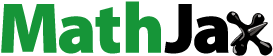
ABSTRACT
Cr (VI) compounds find wide uses in the industrial sector, such as wood preservation, tanning, chrome plating, and anticorrosive agents. Several kinds of both natural and industrial factors can lead to Cr (VI) contamination in wastewater. Human exposure to the hazardous metal ion Cr (VI) can lead to a variety of health problems. The Cr (VI) undergoes a transition into a lower oxidation state once it enters the cell, causing oxidative stress and harming the organelles. Not only do sugar-phosphate backbones, histones, and base pairing of DNA suffer from Cr (VI) induced genotoxicity, but chromosomes are also impacted. Because Cr (VI) is more hazardous compared to other metals, it needs to be removed from water and wastewater. It is feasible to remove Cr (VI) using physiochemical methods, but they are expensive and produce hazardous sludge. Therefore, there is an urgent need for an economical and eco-friendly Cr (VI) removal technique. Biological techniques including biosorption, bioreduction, and bioaccumulation are regarded as economical and environmentally beneficial techniques. The sources of Cr (VI), toxicity, and green technology for Cr (VI) removal were the authors’ main points of emphasis in this review.
1. Introduction
The cause of the Cr (VI) pollution in the water is human activity; the elemental or metallic form of Cr (VI) is not frequently found on Earth. On the other hand, it is mainly found as ionic species or as compounds (Citation1). Chromium-containing substances come in a variety of forms, such as potassium dichromate, sodium dichromate, sodium chromate, and chromite (Citation2). The most prominent of them is chromite (FeCr2O4), which is recognized as the principal commercial source of chromium (Citation3). About 67% of all chromium used in various sectors is used in the metallurgical industry, where chromium is extensively used (Citation4). A thorough list of hazardous compounds has been collaboratively created by the Environmental Protection Agency (EPA) and the Agency for hazardous compounds and Disease Registry (ATSDR), taking into consideration the possible health concerns associated with each substance as well as its level of toxicity. Accordingly, the most dangerous heavy metals or chemicals have been given the highest place on the ATSDR substance priority list. Arsenic is ranked as the most poisonous element of primary concern, holding the top spot in this prioritization. Interestingly, Cr (VI) has a relatively low toxicity ranking compared to other drugs, as seen by its 17th position on the ATSDR's substance priority list (Citation5). It is essential to recognize that Cr (VI) pollution may impact not just humans but also a variety of other living species via several different pathways, including skin contact, inhalation through the respiratory system, and food contamination (Citation5, Citation6).
The environment is contaminated by Cr (VI) due to a variety of human activities. The oxidation of Cr (III) also results in the presence of Cr (VI) in water (Citation7). Cr (III) may be changed into Cr (VI) by several naturally occurring oxides, including manganese dioxide (Citation8). These chromium pollution incidents cause a range of health problems in people and other living things. For instance, exposure to tainted drinking water might result in several digestive tract cancers (Citation9). Chromium in its highly reactive state, Cr (VI), can pass right through cell membranes. Because of their structural similarity to phosphate and sulphate ions, chromate ions can pass across cell membranes through sulphate transporters (Citation10). The activity of chromate reductases transforms the deadly Cr (VI) into a less dangerous oxidation state inside of cells. Free radicals, however, are produced during the reduction process and have the potential to harm proteins, DNA, and other cell components (Citation11). Moreover, Cr (III) and DNA can combine to create a complex known as Cr (III)-DNA, which eventually causes DNA mutations (Citation12).
When water or food components have more Cr (VI) than is permitted, several harmful consequences occur. Because of its extreme toxicity, Cr (VI) presence in contaminated water must be removed (Citation13). A variety of established methods, such as physical adsorption, oxidation, and chemical reduction, have been utilized in the purification process of chromium (VI) and other metal ions (Citation14, Citation15). The elimination of heavy metals from wastewater has been achieved through the utilization of numerous nanomaterials (Citation16–18). Furthermore, composite materials have proven to be effective adsorbents for the extraction of heavy metals (Citation19–24). Additionally, polymeric materials have exhibited their proficiency in removing heavy metals and dyes as adsorbents (Citation25–29). Some limitations of conventional systems encompass the high operational expenses, substantial energy requirements, and the generation of harmful sludge (Citation30). Consequently, it is of utmost importance to explore a financially feasible and environmentally sustainable approach for the eradication of chromium (VI). For this application, several environmentally benign techniques including phytoremediation, biosorption, bioreduction, and bioaccumulation are seen to be the best options (Citation31).
Common biosorption methods include the use of algae, bacteria, fungi, and plant biomass. Through the interaction of several functional groups on the surface of the biosorbent, including carboxyl, nitrogenous, and hydroxyl groups, Cr (VI) attaches to the biosorbent throughout this process (Citation32). Heavy metals can be absorbed by living microorganisms through a process called bioaccumulation (Citation30, Citation33). Antioxidants and chromate reductases work inside the cell to convert the more hazardous Cr (VI) into the less harmful Cr (III) (Citation13, Citation34). Because of their short lifetime, great resistance to Cr (VI), simplicity of cultivation, and broad availability, bacteria are a good choice for the decontamination of Cr (VI) (Citation35). The bacterium species M. paraoxydans was identified by Singh and Mishra (Citation7) from water tainted with coal mining waste. This species showed a remarkable capacity to reduce Cr (VI) (99.96%) at an initial concentration of 50 mg/L. Li et al. (Citation36) found that the microbial strain B. cereus 332 could remove 99.9% of Cr (VI) from polluted soil after incubating it for 24 h. A bacterial species linked to Mammaliicoccus sciuri tannery effluent was identified by Plestenjak et al. (Citation37) and their 50% removal efficiency of Cr (VI) was reported. The many sources of Cr (VI) pollution, the health hazards they pose, including genotoxic consequences, and the biological techniques used to detoxify Cr (VI) are the main topics of this paper.
2. Source of Cr(VI)
Numerous studies have shown that chromium is widely used in a variety of industries, including the chemical, metallurgical, and leather sectors (Citation38). There are other varieties of it, such as potassium dichromate (K2Cr2O7), ammonium dichromate ((NH4)2Cr2O7), and sodium dichromate (Na2Cr2O7) (Citation39). Scientific studies have clarified that chromium usually appears in the form of Cr (III) in nature (Citation40). However, it's important to keep in consideration that several studies have shown that human activities such as those carried out in coal mines, tanneries, and chrome plating industries are the primary sources of Cr (VI) contamination. It has been discovered that these activities are significant contributors to the environmental concentration of Cr (VI), which poses a concern to human health and ecosystems. Thus, understanding the causes and effects of Cr (VI) pollution is crucial for developing mitigation and management plans that will effectively protect the environment and advance sustainable industrial practices (Citation41).
2.1. Natural sources
While a range of human activities may be included with the genesis of Cr (VI), natural sources also contribute significantly to its existence in various environmental sources. Some major natural processes responsible for Cr (VI) contamination in water are described in .
In several countries, including Mexico, Italy, and California, Cr (VI) has been found in groundwater at concentrations between 0.05 and 0.073 mg/L (Citation42, Citation43). Ultramafic rocks provide the most abundant occurrence of naturally existing chromium, primarily in the form of chromite, manesio chromite, and various other compounds (Citation8). The process of transforming trivalent chromium into hexavalent chromium is heavily dependent on environmental factors. In the presence of trivalent chromium in both surface and groundwater, in conjunction with other oxides such as oxyhydroxides, manganese oxides, and ferric oxide (Fe + 3), it undergoes a chemical conversion into the ionic hexavalent form. This conversion is influenced by a multitude of environmental conditions including temperature, pH, and the presence of certain minerals and organic matter. Of all these oxides, manganese oxides have the greatest capacity to oxidize Cr (III) (Citation43, Citation44). EquationEquation 1(1)
(1) illustrates how trivalent chromium oxidizes to hexavalent chromium.
(1)
(1) Moreover, hydrogen peroxide has strong oxidizing abilities that cause Cr (VI) to change into a lower oxidation state. Cr (VI) oxidizes at an exceptionally fast rate when exposed to H2O2 (Citation45). Moreover, chromium tends to change from its trivalent to hexavalent state when exposed to air or dissolved oxygen. Even while dissolved oxygen in water sources helps chromium oxidize to Cr (III), it has very little role in Cr (VI) pollution. The production of Cr (VI) from its trivalent state is notably influenced by ambient oxygen (Citation45, Citation46). A variety of natural processes, such as forest fires, include the conversion of Cr (III) into Cr (VI), which is further facilitated by gaseous oxygen species (Citation46).
2.2. Anthropogenic source of Cr (VI) contamination
Human activities are the predominant source of Cr (VI) contamination found in both surface and groundwater systems. Waste materials containing Cr (VI) are produced by a variety of industrial processes and released into the environment as effluent (Citation5). Soil and water are contaminated with Cr (VI) when these waste products are disposed of directly into the environment (Citation47). illustrates a few examples of industrial operations that release Cr (VI) into their effluent.
Cr (VI) is necessary for tanning, a procedure used in the leather industry to transform unfinished animal hides and skins into usable leather (Citation48). This tanning technique, which modifies the structural composition of the collagen protein in leather, frequently uses chrome sulphate (Citation49). In this process, Cr (VI) plays a major role as a crosslinking agent. Two processes lead to the crosslinking of chromium: (a) the covalent connection of one chromium ion and two collagen molecules; and (b) the hydrogen bonding of protein molecules to chromium ions (Citation49, Citation50). However, there is a serious environmental risk associated with the direct release of industrial effluent containing Cr (VI) into water sources like rivers. This chromium contamination results in various toxicological effects on living organisms (Citation51). To safeguard metallic materials from the corrosive process, anti-corrosion coatings are employed to create a barrier between the materials and their external environment (Citation52). An ideal coating should possess thinness and low porosity, as these characteristics make it an effective corrosion inhibitor. Moreover, a less porous polyaniline film provides the best protection against corrosion. Vehicles and other metallic components are better protected by the resulting Cr (VI) layer (Citation53). For anti-corrosion coating purposes, different chromium-containing compounds, such as ZnCrO4 and SrCrO4, are utilized. However, the use of these compounds leads to various forms of Cr (VI) contamination in water sources (Citation54, Citation55).
In the steel industries, Cr (VI) is widely used in a variety of industries, including automobiles, domestic activities, and industrial equipment construction (Citation56). The stainless steel industry uses a lot of chromium species, including (NH4)2Cr2O7 and K2Cr2O7, which unfortunately contributes to the Cr (VI) pollution in wastewater (Citation54). Chromated copper arsenate (CCA) is another insecticide that contains Cr (VI), As (V), and Cu (II). It is utilized as a wood preservative. Termites and other wood-decaying organisms are kept in CCA (Citation57). On the other hand, the preservative chemical slowly permeates into the environment when wood treated with CCA comes into touch with soil and water (Citation58). The contaminated soil and water are the result of these compounds including Cr (VI). Cr (VI) therefore seeps into the soil and contaminates groundwater (Citation59, Citation60). The existence of arsenic (V), chromium (VI), and copper (II) in wastewater has deleterious consequences for both land-dwelling and aquatic fauna. Owing to the propensity of these metallic elements to amass within fish and other organisms, they are capable of infiltrating the food web and inducing a plethora of malignancies in humans (Citation57). Additionally, chromium trioxide is employed in the protection of timber (Citation13). Humans can become polluted by chromium by eating contaminated food, breathing contaminated air, or coming into touch with contaminated dirt on their skin (Citation61).
The wastewater emanating from coal mines harbors a considerable amount of chemical substances that have the potential to result in diverse manifestations of harm to the environment (Citation62). The term ‘acid mine drainage’ refers to the wastewater that is produced by coal processing facilities. Coal mine effluent contains a wide range of heavy metals and other dangerous materials that pollute the ecosystem around us (Citation63). Cr (VI) is one of the most dangerous compounds discovered in coal washery effluents. Cr (VI) contamination results from the direct dumping of coal washery effluent into surface water sources (Citation64). This effluent has several deleterious effects on the biological system (Citation63, Citation64).
3. Cr (VI) induced toxicity
Cr (VI) is a well-known hazardous chemical whose ionic species and oxidation states have a significant impact on how toxic it is (Citation65). When compared to Cr (III), Cr (VI) is more reactive and soluble. Because of this, it may pass across the plasma membrane with ease and enter cellular compartments via phosphate transporters, anion transporters, and other cell surface receptors (Citation66). Cr (VI) is more hazardous because of its greater solubility and oxidative state (Citation67). The harm that Cr (VI) does to several organs, such as the kidneys, liver, and lungs, is indicative of its deleterious impact on human health (Citation68). shows the unique fatal effects of Cr (VI).
The carcinogenic properties of Cr (VI) and its detrimental impact on various organs, including the kidneys, liver, and heart, have been extensively investigated (Citation69). The administration of Cr (VI) caused damage to the kidneys in rats. Moreover, Bagchi et al. (Citation70) discovered that oral treatment with Cr (VI) led to lipid peroxidation in hepatic mitochondria and microsomes, along with an elevation in the levels of lipid metabolites in urine. It is important to recognize that Cr (VI) poses significant hazardous risks, such as chromosomal irregularities, the fragmentation of DNA strands, and the formation of pulmonary carcinoma (Citation71).
As theorized, the reduction of Cr (VI) indicates a mechanism of elimination that occurs outside the confines of the nucleus and other cellular organelles, thereby suggesting a process of detoxification. It is hypothesized that when this reduction reaction takes place extracellularly or at a distance from the nucleus and other cellular organelles, it aids in the neutralization and elimination of the hazardous effects associated with Cr (VI). However, if this reduction takes place intracellularly, it could lead to oxidative-mediated toxicity, thereby compromising the integrity of the organelles and inducing DNA modifications (Citation72). If, however, Cr (VI) undergoes a process of transformation into Cr (III) outside of the cell, this particular event serves as an obstacle in terms of allowing the reduced Cr (III) and any other intermediate constituents to enter the intracellular area, consequently preventing any negative consequences that have been documented (Citation73). Cr (VI) can enter the intracellular space and pass through the cell membrane before being converted to Cr (III) (Citation74). ROS, which are produced during this reduction process, add to the toxicity of cells. Studies show that an increase in ROS generation brought on by the Fenton reaction is the main cause of Cr (VI) toxicity (Citation75, Citation76). lists the several cell lineages that produce ROS and their negative consequences.
Table 1. The generation of ROS induced by Cr (VI) and their fatal consequences.
The amount of Cr (VI) given and the length of exposure are closely connected to the toxicity of Cr (VI). Cr (VI) causes the expression of genes related to the antioxidant system to decrease while concurrently inducing a rise in genes linked to apoptosis, such as p53 and Caspase (3 & 9) (Citation87). Extended exposure to Cr (VI) increases oxidative stress in cells and causes ROS production, which results in cell death (Citation87).
Reactive oxygen species (ROS) within the cell can harm proteins, DNA, RNA, and mitochondria. The primary mode of toxicity for Cr (VI) is disruption of transcriptional regulation (Citation88). The compromised state of normal gene expression pathways stems from the prevalence of complexation and DNA modifications in regions where DNA replication and transcription occur. Numerous studies, both in vitro and in vivo, have demonstrated that Cr (VI) induces oxidative stress by increasing the production of reactive oxygen species (ROS), thereby leading to the degradation of DNA, lipids, and enzymes (Citation89). The occurrence of oxidative stress caused by Cr (VI) results in various cellular processes, such as the generation of hydroxyl radicals and superoxide anions (Citation90). Moreover, in addition to its other detrimental effects, exposure to chromium (VI) has been found to result in an increase in lipid peroxidation, activation of protein kinase C, DNA damage, changes in gene expression, modulation of intracellular oxidized states, and ultimately, the induction of programed cell death known as apoptosis. As noted by Holmes et al. in their research publication (Citation91), the presence of chromium (VI) can disrupt crucial DNA replication and repair processes. This interference can impede the normal functioning of these vital cellular mechanisms, leading to potentially severe consequences. A study conducted by Bagchi et al. (Citation90) sought to explore the dose-dependent effects of chromium (VI) exposure in female mice. The findings of this study revealed significantly elevated levels of lipid peroxidation and the formation of reactive oxygen species (ROS) in both the liver and brain tissues of the exposed animals. These observations further underscore the harmful impact of chromium (VI) and highlight the need for continued research into its potential health hazards and the development of effective preventive measures. Son et al. propose that Cr (VI) induces necrosis or apoptosis in mouse skin epidermal cells, ultimately resulting in cell death. The rate of cell death is dependent on the quantity of Cr (VI) utilized. Moreover, there is an increase in caspase activation and mitochondrial membrane depolarization when Cr (VI) is present in the culture medium. Son et al. (Citation92) report that Cr (VI) activates p53, which in turn induces apoptosis in mouse skin epidermal cells that is both caspase-dependent and mediated by the mitochondria. shows how cellular organelles are harmed by ROS.
Figure 4. The generation of ROS caused by Cr (VI) and their involvement in the destruction of cell organelles.
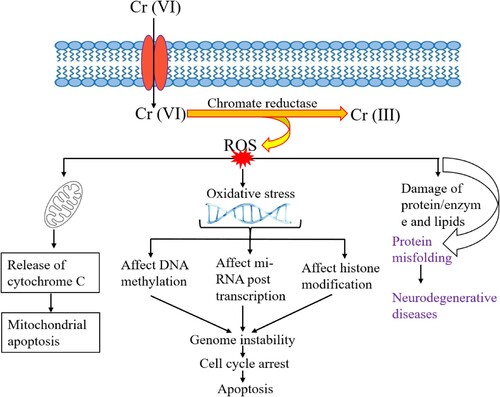
Histone modifications, miRNA expression, and DNA methylation are all altered by Cr (VI) (Citation83). Mitochondrial damage is caused by Cr (VI) oxidizing Trx. Trx must be kept in a decreased condition for cellular functions to function as best they can. The Trx system is essential for cell viability and for preserving a steady thiol redox equilibrium (Citation93).
4. Remediation of heavy metal ions
There are several methods for removing Cr (VI) from wastewater. Among the often-utilized physiochemical procedures are membrane separation, adsorption, coagulation, precipitation, ion exchange, and reverse osmosis (Citation94). It is often known that precipitation is a useful method for getting rid of Cr (VI). However these physiochemical methods are expensive, produce secondary chemical sludge, and work best when the water's heavy metal content is high (more than 2 mM) (Citation95). Given these shortcomings, it is imperative to create economical and eco-friendly techniques for the efficient removal of Cr (VI) from water (Citation96).
The elimination of hexavalent chromium (Cr (VI)) from wastewater through biological means is an especially appealing approach due to its cost-effectiveness and superior efficiency in comparison to conventional methods. A comprehensive investigation has been conducted to explore the utilization of diverse biological agents, such as plant biomass, agricultural waste, microbial biomass, green-synthesized nanoparticles, fruit waste, and biopolymers, in an extremely efficient manner to remove Cr (VI) contaminants from the liquid phase, particularly when faced with low concentrations of heavy metal ions. The works of Kumar et al (Citation97) and Abdelbasir et al (Citation98) provide comprehensive evidence of these investigations. In addition, it is a significant fact that living microbes-like fungi, algae, and bacteria have become more and more relevant in the field of heavy metal removal (Citation99). According to Vieira and Volesky (Citation100), these organisms have the potential to be more effective remediators since they can effectively sequester heavy metals inside their internal habitat by absorbing them from their surrounding medium. Moreover, the extremely poisonous Cr (VI) may be changed into the less hazardous Cr (III), which has lower toxicological characteristics and is notably less soluble in water, by some microbial species, as is widely known. Microorganisms can incorporate Cr (III) into their intracellular regions more easily as a result (Citation101). Therefore, several bioremediation techniques, including biosorption, phytoremediation, bioreduction, and bioaccumulation, have been carefully studied and used in the past to successfully remove Cr (VI) pollution (Citation102).
4.1. Biosorption
It is believed that biosorption is a separate metabolic process that mostly uses dead material. Toxic Cr (VI) ions bind to the outside of the biosorbent during this process. However, it is also carried out by live biomass. Furthermore, Cr (VI) ions are bound to the live cells by surface complexation on the cell surface or metabolic adsorption (Citation103, Citation104).
Wang and Chen (Citation105) provide observation on biosorption as the biological process of ion exchange, wherein hazardous Cr (VI) ions establish bonds with a variety of functional groups, including carboxyl, amide, imidazole, sulfonate, and amino groups. The attachment propensity of the functional groups, as well as the Cr (VI) ions, is influenced by the pKa value. The raw adsorbent material needs to have a reasonable price and convenient accessibility (Citation106). Furthermore, the quality of the biosorbent is impacted by the abundance and diversity of functional groups present on its surface (Citation107). The surface form plays a significant role in the adsorption of Cr (VI) ions, as a rough and porous surface provides more binding capacity on the biosorbent surface. The characterization of the surface form and functional groups of a biosorbent is of utmost importance. For biosorbent characterization, several techniques are available, including FT-IR, SEM, EDX, NMR, and XRD (Citation108, Citation109).
A few factors that influence biosorption are the kind of biomass, temperature, pH, and the presence of other metal ions (such as cations and anions) (Citation110). Positively charged metal ions compete with one another as the pH falls. On the other hand, surface deprotonation and binding group exposure result from raising the pH (Citation110, Citation111). Desorption is a method of regenerating the biosorbent in which the medium's pH is changed to recover the metal ions (Citation112). Moreover, the biosorption mechanism of Cr (VI) is a complex process that includes the binding of anionic Cr (VI) ions to positively charged groups and their reduction into Cr (III) chromium ions via several pathways, according to Netzahuatl-Munoz et al. (Citation113). The biosorption-cum-bioreduction of chromium (VI) ions usually involves three distinct processes, and these phases are commonly seen (Citation113).
In the initial step of the biosorption process, the negatively charged Cr (VI) ions attach themselves to the positively charged surface functional groups. The second step in the biosorption process is the reduction of Cr (VI) to Cr (III) via electron donor groups. These electron donor groups serve to speed up the reduction process. The third phase discusses the release of Cr (III) ions into the solution. The electrical repulsion that exists between the positively charged groups and the Cr (III) ions is what causes this release. As an alternative, the negatively charged groups of the biosorbent may bond with the Cr (III) ions (Citation114, Citation115).
It is also possible to use the biosorption technique in the suspension medium, which immobilizes the biomass. Numerous advantages come about due to this immobilization process, such as increased biosorbent stiffness, strength, and general durability, which in turn increases the method's effectiveness in removing Cr (VI) (Citation116). Polysulfide, alginate, polyurethane, and polyacrylamide are among the matrices that can be used in the immobilization processes (Citation117, Citation118). Notably, these matrices have been widely utilized for the immobilization of many types of biomass, including both plant and microbial origins. Sodium alginate matrices, for example, have proven to be an excellent means of immobilizing Chlorella homosphaera (Biomass) (Citation116, Citation119). Regarding Cr (VI) remediation, offers a thorough summary of the absorption capability demonstrated by several biosorbents.
Table 2. Cr (VI) biosorption capacity of different biosorbent.
Beyond biomass use, green synthesized nanoparticle application has attracted a lot of interest in the field of Cr (VI) removal. Other industries that have found use for these nanoparticles include wastewater treatment, energy, healthcare, agriculture, and electron devices (Citation127, Citation128). In general, nanoparticles are defined by their small size, which is around one to one hundred nanometers. Compared to bulk materials, these nanoparticles have a much greater surface area due to their small size (Citation129). Nanoparticles have several additional significant characteristics besides their small particle size, including the quantum and macro quantum tunnel effect (Citation127, Citation129). The fascinating reactivity and adsorption abilities of nanoparticles are caused by these particular characteristics. According to Vidu et al (Citation130), these very characteristics of nanomaterials make them very beneficial for the removal of Cr (VI) from polluted water sources. A selection of these nanomaterials and their corresponding Cr (VI) adsorption capabilities are shown in .
Table 3. Nanomaterials and their Cr (VI) adsorption capacity.
4.2. Bioaccumulation
The process of Cr (VI) ions building up inside the intracellular space is known as ‘bioaccumulation’ (Citation135). Compared to biosorption, this process is more complex and time-consuming since it includes many metabolic pathways (Citation135). Living cells engage in a metabolic process called bioaccumulation, which requires energy (Citation136). Benefits of bioaccumulation include a decrease in the number of steps required to prepare biosorbents, such as gathering, drying, preparing (washing and crushing), and storing biomass (Citation137). However, according to Ali et al (Citation138), the efficiency of bioaccumulation is very dependent on the experimental setup. Pollutants in the medium have the potential to aggregate on organisms’ surfaces, which can impact bioaccumulation (Citation139). It has been demonstrated that several plant, bacterial, algal, and fungal species are essential to the removal of Cr (VI).
4.2.1. Bacterial mediated remediation
Among these biological systems, bacteria are particularly resistant to heavy metals and may store metal ions inside their cells via certain receptors on the cell's surface (Citation140). Several bacterial genera have been identified for further study since it has been found that they have a high level of resistance to Cr (VI). These genera include Pseudomonas (Citation141), Klebsiella (Citation142), Microbacterium (Citation143), and Bacillus (Citation144). Furthermore, using bacteria in bioremediation procedures is seen to be an economically feasible and environmentally beneficial method (Citation145). According to Liu et al (Citation146), bacterial strains that inhabit Cr (VI)-contaminated areas frequently have unique resistance mechanisms against Cr (VI). Several microbacterium species have been identified from these polluted locations; according to Henson et al (Citation147), Microbacterium sp. (Cr-K29) can remove up to 88% of Cr (VI). Furthermore, a Cr (VI) lowering strain of Microbacterium liquefaciens was isolated from a polluted site by Pattanapipitpaisal et al (Citation148), and it showed remarkable bioremediation potential by eliminating 81% of Cr (VI) from contaminated water. To remove heavy metals, bacteria use a variety of methods. They can either produce soluble enzymes to detoxify the metal or use Cr (VI) as an electron acceptor (Citation149). Toxic metal ion exposure causes microbial cells to generate ROS, which can harm cell organelles and interfere with metabolic processes, impairing regular cell functioning (Citation150, Citation151). presents a compilation of significant bacterial species along with their respective efficacy in removing Cr (VI).
Table 4. Bacterial species and their Cr (VI) efficiency.
4.2.2. Microbial reduction of Cr (VI)
It is widely acknowledged that the reduction of Cr (VI) into a lower oxidation state is a significant phenomenon in mitigating its toxicity. Compared to Cr (III), the specific form of chromium known as Cr (VI) exhibits increased solubility, permeability, and reactivity, rendering it more hazardous. The reduction process converts Cr (VI) into Cr (III), which is less toxic and less soluble. The microbial reduction of Cr (VI) has proven to be a highly valuable and beneficial technique. In contrast to other physiochemical methods, these techniques not only offer cost-effective solutions but also have minimal ecological impact (Citation157, Citation158). According to Donati et al (Citation159), various types of anaerobic and aerobic bacteria secrete compounds and enzymes that play a role in reducing Cr (VI). The reduction of Cr (VI) under anaerobic conditions is known to occur at a sluggish pace. A membrane-bound enzymatic system is necessary to enable this reduction at specific rates. Conversely, the enzymes responsible for the aerobic reduction of Cr (VI) are primarily found in the cytosol in a soluble state (Citation160, Citation161). The efficiency of Cr (VI) reduction is contingent upon several factors, including the type of microbe present and the concentration of Cr (VI) in the growth medium used for microbial cultivation. Furthermore, the reduction of Cr (VI) can occur in both aerobic and anaerobic conditions and is influenced by temperature, components of the medium, and pH levels (Citation162, Citation163). There are three methods for reducing Cr (VI) (Citation164).
In anaerobic environments, several chemicals present in the cell protoplasm are involved in the reduction of Cr (VI). Glutathione, vitamins, carbs, amino acids, heme-containing proteins, and other constituents are necessary for the reduction of Cr (VI). They act as electron donors for the reduction process (Citation165).
On the other hand, extracellular soluble reductase enzymes are necessary for the reduction of Cr (VI) ions under aerobic conditions. The NADPH molecule facilitates this reduction process. The chromate-reducing enzymes secreted by microbial species like Desulfovibrio vulgaris and Pseudomonas putida PRS2000 cause this decrease. Both within and outside the bacterial cell, these enzymes may make use of several electron donors (Citation166). It is essential to remember that this process is energy-dependent and highly controlled. Moreover, the bacterial cell does not need to consume chromium ions for the extracellular chromate reduction to occur. Because it shields the bacterial cell from chromate-mediated DNA damage, this unique property is advantageous (Citation165, Citation166).
The electron donor components required for membrane-associated reductase-mediated reduction of Cr (VI) are glucose or H2. Cr (VI) takes up terminal electrons from the electron transport chain during this process (Citation167). Glucose has been identified as an external electron source in this mechanism by earlier research (Citation168). According to Ibrahim et al (Citation169), glucose has been shown to increase chromate reductase's enzymatic activity by about 3.5 times. Therefore, the use of glucose as an electron donor significantly affects the reduction of Cr (VI) (Citation169).
According to previously published research, there has been little study done on the chromate reductase genes of different bacterial species (Citation157). As previously mentioned (Citation170), the chromate reduction process has been thoroughly investigated in two well-known bacterial strains: Pseudomonas putida (with the ChrR gene) and Escherichia coli (with the YieF gene). It has been discovered that YieF and ChrR function as flavoproteins in the bacterial cytoplasm and can completely convert Cr (VI) to Cr (III). Temperature and the presence of electron donor groups are two recognized variables that affect the mechanism by which chromate reductase mediates the reduction of Cr (VI) (Citation170).
4.2.3. Mycoremediation
According to Archana et al (Citation171), fungi are extremely adaptable to their surroundings and demonstrate their ability to thrive in the natural breakdown of both organic and inorganic materials. The organisms have also proven their ability to flourish in extremely stressful environments, such as severe pH levels, temperatures, and salt concentrations (Citation172). Interestingly, macrofungi such as white rot fungi have a notable capacity to absorb large concentrations of harmful metal ions, such as Cr (VI), inside their fruit bodies. According to Ogbo and Okhuoya (Citation173), the unique characteristics of fungi, and specifically mushrooms, make them good candidates for the extraction of Cr (VI) ions from polluted areas. provides useful information on the effectiveness of different fungal species in eliminating Cr (VI).
Table 5. Fungi and their Cr (VI) removal capacities.
The fungi play an important role in eliminating Cr (VI) from wastewater. Pleurotus sp., a member of the macrofungi group, has greater benefits than other fungal species. Pleurotus sp. is known to be superior when it comes to cleaning polluted soil and water. Moreover, Pleurotus sp. has the amazing ability to grow on a variety of solid substrates (biomass) without difficulty, even in harsh environmental circumstances. Because of its versatility, it can be grown widely, which makes it a very popular choice for researchers as biosorbent (Citation180). Apart from its remedial properties, Pleurotus sp. has other dietary and medicinal advantages. Proteins, vitamins, minerals, and other vital nutrients are abundant in this species. Additionally, it has been discovered to have several medicinal qualities, including antioxidant activity, immunological stimulation, anti-inflammatory benefits, and even anti-cancerous qualities. Pleurotus sp. has a great deal of promise in the field of medicine and health (Citation181).
A further crucial concern is the role played by fungal cell walls in the removal of Cr (VI). When certain Cr (VI) binding sites are present, it has been observed that these components have a considerable impact on the removal of Cr (VI). Furthermore, these active binding sites can aid in the buildup of Cr (VI) ions inside the intracellular region (Citation181, Citation182). Additionally, the breakdown of waste products like plant leftovers and agricultural waste helps to remove Cr (VI) overall. Extracellular enzymes found in P. florida, efficiently break up these waste products. The cellulose, hemicelluloses, and lignin included in these waste products are broken down in large part by enzymes such as lignin peroxidase, cellulase, and laccase. These substances are broken down enzymatically, which allows the fungal cell to absorb them and then use its enzymatic system to break them down intracellularly. Numerous studies have been conducted on the complexity and variability of this enzyme system, indicating its non-specific character. Different Cr (VI) compounds deteriorate because of this non-specificity (Citation183, Citation184). Pleurotus sp. is a very beneficial option as a bioremediating agent for the removal of heavy metal ions from polluted soil and water due to its distinct cultivating capabilities, nutritional advantages, and medicinal uses. Furthermore, Pleurotus sp. fungal cell wall components and their enzymatic system are essential for the binding of heavy metals like Cr (VI) (Citation185, Citation186).
5. Advantages and disadvantages of biological heavy metal removal methods
Biological techniques for Cr (VI) removal are cost-effective and environmentally friendly. Here, the author is summarizing experimental evidence supporting the effectiveness of biological techniques for Cr (VI) removal compared to physicochemical methods in .
Table 6. Advantages of biological hevy metal removal methods compared to physicochemical methods.
On the other hand, the growth of microorganisms requires the use of growth-specific growth media due to the metabolic dependency that removes Cr (VI). Furthermore, it is important to take into account additional growth factors including pH, temperature, and agitation to promote the most optimum development of bacteria. As a result, bioremediation methods that depend on live microorganisms require more energy input and extra media ingredients (Citation187, Citation188). On the other hand, non-living techniques for removing Cr (VI) are metabolically independent and don't need a medium or a significant amount of energy in comparison to a live creature. Moreover, biological techniques are still in the early stages of development and commercialization. Despite these drawbacks, biological techniques remain viable, economical, and ecologically benign even at extremely low concentrations of Cr (VI) ions in water (Citation178, Citation189).
6. Major challenges and future directions
The environmental remediation of Cr (VI) by biological-mediated methods is a labour-intensive and time-consuming process. Moreover, there are a lot of challenges in the way of applying these methods in water treatment facilities and on a commercial scale. Using chelating agents and surfactants to change the adsorbent's surface is one possible way to deal with these issues. Another feasible approach to enhance the removal of Cr (VI) from water is to utilize biopolymers or composite nanomaterials to immobilize bacteria on a solid surface. It is important to investigate the possibilities of biological remediation techniques in the future for the removal of As, Ni, Hg, and Cu, among other harmful metal ions. Moreover, energy-efficient, cost-effective, and environmentally friendly systems may be constructed through thorough technical evaluation processes including life cycle assessment and exergo-environmental and exergoeconomic analysis of large-scale wastewater treatment plants. Additionally, comparative transcriptome analysis and the molecular mechanism of Cr (VI) elimination may be included in future study plans (Citation199, Citation200).
7. Conclusion
Cr (VI) is utilized in various industrial processes, such as chrome plating and tanning. The utilization of Cr (VI), which is deemed the most perilous substance, poses a significant hazard to human well-being. The occurrence of contamination arises when Cr (VI) is discharged into the ecosystem. The existence of Cr (VI) ions in soil and water has an adverse impact on the health of diverse aquatic and terrestrial species, as well as humans. Prominent health ramifications associated with Cr (VI) poisoning encompass multiple forms of cancer, impairment to the kidneys and liver, cutaneous disorders, and cognitive deterioration. Numerous conventional methodologies, namely membrane filtration, reverse osmosis, chemical reduction, and adsorption, are employed for the eradication of Cr (VI) ions from wastewater. Nonetheless, when compared to biological alternatives, these procedures incur higher costs and engender substantial quantities of ancillary pollutants. Conversely, eco-friendly techniques offer several advantages over traditional methodologies. Conversely, this article comprehensively condenses the biological approaches for the elimination of Cr (VI), encompassing both metabolically reliant and independent strategies. Furthermore, this review underscores the origins and toxicity of Cr (VI). The outcomes of this investigation possess the potential to augment the understanding of the design of biological wastewater treatment systems.
Author contributions
Conceptualization, validation, formal analysis, writing – original draft preparation: Shijie Xie. Author has read and agreed to the published version of the manuscript.
Acknowledgements
The author is thankful to Institute of International Rivers and Eco-Security, Yunnan University for providing necessary support for this research work.
Data availability statement
Data mentioned in this manuscript.
Disclosure statement
No potential conflict of interest was reported by the author(s).
References
- Handa, K.; Jindal, R. Genotoxicity induced by hexavalent chromium leading to eryptosis in Ctenopharyngodon idellus. Chemosphere 2020, 247, 125967.
- Singh, V.; Singh, J.; Mishra, V. Sorption kinetics of an eco-friendly and sustainable Cr (VI) ion scavenger in a batch reactor. J Environ Chem Eng 2021, 9, 105125.
- Huda, I.; Panezai, S. Chromite ore mining and associated factors in the muslim bagh ophiolite of Balochistan, Pakistan. Geosciences. (Basel) 2021, 11, 43–54.
- de Borja Ojembarrena, F.; Sammaraie, H.; Campano, C.; Blanco, A.; Merayo, N.; Negro, C. Hexavalent chromium removal from industrial wastewater by adsorption and reduction onto cationic cellulose nanocrystals. Nanomater. 2022, 12, 4172.
- Mitra, S.; Sarkar, A.; Sen, S. Removal of chromium from industrial effluents using nanotechnology: a review. Nanotechnol Environ Eng 2017, 2, 11.
- Saha, R.; Nandi, R.; Saha, B. Sources and toxicity of hexavalent chromium. J. Coord. Chem. 2011, 64, 1782–1806.
- Singh, V.; Mishra, V. Microbial removal of Cr (VI) by a new bacterial strain isolated from the site Contaminated with coal mine effluents. J Environ Chem Eng 2021, 9, 106279.
- Oze, C.; Bird, D.; Fendorf, S. Genesis of hexavalent chromium from natural sources in soil and groundwater. Proc Natl Acad Sci U.S.A 2007, 104, 6544–6549.
- Tumolo, M.; Ancona, V.; De Paola, D.; Losacco, D.; Campanale, C.; Massarelli, C.; Uricchio, V.F. Chromium pollution in european water, sources, health risk, and remediation strategies: an overview. Int. J. Environ. Res. Public Health 2020, 17, 5438.
- Wise, S.S.; Wise, J.P. Sr. Chromium and genomic stability. Mutat. Res. 2012, 733, 78–82.
- Singh, V.; Singh, N.; Verma, M.; Kamal, R.; Tiwari, R.; Chivate, M.S.; Rai, S.N.; Kumar, A.; Singh, A.; Singh, M.P.; Vamanu, E.; Mishra, V. Hexavalent-chromium-induced oxidative stress and the protective role of antioxidants against cellular toxicity. Antioxidants 2022a, 11, 2375. doi:10.3390/antiox11122375.
- Viti, C.; Marchi, E.; Decorosi, F.; Giovannetti, L. Molecular mechanisms of Cr (VI) resistance in bacteria and fungi. FEMS Microbiol. Rev. 2014, 38, 633–659.
- Sharma, P.; Singh, S.P.; Parakh, S.K.; Tong, Y.W. Health hazards of hexavalent chromium (Cr (VI)) and its microbial reduction. Bioengineered 2022, 13, 4923–4938.
- Park, J.E.; Shin, J.H.; Oh, W.; Choi, S.J.; Kim, J.; Kim, C.; Jeon, J. Removal of hexavalent chromium(vi) from wastewater using chitosan-coated iron oxide nanocomposite membranes. Toxics 2022, 10, 98. doi:10.3390/toxics10020098.
- Hasan, M.M.; Salman, M.S.; Hasan, M.N.; Rehan, A.I.; Awual, M.E.; Rasee, A.I.; Waliullah, R.M.; Hossain, M.S.; Kubra, K.T.; Sheikh, M.C.; Khaleque, M.A.; Marwani, H.M.; Islam, A.; Awual, M.R. Facial Conjugate Adsorbent for Sustainable Pb(II) Ion Monitoring and Removal from Contaminated Water. Colloids Surf., A 2023, 673, 131794. doi:10.1016/j.colsurfa.2023.131794.
- Awual, M.R.; Hasan, M.M.; Shahat, A.; Naushad, M.; Shiwaku, H.; Yaita, T. Investigation of Ligand Immobilized Nano-Composite Adsorbent for Efficient Cerium(III) Detection and Recovery. Chem. Eng. J. 2015, 265, 210–218. doi:10.1016/j.cej.2014.12.052.
- Hasan, M.N.; Salman, M.S.; Hasan, M.M.; Kubra, K.T.; Sheikh, M.C.; Rehan, A.I.; Rasee, A.I.; Awual, M.E.; Waliullah, R.M.; Hossain, M.S.; Islam, A.; Khandaker, S.; Alsukaibi, A.K.D.; Alshammari, H.M.; Awual, M.R. Assessing Sustainable Lutetium(III) Ions Adsorption and Recovery Using Novel Composite Hybrid Nanomaterials. J. Mol. Struct. 2023, 1276, 134795. doi:10.1016/j.molstruc.2022.134795.
- Rehan, A.I.; Rasee, A.I.; Awual, M.E.; Waliullah, R.M.; Hossain, M.S.; Kubra, K.T.; Salman, M.S.; Hasan, M.M.; Hasan, M.N.; Sheikh, M.C.; Marwani, H.M.; Khaleque, M.A.; Islam, A.; Awual, M.R. Improving Toxic Dye Removal and Remediation Using Novel Nanocomposite Fibrous Adsorbent. Colloids Surf., A 2023, 673, 131859. doi:10.1016/j.colsurfa.2023.131859.
- Hasan, M.M.; Kubra, K.T.; Hasan, M.N.; Awual, M.E.; Salman, M.S.; Sheikh, M.C.; Rehan, A.I.; Rasee, A.I.; Waliullah, R.M.; Islam, M.S.; Khandaker, S.; Islam, A.; Hossain, M.S.; Alsukaibi, A.K.D.; Alshammari, H.M.; Awual, M.R. Sustainable Ligand-Modified Based Composite Material for the Selective and Effective Cadmium(II) Capturing from Wastewater. J. Mol. Liq. 2023, 371, 121125. doi:10.1016/j.molliq.2022.121125.
- Awual, M.R. A Novel Facial Composite Adsorbent for Enhanced Copper(II) Detection and Removal from Wastewater. Chem. Eng. J. 2015, 266, 368–375. doi:10.1016/j.cej.2014.12.094.
- Awual, M.R. New Type Mesoporous Conjugate Material for Selective Optical Copper(II) Ions Monitoring & Removal from Polluted Waters. Chem. Eng. J. 2017, 307, 85–94. doi:10.1016/j.cej.2016.07.110.
- Awual, M.R. Novel Conjugated Hybrid Material for Efficient Lead(II) Capturing from Contaminated Wastewater. Materials Science and Engineering: C 2019, 101, 686–695. doi:10.1016/j.msec.2019.04.015.
- Kubra, K.T.; Hasan, M.M.; Hasan, M.N.; Salman, M.S.; Khaleque, M.A.; Sheikh, M.C.; Rehan, A.I.; Rasee, A.I.; Waliullah, R.M.; Awual, M.E.; Hossain, M.S.; Alsukaibi, A.K.D.; Alshammari, H.M.; Awual, M.R. The Heavy Lanthanide of Thulium(III) Separation and Recovery Using Specific Ligand-Based Facial Composite Adsorbent. Colloids Surf., A 2023, 667, 131415. doi:10.1016/j.colsurfa.2023.131415.
- Kubra, K.T.; Salman, M.S.; Hasan, M.N.; Islam, A.; Hasan, M.M.; Awual, M.R. Utilizing an Alternative Composite Material for Effective Copper(II) Ion Capturing from Wastewater. J. Mol. Liq. 2021, 336, 116325. doi:10.1016/j.molliq.2021.116325.
- Hasan, M.M.; Shenashen, M.A.; Hasan, M.N.; Znad, H.; Salman, M.S.; Awual, M.R. Natural Biodegradable Polymeric Bioadsorbents for Efficient Cationic Dye Encapsulation from Wastewater. J. Mol. Liq. 2021, 323, 114587. doi:10.1016/j.molliq.2020.114587.
- Kubra, K.T.; Salman, M.S.; Hasan, M.N.; Islam, A.; Teo, S.H.; Hasan, M.M.; Sheikh, M.C.; Awual, M.R. Sustainable Detection and Capturing of Cerium(III) Using Ligand Embedded Solid-State Conjugate Adsorbent. J. Mol. Liq. 2021, 338, 116667. doi:10.1016/j.molliq.2021.116667.
- Kubra, K.T.; Salman, M.S.; Znad, H.; Hasan, M.N. Efficient Encapsulation of Toxic Dye from Wastewater Using Biodegradable Polymeric Adsorbent. J. Mol. Liq. 2021, 329, 115541. doi:10.1016/j.molliq.2021.115541.
- Awual, M.R.; Hasan, M.N.; Hasan, M.M.; Salman, M.S.; Sheikh, M.C.; Kubra, K.T.; Islam, M.S.; Marwani, H.M.; Islam, A.; Khaleque, M.A.; Waliullah, R.M.; Hossain, M.S.; Rasee, A.I.; Rehan, A.I.; Awual, M.E. Green and Robust Adsorption and Recovery of Europium(III) with a Mechanism Using Hybrid Donor Conjugate Materials. Sep. Purif. Technol. 2023, 319, 124088. doi:10.1016/j.seppur.2023.124088.
- Salman, M.S.; Hasan, M.N.; Hasan, M.M.; Kubra, K.T.; Sheikh, M.C.; Rehan, A.I.; Waliullah, R.M.; Rasee, A.I.; Awual, M.E.; Hossain, M.S.; Alsukaibi, A.K.D.; Alshammari, H.M.; Awual, M.R. Improving Copper(II) Ion Detection and Adsorption from Wastewater by the Ligand-Functionalized Composite Adsorbent. J. Mol. Struct. 2023, 1282, 135259. doi:10.1016/j.molstruc.2023.135259.
- Pushkar, B.; Sevak, P.; Parab, S.; Nilkanth, N. Chromium pollution and its bioremediation mechanisms in bacteria: A review. J. Environ. Manage. 2021, 287, 112279.
- Bhattacharya, A.; Gupta, A.; Kaur, A.; Malik, D. Alleviation of hexavalent chromium by using microorganisms: insight into the strategies and complications. Water Sci. Technol. 2019, 79, 411–424.
- Murthy, M.K.; Khandayataray, P.; Padhiary, S.; Samal, D. A review on chromium health hazards and molecular mechanism of chromium bioremediation. Rev. Environ. Health 2022, 38, 461–478.
- Saha, P.; Shinde, O.; Sarkar, S. Phytoremediation of industrial mines wastewater using water hyacinth. Int. J. Phytoremediation 2017, 19, 87–96.
- Garg, S.K.; Tripathi, M.; Srinath, T. Strategies for chromium bioremediation of tannery effluent. Rev. Environ. Contam. Toxicol. 2012, 217, 75–140.
- Su, Y.Q.; Yuan, S.; Guo, Y.C.; Tan, Y.Y.; Mao, H.T.; Cao, Y.; Chen, Y.E. Highly efficient and sustainable removal of Cr (VI) in aqueous solutions by photosynthetic bacteria supplemented with phosphor salts. Chemosphere 2021, 283, 131031.
- Li, M.H.; Gao, X.; Li, C.; Yang, C.L.; Fu, C.A.; Liu, J.; Wang, R.; Chen, L.X.; Lin, J.Q.; Liu, X.M.; Lin, J.Q.; Pang, X. Isolation and identification of chromium reducing bacillus cereus species from chromium-contaminated soil for the biological detoxification of chromium. Int. J. Environ. Res. Public Health 2020, 17, 2118. doi:10.3390/ijerph17062118.
- Plestenjak, E.; Kraigher, B.; Leskovec, S.; Mandic Mulec, I.; Marković, S.; Ščančar, J.; Milačič, R. Reduction of hexavalent chromium using bacterial isolates and a microbial community enriched from tannery effluent. Sci. Rep. 2022, 12, 20197.
- Abreu, P.L.; Ferreira, L.M.R.; Alpoim, M.C.; Urbano, A.M. Impact of hexavalent chromium on mammalian cell bioenergetics: phenotypic changes, molecular basis and potential relevance to chromate-induced lung cancer. Biometals 2014, 27, 409–443.
- Siidra, O.I.; Nazarchuk, E.V.; Suknotova, A.N.; Kayukov, R.A.; Krivovichev, S.V. Cr(VI) trioxide as a starting material for the synthesis of novel zero-, one-, and two-dimensional uranyl dichromates and chromate-dichromates. Inorg. Chem. 2013, 52, 4729–4735.
- Lindsay, D.R.; Farley, K.J.; Carbonaro, R.F. Oxidation of CrIII to CrVI during chlorination of drinking water. J. Environ. Monit. 2012, 14, 1789–1797.
- Singh, V.; Singh, J.; Mishra, V. Development of a cost-effective, recyclable and viable metal ion doped adsorbent for simultaneous adsorption and reduction of toxic Cr (VI) ions. J Environ Chem Eng 2021, 9, 105124.
- Becquer, T.; Quantin, C.; Sicot, M.; Boudot, J.P. Chromium availability in ultramafic soils from New Caledonia. Sci. Total Environ. 2003, 301, 251–261.
- Fantoni, D.; Brozzo, G.; Canepa, M.; Marini, F.C.L.; Ottonello, G.; Zuccolini, M.V. Natural hexavalent chromium in groundwaters interacting with ophiolitic rocks. Environ. Geol. 2002, 42, 871–882.
- Thomas, A.N.; Eiche, E.; Göttlicher, J.; Steininger, R.; Benning, L.G.; Freeman, H.M.; Tobler, D.J.; Mangayayam, M.; Dideriksen, K.; Neumann, T. Effects of metal cation substitution on hexavalent chromium reduction by green rust. Geochem. Trans. 2020, 21, 2. doi:10.1186/s12932-020-00066-8.
- Bokare, A.D.; Choi, W. Advanced oxidation process based on the Cr(III)/Cr(VI) redox cycle. Environ. Sci. Technol. 2011, 45, 9332–9338.
- Velma, V.; Vutukuru, S.S.; Tchounwou, P.B. Ecotoxicology of hexavalent chromium in freshwater fish: a critical review. Rev. Environ. Health 2009, 24, 129–145.
- Nur-E-Alam, M.; Mia, M.A.S.; Ahmad, F.; Rahman, M.M. An overview of chromium removal techniques from tannery effluent. Appl. Water. Sci. 2020, 10, 205. doi:10.1007/s13201-020-01286-0.
- Parisi, M.; Nanni, A.; Colonna, M. Recycling of chrome-tanned leather and its utilization as polymeric materials and in polymer-based composites: A Review. Polymers (Basel) 2021, 13, 429. doi:10.3390/polym13030429.
- Yılmaz, O.; Kantarli, I.C.; Yuksel, M.; Saglam, M.; Yanik, J. Conversion of leather wastes to useful products. Resour. Conserv. Recycl. 2007, 49, 436–448.
- Usha, R.; Ramasami, T. Effect of crosslinking agents (basic chromium sulfate and formaldehyde) on the thermal and thermomechanical stability of rat tail tendon collagen fibre. Thermochim. Acta 2000, 356, 59–66.
- Pati, A.; Chaudhary, R.; Subramani, S. A review on management of chrome-tanned leather shavings: a holistic paradigm to combat the environmental issues. Environ. Sci. Pollut. Res. Int. 2014, 21, 11266–82.
- Kordas, G. Corrosion barrier coatings: progress and perspectives of the chemical route. Corros Mater Degrad 2022, 3, 376–413.
- Ma, X.L.; Fei, G.T.; Xu, S.H. Synthesis of polyaniline coating on the modified fiber ball and application for Cr(VI) removal. Nanoscale Res. Lett. 2021, 16, 58.
- Das, A.P.; Mishra, S. Hexavalent chromium (vi): environment pollutant and health hazard. J Environ Res Dev 2008, 3, 386–392.
- Qian, Y.; Li, Y.; Jungwirth, S.; Seely, N.; Fang, Y.; Shi, X. The application of anti-corrosion coating for preserving the value of equipment asset in chloride-laden environments: a review. Int. J. Electrochem. Sci. 2015, 10, 10756–10780.
- Rutala, W.A.; Weber, D.J. Disinfection, sterilization, and control of hospital waste. Mandell, Douglas, and Bennett's Principles and Practice of Infectious Diseases 2015, 2015, 3294–3309.e4.
- Morais, S.; Fonseca, H.M.A.C.; Oliveira, S.M.R.; Oliveira, H.; Gupta, V.K.; Sharma, B.; de Lourdes Pereira, M. Environmental and health hazards of chromated copper arsenate-treated wood: A Review. Int. J. Environ. Res. Public Health 2021, 18, 5518.
- Kim, H.; Kim, D.J.; Koo, J.H.; Park, J.G.; Jang, Y.C. Distribution and mobility of chromium, copper, and arsenic in soils collected near CCA-treated wood structures in Korea. Sci. Total Environ. 2007, 374, 273–281.
- Kamchanawong, S.; Veerakajohnsak, C. Arsenic, chromium, and copper leaching from CCA-treated wood and their potential impacts on landfill leachate in a tropical country. Environ. Technol. 2010, 31, 381–394.
- Shibata, T.; Solo-Gabriele, H.M.; Fleming, L.E.; Cai, Y.; Townsend, T.G. A mass balance approach for evaluating leachable arsenic and chromium from an in-service CCA-treated wood structure. Sci. Total Environ. 2007, 372, 624–635.
- Balali-Mood, M.; Naseri, K.; Tahergorabi, Z.; Khazdair, M.R.; Sadeghi, M. Toxic mechanisms of five heavy metals: mercury, lead, chromium, cadmium, and arsenic. Front. Pharmacol. 2021, 12, 643972.
- Gasparotto, J.; Martinello, K.D.B. Coal as an energy source and its impacts on human health. Energy Geosci 2021, 2, 113–120.
- Finkelman, R.B.; Wolfe, A.; Hendryx, M.S. The future environmental and health impacts of coal. Energy Geosci 2021, 2, 99–112.
- Ribeiro, J.; Flores, D. Occurrence, leaching, and mobility of major and trace elements in a coal mining waste dump: The case of Douro Coalfield, Portugal. Energy Geosci 2021, 2, 121–128.
- Bokare, A.D.; Choi, W. Advanced oxidation process based on the Cr (III)/Cr (VI) redox cycle. Environ. Sci. Technol 2011, 45, 9332–9338.
- Mishra, S.; Bharagava, R.N. Toxic and Genotoxic Effects of Hexavalent Chromium in Environment and Its Bioremediation Strategies. J. Environ. Sci. Health C Environ. Carcinog. Ecotoxicol. Rev. 2016, 34, 1–32.
- Li, X.; Abdel-Moneim, A.-M.E.; Yang, B. Signaling Pathways and Genes Associated with Hexavalent Chromium-Induced Hepatotoxicity. Biol. Trace Elem. Res. 2011, doi:10.1007/s12011-022-03291-7.
- Chakraborty, R.; Renu, K.; Eladl, M.A.; El-Sherbiny, M.; Elsherbini, D.M.A.; Mirza, A.K.; Vellingiri, B.; Iyer, M.; Dey, A.; Gopalakrishnan, A.V. Mechanism of Chromium-Induced Toxicity in Lungs, Liver, and Kidney and Their Ameliorative Agents. Biomed. Pharmacother. 2022, 151, 113119.
- Kim, H.G.; Yoon, D.H.; Lee, W.H.; Han, S.K.; Shrestha, B.; Kim, C.H.; Lim, M.H.; Chang, W.; Lim, S.; Choi, S.; Song, W.O. Phellinus linteus inhibits inflammatory mediators by suppressing redox-based NF-κB and MAPKs activation in lipopolysaccharide-induced RAW 264.7 macrophage. J. Ethnopharmacol 2007, 114, 307–315.
- Bagchi, D.; Vuchetich, P.J.; Bagchi, M.; Hassoun, E.A.; Tran, M.X.; Tang, L.; Stohs, S.J. Induction of oxidative stress by chronic administration of sodium dichromate [chromium VI] and cadmium chloride [cadmium II] to rats. Free Radical Biol. Med 1997, 22, 471–478.
- Wise, S.S.; Holmes, A.L.; Ketterer, M.E.; Hartsock, W.J.; Fomchenko, E.; Katsifis, S.; Thompson, W.D.; Wise Sr, J.P. Chromium is the proximate clastogenic species for lead chromate-induced clastogenicity in human bronchial cells. Mutat. Res. Genet. Toxicol. Environ. Mutagen 2004, 560, 79–89.
- Wakeel, A.; Xu, M.; Gan, Y. Chromium-induced reactive oxygen species accumulation by altering the enzymatic antioxidant system and associated cytotoxic, genotoxic, ultrastructural, and photosynthetic changes in plants. Int. J. Mol. Sci 2020, 21, 728.
- Cohen, M.D.; Kargacin, B.; Klein, C.B.; Costa, M. Mechanisms of chromium carcinogenicity and toxicity. Crit. Rev. Toxicol 1993, 23, 255–281.
- Balali-Mood, M.; Naseri, K.; Tahergorabi, Z.; Khazdair, M.R.; Sadeghi, M. Toxic Mechanisms of Five Heavy Metals: Mercury, Lead, Chromium, Cadmium, and Arsenic. Front. Pharmacol 2021, 12, 227–245.
- Mohamed, A.A.; El-Houseiny, W.; El-Murr, A.E.; Ebraheim, L.L.M.; Ahmed, A.I.; El-Hakim, Y.M.A. Effect of Hexavalent Chromium Exposure on the Liver and Kidney Tissues Related to the Expression of CYP450 and GST Genes of Oreochromis Niloticus Fish: Role of Curcumin Supplemented Diet. Ecotoxicol. Environ. Saf 2020, 188, 109890.
- Li, S.; Baiyun, R.; Lv, Z.; Li, J.; Han, D.; Zhao, W.; Yu, L.; Deng, N.; Liu, Z.; Zhang, Z. Exploring the Kidney Hazard of Exposure to Mercuric Chloride in Mice:Disorder of Mitochondrial Dynamics Induces Oxidative Stress and Results in Apoptosis. Chemosphere 2019, 234, 822–29.
- Li, H.; Shi, J.; Gao, H.; Yang, X.; Fu, Y.; Peng, Y.; Xia, Y.; Zhou, D. Hexavalent Chromium Causes Apoptosis and Autophagy by Inducing Mitochondrial Dysfunction and Oxidative Stress in Broiler Cardiomyocytes. Biol. Trace Elem. Res 2022, 200, 2866–75.
- Shaw, P.; Mondal, P.; Dey Bhowmik, A.; Bandyopadhyay, A.; Sudarshan, M.; Chakraborty, A.; Chattopadhyay, A. Environmentally Relevant Hexavalent Chromium Disrupts Elemental Homeostasis and Induces Apoptosis in Zebrafish Liver. Bull. Environ. Contam. Toxicol. 2022, 108, 716–24.
- Patlolla, A.K.; Barnes, C.; Yedjou, C.; Velma, V.R.; Tchounwou, P.B. Oxidative Stress, DNA Damage, and Antioxidant Enzyme Activity Induced by Hexavalent Chromium in Sprague-Dawley Rats. Environ. Toxicol. 2009, 24, 66–73.
- Das, J.; Sarkar, A.; Sil, P.C. Hexavalent Chromium Induces Apoptosis in Human Liver (HepG2) Cells via Redox Imbalance. Toxicol. Rep 2015, 2, 600–608.
- Lei, T.; He, Q.Y.; Cai, Z.; Zhou, Y.; Wang, Y.L.; Si, L.S.; Cai, Z.; Chiu, J.F. Proteomic Analysis of Chromium Cytotoxicity in Cultured Rat Lung Epithelial Cells. Proteomics 2008, 8, 2420–29.
- Velma, V.; Tchounwou, P.B. Chromium-Induced Biochemical, Genotoxic and Histopathologic Effects in Liver and Kidney of Goldfish, Carassius Auratus. Mutat. Res. 2010, 698, 43–51.
- Wang, X.; Son, Y.O.; Chang, Q.; Sun, L.; Hitron, J.A.; Budhraja, A.; Zhang, Z.; Ke, Z.; Chen, F.; Luo, J.; Shi, X. NADPH Oxidase Activation Is Required in Reactive Oxygen Species Generation and Cell Transformation Induced by Hexavalent Chromium. Toxicol. Sci. 2011, 123, 399–410.
- Bagchi, D.; Stohs, S.J.; Downs, B.W.; Bagchi, M.; Preuss, H.G. Cytotoxicity and Oxidative Mechanisms of Different Forms of Chromium. Toxicol 2002, 180, 5–22.
- Ahmad, I.; Maria, V.L.; Oliveira, M.; Pacheco, M.; Santos, M.A. Oxidative stress and genotoxic effects in gill and kidney of Anguilla anguilla L. exposed to chromium with or without pre-exposure to beta-naphthoflavone. Mutat. Res. 2006, 608, 16–28. doi:10.1016/j.mrgentox.2006.04.020.
- Zheng, X.; Li, S.; Li, J.; Lv, Y.; Wang, X.; Wu, P.; Yang, Q.; Tang, Y.; Liu, Y.; Zhang, Z. Hexavalent Chromium Induces Renal Apoptosis and Autophagy via Disordering the Balance of Mitochondrial Dynamics in Rats. Ecotoxicol. Environ. Saf 2020, 204, 111061.
- Xu, J.; Zhao, M.; Pei, L.; Zhang, R.; Liu, X.; Wei, L.; Yang, M.; Xu, Q. Oxidative stress and DNA damage in a long-term hexavalent chromium-exposed population in North China: a cross-sectional study. BMJ Open 2018, 8, e021470.
- Pavesi, T.; Moreira, J.C. Mechanisms and Individuality in Chromium Toxicity in Humans. J. Appl. Toxicol 2020, 40, 1183–97.
- Bagchi, D.; Stohs, S.J.; Downs, B.W.; Bagchi, M.; Preuss, H.G. Cytotoxicity and oxidative mechanisms of different forms of chromium. Toxicology 2002, 180, 5–22.
- Bagchi, D.; Bagchi, M.; Stohs, S.J. Chromium (VI)-induced oxidative stress, apoptotic cell death and modulation of p53 tumor suppressor gene. Mol. Cell. Biochem 2001, 222, 149–58.
- Holmes, A.L.; Wise, S.S.; Wise, J.P. Sr. Carcinogenicity of hexavalent chromium. Indian J. Med. Res 2008, 128, 353–372.
- Son, Y.O.; Hitron, J.A.; Wang, X.; Chang, Q.; Pan, J.; Zhang, Z.; Liu, J.; Wang, S.; Lee, J.C.; Shi, X. Cr(VI) induces mitochondrial-mediated and caspase-dependent apoptosis through reactive oxygen species-mediated p53 activation in JB6 Cl41 cells. Toxicol. Appl. Pharmacol 2010, 245, 226–35.
- Monga, A.; Fulke, A.B.; Dasgupta, D. Recent Developments in Essentiality of Trivalent Chromium and Toxicity of Hexavalent Chromium: Implications on Human Health and Remediation Strategies. J. Hazard. Mater. Adv 2022, 7, 100113.
- Khulbe, K.C.; Matsuura, T. Removal of Heavy Metals and Pollutants by Membrane Adsorption Techniques. Appl. Water. Sci. 2018, 8 (1). doi:10.1007/s13201-018-0661-6.
- Sankarammal, M.; Thatheyus, A.; Ramya, D. Bioremoval Of Cadmium Using Pseudomonas Fluorescens. Open Journal of Water Pollution and Treatment 2014,, 2014 (2), 92–100. doi:10.15764/wpt.2014.02010.
- Wołowiec, M.; Komorowska-Kaufman, M.; Pruss, A.; Rzepa, G.; Bajda, T. Removal of Heavy Metals and Metalloids from Water Using Drinking Water Treatment Residuals as Adsorbents: A Review. Minerals 2019, 9 (8), 487. doi:10.3390/min9080487.
- Kumar, S.; Shahnaz, T.; Selvaraju, N.; Rajaraman, P.V. Kinetic and Thermodynamic Studies on Biosorption of Cr(VI) on Raw and Chemically Modified Datura Stramonium Fruit. Environ. Monit. Assess. 2020, 192 (4). doi:10.1007/s10661-020-8181-x.
- Abdelbasir, S.M.; McCourt, K.M.; Lee, C.M.; Vanegas, D.C. Waste-Derived Nanoparticles: Synthesis Approaches, Environmental Applications, and Sustainability Considerations. Front. Chem. 2020, 8, doi:10.3389/fchem.2020.00782.
- Medfu Tarekegn, M.; Zewdu Salilih, F.; Ishetu, A.I. Microbes Used as a Tool for Bioremediation of Heavy Metal from the Environment. Cogent Food & Agriculture 2020, 6 (1), 1783174. doi:10.1080/23311932.2020.1783174.
- Volesky, B. Biosorption and Me. Water Res. 2007, 41 (18), 4017–4029. doi:10.1016/j.watres.2007.05.062.
- Kannan, A.; Mishra, R.; Sinha, V.; Upreti, R. Reduction of Chromium-vi by Chromium Resistant Lactobacilli: A Prospective Bacterium for Bioremediation. Toxicol. Int. 2012, 19 (1), 25. doi:10.4103/0971-6580.94512.
- Mishra, A.; Dubey, A.; Shinghal, S. Biosorption of Chromium(VI) from Aqueous Solutions Using Waste Plant Biomass. Int. J. Environ. Sci. Technol. 2014, 12 (4), 1415–1426. doi:10.1007/s13762-014-0516-0.
- Malik, A. Metal Bioremediation through Growing Cells. Environ. Int. 2004, 30 (2), 261–278. doi:10.1016/j.envint.2003.08.001.
- Peng, S.-H.; Wang, R.; Yang, L.-Z.; He, L.; He, X.; Liu, X. Biosorption of Copper, Zinc, Cadmium and Chromium Ions from Aqueous Solution by Natural Foxtail Millet Shell. Ecotoxicol. Environ. Saf. 2018, 165, 61–69. doi:10.1016/j.ecoenv.2018.08.084.
- Wang, J.; Chen, C. Biosorption of Heavy Metals by Saccharomyces Cerevisiae: A Review. Biotechnol. Adv. 2006, 24 (5), 427–451. doi:10.1016/j.biotechadv.2006.03.001.
- Verma, N.; Sharma, R. Bioremediation of Toxic Heavy Metals: A Patent Review. Recent Pat. Biotechnol. 2017, 11 (3), doi:10.2174/1872208311666170111111631.
- Ojuederie, O.; Babalola, O. Microbial and Plant-Assisted Bioremediation of Heavy Metal Polluted Environments: A Review. Int. J. Environ. Res. Public Health 2017, 14 (12), 1504. doi:10.3390/ijerph14121504.
- Chojnacka, K. Biosorption and Bioaccumulation – the Prospects for Practical Applications. Environ. Int. 2010, 36 (3), 299–307. doi:10.1016/j.envint.2009.12.001.
- Tsezos, M.; Remoudaki, E.; Angelatou, V. Biosorption Sites of Selected Metals Using Electron Microscopy. Comparative Biochemistry and Physiology Part A: Physiology 1997, 118 (3), 481–487. doi:10.1016/s0300-9629(97)00009-1.
- Duwiejuah, A.B.; Abubakari, A.H.; Quainoo, A.K.; Amadu, Y. Review of Biochar Properties and Remediation of Metal Pollution of Water and Soil. Journal of Health and Pollution 2020, 10 (27), doi:10.5696/2156-9614-10.27.200902.
- Dias, M.A.; Rosa, C.A.; Linardi, V.R.; Conte, R.A.; De Castro, H.F. Application of Factorial Design to Study of Heavy Metals Biosorption by Waste Biomass from Beverage Distillery. Appl. Biochem. Biotechnol. 2001, 91–93 (1–9), 413–422. doi:10.1385/abab:91-93:1-9:413.
- Jobby, R.; Jha, P.; Yadav, A.K.; Desai, N. Biosorption and Biotransformation of Hexavalent Chromium [Cr(VI)]: A Comprehensive Review. Chemosphere 2018, 207, 255–266. doi:10.1016/j.chemosphere.2018.05.050.
- Netzahuatl-Muñoz, A.R.; Cristiani-Urbina, M.d.C.; Cristiani-Urbina, E. Chromium Biosorption from Cr(VI) Aqueous Solutions by Cupressus Lusitanica Bark: Kinetics, Equilibrium and Thermodynamic Studies. PLoS One 2015, 10 (9), e0137086. doi:10.1371/journal.pone.0137086.
- Park, D.; Lim, S.-R.; Yun, Y.-S.; Park, J.M. Reliable Evidences That the Removal Mechanism of Hexavalent Chromium by Natural Biomaterials Is Adsorption-Coupled Reduction. Chemosphere 2007, 70 (2), 298–305. doi:10.1016/j.chemosphere.2007.06.007.
- Deng, L.; Zhang, Y.; Qin, J.; Wang, X.; Zhu, X. Biosorption of Cr(VI) from Aqueous Solutions by Nonliving Green Algae Cladophora Albida. Miner. Eng. 2009, 22 (4), 372–377. doi:10.1016/j.mineng.2008.10.006.
- Moghal, A.A.B.; Lateef, M.A.; Abu Sayeed Mohammed, S.; Ahmad, M.; Usman, A.R.A.; Almajed, A. Heavy Metal Immobilization Studies and Enhancement in Geotechnical Properties of Cohesive Soils by EICP Technique. Applied Sciences 2020, 10 (21), 7568. doi:10.3390/app10217568.
- Khanpour-Alikelayeh, E.; Partovinia, A.; Talebi, A.; Kermanian, H. Enhanced Biodegradation of Light Crude Oil by Immobilized Bacillus Licheniformis in Fabricated Alginate Beads through Electrospray Technique. Environ. Monit. Assess. 2021, 193 (6), doi:10.1007/s10661-021-09104-z.
- Pal, D.; Maiti, S.K. An Approach to Counter Sediment Toxicity by Immobilization of Heavy Metals Using Waste Fish Scale Derived Biosorbent. Ecotoxicol. Environ. Saf. 2020, 187, 109833. doi:10.1016/j.ecoenv.2019.109833.
- Hassanshahian, M.; Emtiazi, G.; Caruso, G.; Cappello, S. Bioremediation (Bioaugmentation/Biostimulation) Trials of Oil Polluted Seawater: A Mesocosm Simulation Study. Mar. Environ. Res. 2014, 95, 28–38. doi:10.1016/j.marenvres.2013.12.010.
- Mancilla, H.B.; Cerrón, M.R.; Aroni, P.G.; Paucar, J.E.P.; Tovar, C.T.; Jindal, M.K.; Gowrisankar, G. Effective Removal of Cr (VI) Ions Using Low-Cost Biomass Leaves (Sambucus Nigra L.) in Aqueous Solution. Environmental Science and Pollution Research 2022, 30 (49), 106982–106995. doi:10.1007/s11356-022-24064-8.
- Beig, S.-U.-R.; Shah, S.A. Biosorption of Cr (VI) by Acid-Modified Based-Waste Fungal Biomass from Calocybe Indica Fruiting Bodies Production. Int. J. Phytoremediation 2022, 25 (10), 1269–1288. doi:10.1080/15226514.2022.2147145.
- Ayele, A.L.; Tizazu, B.Z.; Wassie, A.B. Chemical Modification of Teff Straw Biomass for Adsorptive Removal of Cr (VI) from Aqueous Solution: Characterization, Optimization, Kinetics, and Thermodynamic Aspects. Adsorpt. Sci. Technol. 2022, 2022, 1–25. doi:10.1155/2022/5820207.
- Akl, M.A.; El-Zeny, A.S.; Hashem, M.A.; El-Gharkawy, E.-S.R.H.; Mostafa, A.G. Flax Fiber Based Semicarbazide Biosorbent for Removal of Cr(VI) and Alizarin Red S Dye from Wastewater. Sci. Rep. 2023, 13 (1). doi:10.1038/s41598-023-34523-y.
- Zhang, C.; Ren, H.-X.; Zhong, C.-Q.; Wu, D. Biosorption of Cr(VI) by Immobilized Waste Biomass from Polyglutamic Acid Production. Sci. Rep. 2020, 10 (1). doi:10.1038/s41598-020-60729-5.
- Vaddi, D.R.; Gurugubelli, T.R.; Koutavarapu, R.; Lee, D.-Y.; Shim, J. Bio-Stimulated Adsorption of Cr(VI) from Aqueous Solution by Groundnut Shell Activated Carbon@Al Embedded Material. Catalysts 2022, 12 (3), 290. doi:10.3390/catal12030290.
- Shakya, A.; Vithanage, M.; Agarwal, T. Influence of Pyrolysis Temperature on Biochar Properties and Cr(VI) Adsorption from Water with Groundnut Shell Biochars: Mechanistic Approach. Environ. Res. 2022, 215, 114243. doi:10.1016/j.envres.2022.114243.
- Yang, P.; Xu, Y.; Tuo, J.; Li, A.; Liu, L.; Shi, H. Preparation of Modified Pomelo Peel’s Pulp Adsorbent and Its Adsorption to Uranyl Ions. R. Soc. Open. Sci. 2019, 6 (3), 181986. doi:10.1098/rsos.181986.
- Ahmed, S.; Ahmad, M.; Swami, B.L.; Ikram, S. A Review on Plants Extract Mediated Synthesis of Silver Nanoparticles for Antimicrobial Applications: A Green Expertise. J. Adv. Res. 2016, 7 (1), 17–28. doi:10.1016/j.jare.2015.02.007.
- Khan, I.; Saeed, K.; Khan, I. Nanoparticles: Properties, Applications and Toxicities. Arabian Journal of Chemistry 2019, 12 (7), 908–931. doi:10.1016/j.arabjc.2017.05.011.
- Vidu, R.; Matei, E.; Predescu, A.M.; Alhalaili, B.; Pantilimon, C.; Tarcea, C.; Predescu, C. Removal of Heavy Metals from Wastewaters: A Challenge from Current Treatment Methods to Nanotechnology Applications. Toxics 2020, 8 (4), 101. doi:10.3390/toxics8040101.
- Mittal, R.; Sharma, A.; Bhardwaj, A.K.; Bhateria, R.; Bansal, S.; Kashyap, R.; Bhukal, S. Removal of Chromium (VI) Using Spirulina Assisted Synthesized Mesoporous Iron Oxide Nanoparticles. Inorg. Chem. Commun. 2023, 154, 110881. doi:10.1016/j.inoche.2023.110881.
- Kumar, H.; Sinha, S.K.; Goud, V.V.; Das, S. Removal of Cr(VI) by Magnetic Iron Oxide Nanoparticles Synthesized from Extracellular Polymeric Substances of Chromium Resistant Acid-Tolerant Bacterium Lysinibacillus Sphaericus RTA-01. Journal of Environmental Health Science and Engineering 2019, 17 (2), 1001–1016. doi:10.1007/s40201-019-00415-5.
- Du, L.; Gao, P.; Liu, Y.; Minami, T.; Yu, C. Removal of Cr(VI) from Aqueous Solution by Polypyrrole/Hollow Mesoporous Silica Particles. Nanomaterials 2020, 10, 686. doi:10.3390/nano10040686.
- Rong, K.; Wang, J.; Li, X.; Zhang, Z.; Yang, Q.; Shan, C.; Wu, T.; Liu, J. Removal of Cr(VI) by iron nanoparticles synthesized by a novel green method using Yali pear peels extracts: optimization, reactivity, and mechanism. Biomass Conversion and Biorefinery 2024, 14, 4355–4368.
- Diep, P.; Mahadevan, R.; Yakunin, A.F. Heavy Metal Removal by Bioaccumulation Using Genetically Engineered Microorganisms. Front. Bioeng. Biotechnol. 2018, 6. doi:10.3389/fbioe.2018.00157.
- Timková, I.; Sedláková-Kaduková, J.; Pristaš, P. Biosorption and Bioaccumulation Abilities of Actinomycetes/Streptomycetes Isolated from Metal Contaminated Sites. Separations 2018, 5 (4), 54. doi:10.3390/separations5040054.
- Arishi, A.; Mashhour, I. Microbial Mechanisms for Remediation of Hexavalent Chromium and Their Large-Scale Applications; Current Research and Future Directions. Journal of Pure and Applied Microbiology 2021, 15 (1), 53–67. doi:10.22207/jpam.15.1.32.
- Ali, H.; Khan, E.; Ilahi, I. Environmental Chemistry and Ecotoxicology of Hazardous Heavy Metals: Environmental Persistence, Toxicity, and Bioaccumulation. J. Chem. 2019, 2019, 1–14. doi:10.1155/2019/6730305.
- Yilmazer, P.; Saracoglu, N. Bioaccumulation and Biosorption of Copper(II) and Chromium(III) from Aqueous Solutions by Pichia Stipitis Yeast. J. Chem. Technol. Biotechnol. 2008, 84 (4), 604–610. doi:10.1002/jctb.2088.
- Jacob, J.M.; Karthik, C.; Saratale, R.G.; Kumar, S.S.; Prabakar, D.; Kadirvelu, K.; Pugazhendhi, A. Biological Approaches to Tackle Heavy Metal Pollution: A Survey of Literature. J. Environ. Manag. 2018, 217, 56–70. doi:10.1016/j.jenvman.2018.03.077.
- El-Naggar, N.E.-A.; El-khateeb, A.Y.; Ghoniem, A.A.; El-Hersh, M.S.; Saber, W.I.A. Innovative Low-Cost Biosorption Process of Cr6+by Pseudomonas Alcaliphila NEWG-2. Sci. Rep. 2020, 10 (1). doi:10.1038/s41598-020-70473-5.
- Tekerlekopoulou, A.G.; Tsiflikiotou, M.; Akritidou, L.; Viennas, A.; Tsiamis, G.; Pavlou, S.; Bourtzis, K.; Vayenas, D.V. Modelling of Biological Cr(VI) Removal in Draw-Fill Reactors Using Microorganisms in Suspended and Attached Growth Systems. Water Res. 2013, 47 (2), 623–636. doi:10.1016/j.watres.2012.10.034.
- Humphries, A.C.; Nott, K.P.; Hall, L.D.; Macaskie, L.E. Reduction of Cr(VI) by Immobilized Cells of Desulfovibrio Vulgaris NCIMB 8303 and Microbacterium Sp. NCIMB 13776. Biotechnol. Bioeng. 2005, 90 (5), 589–596. doi:10.1002/bit.20450.
- Li, M.; Gao, X.; Li, C.; Yang, C.; Fu, C.; Liu, J.; Wang, R.; Chen, L.; Lin, J.; Liu, X.; Lin, J.; Pang, X. Isolation and Identification of Chromium Reducing Bacillus Cereus Species from Chromium-Contaminated Soil for the Biological Detoxification of Chromium. Int. J. Environ. Res. Public Health 2020, 17 (6), 2118. doi:10.3390/ijerph17062118.
- Ibrahim, A.S.S.; El-Tayeb, M.A.; Elbadawi, Y.B.; Al-Salamah, A.A.; Antranikian, G. Hexavalent Chromate Reduction by Alkaliphilic Amphibacillus Sp. KSUCr3 Is Mediated by Copper-Dependent Membrane-Associated Cr(VI) Reductase. Extremophiles 2012, 16 (4), 659–668. doi:10.1007/s00792-012-0464-x.
- Liu, Z.; Wu, Y.; Lei, C.; Liu, P.; Gao, M. Chromate Reduction by a Chromate-Resistant Bacterium, Microbacterium Sp. World J. Microbiol. Biotechnol. 2011, 28 (4), 1585–1592. doi:10.1007/s11274-011-0962-5.
- Henson, M.W.; Santo Domingo, J.W.; Kourtev, P.S.; Jensen, R.V.; Dunn, J.A.; Learman, D.R. Metabolic and Genomic Analysis Elucidates Strain-Level Variation inMicrobacterium Spp.Isolated from Chromate Contaminated Sediment. PeerJ. 2015, 3, e1395. doi:10.7717/peerj.1395.
- P.; N, P.; B.; L, M. Chromate Reduction and 16S rRNA Identification of Bacteria Isolated from a Cr(VI)-Contaminated Site. Appl. Microbiol. Biotechnol. 2001, 57 (1–2), 257–261. doi:10.1007/s002530100758.
- Ahemad, M. Enhancing Phytoremediation of Chromium-Stressed Soils through Plant-Growth-Promoting Bacteria. Journal of Genetic Engineering and Biotechnology 2015, 13 (1), 51–58. doi:10.1016/j.jgeb.2015.02.001.
- Kubrak, O.I.; Lushchak, O.V.; Lushchak, J.V.; Torous, I.M.; Storey, J.M.; Storey, K.B.; Lushchak, V.I. Chromium Effects on Free Radical Processes in Goldfish Tissues: Comparison of Cr(III) and Cr(VI) Exposures on Oxidative Stress Markers, Glutathione Status and Antioxidant Enzymes. Comp. Biochem. Physiol. C: Toxicol. Pharmacol. 2010, 152 (3), 360–370. doi:10.1016/j.cbpc.2010.06.003.
- Kumar, M.S.; Praveenkumar, R.; Ilavarasi, A.; Rajeshwari, K.; Thajuddin, N. Biochemical Changes of Fresh Water Cyanobacteria Dolichospermum Flos-Aquae NTMS07 to Chromium-Induced Stress with Special Reference to Antioxidant Enzymes and Cellular Fatty Acids. Bull. Environ. Contam. Toxicol. 2013, 90 (6), 730–735. doi:10.1007/s00128-013-0984-9.
- Singh, V.; Mishra, V. Microbial Removal of Cr (VI) by a New Bacterial Strain Isolated from the Site Contaminated with Coal Mine Effluents. Journal of Environmental Chemical Engineering 2021, 9 (5), 106279. doi:10.1016/j.jece.2021.106279.
- Ilias, M.; Rafiqullah, I.M.; Debnath, B.C.; Mannan, K.S.B.; Mozammel Hoq, M. Isolation and Characterization of Chromium(VI)-Reducing Bacteria from Tannery Effluents. Indian J. Microbiol. 2011, 51 (1), 76–81. doi:10.1007/s12088-011-0095-4.
- Kabir, M.M.; Fakhruddin, A.N.M.; Chowdhury, M.A.Z.; Pramanik, M.K.; Fardous, Z. Isolation and Characterization of Chromium(VI)-Reducing Bacteria from Tannery Effluents and Solid Wastes. World J. Microbiol. Biotechnol. 2018, 34 (9). doi:10.1007/s11274-018-2510-z.
- Zahoor, A.; Rehman, A. Isolation of Cr(VI) Reducing Bacteria from Industrial Effluents and Their Potential Use in Bioremediation of Chromium Containing Wastewater. J. Environ. Sci. 2009, 21 (6), 814–820. doi:10.1016/s1001-0742(08)62346-3.
- Wang, X.; Zhang, Y.; Sun, X.; Jia, X.; Liu, Y.; Xiao, X.; Gao, H.; Li, L. Efficient Removal of Hexavalent Chromium from Water by Bacillus Sp. Y2-7 with Production of Extracellular Polymeric Substances. Environ. Technol. 2023, 1–11. doi:10.1080/09593330.2023.2185817.
- Baldiris, R.; Acosta-Tapia, N.; Montes, A.; Hernández, J.; Vivas-Reyes, R. Reduction of Hexavalent Chromium and Detection of Chromate Reductase (ChrR) in Stenotrophomonas Maltophilia. Molecules 2018, 23 (2), 406. doi:10.3390/molecules23020406.
- Wang, S.; Zhang, L.; Xia, Z.; Roy, A.; Chang, D.W.; Baek, J.; Dai, L. BCN Graphene as Efficient Metal-Free Electrocatalyst for the Oxygen Reduction Reaction. Angew. Chem., Int. Ed. 2012, 51 (17), 4209–4212. doi:10.1002/anie.201109257.
- Donati, E.; Oliver, C.; Curutchet, G. Reduction of Chromium (VI) by the Indirect Action of Thiobacillus Thioparus. Braz. J. Chem. Eng. 2003, 20 (1), 69–73. doi:10.1590/s0104-66322003000100013.
- Somasundaram, V.; Philip, L.; Bhallamudi, S.M. Experimental and Mathematical Modeling Studies on Cr(VI) Reduction by CRB, SRB and IRB, Individually and in Combination. J. Hazard. Mater. 2009, 172 (2–3), 606–617. doi:10.1016/j.jhazmat.2009.07.043.
- Puzon, G.J.; Petersen, J.N.; Roberts, A.G.; Kramer, D.M.; Xun, L. A Bacterial Flavin Reductase System Reduces Chromate to a Soluble Chromium(III)–NAD+ Complex. Biochem. Biophys. Res. Commun. 2002, 294 (1), 76–81. doi:10.1016/s0006-291x(02)00438-2.
- Alam, M.Z.; Malik, A. Chromate Resistance, Transport and Bioreduction by Exiguobacterium Sp. ZM-2 Isolated from Agricultural Soil Irrigated with Tannery Effluent. J. Basic Microbiol. 2008, 48 (5), 416–420. doi:10.1002/jobm.200800046.
- Xu, W.H.; Liu, Y.G.; Zeng, G.M.; Li, X.; Zhang, W. Promoting Influence of Organic Carbon Source on Chromate Reduction by Bacillus Sp. Adv. Mat. Res. 2012, 610–613, 1789–1794. doi:10.4028/www.scientific.net/amr.610-613.1789.
- Ngwenya, N.; Chirwa, E.M.N. Biological Removal of Cationic Fission Products from Nuclear Wastewater. Water Sci. Technol. 2011, 63 (1), 124–128. doi:10.2166/wst.2011.021.
- Ahemad, M. Bacterial Mechanisms for Cr(VI) Resistance and Reduction: An Overview and Recent Advances. Folia Microbiol. 2014, 59 (4), 321–332. doi:10.1007/s12223-014-0304-8.
- Loryuenyong, V.; Jarunsak, N.; Chuangchai, T.; Buasri, A. The Photocatalytic Reduction of Hexavalent Chromium by Controllable Mesoporous Anatase TiO2Nanoparticles. Advances in Materials Science and Engineering 2014, 2014, 1–8. doi:10.1155/2014/348427.
- Cheung, K.H.; Gu, J.-D. Chromate Reduction by Bacillus Megaterium TKW3 Isolated from Marine Sediments. World J. Microbiol. Biotechnol. 2005, 21 (3), 213–219. doi:10.1007/s11274-004-3619-9.
- Rahman, Z.; Thomas, L. Chemical-Assisted Microbially Mediated Chromium (Cr) (VI) Reduction Under the Influence of Various Electron Donors, Redox Mediators, and Other Additives: An Outlook on Enhanced Cr(VI) Removal. Front. Microbiol. 2021, 11, doi:10.3389/fmicb.2020.619766.
- Ibrahim, A.S.S.; El-Tayeb, M.A.; Elbadawi, Y.B.; Al-Salamah, A.A.; Antranikian, G. Hexavalent Chromate Reduction by Alkaliphilic Amphibacillus Sp. KSUCr3 Is Mediated by Copper-Dependent Membrane-Associated Cr(VI) Reductase. Extremophiles 2012, 16 (4), 659–668. doi:10.1007/s00792-012-0464-x.
- Ackerley, D.F.; Gonzalez, C.F.; Park, C.H.; Blake, R.; Keyhan, M.; Matin, A. Chromate-Reducing Properties of Soluble Flavoproteins from Pseudomonas Putida and Escherichia Coli. Appl. Environ. Microbiol. 2004, 70 (2), 873–882. doi:10.1128/aem.70.2.873-882.2004.
- Archana; Jaitly, A.K. Analysis of Trace Metals in Underground Drinking Water of Bareilly. Invertis Journal of Renewable Energy 2016, 6 (2), 106. doi:10.5958/2454-7611.2016.00014.x.
- Agnello, A.C.; Bagard, M.; van Hullebusch, E.D.; Esposito, G.; Huguenot, D. Comparative Bioremediation of Heavy Metals and Petroleum Hydrocarbons Co-Contaminated Soil by Natural Attenuation, Phytoremediation, Bioaugmentation and Bioaugmentation-Assisted Phytoremediation. Sci. Total Environ. 2016, 563–564, 693–703. doi:10.1016/j.scitotenv.2015.10.061.
- Ogbo, E.M.; Okhuoya, J.A. Bio-Absorption of Some Heavy Metals by Pleurotus Tuber-Regium Fr. Singer (An Edible Mushroom) from Crude Oil Polluted Soils Amended with Fertilizers and Cellulosic Wastes. Int. J. Soil Sci. 2010, 6 (1), 34–48. doi:10.3923/ijss.2011.34.48.
- Hlihor, R.M.; Roşca, M.; Drăgoi, E.N.; Simion, I.M.; Favier, L.; Gavrilescu, M. New Insights into the Application of Fungal Biomass for Chromium(VI) Bioremoval from Aqueous Solutions Using Design of Experiments and Differential Evolution Based Neural Network Approaches. Chem. Eng. Res. Des. 2023, 190, 233–254. doi:10.1016/j.cherd.2022.12.024.
- Cárdenas-González, J.F.; Acosta-Rodríguez, I. Hexavalent Chromium Removal by aPaecilomycessp. Fungal Strain Isolated from Environment. Bioinorg. Chem. Appl. 2010, 2010, 1–6. doi:10.1155/2010/676243.
- Sharma, S.; Adholeya, A. Detoxification and Accumulation of Chromium from Tannery Effluent and Spent Chrome Effluent by Paecilomyces Lilacinus Fungi. Int. Biodeterior. Biodegrad. 2011, 65 (2), 309–317. doi:10.1016/j.ibiod.2010.12.003.
- Zapana-Huarache, S.V.; Romero-Sánchez, C.K.; Gonza, A.P.D.; Torres-Huaco, F.D.; Rivera, A.M.L. Chromium (VI) Bioremediation Potential of Filamentous Fungi Isolated from Peruvian Tannery Industry Effluents. Braz. J. Microbiol. 2019, 51 (1), 271–278. doi:10.1007/s42770-019-00209-9.
- Rivera-Martínez, E.; Cárdenas-González, J.F.; Martínez-Juárez, V.M.; Acosta-Rodríguez, I. Remoción de Cromo (VI) Por Una Cepa de Aspergillus Niger Resistente a Cromato. Información tecnológica 2015, 26 (4), 13–20. doi:10.4067/s0718-07642015000400003.
- Samuel, M.S.; Abigail, M.; Ramalingam, C. Biosorption of Cr(VI) by Ceratocystis Paradoxa MSR2 Using Isotherm Modelling, Kinetic Study and Optimization of Batch Parameters Using Response Surface Methodology. PLoS One 2015, 10 (3), e0118999. doi:10.1371/journal.pone.0118999.
- Zheng, S.; Li, C.; Ng, T.B.; Wang, H.X. A Lectin with Mitogenic Activity from the Edible Wild Mushroom Boletus Edulis. Process Biochem. 2007, 42 (12), 1620–1624. doi:10.1016/j.procbio.2007.09.004.
- Vaseem, H.; Singh, V.K.; Singh, M.P. Heavy Metal Pollution Due to Coal Washery Effluent and Its Decontamination Using a Macrofungus, Pleurotus Ostreatus. Ecotoxicol. Environ. Saf. 2017, 145, 42–49. doi:10.1016/j.ecoenv.2017.07.001.
- Gadd, G.M. Geomycology: Biogeochemical Transformations of Rocks, Minerals, Metals and Radionuclides by Fungi, Bioweathering and Bioremediation. Mycol. Res. 2007, 111 (1), 3–49. doi:10.1016/j.mycres.2006.12.001.
- Kumla, J.; Suwannarach, N.; Sujarit, K.; Penkhrue, W.; Kakumyan, P.; Jatuwong, K.; Vadthanarat, S.; Lumyong, S. Cultivation of Mushrooms and Their Lignocellulolytic Enzyme Production Through the Utilization of Agro-Industrial Waste. Molecules 2020, 25 (12), 2811. doi:10.3390/molecules25122811.
- Kumar, A.; Chandra, R. Ligninolytic Enzymes and Its Mechanisms for Degradation of Lignocellulosic Waste in Environment. Heliyon 2020, 6 (2), e03170. doi:10.1016/j.heliyon.2020.e03170.
- Hestbjerg, H.; Willumsen, P.A.; Christensen, M.; Andersen, O.; Jacobsen, C.S. Bioaugmentation of Tar-contaminated Soils under Field Conditions Using Pleurotus Ostreatus Refuse from Commercial Mushroom Production. Environ. Toxicol. Chem. 2003, 22 (4), 692–698. doi:10.1002/etc.5620220402.
- Kapahi, M.; Sachdeva, S. Mycoremediation Potential of Pleurotus Species for Heavy Metals: A Review. Bioresources and Bioprocessing 2017, 4 (1). doi:10.1186/s40643-017-0162-8.
- Akhtar, F.Z.; Archana, K.M.; Krishnaswamy, V.G.; Rajagopal, R. . Remediation of Heavy Metals (Cr, Zn) Using Physical, Chemical and Biological Methods: A Novel Approach. SN Applied Sciences 2020, 2 (2). doi:10.1007/s42452-019-1918-x.
- Qasem, N.A.A.; Mohammed, R.H.; Lawal, D.U. Removal of Heavy Metal Ions from Wastewater: A Comprehensive and Critical Review. npj Clean Water 2021, 4 (1), 22. doi:10.1038/s41545-021-00127-0.
- Sundarraj, S.; Sudarmani, D.N.P.; Samuel, P.; Sevarkodiyone, S.P. Bioremediation of Hexavalent Chromium by Transformation ofEscherichia coliDH5α with Chromate Reductase (ChrR) Genes ofPseudomonas Putidaisolated from Tannery Effluent. J. Appl. Microbiol. 2022, 134 (1), doi:10.1093/jambio/lxac019.
- Singh, V.; Singh, N.; Rai, S.N.; Kumar, A.; Singh, A.K.; Singh, M.P.; Sahoo, A.; Shekhar, S.; Vamanu, E.; Mishra, V. Heavy Metal Contamination in the Aquatic Ecosystem: Toxicity and Its Remediation Using Eco-Friendly Approaches. Toxics 2023, 11 (2), 147. doi:10.3390/toxics11020147.
- Singh, V.; Singh, J.; Singh, N.; Rai, S.N.; Verma, M.K.; Verma, M.; Singh, V.; Chivate, M.S.; Bilal, M.; Mishra, V. Simultaneous Removal of Ternary Heavy Metal Ions by a Newly Isolated Microbacterium Paraoxydans Strain VSVM IIT(BHU) from Coal Washery Effluent. BioMetals 2022, 36 (4), 829–845. doi:10.1007/s10534-022-00476-4.
- Ahmed, G.; Jamal, F.; Tiwari, R.K.; Singh, V.; Rai, S.N.; Chaturvedi, S.K.; Pandey, K.; Singh, S.K.; Kumar, A.; Narayan, S.; Vamanu, E. Arsenic Exposure to Mouse Visceral Leishmaniasis Model through Their Drinking Water Linked to the Disease Exacerbation via Modulation in Host Protective Immunity: A Preclinical Study. Sci. Rep. 2023, 13 (1), doi:10.1038/s41598-023-48642-z.
- Singh, V.; Ahmed, G.; Vedika, S.; Kumar, P.; Chaturvedi, S.K.; Rai, S.N.; Vamanu, E.; Kumar, A. Toxic Heavy Metal Ions Contamination in Water and Their Sustainable Reduction by Eco-Friendly Methods: Isotherms, Thermodynamics and Kinetics Study. Sci. Rep. 2024, 14 (1), doi:10.1038/s41598-024-58061-3.
- Singh, V.; Singh, N.; Verma, M.; Kamal, R.; Tiwari, R.; Sanjay Chivate, M.; Rai, S.N.; Kumar, A.; Singh, A.; Singh, M.P.; Vamanu, E.; Mishra, V. Hexavalent-Chromium-Induced Oxidative Stress and the Protective Role of Antioxidants against Cellular Toxicity. Antioxidants 2022, 11 (12), 2375. doi:10.3390/antiox11122375.
- Singh, N.; Singh, V.; Rai, S.N.; Vamanu, E.; Singh, M.P. Metagenomic Analysis of Garden Soil-Derived Microbial Consortia and Unveiling Their Metabolic Potential in Mitigating Toxic Hexavalent Chromium. Life 2022, 12 (12), 2094. doi:10.3390/life12122094.
- Razzak, S.A.; Faruque, M.O.; Alsheikh, Z.; Alsheikhmohamad, L.; Alkuroud, D.; Alfayez, A.; Hossain, S.M.Z.; Hossain, M.M. A Comprehensive Review on Conventional and Biological-Driven Heavy Metals Removal from Industrial Wastewater. Environmental Advances 2022, 7, 100168. doi:10.1016/j.envadv.2022.100168.
- Yadav, M.; Singh, G.; Jadeja, R.N. Physical and Chemical Methods for Heavy Metal Removal. Pollutants and Water Management 2021, 377–397. doi:10.1002/9781119693635.ch15.
- Staszak, K.; Regel-Rosocka, M. Removing Heavy Metals: Cutting-Edge Strategies and Advancements in Biosorption Technology. Materials. (Basel) 2024, 17 (5), 1155. doi:10.3390/ma17051155.
- Qasem, N.A.A.; Mohammed, R.H.; Lawal, D.U. Removal of heavy metal ions from wastewater: a comprehensive and critical review. npj Clean Water 2021, 4, 36. doi:10.1038/s41545-021-00127-0.
- Singh, V.; Mishra, V. Sustainable reduction of Cr (VI) and its elemental mapping on chitosan coated citrus limetta peels biomass in synthetic wastewater. Sep. Sci. Technol. 2021, 57, 1609–1626.