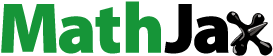
ABSTRACT
Electric arc furnace dust (EAFD), categorized as hazardous waste, contains notable concentrations of zinc. This research involved inducing the in situ deposition of zinc ions from EAFD on activated carbon (AC) surfaces, followed by the formation of ZIF-8 on the AC surface through the addition of 2-methylimidazole. The resulting ZIF-8/AC composite was utilized for adsorbing reactive and dispersed dyes. The characterization of the as-prepared ZIF-8/AC was accomplished by XRD, FT-IR, SEM, EDX, BET, XPS, and TGA. The as-prepared composite was tested for adsorption of reactive blue 19 (RB19) and chromatint yellow 3247 (CY3247) dispersion dyes. The ZIF-8/AC6 adsorbent produced the best removal efficiency for CY3247 (96.47%) and RB19 (98.63%). The highest removal efficiencies were 99.51 and 96.52% for RB19 and CY3247, respectively (concentration of 25 mg/mL). The Langmuir model yielded the maximum adsorption capacity (84.03 mg/g for RB19 and 72.46 mg/g for CY3247) coupled with strong correlation coefficient values. The Langmuir isotherm model was identified as the most fitting representation of equilibrium based on experimental data. The pseudo-second order rate model offers the most accurate depiction of the adsorption kinetics, and the Langmuir model proves suitable for describing the adsorption of RB19 and CY3247 on ZIF-8/AC.
1. Introduction
The coloring agents are extensively employed across the textile, pharmaceutical, food, cosmetic, plastic, photographic, and paper industries, owing to their robust coloring capabilities. The textile sector is a particularly significant contributor to industrial wastewater (Citation1,Citation2). The appeal of fabric is intricately tied to its color, leading to the flourishing business of manufacturing and using synthetic dyes. These dyes offer a broad spectrum of lifelike and vibrant colors. Despite their visual appeal, concerns about the environmental impact arise due to potential toxicity. Textile dyes are implicated in health risks such as cancer, allergic reactions, and mutagenesis (Citation3). Approximately 10–15% of synthetic colors are wasted in various textile manufacturing operations. The incomplete breakdown of colors on textile fibers during aqueous dyeing is a major source of dye release into the environment, and the need to decrease dye residues in textile wastewater has become a key emphasis in recent years (Citation4). The reactive blue 19 (RB19) and chromatint yellow 3247 (CY3247) dispersion dyes are commonly found in high concentrations in effluents from the textile industry. Such dyes are often resistant to degradation and tend to persist in the environment for extended periods of time, increasing their effects on water and the environment (Citation5,Citation6). The CY3247 dye is designed to be suitable for a wide range of industrial applications, including coatings, paints, stains, and graphic arts. These dyes exhibit excellent stability to light and are resistant to bases, acids, and detergents. Meanwhile, various treatment processes for dye removal from wastewater have been extensively researched and used, including coagulation and flocculation, biological treatment with anaerobic granular, catalytic oxidation photochemical treatment, ozone-based oxidation, Fenton’s process, and adsorption. Adsorption was shown to be a more adaptable and efficient method that is widely recognized (Citation7,Citation8).
The removal of hazardous pollutants such as organic dyes is a critical component of wastewater treatment. Numerous porous materials have been investigated for this purpose, but recently developed metal–organic frameworks (MOFs) have piqued the curiosity of researchers due to their enormous surface area and unusual chemical properties (Citation5,Citation9). Zinc-based zeolite imidazolate (ZIF-8) is a typical type of MOFs made from Zn ions and 2-methylimidazole. ZIF-8 was widely employed in a variety of applications, including gas adsorption (Citation10) and separation (Citation11), energy storage (Citation12), photocatalysis (Citation13), electrocatalysis (Citation14), chemical sensing (Citation15), and the adsorption of metal ions and organic dyes (Citation16). ZIF-8 and composite material based on it were used as adsorbents for various organic pollutions, such as methylene blue (Citation17), malachite green (Citation18), acid red 57, remazol red (Citation19), and tetracycline (Citation20). The high adsorption of organic dyes onto ZIF-8 is attributed to Zn2+ exposure to the porous surface, which ensures high adsorption capacity (Citation21,Citation22). Electric arc furnace dust (EAFD) is solid waste generated by the secondary steelmaking process. For every ton of steel produced, approximately 10–30 kg of EAFD is typically generated. EAFD is currently considered a type of waste that has harmful effects on the environment and human health because it contains various unsafe metals. EAFD contains about 7–40% of zinc. The zinc recovery can be obtained from EAF dust by hydrometallurgical and pyrometallurgical methods. The recovering zinc from EAFD not only benefits the steel industry by reducing costs and ensuring compliance but also has broader positive effects on the environment, resource conservation, and the economy. We aim to create an efficient adsorbent by improving materials for organic dye adsorption. ZIF-8 was synthesized with zinc ions from EAFD and combined with 2-methylimidazole. The ZIF-8 was in situ grown on activated carbon, forming the ZIF-8/AC composite that facilitated the adsorption of RB19 and CY 3247 dyes in aqueous solutions. The impact of various adsorption parameters, including contact time, initial dye concentration, temperature, and pH, on the removal of RB19 and CY 3247 were explored. Additionally, the kinetics, isotherms, and thermodynamics of the adsorption process were examined.
2. Materials and methods
2.1. Materials
Zinc sulfate heptahydrate (ZnSO4.7H2O) was obtained from waste zinc from electric arc furnace dust (Phu My, Viet Nam). 2-methylimidazole (C4H6N2) was obtained from Sigma–Aldrich Korea Ltd. Methanol (CH3OH), hydrogen chloride (HCl), and sodium hydroxide (NaOH) were purchased from Merck (Germany). Activated carbon was obtained from Damao Chemical Reagent Factory (China). All chemical reagents were analytical grade and utilized without additional purification. Reactive Blue 19 (RB19) and Chromatint Yellow 3247 were donated from Thanh Cong textile dying company (Viet Nam).
2.2. Characterization
The Fourier transform infrared (FT-IR) spectroscopy conducted on a Tensor 27 spectrophotometer (Bruker, Germany) involved scanning wave numbers within the range of 400–4000 cm−1. X-ray diffraction (XRD) analysis was performed on a LabX XRD-6100 instrument (Shimadzu, Japan) using a Cu Kα radiation source (λ = 1.54 Å) and covered the range of 10–80 o. Morphology and element components were observed using field emission scanning electron microscopy (FESEM), energy-dispersive X-ray spectroscopy (EDX) (SU 8010, Hitachi), and X-ray photoelectron spectroscopy (XPS) (PHI Quantera SXM, Japan). The thermal stability of the composites was assessed using thermogravimetric analysis instruments (LABSYS evo, Setaram Instrumentation) under an air atmosphere, with a heating rate of 10°C/min, ranging from room temperature to 800°C.
2.3. In situ growth of ZIF-8 onto activated carbon (ZIF-8/AC)
Activated carbon (0.05 g) and 2.0 g of ZnSO4.7H2O (0.68 mmol) were dissolved in 40 mL CH3OH and sonicated for 15 min. The resulting mixture was agitated for 3 h at 70°C, then dried for 12 h at 70°C to produce a dry powder with a dark gray color. Subsequently, 0.228 g of 2-methylimidazole (2.4 mmol) in 120 mL of CH3OH was added to the above solution. The resultant mixture was stirred at room temperature for 24 h. After that, the product was centrifuged and washed with ethanol. The ZIF-8/AC with a whitish gray tint was achieved after drying for 5 h at 80°C in a vacuum oven. The various mass ratios of ZnSO4.7H2O and activated carbon powder were conducted (4:1, 6:1, 8:1). The as-synthesized materials were referred to as ZIF-8/AC4, ZIF-8/AC6, and ZIF-8/AC8, respectively.
2.4. Adsorption experiments
The dye adsorption capacity of as-synthesized materials with different ZIF-8 and AC ratios was examined. The experiments were carried out in 50 mL of dye solution (50 mg/L) for 30 min, the solution was shaken at 250 rpm, and the adsorbent dosage was 0.05 g. The adsorbent is then centrifuged out of the solution and the concentration of residual dye in the solution is measured using a spectrophotometer (Agilent Cary 60) at wavelength of 592 nm (RB19) and 376 nm (CY3247). The effect of adsorbent dosage (0.01, 0.03, and 0.05 g) was also investigated for 30 min in 50 mL dye solution (50 mg/L). The influence of dye concentration and initial time were performed using 0.05 g of adsorbent and 50 mL of different dye solutions ranging from 25 to 120 mg/L for RB19 and 25 to 75 mg/L for CY3247. The effect of temperature was conducted with three temperature ranges of 293, 313, and 333 K using 50 mL of 50 mg/L dyes and the dosage of 0.05 mg with the time of 5–60 min. Furthermore, adsorption was performed at different pH values (3–9) by adding HCl (0.1 M) or NaOH (0.1 M) to investigate the effect of pH medium on the adsorption capacity of the produced materials.
The following equation was used to calculate the adsorption efficiency (Equation (1)):
(1)
(1) Where Co and Ce are the initial and equilibrium concentration of dye solution (mg/L).
3. Results and discussion
3.1. Characteristics of adsorbents
shows the FT-IR spectra (400–4000 cm−1) of ZIF-8/AC4, ZIF-8/AC6, and ZIF-8/AC8 samples. Bands at 2918 cm−1 indicate the aromatic and aliphatic C–H stretch of imidazole (). The C=N group’s stretching vibration is responsible for the major band at 1586 cm−1. The band at 423 cm−1 corresponds to Zn-N stretch, while the peaks at 600–1500 cm−1 indicate total ring stretching or bending (Citation23). The ZIF-8/AC composites show similar peaks. Furthermore, on the ZIF-8/AC sample, there is a peak with considerable adsorption at 3364 cm−1, featuring the OH group of activated carbon (Citation24).
The XRD analysis reveals significant disruption in 2θ at 20–30 and 40–50o, confirming the existence of amorphous carbon stacked indirectly by carbon rings (Citation25). ZIF-8 crystals formed a stable breed with ZIF-8 in samples ZIF-8/AC4, ZIF-8/AC6, and ZIF-8/AC8. The findings are consistent with a prior investigation of ZIF-8 (Citation23). Peaks at 2θ = 7.55, 10.37, 12.69, 14.74, 16.72, 18.09, 22.12, 24.61, 25.5, 26.88, 29.78, 30.68, 31.60, and 32.72o indicate a high concentration of crystals in the synthetic ZIF-8 material. Aside from the ZIF-8 peaks, the aforementioned ZIF-8/AC materials eventually formed vertices at 2θ = 41.06, 43.07, 44.22, and 46.23o, which are typical of activated carbon (Citation25). As a result, ZIF-8 has grown on the activated carbon surface ().
Scanning electron microscopy (SEM) images show the surface features and interfacial interactions of pure ZIF-8 and ZIF-8/AC composites. As shown in , a rhombic structure of the ZIF-8 powders was observed. According to the image, the surfaces of both ZIF-8 and ZIF-8/AC materials reveal uniform dodecahedron crystals of ZIF-8. However, the composite material has a large number of cavities, resulting in excellent color absorption. ) depict the elemental mappings for Zn, N, O, and C, respectively. The ZIF-8/AC frame is primarily composed of C (60.6%), N (19.0%), Zn (13.6%), and O (6.7%). Zn signals are dominant on both the deformed particles and the ZIF-8/AC octahedrons. Thus, increases in Zn and O percentages imply the creation of ZnO particles and their random dispersion throughout the matrix (Citation26). When ZIF-8 is combined with activated carbon, the crystal structure is effectively completed on the surface of the activated carbon, resulting in a more irregular material structure than that of pure ZIF-8. This irregularity creates additional pore spaces, leading to ZIF-8/AC materials that possess higher adsorption capacities compared to ZIF-8 alone. Furthermore, carbon circuits of activated carbon linked between crystals aid in the durability of materials, assuring their stability.
Figure 4. Eds mapping analysis of ZIF-8/AC: (a) Zn, (b) N, (c) O, (d) C, (e) overlapped EDS image of Zn, N, O, and C elements and (f) EDX of ZIF-8/AC.
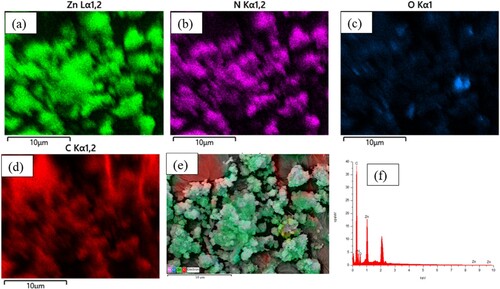
The porosity and surface area of the synthesized samples were calculated using isotherms of nitrogen uptake and excretion at 77 K and atmospheric pressure. displays the surface area for the ZIF-8/AC sample. The synthesized samples exhibit type I isotherms based on the IUPAC classification, indicating a microporous structure. The Brunauer–Emmett–Teller (BET), Langmuir surface area, and micropore volume are 62.5, 114.5 m2/g, and 0.0398 cm3/g, respectively ().
Table 1. Isotherm parameters for the adsorption of RB19 and CY3247 on ZIF-8/AC.
shows the elemental composition and chemical state of the ZIF-8/AC sample. The XPS spectra were measured by ThermoScientific K-Alpha XPS spectrometer. The O, C, N, and Zn elements can be clearly observed in the full spectrum. The high-resolution Zn 2p spectrum, shown in (b), has two significant peaks at 1044.3 and 1021.1 eV, which are well ascribed to the Zn 2p 1/2 and Zn 2p 3/2 components, respectively. It indicates that the chemical state of Zn was +2 (Citation27). The deconvolution C1s peak, shown in (c), has three peaks at the binding energies of 284.6, 285.8, and 286.2 eV, which are associated with C–OH bonds, C–N bonds, and C–O/C–N bonds, respectively (Citation28). The deconvolution N1s peak, shown in (d), has two peaks at 399.8 and 398.4 eV that relate to the N in 2-methylimidazole (Citation29) and pyridinic nitrogen (Citation30). The deconvolution O1s peak, shown in (e), has two peaks detected at a binding energy of 530.2 eV (Zn–O) and 531.7 eV (C–O, C=O and C–O–Zn) (Citation31).
Figure 6. Xps spectra of ZIF-8 catalysts: (a) survey spectrum and high-resolution spectra, (b) Zn 2p, (c) C 1s, (d) N 1s, and (e) O1s.
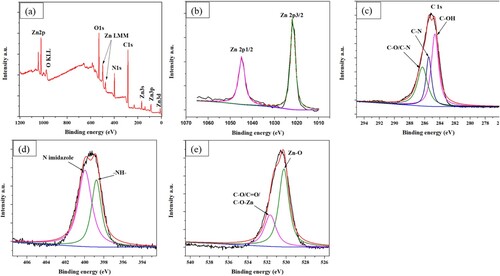
TGA analyses were conducted within the temperature range of 25–800°C, with the results depicted in . Initially, a minor mass loss of about 3% was observed below 150°C, attributed to the evaporation of water molecules and volatile compounds within the ZIF-8/AC composites. Subsequently, between 150°C and 300°C, the pyrolysis of certain oxygen-containing functional groups on the ZIF-8/AC surface led to a further mass loss of 6%. Beyond 300°C, a significant weight reduction of approximately 40% was noted in the TGA curve, extending up to 700°C. This drastic decrease was attributed to the thermal decomposition of carbon and ZIF-8, with 2-MIM decomposing at high temperatures, resulting in the formation of zinc oxide (ZnO) as the final calcination product of ZIF-8. At temperatures exceeding 750°C, the material ceased disintegrating after experiencing a total weight loss of 45%. Comparatively, the volume of ZIF-8/AC material decomposed at temperatures below 700°C was considerably lower than that of pure ZIF-8, which decomposed by 70% at 700°C (Citation32). This indicates that the incorporation of activated carbon as the filler effectively enhanced the thermal stability of ZIF-8.
To further understand the adsorption mechanism, the zeta potential variations of ZIF-8/AC were examined, as shown in . The isoelectric point of ZIF-8/AC was ∼8.5, indicating that the surface charge of the adsorbent was positive at lower pH (<8.5) and perhaps negative at higher pH (>8.5).
3.2. Adsorption experiment for RB19 and CY3247 dyes
3.2.1. Optimization of the ZIF-8 and activated carbon ratio in ZIF-8/AC composite
The initial step of the investigation involved assessing the impact of various combinations of ZIF-8 and activated carbon on adsorption to determine the optimal composite. As depicted in , the adsorption capacity of ZIF-8/AC surpassed that of pure ZIF-8. Notably, among the tested composites, ZIF-8/AC6 exhibited the highest adsorption capacity for both RB19 and CY3247 dyes. The results indicated a superior adsorption capacity of ZIF-8 for CY3247 (75.90%) compared to RB19 (70.34%), aligning with previous findings suggesting ZIF-8’s preference for neutral and cationic molecules over anionic ones (Citation21). However, contrary to this trend, the ZIF-8/AC composite yielded different results, possibly due to activated carbon having a better adsorption capacity for both cationic and anionic dyes compared to dispersed dyes (Citation33). The combination of ZIF-8 with activated carbon appeared to impart adsorption characteristics akin to activated carbon, affirming the successful development of ZIF-8 on activated carbon. Remarkably, the new ZIF-8/AC materials retained the high adsorption efficiency (exceeding 85%) for both RB19 and CY3247 dyes. Among these, ZIF-8/AC6 demonstrated the highest efficiency for CY3247 (96.47%) and RB19 (98.63%). However, ZIF-8/AC4 seemed to present an impractical combination ratio, resulting in relatively low dye adsorption efficiencies of 84.91% for CY3247 and 92.75% for RB19, respectively. As a result, further adsorption studies were carried out utilizing ZIF-8/AC6.
3.2.2. Effect of material dosage
The adsorption efficacy of RB19 and CY3247 was influenced by the dosage of ZIF-8/AC6 (). As anticipated, a higher dose of adsorbent led to greater dye absorption efficiency. As the amount of ZIF-8/AC6 increased from 0.01–0.05 g, the adsorption efficiency of RB19 increased from 44.21% to 98.63%, and that of CY3247 rose from 29.32% to 94.10%. The augmentation in ZIF-8/AC6 dosage resulted in the creation of more adsorption sites (Citation20).
3.2.3. Effect of initial dye concentration and contact time
illustrates the influence of both initial dye concentration and contact time on the removal of RB19 and CY3247. While longer contact times enhance removal efficiency, increasing initial dye concentrations adversely affect the efficacy of ZIF-8/AC in dye removal. Specifically, as the initial concentrations of RB19 dye escalated from 25 to 120 mg/L, removal percentages decreased from 99.51% to 85.30%. Similarly, for CY3247, increasing initial dye concentrations from 25 to 75 mg/L led to a decrease in removal percentages from 96.42% to 77.74%. Moreover, the time taken to achieve equilibrium prolonged with higher dye concentrations. For instance, with initial dye concentrations of 25 mg/L for RB19 or CY3247, equilibrium was attained within 30 min, whereas with a concentration of 75 mg/L, equilibrium required 45 min. Consequently, the elevation in initial dye concentration resulted in heightened electrostatic repulsion forces between dye molecules in the solution and those adsorbed by ZIF-8/AC (Citation34).
3.2.4. Effect of temperature
Various concentrations of CY3247 (ranging from 25 to 75 mg/L) and RB19 (ranging from 25 to 120 mg/L) were employed at different temperatures (293, 313, and 333 K) to investigate the impact of temperature on the removal of dyes from aqueous solutions. The influence of initial dye concentration and temperature is illustrated in . It was observed that the adsorption efficiency rose with increasing temperature, suggesting an endothermic nature of the adsorption process. Specifically, at low dye concentrations (C0 = 25 mg/L), the adsorption capacity showed only a slight increase with rising temperature, such as from 87.93% to 95.91% for CY3247 and from 93.12% to 98.81% for RB19. However, at higher temperatures and initial dye concentrations (C0 > 50 mg/L), significant enhancements in dye removal were noted, with CY3247 increasing from 49.63% to 83.54% and RB19 from 64.92% to 86.34%. These findings suggest that the adsorption process of CY3247 and RB19 on ZIF-8/AC was endothermic.
3.2.5. Effect of pH
At pH values ranging from 3 to 9, the influence of initial pH on RB 19 and CY3247 adsorption ability onto ZIF-8/AC was investigated. These findings indicated that at lower pH, abundant H3O+ ions resulted in a significant capacity to proton ions on the ZIF-8/AC6 surface (Citation35). As a result, in an acidic media, there may be fewer chances to favor positively charged colors ().
3.3. Adsorption isotherms
The Langmuir, Freundlich, and Dubinin–Radushkevich models were used to describe the adsorption of RB19 and CY3247 onto ZIF-8/AC. The Langmuir isotherm illustrates the monolayer and homogeneous adsorption of dye molecules onto the adsorbent surface (Citation36–38). The linear form of Langmuir isotherm is expressed as (Equation (2)):
(2)
(2) where qe (mg/g), Ce (mg/L), qmax (mg/g), and b (l/mg) are the amounts of dye adsorbed at equilibrium, the equilibrium concentration of the adsorbate, the maximum monolayer adsorption capacity, and the Langmuir equilibrium constant, respectively. The b and qmax were calculated from the plot of 1/Ce versus 1/qe.
The Freundlich isotherm assumes that the adsorption of dye onto the heterogeneous surface of the adsorbent is multilayer. The linear form of Freundlich isotherm is given as (Equation (3)):
(3)
(3) where Kf and n are the Freundlich constant and intensity of adsorption, respectively. Kf and n can be determined from the linear plot of log qe against log Ce.
The adsorption isotherm was also described by the Dubinin–Radushkevich model (Citation36). The linear form of Dubinin–Radushkevich isotherm model is represented by the following equation (Equation (4)):
(4)
(4) where qm (mg/g),
(mol2/J2) and
are the adsorption capacity, the constant related to the mean adsorption energy, and the Polanyi potential, respectively.
and qm were determined from the linear plot of qe against
.
and depict the Langmuir, Freundlich, and Dubinin–Radushkevich adsorption isotherm parameters for the RB19 and CY3247 dyes by ZIF-8/AC. Regarding the correlation coefficient (R2) values for the three models, the Langmuir model exhibits a superior fit compared to the Freundlich and Dubinin–Radushkevich models. This finding aligns with a prior investigation on ZIF-8-derived porous carbon material (Citation39). The higher R2 values associated with the Langmuir model suggest that it more accurately describes the experimental results, indicating that RB19 and CY3247 molecules are uniformly adsorbed onto the ZIF-8/AC surface. Moreover, the 1/n constants fall within the range of 0–1 across all temperatures, indicating favorable adsorption of RB19 and CY3247 on ZIF-8/AC (Citation40). Notably, ΔE values are all below 8 kJ/mol, signifying that the adsorption of dyes RB19 and CY3247 on ZIF-8/AC is physisorptive in nature.
Figure 14. (a) Langmuir, (c) Freundlich and (e) Dubinin–Radushkevich models of RB19. (b) Langmuir, (d) Freundlich and (f) Dubinin-Radushkevich models of CY3247.
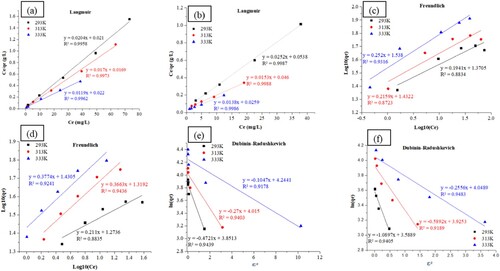
compares the maximum adsorption efficiency of RB19 and other dyes on various adsorbents. The ZIF-8/AC has higher adsorption capacity and efficiency for RB19 than other adsorbents. The ZIF-8/AC composite is regarded to be a promising candidate for textile dyes removal from aqueous solutions.
Table 2. Researches on the removal of textile dyes by various adsorbents.
3.4. Adsorption kinetics
Kinetic investigations provide valuable insights into the mechanisms and speed of the adsorption process. In this study, three models of pseudo-first order, pseudo-second order, and intraparticle diffusion were employed to analyze the adsorption kinetics of RB19 and CY3247 dyes onto ZIF-8/AC under optimal conditions.
The pseudo-first order model (Citation50) is given as (Equation (5)):
(5)
(5) where qe (mg/g) and qt (mg/g), are the mass of dye adsorbed at the equilibrium and time t. k1 (min−1) and t (min) are the rate constant and time of reaction, respectively. K1 and qe were computed from the linear plot of log (qe − qt) against time, respectively.
The pseudo-second order model (Citation51) is presented by the following equation (Equation (6)):
(6)
(6) where k2 (g/mg min) is the pseudo-second-order rate constant. qe and k2 were determined from the slope and intercept of the linear plot of t/qt against t.
The intraparticle diffusion kinetic assumes that the intraparticle diffusion of dye molecules on the adsorbent is the rate-limiting step in the adsorption process (Citation51) The intraparticle diffusion model expressed as (Equation (7)):
(7)
(7) where kid (mg/g min1/2) is the intraparticle diffusion rate constant, and C is a constant that describes the thickness of the boundary layer. kid and C were calculated from the linear plot of qt versus t1/2.
The adsorption kinetics of ZIF-8/AC was studied based on the above adsorption equilibrium results of RB19 and CY3247 dyes. By plotting a graph of processing time T (min) vs. ln(Qe − Qt), the pseudo-first order kinetic model fitting curves can be obtained as shown in . By plotting a graph of processing time T (min) vs. t/Qt, the pseudo-second order kinetic model fitting curves can be obtained as shown in . By plotting the graph of t0.5 vs. Qt, the fitting curve of particle diffusion can be obtained as shown in . According to the formulas given above, the calculated kinetic parameters are summarized in .
Table 3. Kinetic parameters for dye adsorption onto ZIF-8/AC.
The adsorption equilibrium of RB19 and CY3247 dyes, as measured from the experiment, was found to be 48.35 and 45.91 mg/g, respectively. By comparing the theoretical average adsorption quantity (qe) and the correlation coefficient (R2) of the fitting curve from , it becomes evident that, under the same conditions, the pseudo-second order kinetic model for dynamic adsorption is more accurate in describing the adsorption process of ZIF-8/AC for RB19 and CY3247, relative to the pseudo-first order kinetic model and intraparticle diffusion model. The correlation coefficient of the pseudo-second order kinetic model for RB19 was determined to be 0.9997, indicating a higher degree of conformity in the adsorption process of ZIF-8/AC with RB19. Furthermore, the intercept of the diffusion equation for RB19 particles was larger, indicating a more significant adsorption effect on the inner surface. However, the linear fitting of the two dyes to qt vs t0.5 was low, suggesting that the particle diffusion process on ZIF-8/AC does not control the adsorption rate for RB19 and CY3247. These findings imply that complexation, coordination, or chelation may occur between the adsorbent and the adsorbate through covalent or electronic exchange, representing a chemical adsorption process and revealing the inherent regularities of ZIF-8/AC adsorption.
The adsorption of RB19 and CY3247 dyes on ZIF-8/AC was studied, with emphasis on electrostatic attraction, pore-filling effects, π–π interactions, and hydrogen bonding interactions (Citation52,Citation53). The development of ionic bonding between the negatively charged functional groups of RB19 (–SO3¯) and positively charged Zn2+ in ZIF-8 may be the primary contributor to the rapid and high RB19 adsorption on ZIF-8/AC. The results of this investigation demonstrated ZIF-8/AC’s capacity to efficiently absorb dye and its prospective applications in wastewater treatment.3.5 Thermodynamic studies.
The thermodynamic parameters including standard free energy change (G0), enthalpy (
H0), and entropy (
S0), were examined by following equations (Equations (8)–(10)).
(8)
(8)
(9)
(9)
(10)
(10) where R is the universal gas constant (8.314 J/mol K), T is the absolute temperature in Kelvin, and Kd is the equilibrium constant obtained from Langmuir constant KL. By drawing the Van’t Hoff plot of ln Kd versus 1/T, the
,
and
values were found from the slope and intercept.
lists the ,
, and
values at various temperatures for RB19 and CY3247 adsorption on ZIF-8/AC. When assessing the obtained thermodynamic parameters, negative
values reveal that RB19 and CY3247 adsorption on ZIF-8/AC is spontaneous. With increasing temperature, the increase in the negativity of ΔGo confirms that the ‘favorability’ increases with temperature. Moreover, the positive values of
show that an increase occurs in the randomness in the system of the solid/solution interface during the adsorption process. The fact that
values are positive reconfirms that the adsorption of RB19 and CY3247 is endothermic (Citation40,Citation54,Citation55).
Table 4. Thermodynamic parameters for RB19 and CY3247 adsorptions onto ZIF-8/AC.
3.6. Regeneration and stability
Regeneration and stability are essential considerations when evaluating the adsorbent’s practical applicability. The reusability of the produced material was investigated using four desorption–adsorption cycles at pH 3. As a result, the adsorbent’ s removal effectiveness against RB19 decreased from 99.51% to 81.52% after four consecutive cycles. The re-adsorption of RB19 onto ZIF-8/AC remained 90.21%. This indicates that the prepared composite ZIF-8/C showed good regenerability.
4. Conclusion
The impact of various operational factors on the removal of dyes from colored textile wastewater was investigated utilizing ZIF-8/AC6, a composite adsorbent consisting of ZIF-8 grown in situ over activated carbon. Compared to pure ZIF-8, ZIF-8/AC exhibited significantly higher efficacy in removing RB19 and CY3247 dyes. The study revealed that the removal of dyes was influenced by several parameters including adsorbent dosage, initial dye concentration, contact time, temperature, and pH. The highest efficacy for dyes removal using ZIF-8/AC was determined to be at pH 9 with an adsorbent dosage of 0.05 g/L. The maximum removal efficiencies for CY3247 (96.47%) and RB19 (98.63%) were achieved with the ZIF-8/AC6 adsorbent. RB19 and CY3247 had maximum removal efficiencies of 99.51% and 96.52%, respectively. The maximum adsorption capacity (84.03 mg/g for RB19 and 72.46 mg/g for CY3247) was obtained using the Langmuir model, which also produced good correlation coefficient values. The adsorption process onto ZIF-8/AC followed a pseudo-second-order kinetic model and Langmuir isotherm. In summary, ZIF-8/AC proves to be an efficient dye removal system suitable for application in aquatic environments.
Acknowledgments
This research was conducted at the Industrial University of Ho Chi Minh City, Viet nam and Ho Chi Minh City University of Industry and Trade, Viet nam.
Disclosure statement
No potential conflict of interest was reported by the author(s).
References
- Yaseen, D.A.; Scholz, M. Textile Dye Wastewater Characteristics and Constituents of Synthetic Effluents: A Critical Review. Int. J. Environ. Sci. Technol. 2019, 16, 1193–1226. DOI: 10.1007/s13762-018-2130-z.
- Abou-Hadid, A.F.; El-Behairy, U.A.; Elmalih, M.M.; Amdeha, E.; El Naggar, A.M.A.; Taha, M.H.; Hussein, A.E.M. Conversion of Corn Shell as Biomass Solid Waste into Carbon Species for Efficient Decontamination of Wastewater via Heavy Metals Adsorption. Biomass Convers. Biorefin. 2023, Published: 20 March 2023. DOI: 10.1007/s13399-023-04057-4.
- Lellis, B.; Fávaro-Polonio, C.Z.; Pamphile, J.A.; Polonio, J.C. Effects of Textile Dyes on Health and the Environment and Bioremediation Potential of Living Organisms. Biotechnol. Res. Innov. 2019, 3, 275–290. DOI: 10.1016/J.BIORI.2019.09.001.
- Pavithra, K, Engineering VJ-J of I and, 2019 Undefined. Removal of Colorants from Wastewater: A Review on Sources and Treatment Strategies. J. Ind. Eng. Chem. 2019, 75, 1–19. DOI: 10.1016/j.jiec.2019.02.011.
- Dalvand, A.; Nabizadeh, R.; Reza Ganjali, M.; Khoobi, M.; Nazmara, S.; Hossein Mahvi, A. Modeling of Reactive Blue 19 Azo Dye Removal from Colored Textile Wastewater using L-Arginine-Functionalized Fe3O4 Nanoparticles: Optimization, Reusability, Kinetic and Equilibrium Studies. J. Magn. Magn. Mater. 2016, 404, 179–189. DOI: 10.1016/J.JMMM.2015.12.040.
- Siddique, M.; Farooq, R.; Khan, Z.M.; Khan, Z.; Shaukat, S.F. Enhanced Decomposition of Reactive Blue 19 Dye in Ultrasound Assisted Electrochemical Reactor. Ultrason. Sonochem. 2011, 18, 190–196. DOI: 10.1016/j.ultsonch.2010.05.004.
- Khrueakham, A.; Masomboon, J.; Roongruang, J.; Sairiam, S. Efficient Reactive Blue 19 Decolorization by the Comparison of Ozonation Membrane Contacting Process and Fenton Oxidation. RSC Adv. 2021, 11, 17775–17788. DOI: 10.1039/d1ra01871j.
- El-Desouky, M.G.; Alayyafi, A.A.; Al-Hazmi, G.A.A.M.; El-Bindary, A.A. Effect of Metal Organic Framework Alginate Aerogel Composite Sponge on Adsorption of Tartrazine from Aqueous Solutions: Adsorption Models, Thermodynamics and Optimization via Box-Behnken Design. J. Mol. Liq. 2024, 399, 124392. DOI: 10.1016/j.molliq.2024.124392.
- Alsuhaibani, A.M.; Alayyafi, A.A.; Albedair, L.A.; El-Desouky, M.G.; El-Bindary, A.A. Efficient Fabrication of a Composite Sponge for Cr(VI) Removal via Citric Acid Cross-linking of Metal–organic Framework and Chitosan: Adsorption Isotherm, Kinetic Studies, and Optimization Using Box-Behnken Design. Mater. Today. Sustain. 2024, 26, 100732. DOI: 10.1016/j.mtsust.2024.100732.
- Lai, W.-H.; Wang, D.K.; Wey, M.-Y.; Tseng, H.-H. ZIF-8/Styrene-IL Polymerization Hollow Fiber Membrane for Improved CO2/N2 Separation. J. Clean. Prod. 2022, 372, 133785. DOI: 10.1016/j.jclepro.2022.133785.
- Hu, Z.; Zhang, H.; Zhang, X.F.; Jia, M.; Yao, J. Polyethylenimine Grafted ZIF-8@Cellulose Acetate Membrane for Enhanced Gas Separation. J. Memb. Sci. 2022, 662, 120996. DOI: 10.1016/j.memsci.2022.120996.
- Ebrahimi, Z.; Rad, M.; Safarifard, V.; Moradi, M. Solvent-Assisted Ligand Exchange as a Post-synthetic Surface Modification Approach of Zn-based (ZIF-7, ZIF-8) and Co-based (ZIF-9, ZIF-67) Zeolitic Frameworks for Energy Storage Application. J. Mol. Liq. 2022, 364, 120018. DOI: 10.1016/j.molliq.2022.120018.
- El Ouardi, M.; El aouni, A.; Ait Ahsaine, H.; Zbair, M.; BaQais, A.; Saadi, M. ZIF-8 Metal Organic Framework Composites as Hydrogen Evolution Reaction Photocatalyst: A Review of the Current State. Chemosphere 2022, 308, 136483. DOI: 10.1016/j.chemosphere.2022.136483.
- Xie, J.; Yang, H.; Wang, X.; Gao, F. ZIF-8/Electro-reduced Graphene Oxide Nanocomposite for Highly Electrocatalytic Oxidation of Hydrazine in Industrial Wastewater. Microchem. J. 2021, 168, 106521. DOI: 10.1016/j.microc.2021.106521.
- Miao, M.; Mu, L.; Cao, S.; Yang, Y.; Feng, X. Dual-functional CDs@ZIF-8/Chitosan Luminescent Film Sensors for Simultaneous Detection and Adsorption of Tetracycline. Carbohydr. Polym. 2022, 291, 119587. DOI: 10.1016/j.carbpol.2022.119587. Cited: in: PMID: 35698342.
- Zhang, T.; Jin, X.; Owens, G.; Chen, Z. Remediation of Malachite Green in Wastewater by ZIF-8@Fe/Ni Nanoparticles Based on Adsorption and Reduction. J. Colloid Interface Sci. 2021, 594, 398–408. DOI: 10.1016/j.jcis.2021.03.065.
- Feng, Y.; Li, Y.; Xu, M.; Liu, S.; Yao, J. Fast Adsorption of Methyl Blue on Zeolitic Imidazolate Framework-8 and Its Adsorption Mechanism. RSC Adv. 2016, 6, 109608–109612. DOI: 10.1039/c6ra23870j.
- Khoshnamvand, N.; Jafari, A.; Kamarehie, B.; Mohammadi, A.; Faraji, M. Removal of Malachite Green Dye from Aqueous Solutions Using Zeolitic Imidazole Framework-8. Environ. Process. 2019, 6, 757–772. DOI: 10.1007/s40710-019-00384-9.
- El-Desouky, M.G.; El-Bindary, A.A. Magnetic metal–organic framework (Fe3O4@ZIF-8) nanocomposites for adsorption of anionic dyes from wastewater. Inorg. Nano-Met. Chem. 2021, Published online: 09 Dec 2021. DOI: 10.1080/24701556.2021.2007131.
- Sun, S.; Yang, Z.; Cao, J.; Wang, Y.; Xiong, W. Copper-doped ZIF-8 With High Adsorption Performance for Removal of Tetracycline from Aqueous Solution. J. Solid State Chem. 2020, 285, 121219. DOI: 10.1016/j.jssc.2020.121219.
- Luan Tran, B.; Chin, H.Y.; Chang, B.K.; Chiang, A.S.T. Dye Adsorption in ZIF-8: The Importance of External Surface Area. Microporous Mesoporous Mater. 2019, 277, 149–153. DOI: 10.1016/j.micromeso.2018.10.027.
- Al-Hazmi, G.A.A.; El-Bindary, M.A.; El-Desouky, M.G.; El-Bindary, A.A. Efficient Adsorptive Removal of Industrial Dye from Aqueous Solution by Synthesized Zeolitic Imidazolate Framework-8 Loaded Date Seed Activated Carbon and Statistical Physics Modeling. Desalination Water Treat 2022, 258, 85–103. DOI: 10.5004/dwt.2022.28397.
- Niknam Shahrak, M.; Ghahramaninezhad, M.; Eydifarash, M. Zeolitic Imidazolate Framework-8 for Efficient Adsorption and Removal of Cr(VI) Ions from Aqueous Solution. Environ. Sci. Pollut. Res. 2017, 24, 9624–9634. DOI: 10.1007/s11356-017-8577-5. Cited: in:PMID: 28247275.
- Yan, X.; Li, Y.; Hu, X.; Feng, R.; Zhou, M.; Han, D. Enhanced Adsorption of Phenol from Aqueous Solution by Carbonized Trace ZIF-8-Decorated Activated Carbon Pellets. Chin. J. Chem. Eng. 2021, 33, 279–285. DOI: 10.1016/j.cjche.2020.06.027.
- Li, Y.; Yan, X.; Hu, X.; Feng, R.; Zhou, M.; Han, D. In situ Growth of ZIF-8 Onto Porous Carbons as an Efficient Adsorbent for Malachite Green Removal. J. Porous Mater. 2020, 27, 1109–1117. DOI: 10.1007/s10934-020-00887-z.
- Tuncel, D.; Ökte, A.N. Improved Adsorption Capacity and Photoactivity of ZnO-ZIF-8 Nanocomposites. Catal Today [Internet] 2021, 361, 191–197. DOI: 10.1016/j.cattod.2020.04.014.
- Li, C.; Hu, C.; Zhao, Y.; Song, L.; Zhang, J.; Huang, R.; Qu, L. Decoration of Graphene Network with Metal–Organic Frameworks for Enhanced Electrochemical Capacitive Behavior. Carbon. N. Y. 2014, 78, 231–242. DOI: 10.1016/j.carbon.2014.06.076.
- Pan, D.; Wang, L.; Li, Z.; Geng, B.; Zhang, C.; Zhan, J.; Yin, L.; Wang, L. Synthesis of Graphene Quantum Dot/Metal–Organic Framework Nanocomposites as Yellow Phosphors for White Light-Emitting Diodes. New J. Chem. 2018, 42, 5083–5089. DOI: 10.1039/c7nj04909a.
- Yang, J.; Zhao, F.; Zeng, B. Well-Defined Gold Nanoparticle@N-doped Porous Carbon Prepared from Metal Nanoparticle@Metal–Organic Frameworks for Electrochemical Sensing of Hydrazine. RSC Adv. 2016, 6, 23403–23410. DOI: 10.1039/c6ra00096g.
- Pu, X.; Li, L.; Liu, M.; Jiang, C.; Du, C.; Zhao, Z.; Hu, W.; Lin Wang, Z.; Pu, X., Li, L., et al. Wearable Self-Charging Power Textile Based on Flexible Yarn Supercapacitors and Fabric Nanogenerators. Adv. Mater. 2016, 28, 98–105. DOI: 10.1002/adma.201504403.
- Wang, D.; Chi, M.; Zhang, D.; Wu, D. Ammonia Sensing Properties of Metal–Organic Frameworks-Derived Zinc Oxide/Reduced Graphene Oxide Nanocomposite. J. Mater. Sci.: Mater. Electron. 2020, 31, 4463–4472. DOI: 10.1007/s10854-019-02778-9.
- Amin, P.; Shojaei, A.; Hamzehlouyan, T. ZIF-8/Chitosan Hybrid Nanoparticles With Tunable Morphologies as Superior Adsorbents Towards Both Anionic and Cationic Dyes for a Broad Range of Acidic and Basic Environments. Microporous Mesoporous Mater. 2022, 343. 112149. DOI: 10.1016/j.micromeso.2022.112149.
- Zhang, T.; Jin, X.; Owens, G.; Chen, Z. Remediation of Malachite Green in Wastewater by ZIF-8@Fe/Ni Nanoparticles Based on Adsorption and Reduction. J. Colloid Interface Sci. [Internet] 2021, 594, 398–408. DOI: 10.1016/j.jcis.2021.03.065.
- Nguyen, T.H.A.; Tran, T.D.M.; Ky Vo, T.; Nguyen, Q.T.; Nguyen, V.-C. Facile Synthesis of Low-Cost Chitosan/Fe3O4@C Composite for Highly Efficient Adsorption of Levofloxacin Antibiotic. Chem. Eng. Commun. [Internet] 2023, 210, 1073–1085. DOI: 10.1080/00986445.2022.2053680.
- Li, Y.; Yan, X.; Hu, X.; Feng, R.; Zhou, M.; Han, D. In situ Growth of ZIF-8 Onto Porous Carbons as an Efficient Adsorbent for Malachite Green Removal. J. Porous Mater. 2020, 27, 1109–1117. DOI: 10.1007/s10934-020-00887-z.
- Karmaker, S.; Nag, A.J.; Saha, T.K. Adsorption of Reactive Blue 4 Dye Onto Chitosan 10B in Aqueous Solution: Kinetic Modeling and Isotherm Analysis. Russ. J. Phys. Chem. A [Internet] 2020, 94, 2349–2359. DOI: 10.1134/s0036024420110126.
- Vo, T.K.; Trinh, T.P.; Nguyen, V.C.; Kim, J. Facile Synthesis of Graphite Oxide/MIL-101(Cr) Hybrid Composites for Enhanced Adsorption Performance Towards Industrial Toxic Dyes. J. Indus. Eng. Chem. [Internet] 2021, 95, 224–234. DOI: 10.1016/j.jiec.2020.12.023.
- An, T.N.M.; Phuc, T.T.; Nhi, D.N.T.; Van Cuong, N. Removal of Reactive Red Dye by Reusable Chitosan-Polyaniline/fe3O4 Nanocomposite. Vietnam J. Chem. [Internet] 2020, 58, 477–481. DOI: 10.1002/vjch.201900145.
- Hakro, R.A.; Mehdi, M.; Qureshi, R.F.; Mahar, R.B.; Khatri, M.; Ahmed, F.; Khatri, Z.; Kim, I.S. Efficient Removal of Reactive Blue-19 Dye by Co-electrospun Nanofibers. Mater. Res. Express [Internet] 2021, 8, 55502. DOI: 10.1088/2053-1591/abfc7d.
- Hassan, N.; Shahat, A.; El-Didamony, A.; El-Desouky, M.G.; El-Bindary, A.A. Mesoporous Iron Oxide Nano Spheres for Capturing Organic Dyes from Water Sources. J. Mol. Struct. [Internet] 2020, 1217, 128361. DOI: 10.1016/j.molstruc.2020.128361.
- Jawad, A.H.; Rangabhashiyam, S.; Abdulhameed, A.S.; Syed-Hassan, S.S.A.; ALOthman, Z.A.; Wilson, L.D. Process Optimization and Adsorptive Mechanism for Reactive Blue 19 Dye by Magnetic Crosslinked Chitosan/MgO/Fe3O4 Biocomposite. J. Polym. Environ. 2022, 30, 2759–2773. DOI: 10.1007/s10924-022-02382-9.
- Jawad, A.H.; Abdulhameed, A.S.; Malek, N.N.A.; ALOthman, Z.A. Statistical Optimization and Modeling for Color Removal and COD Reduction of Reactive Blue 19 Dye by Mesoporous Chitosan-Epichlorohydrin/Kaolin Clay Composite. Int. J. Biol. Macromol. 2020, 164, 4218–4230. DOI: 10.1016/j.ijbiomac.2020.08.201.
- Nga, N.K.; Thuy Chau, N.T.; Viet, P.H. Preparation and Characterization of a Chitosan/MgO Composite for the Effective Removal of Reactive Blue 19 Dye from Aqueous Solution. J. Sci.: Adv. Mater. Dev. 2020, 5, 65–72. DOI: 10.1016/j.jsamd.2020.01.009.
- Jawad, A.H.; Abdulhameed, A.S.; Surip, S.N.; Alothman, Z.A. Hybrid Multifunctional Biocomposite of Chitosan Grafted Benzaldehyde/Montmorillonite/Algae for Effective Removal of Brilliant Green and Reactive Blue 19 Dyes: Optimization and Adsorption Mechanism. J. Clean. Prod. 2023, 393, 136334. DOI: 10.1016/j.jclepro.2023.136334.
- Anitha T, Senthil Kumar P, Sathish Kumar K. Synthesis of Nano-sized Chitosan Blended Polyvinyl Alcohol for the Removal of Eosin Yellow Dye from Aqueous Solution. J. Water Process Eng. 2016, 13, 127–136. DOI: 10.1016/j.jwpe.2016.08.003.
- Pagalan Jr, E.; Sebron, M.; Gomez, S.; Salva, S.J.; Ampusta, R.; Macarayo, A.J.; Joyno, C.; Ido, A.; Arazo, R. Activated Carbon from Spent Coffee Grounds as an Adsorbent for Treatment of Water Contaminated by Aniline Yellow Dye. Ind. Crops Prod. 2020, 145, 111953. DOI: 10.1016/j.indcrop.2019.111953.
- Ahmad, N.; Suryani Arsyad, F.; Royani, I.; Lesbani, A. Charcoal Activated as Template Mg/Al Layered Double Hydroxide for Selective Adsorption of Direct Yellow on Anionic Dyes. Results Chem. 2023, 5, 100766. DOI: 10.1016/j.rechem.2023.100766.
- Ecer, Ü; Zengin, A.; Şahan, T. Magnetic Clay\Zeolitic Imidazole Framework Nanocomposite (ZIF-8@Fe3O4@BNT) for Reactive Orange 16 Removal from Liquid Media. Colloids Surf. A. Physicochem. Eng. Asp. 2021, 630, 127558. DOI:10.1016/j.colsurfa.2021.127558.
- Nguyen, T.T.; Tran, T.P.; Nguyen, T.H.A.; Cao, X.T.; Nguyen, V.C. Effective Removal of Reactive Blue 198 from Aqueous Solutions by Hybrid Chitosan–PANI/Fe3O4@C Composite Based on Rice Husk. Desalination Water Treat 2022, 273, 270–280. DOI: 10.5004/dwt.2022.28888.
- Vo, T.K.; Phuong, N.H.Y.; Nguyen, V.C.; Quang, D.T. ZIF-67 Grafted-boehmite-PVA Composite Membranes with Enhanced Removal Efficiency Towards Cr(VI) from Aqueous Solutions. Chemosphere [Internet] 2023, 341, 139996. DOI: 10.1016/j.chemosphere.2023.139996.
- Hussain, S.; Kamran, M.; Khan, S.A.; Shaheen, K.; Shah, Z.; Suo, H.; Khan, Q.; Shah, A.B.; Rehman, W.U., Al-Ghamdi, Y.O., et al. Adsorption, Kinetics and Thermodynamics Studies of Methyl Orange Dye Sequestration Through Chitosan Composites Films. Int. J. Biol. Macromol. [Internet] 2021, 168, 383–394. DOI: 10.1016/j.ijbiomac.2020.12.054.
- Luan Tran, B.; Chin, H.-Y.; Chang, B.K.; Chiang, A.S.T. Dye Adsorption in ZIF-8: The Importance of External Surface Area. Microporous Mesoporous Mater. 2019, 277, 149–153. DOI: 10.1016/j.micromeso.2018.10.027.
- Panda, J.; Sahoo, J.K.; Panda, P.K.; Sahu, S.N.; Samal, M.; Pattanayak, S.K.; Sahu, R. Adsorptive Behavior of Zeolitic Imidazolate Framework-8 Towards Anionic Dye in Aqueous Media: Combined Experimental and Molecular Docking Study. J. Mol. Liq. 2019, 278, 536–545. DOI: 10.1016/j.molliq.2019.01.033.
- Fathi, M.R.; Asfaram, A.; Farhangi, A. Removal of Direct Red 23 from Aqueous Solution Using Corn Stalks: Isotherms, Kinetics and Thermodynamic Studies. Spectrochim. Acta A Mol. Biomol. Spectrosc. [Internet] 2015, 135, 364–372. DOI: 10.1016/j.saa.2014.07.008.
- Prasad, C.; Karlapudi, S.; Venkateswarlu, P.; Bahadur, I.; Kumar, S. Green Arbitrated Synthesis of Fe3O4 Magnetic Nanoparticles with Nanorod Structure from Pomegranate Leaves and Congo Red Dye Degradation Studies for Water Treatment. J. Mol. Liq. [Internet] 2017, 240, 322–328. DOI: 10.1016/j.molliq.2017.05.100.