steel in 1 M H2SO4 Solution. Int. J. Press. Vessel. Pip. 2020, 188, 104241.
- Kadapparambil, S.; Yadav, K.; Ramachandran, M.; Selvam, N.V. Electrochemical Investigation of the Corrosion Inhibition Mechanism of Tectona Grandis Leaf Extract for SS304 Stainless Steel in Hydrochloric Acid. Corros. Rev. 2017, 35, 111–121.
- Helen, L.Y.S.; Rahim, A.A.; Saad, B.; Saleh, M.I.; Raja, P.B. Aquilaria Crassna Leaves Extracts – A Green Corrosion Inhibitor for Mild Steel in 1 M HCl Medium. Int. J. Electrochem. Sci. 2014, 9, 830–846.