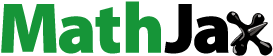
ABSTRACT
Green synthesis of four novel nonionic polymeric surfactants: from the recycled product of poly (ethylene terephthalate) plastic waste for application in corrosion protection of carbon steel. In this respect, PET waste was subjected to aminolysis and glycolysis via a reaction with Trimethylenediamine (TEDA) and Tri ethylene glycol (TEG) in the presence of suitable catalysts. The prepared materials were characterized by FT-IR, 1HNMR, and elemental analysis. It was evaluated as a corrosion inhibitor for steel (type-X60) used in the petroleum industry in the marine environment. Chemical, analytical, and electrochemical techniques were used for the evaluation of the corrosion inhibition efficiency of the prepared polymeric surfactants. The effect of concentration and temperature was studied. The maximum inhibition efficiency (IE) of 96% at an inhibitor concentration of 200 ppm and a temperature of 303 K. The glycoside and amide products were compared, the efficiency of amide products (96%) was higher than the efficiency of glycoside products (91%). According to potentiodynamic polarization data, the prepared surfactant boosts polarization resistance and inhibition performance by adsorbing on the metal/electrolyte interface. The surface morphology of steel was examined using SEM. A protective coating of inhibitor molecules forms on the steel surface.
1. Introduction
The widely used thermoplastic polyethylene terephthalate (PET) produces tons of waste due to its non-biodegradability in the environment, which may otherwise upset the ecosystem's balance (Citation1–3). Plastic bottles (PET) are produced annually in large amounts and due to the single use of the PET bottles; it was left in the environment causing environmental pollution (Citation1–4). Finding quick solutions to get rid of the solid and plastic trash represented by plastic bottles is also critical. Because of the wide range of applications for it in a wide range of consumer products, a lot of PET trash is generated, including waste from the production of polymers and products that have reached the end of their useful lives. Environmental contamination caused by the accumulation of solid and plastic trash causes major environmental issues (Citation1–5). Because of the increasing pressure to keep the environment clean, the only solution is to recycle PET waste in an environmentally sustainable manner. PET waste can be recycled in several ways, including chemical and physical recycling. Chemical recycling of PET to usable materials is a recognized process among mechanical, thermal, and chemical recycling techniques that adhere to the principles of sustainable development (Citation1–7). Several chemical depolymerization techniques, such as methanolysis, glycolysis, hydrolysis, aminolysis, aminolysis, and hydrogenation, are used for PET chain scission based on the types of chemical reagents (Citation1–7). Chemical recycling can depolymerize PET trash to base monomers or oligomers. The two main pathways for polymer solvolysis, also known as solvent of depolymerization, are methanolysis and glycolysis (Citation4–7). Steel corrosion control is critical from a technical, economic, and environmental standpoint. Corrosion inhibitors are commonly used to reduce corrosion attack on steel surfaces (Citation4–7). Steel's lifetime increases due to inhibitors’ ability to slow down the corrosion process (Citation4–7). The majority of effective acid inhibitors are organic compounds containing nitrogen, oxygen, and/or sulfur; these compounds adsorb on steel surfaces to slow corrosion (Citation8–12). The chemical composition of the inhibitors, the presence of electro donating or repelling groups, the inhibitor's molecular weight, temperature, and the electrochemical potential at the metal/solution interface all influence the strength of the adsorption (Citation13, Citation14). Surfactant inhibitors have many advantages, including high inhibition efficiency, low toxicity, and ease of manufacture (Citation7, Citation15). Adsorption of the surfactant on the metal surface can significantly alter the metal's ability to resist corrosion (Citation15–18). Tantawy et al, studied the effect of naturally synthesized cationic surfactants as inhibitors for steel pipeline corrosion in CO2-3.5%NaCl using electrochemical studies and DFT theoretical calculations (Citation19). Hany et al, used empirical and quantum chemical studies to evaluate the corrosion inhibition performance of some novel synthesized cationic gemini surfactants on carbon steel pipelines in acid pickling processes (Citation20, Citation21). This article is part of a series aimed to reducing environmental pollution caused by the accumulation of plastic waste by recycling waste into modified products and evaluating the abilities of those modified products to prevent corrosion of metals and alloys in various aqueous conditions (Citation7, Citation22–25). The present work is one from a series aimed to alleviating the environmental pollution caused by the accumulation of PET waste and evaluation of the products as corrosion inhibitors for metals and alloys in different corrosive environments (Citation1–18). The previously published papers focusing on the study of the corrosion in an acidic media like (HCl, H2SO4, and HNO3) all contain H+ ions (investigate the effects of H+ presence) with the conclusion of different inhibition efficiencies. Reviewing the reported inhibition efficiencies in the literature were summarized in the . In the present study, PET plastic waste was recycled via aminolysis and glycolysis. Depolymerization via reaction with Trimethylenediamine (TEDA) in the presence of a catalyst and Tri ethylene glycol (TEG) in the presence of a transesterification catalyst. The corresponding products are poly amino amide (PETTEDAA) and poly glycol ester (PETTEG) respectively. The obtained materials were reacted with oleic acid to produce the N1,N4-bis(3-((E)-octadec-9- enamido)propyl)terephthalamide (PETTEDAA-Oli) and bis((E)-13-oxo-3,6,9,12-tetraoxatriacont-21-en-1-yl)terephthalate(PETTEG-Oli). The recycled surfactant materials are characterized using elemental and spectral analysis then evaluated as corrosion inhibitors for carbon steel in a marine environment. Chemical, analytical, and electrochemical techniques were used for the evaluation of the corrosion inhibition efficiency of the prepared inhibitors. Studying the change in concentrations, temperature, and adsorption models is the other target of the present work. The novelty of the present work was summarized in the following points:
Using green synthesis methods for recycling PET waste instead of traditional chemical recycling.
Green synthesis of four novel nonionic surfactants.
Comparison between green aminolysis recycling and green glycolysis recycling of PET waste. (amide and glycoside products were compared in terms of green synthesis and inhibition performance to corrosion.
Applying the products of non-ionic surfactants in the corrosion control of carbon steel used in the petroleum industry in the marine environment.
Using steel samples for a study similar to the carbon steel used in the petroleum industry, (type-X60 carbon steel rods (CSX60, SABIC) have a comparable composition to the carbon steel used in many heavy industries like boilers, automobiles, and water tanks.
Using analytical, chemical, and electrochemical techniques for evaluation of the corrosion inhibition efficiency of the prepared non-ionic surfactants inhibitors.
Comparison of the finding results with the previously published works and suggestion of the corrosion inhibition mechanism.
Table 1. Summary of the reported review previous studies compared with the present study.
2. Experimental
2.1. Materials and solutions
Analytical grade chemicals are used which were obtained from Sigma Aldrich Chemicals Co., with the exception of PET waste, which was collected from beverage drinking water bottles. The bottle label and other contaminants were removed, and the PET waste was chopped into 1 mm2 pieces, washed with detergent and water, dried, and recycled in a green manner. shows the gravimetric composition of the steel materials used in this study (type-X60 carbon steel rods (CSX60, SABIC) it has a comparable composition to the carbon steel used in many heavy industry like boilers, automobiles, and water tanks. The corrosive solution prepared in this study is an aggressive 3.5% sodium chloride solution as an artificial seawater prepared from analytical grade 99.9% sodium chloride (Sigma Aldrich).
Table 2. The chemical percent composition of the studied metal alloy.
2.2. Solvent-free green recycling of PET waste
Solvent-free mixing of 10 g of cleaned, Chopped, and dried PET waste with 30 g of tri methylene di amine (TEDA) and (1.0 wt %) sodium acetate as a catalyst with 0.5 acetic acid as co-catalyst was added to the reaction mixture and refluxed under the continuous current of nitrogen (scheme 1). The reaction mixtures in both cases were heated for 4 hours at a temperature of about 170–190 °C and for 3 hours at 200 °C while being vigorously stirred in a nitrogen environment. The reaction's temperature was subsequently decreased to 100 °C for one hour. It took some time for the combination to cool to room temperature. Phase separation was performed once the reaction was complete, and the products were separated in an organic layer. The same procedure applied with glycolysis with triethylene glycol with changing only sodium acetate and acetic acid by (1.0 wt%) manganese acetate which act as transesterification catalyst for glycolysis process as shown in scheme 2. The product obtained in case of depolymerization with tri methylene diamine TEDA is (N1,N4-bis(3-aminopropyl)terephthal amide) symbolized here as (PETTEDAA)), whereas, the product obtained in case of depolymerization with triethylene glycol (TEG) is bis(2-(2-(2-(2-hydroxyethoxy)ethoxy)ethoxy)ethyl)terephthalate symbolized here as (PETTEG), as shown in and .
2.3. Nonionic-surfactants synthesis from PET recycled products
Polymeric surfactants are synthesized from the reaction of recycled products of PET with both TEDA and TEG which whre prepared from the previous step. The refluxing of both (PETTEDAA) and (PETTEG) compounds separately with oleic acid with xylene in the presence of p-toluene sulfonic acid and refluxed for 7 hours in xylene until the calculated amount of water was removed. The schematic diagram of the full synthesis process as in and . The obtained polymeric surfactants are N1,N4-bis(3-((E)-octadec-9-enamido)propyl)terephthalamide symbolized as (PETTEDAA-Oli) and bis((E)-13-oxo-3,6,9,12-tetraoxatriacont-21-en-1-yl)terephthalate, symbolized as(PETTEG-Oli). The prepared polymeric surfactant (PETTEDAA-Oli) and (PETTEG-Oli) were used for the preparation of the corrosion inhibitor in the present study.
2.4. Spectroscopic and elemental analysis
The Cairo University Micro Analytical Center performed spectroscopic and elemental analyses. Aldrich Co. was the source of the fine chemicals. The resulting compounds were crystallized and then further purified using a column chromatography process while the reactions were being tracked using TLC.
2.5. Nuclear magnetic resonance spectroscopy analysis
In order to determine the chemical structure and the HLB by comparing the integral traces of various peaks, the produced surfactants were dissolved in DMSO and subjected to spectroscopic analysis using a Jeol NMR spectrometer model JNM-EX (270 MHz). The data of 1NMR are represented in the Supplementary Figures S1, S3, S5, and S7).
2.6. Infrared spectroscopic analysis
The pure produced chemicals were evaluated using an ATI Mattson Genesis Series FT-IR spectrophotometer. The materials’ thin films were examined between two KBr discs. The data of FT-IR are represented in the Supplementary Figures S2, S4, S6, and S8).
2.7. Gravimetric measurements using direct and indirect gravimetric techniques
2.7.1. Weight loss measurement (direct gravimetric technique)
The weight loss measurements and other studied techniques were conducted under static conditions not dynamic conditions. Static condition is important to be sure the applied current is stable in electrochemical measurements. In the weight loss the static condition helped to adsorption of surfactant inhibitor molecule on the steel surface but the dynamic agitation cause the desorption and heling of the inhibitor out of the steel surface. Weight loss (WL) method is based on calculating the mass loss (ML) of a coupon sample of the surface area (S) that has been submerged in the abrasive solution for a period of time (t). The tests are carried out in non-aerated media in 100 ml glass vials at room temperature. The iron samples are cleaned with distilled water, degreased with acetone, and then dried before and after the 48-hour immersion. The weight loss technique was applied for all the studied temperatures ranging from 303,313,323,333 K. The following relationship (Citation15–18) was used to calculate the corrosion rate W:
(1)
(1) where W (mg.cm−2.h−1) is the corrosion rate, mi (mg) and mf (mg) are the mass before and after exposure to test solution, respectively, S (cm2): is the surface of area of specimen, t (h): is the immersion time. Regarding the inhibitory efficiency I.E.% and the surface coverage (θ), which represents the part of the metal surface covered by the inhibitors molecules, were calculated according to the following equations (Citation15–18):
(2)
(2)
(3)
(3) where Wo and W represent the corrosion rates in the absence and presence of the inhibitors, respectively.
2.7.2. Atomic absorption spectroscopy (AAS) (indirect gravimetric technique))
The quantity identification of the amounts of iron ions in corrosive solutions in both the absence and presence of the employed inhibitor the atomic absorption spectroscopy was utilized (AAS). Atomic absorption spectroscopy on a Varian Spectra AA 220 was used to concentrate the ferric ions introduced into the solution. We dissolved the corrosive medium with aqua regia in order to measure the amounts of iron ions in the corrosive solution both when the inhibitor was absent and present (Citation26, Citation29–33).
2.8. Thermometric measurements
The carbon steel sheet was press cut 2×2×0.1 cm with chemical composition as mentioned in . The measurements were carried out in a Dolvacpyrex flask covered with a sheet of aluminum foil. The reaction vessel consists of 50 ml of the test solution put into the flask covered with sheets of aluminum foil, corked with a Check temp digital thermometer in place. The metal coupon was introduced into the corrosive solution and quickly covered. Thermometric measurements depend on measuring the temperature variation during the reaction of a metal with the test piece with a definite volume of a corroding solution (Citation26, Citation29–33). The variation of temperature of the system was monitored with time and the reaction number (RN) is defined as (Citation26, Citation29–33).
(4)
(4)
Where Tm and Ti are the maximum and initial temperature respectively, tm is the time in minutes taken to attain the maximum temperature.
2.9. Open circuit potential measurement
At 30 °C, the potential of the carbon steel electrode was measured in 3.5% NaCl solution against a saturated calomel electrode (SCE) in the absence and presence of varied amounts of the employed inhibitor. The Multi-tester was used for all measurements until the steady-state potentials were attained.
2.10. Potentiodynamic polarization measurements (electrochemical studies)
The tests are carried out utilizing the radiometer analytical, Volta master for all electrochemical measurements (PGZ301, DYNAMIC ELS VOLTAMMETRY). A platinum wire counter electrode is employed. Calomel is used as a reference electrode because it is standard and all potential is attributed to it. In addition, as a working electrode, a steel cylindrical electrode (1 cm2 in area) is used. Silicon carbide abrasive sheets of grades 600, 1000, 1200, 1500, and 2000 are used to abrade and clean it. The electrode was then rinsed with distilled water, degreased with ethyl alcohol, cleaned with water, and dried to be ready to serve as a functioning electrode. Before each experiment, this protocol was followed. For 50 minutes, the working electrode was immersed in the corrosive solutions. The electrical scanning is done at a 1 mV/sec scan rate. Using the following mathematical equation the inhibition efficiency percent I.E.% and surface coverage (θ) were computed (Citation15–18).
(5)
(5)
(6)
(6)
Where I and Io are the corrosion current densities in the absence of inhibitors and in the presence of inhibitors, respectively. The corrosion potential (Ecorr), corrosion current (Icorr), and Tafel constants (βa & βc) were computed and tabulated using the values of the cathodic and anodic Tafel slopes obtained from the Tafel plot.
2.11. Electrochemical impedance measurements (electrochemical studies)
A three-electrode assembly with calomel as a reference electrode, platinum wire as a counter electrode, and carbon steel specimens with an exposed surface area of 1 cm2 as a working electrode was used in the electrochemical studies. Impedance responses were measured at an open circuit potential (frequency range: 10–100 KHz, 5 mV amplitude). All of the tests and measurements were conducted at room temperature in a motionless or unstirred environment. The inhibitory efficiency was calculated using charge-transfer resistance, which was represented as Rp in the Nyquist plots’ impedance data (Citation34–40).
The inhibition efficiency was determined using charge-transfer resistance, which was labeled as Rct in the Nyquist plots’ impedance data, as follows (Citation34–40):
(7)
(7)
The charge transfer resistance with inhibitor is Rct, while the charge transfer resistance without an inhibitor is Rct0.
2.12. Scanning electron microscope (SEM)
The surface morphology of the carbon steel was examined before and after treatment using a scanning electron microscope(SEM) image. Scanning electron microscopy was used to get information about the changes that occurred on the surface of corrosive samples before and after the addition of the selected inhibitors. The specimens were first immersed in 3.5% NaCl solutions in the absence and presence of optimal concentrations (200 ppm) of the tested compounds for 48 h at room temperature, then taken out from the test solutions, cleaned with bi-distilled water and acetone, and dried with cool air. The SEM images were conducted using JEOL model JSM-53000 scanning electron microscope (SEM).
3. Results and discussion
3.1. Chemistry of the green synthesis process (recycling process)
Solvent free degradation of polyethylene terephthalate plastic waste (PET) with both trimethylene di amine TEDA and tri ethylene glycol TEG to produce corresponding amide and ester. Both the produced amide and aster were separately reacted with oleic acid to produce the corresponding oleic acid amide and oleic acid ester. The elemental analysis of the prepared compounds are illustrated in . The details of the chemistry of the synthesis procedures are mentioned in detail as the following:
Table 3. Show the elemental analysis of the prepared surfactants materials from PET.
3.1.1. Synthesis of (N1,N4-bis(3-((E)-octadec-9-enamido)propyl)terephthalamide)
Solvent free green synthesis of amide based non-ionic surfactant compound (N1,N4-bis(3-((E)-octadec-9-enamido)propyl)terephthalamide). Poly ethylene terephthalate (PET) (1% w/w) and a tri molecular ratio of trimethylene diamine (TEDA) (3% w/w) were refluxed for 6 hours at temperature (190–200 °C) as indicated in Part 2.2. The reaction proceeded in the presence of a co-catalyst made up of 1.0% sodium acetate as a catalyst and 1.0% acetic acid as a co-catalyst (W % from the total weight of the reactants) (Citation4–6). Saline water was added to the reaction mixture after it had been cooled to 100 °C and brought to room temperature in order to remove bi-product glycol. The result was a yellowish, viscous residue, which was further purified using column chromatography. depicts the product's chemical makeup and the synthesis method in diagram form. The obtained PETTEDAA compound was reacted with oleic acid in the presence of sodium acetate as a catalyst and acetic acid as a co-catalyst in the presence of p-toluene sulfonic acid and refluxed for 7 hours in xylene until the calculated amount of water was produced. The schematic diagram of the full synthesis process as in . The reaction produces the corresponding oleic acid phthalimide compound, ((N1,N4-bis(3-((E)-octadec-9-enamido)propyl)terephthalamide)), the elemental analysis of the prepared compound is shown in . This compound symbolized here as (PETTEDAA-Oli) and used as corrosion inhibitor for carbon steel in marine corrosive environment. shows the chemical structure of the nonionic surfactant (PETTEDAA-Oli) inhibitor molecule and the scheme of the synthesis process.
3.1.2. Synthesis of bis((E)-13-oxo-3,6,9,12-tetraoxatriacont-21-en-1-yl)terephthalate
For the green synthesis of surfactant compound (PETTEG-Oli), poly ethylene terephthalate (PET) (1% w/w) and a tri molecular ratio of Propylene glycol (PG) (3% w/w) were refluxed for 6 hours at temperature (190–200 °C) as indicated in Part 2.3. The reaction proceeded in the presence of anhydrous manganese acetate (CH3COO)2Mn) as a transesterification catalyst (1% by weight compared to the total weight of the reactants (Citation4–6). Hot saline water was added to the reaction mixture after it had been cooled to 100 °C and brought to room temperature in order to remove bi-product glycol. The result was a white gelatinous residue, which was further purified using column chromatography. depicts the product's chemical compounds and the synthesis method in diagram form. The obtained PETTEG compound was reacted with oleic acid in the presence of p-toluene sulfonic acid and refluxed for 7 hours in xylene until the calculated amount of water was produced. The schematic diagram of the full synthesis process as in . The reaction produces the corresponding oleic acid ester compound, bis((E)-13-oxo-3,6,9,12-tetraoxatriacont-21-en-1-yl)terephthalate. The prepared compound in this step designed in the (PETTEG-Oli) and used as corrosion inhibitor for carbon steel in 3.5% NaCl corrosive environment, the elemental analysis of the prepared compound is shown in . shows the chemical structure of the nonionic surfactant (PETTEG-Oli) inhibitor molecule and scheme of the synthesis process.
3.2. Spectroscopic characterization of the prepared polymeric compounds
The green synthesized polymeric surfactant compounds were characterized using FT-IR, 1HNMR, and elemental analysis as the following:
3.2.1. Characterization of PETTEDAA compound using FT-IR, 1HNMR
The described works used infrared spectroscopy to determine the characteristic function groups in both organic and inorganic materials. From the scaling IR spectra, the frequency scaling factors for stable configuration were theoretically computed (Citation41). The prepared PETTEDAA compound with the IUPAC name N1,N4-bis(3-aminopropyl)terephthalamide which was elucidated using (FT-IR (Figure S2) and (1HNMR9 Figure S1). The resulting IR spectra of this compound show a strong bands at v cm−1, including 3421–3274 (NH2, NH), 2830–2950 (C–H methylene groups), and 1665 (C = O) cm−1. The products’ end with amino groups is indicated by the appearance of a prominent band at 3421 cm−1 in the spectra. On the other hand, the band seen at 810 cm−1 for all depolymerized PET is attributed to p-substituted phenyl's -CH out-of-plane bending. This band verifies that depolymerized materials include phenyl rings. Strong peaks at 1745 cm−1, which were attributed to amide group C = O stretching, show that all depolymerized PET products contain the amide functional group. 1HNMR provides additional support for the products of PET with trimethylene diamine (TEDA). In this regard, the PETTEDAA spectra display signals at chemical shifts of 8 ppm and 4.5 ppm, respectively, which stand in for the p-substituted phenyl group and COOCH2CH2O-H of the generated PET. The 6.93 signal was seen (s, NH). The product compound made from PET waste contains active amino amide groups, according to the results of FT-IR and 1HNMR analysis.
3.2.2. Characterization of (PETTEDAA –oli) compound using FT-IR, 1HNMR
Green synthesized surfactant compound (PETTEDAA –Oli) from the reaction between oleic acid and recycled product of PET compound PETTEDAA d which was obtained in the previous step (point 3.2.1). The IUPAC name of this compound suggested as (N1,N4-bis(3-((E)-octadec-9-enamido)propyl)terephthalamide) this suggestion was depended on its FT-IR (Figure S4) and 1HNMR (Figure S3). The resulting IR spectra of this compound show the disappearance of the strong band at v cm−1, 3421 (NH2), this is due to changing of terminal (NH2) into amide (CONH) through the reaction with the carboxylic group of the oleic acid. This result was proved by the presence of the strong absorption band at v cm−1, 3274 (NH), and band at v cm−1, 1665 (CO) indicating the presence of Amide linkage in the PETTEDAA –Oli compound. Proton nuclear magnetic resonance 1HNMR of the prepared PETTEDAA –Oli compound provides additional support. 1HNMR spectra display signals at chemical shifts of 8 ppm and 4.5 ppm, respectively, which stand in for the p-substituted phenyl group and COOCH2CH2O- of the generated PET. The 8.3 signal was seen (s, NH). The 5.4 signal was seen (d, HC = CH) for the unsaturated double for the oleic acid reacting backbone. In addition, to the appearance of (m, CH2) signals at (1.28, 1.3, 1.33, 2.16). all this observation proves that the product compound made from PETAA and oleic acid is the PETTEDAA –Oli compound of IUPAC name (N1,N4-bis(3-((E)-octadec-9-enamido)propyl)terephthalamide) as shown in . This confirmation was depended on the synthesis procedure and the results of FT-IR and 1HNMR analysis.
3.2.3. Spectroscopic characterization of PETTEG compound
Green synthesized compound symbolized PETTEG which prepared from the recycling of PET with tri ethylene glycol TEG with suggested IUPAC name of (bis(2-(2-(2-(2-hydroxyethoxy)ethoxy)ethoxy)ethyl)terephthalate)its structure was confirmed by FT-IR (Figure S6) and 1HNMR (Figure S5) analysis. The significant broad band appears in IR spectrum at v cm���1, 3350(OH) is a characteristic band for the terminal hydroxyl group (OH) . with the presence of bands at v cm−1, (2900, 1100) (aliphatic groups). With the significant sharp band at v cm−1, (1740, 1171, (C = O, C-O ester group). The 1HNMR (Figure S5) spectrum of the resulting PETTEG compound display signals at chemical shifts of 8 ppm and 4.5 ppm, respectively, which stand in for the p-substituted phenyl group and COOCH2CH2O- of the generated PET. The 5.3 signal was seen (s, OH), and 1.11 signal was seen (t, CH3 terminal), the 3.9 signal was seen (s, CH), and 3.8, 4.7 signals for (m, -O-CH2CH2-C = O) methylene groups.
3.2.4. Spectroscopic characterization of PETTEG –oli compound
Nonionic polymeric surfactant compound symbolized as (PETTEG –Oli) derived from the reaction of oleic acid with (PETTEG) compound prepared from the previous step (point 3.2.3) was characterized by FT-IR (Figure S8), 1HNMR (Figure S7) spectroscopic analysis. The IR spectra (Figure S8) of this compound show the disappearance of the broadband at v cm−1, 3350 (OH) due to the incorporation of a hydroxyl group in the reaction with the carboxylic acid group of the oleic acid molecule forming the corresponding ester group with band appears at v cm−1, 1740, 1115 (O = C-O-C). The 1HNMR analysis (Figure S7) of the (PETTEG –Oli) compound show the disappearance of the 5.3 signal was seen (s, OH) proving the incorporation of (OH) group in the formation of the ester group when it react with oleic acid in the presence of PTSA. The presence of 5.4 signal indicating for (d, HC = CH) for the unsaturated double for the oleic acid reacting backbone. In addition, to the appearance of (m, CH2) signals at (2.16, 1.29, 1.33, 1.66, and 2.35) for (HC = CH2-CH2-O-CH2-CH2-OCH2CH2-C = O). All this observation proves that the product compound made from PETTEG and oleic acid is the PETTEG –Oli compound of IUPAC name bis ((E)-13-oxo-3,6,9,12-tetraoxatriacont-21-en-1-yl)terephthalate as shown in . This confirmation suggestion was depended on the synthesis procedure and the results of FT-IR and 1HNMR analysis.
3.3. Applications of the prepared surfactants for corrosion protection of steel
Nonionic polymeric surfactants materials synthesized in the present study symbolized as (PETTEDAA –Oli, PETTEDAA, PETTEG-Oli, and PETTEG) were applied for the protection of steel substrate in the marine environment. For the evaluation of the inhibition performance of the prepared surfactants towards the carbon steel substrate, chemical, analytical, and electrochemical techniques are used. The chemical techniques (weight loss method) are used for studying the effect of all inhibitors concentrations range (50–200 ppm). Whereas, the analytical techniques which are atomic absorption spectroscopy (AAS) and thermometric measurements were used for the evaluation of different concentrations (50–200 ppm) from the four prepared polymeric surfactant materials (PETTEDAA –Oli, PETTEDAA, PETTEG-Oli, and PETTEG). From the results of chemical and analytical techniques, it was concluded that the order of corrosion inhibition performance of the four prepared nonionic polymeric surfactants is: PETTEDAA –Oli > PETTEDAA > PETTEG-Oli > PETTEG. The effect of temperatures (30, 40, 50, and 60 °C) on the corrosion inhibition efficiency was also studied using 200-ppm inhibited solution from the four prepared materials. Finally, the electrochemical techniques (potentiodynamic polarization (PDP), open circuit potential (OCP), and Electrochemical impedance spectroscopy(EIS) as accurate, fast, and high-performance techniques were used for the evaluation of the efficiency of the esterified amide derivative (PETTEDAA –Oli) towards the protection of the steel substrate used in the manufacturer of the petroleum pipelines, in the petroleum industry.
3.3.1. Mass loss measurements (direct gravimetric measurements)
Weight loss of the steel coupons in the presence and absence of the different concentrations (50–200 ppm) from the four prepared nonionic polymeric surfactants (PETTEDAA –Oli, PETTEDAA, PETTEG-Oli, and PETTEG) were studied using mass loss method (Citation16–19). A sodium chloride solution of 3.5% concentration as an artificial seawater is used as a blank solution which causes the corrosion of steel materials in the marine environment. illustrates the surface coverage (θ), corrosion rate, and corrosion inhibition efficiency of the four prepared nonionic polymeric surfactant materials (PETTEDAA –Oli, PETTEDAA, PETTEG-Oli, and PETTEG) at four selected concentrations (50, 100, 150, and 200 ppm). The corrosion rate, corrosion inhibition efficiency, and surface coverage (θ) were calculated from the mathematical formulas (equations 1, 2, and 3) respectively. From we can conclude that: the inhibition efficiency of all prepared materials was found to increase with increasing the inhibitor concentrations reaching maximum inhibition efficiency of 93% using 200 ppm of the (PETTEDAA –Oli) inhibitor. The observed inhibition efficiency in the case of amide-based inhibitor compounds (PETTEDAA –Oli and PETTEDAA) is more than the inhibition efficiency in the case of glycol-based inhibitor compounds (PETTEG-Oli and PETTEG). From it was concluded that the order of corrosion inhibition performance of the four prepared nonionic polymeric surfactants is: PETTEDAA –Oli > PETTEDAA > PETTEG-Oli > PETTEG. This observation may be due to the strong linkage formed between the amide bond and the steel surface (Citation1–5). , , , and depicts the weight loss of steel in 3.5% NaCl as artificial seawater over time with and without the presence of four-prepared polymeric surfactant inhibitor materials derived from the recycling of PET plastic waste. As shown in , , , and , the weight loss of steel materials is increased due to the corrosion process with a time of immersion in the corrosive environment; this observation is for both blank and inhibited solutions as well. The corrosion rates increase as immersion time increases, but decrease as inhibitor concentration increases. The efficiency of corrosion inhibition improves as the concentration of inhibitors rises. The inhibition of the prepared nonionic polymeric surfactants due to adsorption, adhesion, and passive layer generation on the steel surface, the formed passive layer protecting the steel against corrosion in marine corrosive environment (Citation15–18).
Figure 3. Depicts the weight loss of steel in 3.5% NaCl over time with and without the presence of a prepared polymeric surfactant (PETTEDAA -Oli) inhibitor derived from plastic waste.
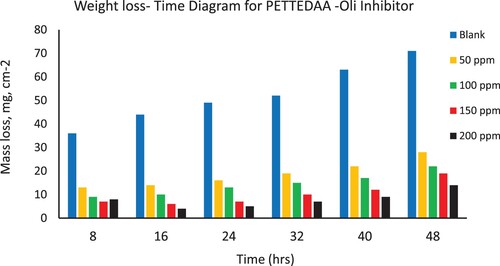
Figure 4. Depicts the weight loss of steel in 3.5% NaCl over time with and without the presence of a polymeric surfactant (PETTEDAA) inhibitor derived from plastic waste.
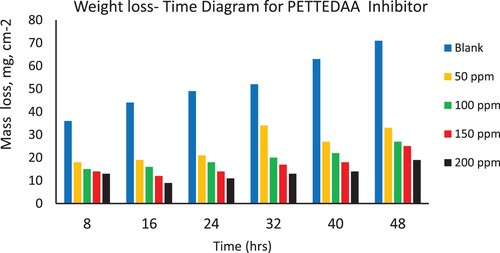
Figure 5. Depicts the weight loss of steel in 3.5% NaCl over time with and without the presence of a polymeric surfactant (PETTEG-Oli) inhibitor derived from plastic waste.
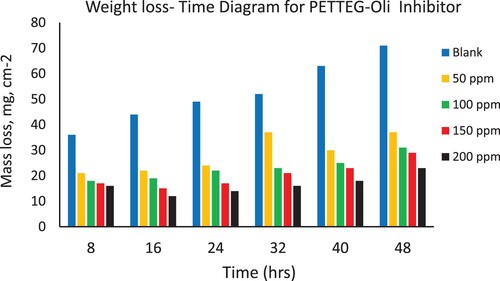
Figure 6. Depicts the weight loss of steel in 3.5% NaCl over time with and without the presence of a prepared polymeric surfactant (PETTEG) inhibitor derived from plastic waste.
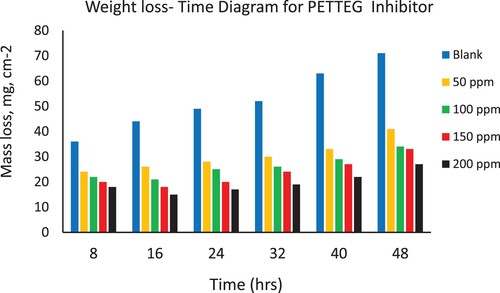
Table 4. Corrosion inhibition parameters for various concentrations of the prepared nonionic surfactants at 303 k, for c-steel in marine environment data from Mass loss.
3.3.2. Effect of temperature
To understand the inhibitory mechanism and determine the kinetic parameters of the corrosion process, gravimetric (weight loss) measurements were taken at different temperatures, 303,313,323,333 K. The percentage of steel that is inhibited in the presence of the inhibitor is graphically depicted in as being affected by temperature. Indicating that the protective film of these compounds generated on the surface of the steel is less stable at higher temperatures, it is evident that the % I.E values declined as the temperature increased. This might be because more of the metal is exposed to the corrosive environment at higher temperatures. After all, certain molecules that have been adsorbed desorb from the surface of the steel (Citation15–18).
3.4. Analytical measurements
3.4.1. Indirect gravimetric measurements (AAS)
The iron ions released into the solution as a result of corrosion were immediately identified using atomic absorption spectroscopy (AAS). High salinity, falling pH, chloride ion presence, and temperatures all accelerated the corrosion of iron samples in solution (Citation34–36). When iron is exposed to oxygen, humidity, and chloride ions, a complex process known as iron corrosion occurs. Following the corroding material's characteristics will allow you to calculate the societal costs of corrosion of iron structures or other components (Citation34–36). The current study employed atomic absorption spectroscopy to determine the concentrations of ferrous ions Fe2+ produced by 3.5% NaCl corrosion of steel. The ions flowed into the solution were concentrated using AAS to determine the amount of ferrous ions present in corrosive solution when the green inhibitor was absent or present. According to the AAS data in and , the ferrous ion Fe2+ concentrations in the corrosive medium were decreased by increasing inhibitor concentration. The concentration of ferrous ions in the solution is modeled as a function of the corrosion rate in this case; as a result, as the concentration of ferrous ions in the solution rises, so does the corrosion rate, and vice versa. Every step is affected by the addition of an inhibitor. From it was observed that the concentration of ferrous ions in the corrosive solution was found to decrease by increasing of the inhibitor concentrations. The observed ferrous ion concentration in the case of amid-based inhibitor compounds (PETTEDAA –Oli, and PETTEDAA) is lower than the observed ferrous ion concentrations in the case of glycol-based inhibitor compounds (PETTEG-Oli, and PETTEG). The lowest ferrous ions concentrations were observed in the case of amide-based nonionic polymeric surfactant material (PETTEDAA –Oli) inhibitor compound. The data in show that the results of the two gravimetric approaches (weight loss method and ASS method) are in good agreement, demonstrating that the addition of prepared inhibitor materials inhibits steel corrosion in a marine environment and reduces the iron dissolving process.
Figure 8. Graphical presentation of the Atomic Absorption Spectroscopy (AAS) data of the prepared surfactants from PET waste used as inhibitor for steel in 3.5% NaCl solution.
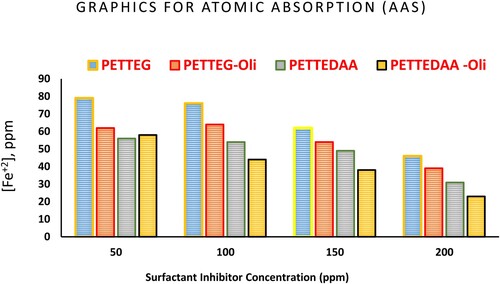
Table 5. Atomic absorption spectroscopy results from corrosion of steel alloy in marine environment in the presence and absence of the prepared polymeric surfactants.
3.4.2. Thermometric measurements (analytical technique)
Reaction number (RN) values are known as a relative measure of retardation of the dissolution process (Citation26, Citation29–33). The extent of corrosion inhibition can have expressed in terms of the percentage reduction in reaction number (% RR) given by the following equation (Citation26, Citation29–33).
(8)
(8)
The thermometric parameters and inhibition efficiency are summarized in . The inhibition efficiency and Δtm increase by increasing inhibitor concentrations. The reduction in the reaction number and time delay (Δtm) of the four studied polymeric surfactant inhibitor materials increases in the case of amide-based inhibitors than in glycol-based inhibitors. It was found in the following order PETTEDAA -Oli > PETTEDAA > PETTEG-Oli > PETTEG. This observation agrees well with the data obtained from both mass loss measurement and atomic absorption spectroscopy (AAS). Meaning that the data of different studied techniques are in good agreement with each other to prove the good protection efficiency of the four prepared nonionic polymeric surfactants towards the steel surface.
Table 6. Inhibited artificial NaCl solution thermometric parameters by the prepared polymeric surfactants as inhibitor for c-steel.
3.5. Adsorption of polymeric materials on steel surface
The protection of steel from corrosion in the corrosive environment due to the adsorption and adhesion of inhibitor molecules on the steel surface forming a barrier layer. The charge and type of the metal surface, its electronic properties, the adsorption of solvent and other ionic species, the temperature at which corrosion occurs, and the electrochemical potential at the solution interface are the primary factors influencing the adsorption process. The inclusion of electro-repelling or electro-donating groups in derivatives. The adsorption of the four studied inhibitor materials on the steel surface was studied using weight loss data. The adsorption behavior of the inhibitor materials on the metal substrate is a function of protection and inhibition performance.
The calculated values of the surface coverage (θ) at various inhibitor concentrations were used to explain the best fit isotherm for the adsorption process. The Langmuir adsorption isotherm best fits the results, according to the following equation (Citation34, Citation42–61):
(9)
(9) Where K and C are the adsorption process's equilibrium constant and the inhibitor concentration, respectively (Citation34, Citation42–61). The straight line obtained by plotting Ci/θ vs Ci is shown in . 1/K is the intercept of the line with approximately unit slope. The standard free energy of adsorption ΔG°ads is calculated using the equation (Citation62–65):
(10)
(10)
Figure 9. Adsorption of the four prepared surfactant inhibitors on the C-steel surface immersed in 3.5% NaCl solution at 30 °C according Langmuir adsorption isotherm model.
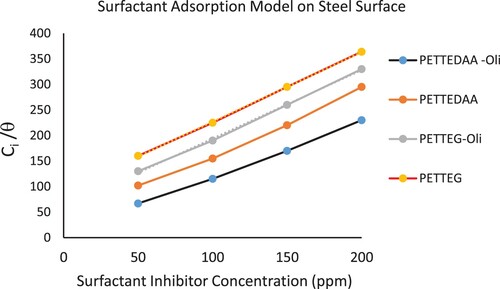
Where T is the absolute temperature, R is the gas constant, and the majority of the solution per liter contains 55.5 moles of water. The adsorption equilibrium constant is denoted by Kads. In the case of amide-based polymeric surfactant material (PETAA-OL) inhibitor the ΔG°ads has been calculated to be −38.63 kJ/mol. The absence of a positive result for ΔG°ads indicates that inhibitors molecules adsorb spontaneously on the metal surface (Citation57, Citation66–70). However, the obtained value is less than the −40 kJ/mol threshold value required for chemical adsorption, indicating that the adsorption mechanism is physical (Citation15–18). The investigated compound is widely acknowledged to inhibit corrosion by adhering at the metal/solution contact. Furthermore, it is believed that the development of a solid organic molecule complex with a metal atom has piqued the interest of many (Citation57, Citation66–70).
3.6. Open circuit potential measurements
A steady-state open circuit potential was measured by submerging the working electrode in the corrosion medium for 30 minutes before performing any electrochemical tests. Following 30 minutes of immersing in 3.5% NaCl corrosive solution alone and with the different inhibitor concentrations. The focus of the research was to discover the change in the open-circuit potential (OCP) of the carbon steel when it was placed in a 3.5% NaCl corrosive solution at 298 K, at conditions where corrosion could occur naturally (Citation27–71). shows the relation between open Circuit potential (OCP) and time of the immersion for the blank solution and inhibited solution of different concentrations (50,100,150, and 200 ppm) of the nonionic polymeric surfactant compound (PETTEDAA –Oli) prepared from the recycling of PET plastic waste. The steady state was observed after 50 min of the immersion time. The potential of a steel electrode immersed in 3.5% NaCl solution (blank curve) clearly moves to a higher negative potential first, resulting in a short step. According to the equation (Citation15–18):
(11)
(11)
Figure 10. Open Circuit Potential (OCP) vs time measurements for C- steel immersed in 3.5% NaCl solution in the absence and presence of nonionic surfactant inhibitor (PETTEDAA -Oli) compound derived from recycled PET waste.
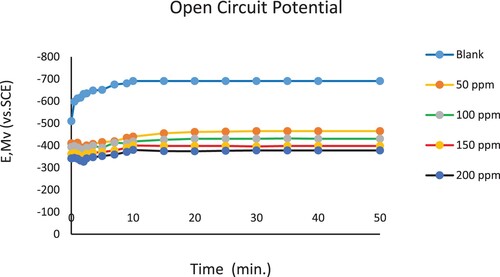
Following this, a new oxide film forms within the solution, shifting the potential back to a more noble direction until a steady-state potential is reached. When inhibitor molecules are added to the aggressive medium, the cathodic reaction is slowed, resulting in a negative shift in the open circuit potential (Citation16–18).
3.7. Potentiodynamic polarization measurements (PDP)
The electrochemical techniques (potentiodynamic polarization (PDP), and Electrochemical impedance spectroscopy(EIS) as accurate, fast, and high-performance techniques were used for the evaluation of the efficiency of the esterified amide derivative (PETTEDAA –Oli) towards the protection of the steel substrate used in the manufacturer of the petroleum pipelines, in the petroleum industry. Potentiodynamic polarization curves are used to investigate the components of the corrosion inhibition process that cause an anodic/cathodic process change. Potentiodynamic polarization curves are used to investigate the components of the corrosion inhibition process that cause an anodic/cathodic process change lists the parameters that were estimated. The polymeric surfactant inhibitor compound (PETTEDAA –Oli) was shown to have an excellent ability to form a surface coating on the steel surface via adsorption. Inhibitors can alter the process by slowing down the rate of corrosion. The corrosion current density progressively decreases as the concentration of the synthesized compound rises from 50 to 200 ppm. As the concentration of the synthesized materials goes up, there is a corresponding increase in both the extent of surface coverage and the leverage of corrosion inhibition. This may be due to the inhibitor molecules adsorbing onto the surface of the carbon steel electrode when it is submerged in a 3.5.% NaCl corrosive solution, ultimately forming a protective layer on the electrode surface. Previous studies have reported that when a corrosion inhibitor is present in a corrosive substance, it can impede both the anodic and cathodic reactions on a metal surface. This ultimately leads to a decrease in corrosion current density (Citation27–72). The corrosion current density and potential are determined by the intersection of the anodic and cathodic curves. When compared to a blank, Ecorr tends toward value in the presence of an inhibitor, but no discernible difference has been found (). I corr. is determined by extrapolating of the anodic and cathodic Tafel lines with steady-state potential (Ecorr.). As a result, the nonionic surfactant inhibitor compound (PETTEDAA –Oli) functions as a mixed-type. The Icorr values decreased as polymeric surfactants inhibitor concentrations increased, indicating that the formation of a shield on the carbon steel surface in the presence of inhibitors impedes electrochemical processes (Citation57, Citation63–68).
Figure 11. Tafel curves (Potentiodynamic Polarization) for C-steel immersed in .5% NaCl solution at 30 °C in the absence and presence of nonionic surfactant inhibitor (PETTEDAA -Oli) compound derived from recycled PET waste.
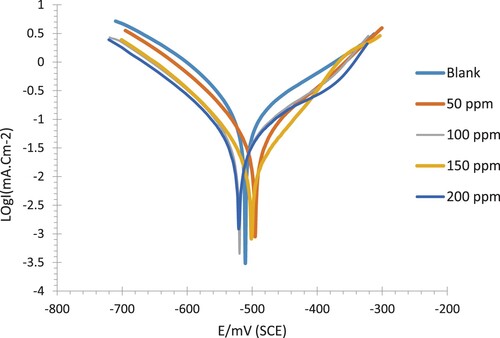
Table 7. Potentiodynamic (PDP) polarization parameters for corrosion and inhibition of steel by the surfactant polymer compound (PETTEDAA -Oli) in 3.5% NaCl solution at 303 k.
3.8. Electrochemical impedance spectroscopy, EIS, (electrochemical studies)
EIS measurement is used in this study to provide more information on the elementary steps that make up the overall electrochemical process (Citation73). The primary method for keeping track of the electrochemical changes occurring at the metal/interface is electrochemical impedance spectroscopy, or EIS. Gaining crucial understanding of the kinetics of electrode reactions and the characteristics of the involved surface is made possible by this technique (Citation27–72). Electrochemical impedance spectroscopy (EIS) is an accurate, fast, and a powerful tool for investigating corrosion and inhibitory mechanisms (80). Electrochemical impedance spectroscopy (EIS) is an accurate, fast, and a powerful tool for investigating corrosion and inhibitory mechanisms. Electrochemical impedance spectroscopy (EIS) with and without polymeric surfactant inhibitor was used to investigate corrosion inhibition at various concentrations of nonionic surfactant inhibitor (PETTEDAA -Oli). Electrical resistance of the steel electrode immersed in 3.5% sodium chloride corrosive solution was measured using different concentrations(50,100, 150, and 200 ppm) from the amide-based surfactant compound (PETTEDAA -Oli) the obtained EIS data are tabulated and discussed. displays the results for the impedance diagram characteristic parameters. Rct, a measure of electron transmission across a contact, is found to be inversely proportional to the corrosion rate. The semicircle fitting method was used to calculate the electrochemical impedance parameters. A single time constant is represented as a perfect semicircle in Nyquist plots. Impedance tests reveal surface resistance and capacitance, as well as a substance's ability to inhibit corrosion and the nature of the inhibition process. Deviations from perfect circular shapes are attributed to interfacial impedance frequency dispersion, which is linked to surface non-homogeneity and metal roughness (Citation57, Citation63–68). Our investigation yielded a pattern that was not a perfect semicircle. This could be related to the addition of an inhibitor, which completely distracts the semicircle. Single semicircles are shifted in lockstep with the true impedance of the x-axis in the Nyquist plot (). The graphic depicts the impedance spectra with solitary, lowered semicircles located beneath the real axis. The transfer of charges had an impact on the corrosion process, according to this. These findings demonstrate the undesirable capacitive trend that characterizes the behavior of the solid-liquid interface in the field of electrochemistry. Additionally, the impedance (EIS) diagram's size increases in step with the concentration of surfactant inhibitor compound (PETTEDAA -Oli). Inhibition becomes more effective as a result of the inhibitor particles’ adsorption to the surface of the carbon steel. It ought to be noted that the reduced semicircles correspond to a clear departure from the expected behavior at the interface (Citation27–72). The impedances increased as the concentrations of inhibitor increased. In addition, the impedance profiles were consistent across all concentrations. Inhibitors can alter the process by slowing down the rate of corrosion. Furthermore, the rate-controlling mechanism can be determined to be a reaction-controlled mechanism due to inhibitor corrosion (Citation28, Citation71, Citation72). As inhibitor concentrations rise, Rct values rise. As a result, the charge transfer mechanism is in charge of the vast majority of corrosion responses (Citation73). As inhibitor concentrations rise, Rct values rise. As a result, the charge transfer mechanism is in charge of the vast majority of corrosion responses. Furthermore, an increase in inhibitor concentration results in a decrease in Cdl values due to the increased surface coverage of the inhibitor. This is because inhibitory efficiency has increased. The decrease in the Cdl value could be explained by a lower local dielectric constant and/or a thicker electric double layer on the carbon steel surface (Citation28, Citation71–73). The analysis of the electrochemical parameters presented in shows that the value of the pseudo capacity of the double layer (Cdl) increases with increasing surfactant inhibitor concentrations. On the other hand, it was also found that the charge transfer resistance (Rct) value increases with inhibitor concentration. The evolution of Cdl and Rct shows a decrease in the active surface of the metal, which can be related to the adsorption process of this compound onto a carbo steel surface forming a protective film (Citation73). The inhibition efficiency reaches a value of 94.5% at the 200 ppm concentration.
Figure 12. Nyquist plots for a carbon steel electrode in 3.5% NaCl solution at 30 °C with various concentrations of the prepared nonionic surfactant (PETTEDAA -Oli) inhibitor derived from plastic waste.
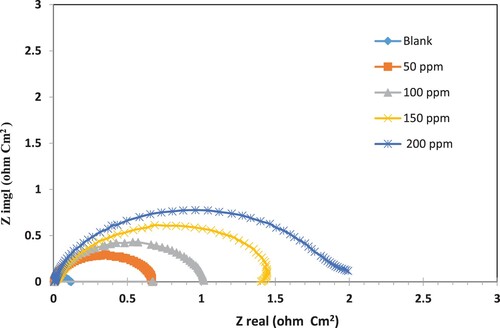
Table 8. Shows the electrochemical impedance spectroscopy (EIS) parameters of steel corrosion by3.5% NaCl at 303 k in the presence and absence of different concentrations from the prepared surfactant inhibitor (PETTEDAA -Oli).
3.9. Surface morphology studies using scanning electron microscope studies (SEM)
For evaluating the surface structure of carbon steel specimens flooded in 1M HCl either with or without an inhibitor compound. Scanning electron microscopy (SEM) imaging was used to study the surface morphology of the carbon steel samples. A narrowly focused electron beam is employed by the scanning electron microscope (SEM) to scan over the area of interest. The signals produced by the electrons’ interactions with the atoms that make up the sample (at or near its surface) convey information about the topography of the sample's surface (Citation70). ((b)) displays an SEM image of a carbon steel specimen's surface following immersion in a 3.5% NaCl solution for 48 hours. As carbon steel is significantly corroded as a result of metal dissolution during immersion in the corrosive medium without an inhibitor. It is evident that the entire surface of the metal is typically covered with products that formed from corrosion. Furthermore, the layer of porous corrosion protection cannot shield the surface from severe corrosion. (a) displays an SEM image of a different steel specimen following the same procedure in the presence of 200 ppm of inhibitors (PETTEDAA -Oli). A protective coating of inhibitor molecules forms on the steel surface, according to SEM measurements of the surface. At a concentration of 200 ppm of Inhibitor (PETTEDAA -Oli), the protective coating that is shown on the surface of steel ((a)) appears to be very smooth and covers the entire surface without any imperfections. This supports the previously reported high percentage inhibition efficiency (% I.E) of the utilized polymeric surfactants inhibitor chemical (PETTEDAA -Oli), which is generated from plastic waste.
3.10. Corrosion inhibition mechanism
The first stage of the inhibitory mechanism, which regulates corrosion, is the adsorption of the inhibitor molecules at the metal/solution contact (Citation15–18). At the metal/solution interaction, four different types of adsorption may take place when organic molecules are inhibited:
The electrostatic forces that draw charged molecules and metals together
The way the metal interacts with the molecule's unshared electron pairs.
Pi-electron interactions with metal, the third possibility;
and a combination of the first three.
The quantity of adsorption sites and their charge density, molecule size, the heat of hydrogenation, the mechanism of contact with the metal surface, and the generation of metallic complexes are a few variables that influence an inhibitor's ability to inhibit (Citation73). Physical adsorption requires the presence of charged species in the bulk of the solution and a metal with a vacant low-energy electron orbital, an inhibitor with molecules that are extremely loosely connected to electrons, or a heteroatom with lone pair electrons. Chemical interactions coexist with electrical connections as well. Chemical contact was started by non-bonding oxygen, nitrogen, and benzene ring electrons (Citation28, Citation71–73). The current effort will provide a concise description of the interaction of both amido amide inhibitor compounds and ester derivative inhibitor compounds with steel. Through an electrical contact between the unshared electron pairs and the steel material's d-orbital. The amide and ester functional group's heteroatoms are adsorbed on the surface. Furthermore, the alkyl chain is adsorbed on the material's surface via the orbitals of unsaturation from the aromatic nucleus. As a result, the molecule is found in the steel. To keep the medium from coming into touch with the steel, the alkyl chain is placed closer to the surface than the amido amide and ester compounds prepared from waste plastic. The combination of these two forms improves the capacity of amido amide produced from plastic waste to resist corrosion.
4. Conclusion
Solvent-free green recycling of PET plastic waste is an efficient recycling process for the synthesis of four nonionic polymeric surfactants with good yield in the presence of a catalyst. The four prepared nonionic polymeric surfactant materials act as a good corrosion inhibitor for steel substrate in a 3.5% NaCl marine corrosive environment. The protection of corrosion due to the adhesion and adsorption of polymeric materials on the steel surface which obey the Langmuir adsorption isotherm model. The inhibition efficiency in the case of amide-based inhibitor compounds (PETTEDAA -Oli and PETTEDAA) is more than glycol-based inhibitor compounds (PETTEG-Oli and PETTEG). The order of corrosion inhibition performance of the four prepared nonionic polymeric surfactants is PETTEDAA -Oli > PETTEDAA > PETTEG-Oli > PETTEG. The AAS data demonstrate that increasing inhibitor doses led to a decrease in ferric ion Fe2+ concentrations. Addition of inhibitor molecules to the aggressive medium produces a negative shift in the open circuit potential due to the retardation of the cathodic reaction. Potentiodynamic polarization data indicates the mixed-type nature of surfactant inhibitors. According to potentiodynamic polarization data, the prepared surfactant boosts polarization resistance and inhibition performance by adsorbing on the metal/electrolyte interface. Rct values increase as inhibitor concentrations rise. As a result, the charge transfer mechanism controls the majority of the corrosion response. Furthermore, an increase in inhibitor concentration leads to a decrease in Cdl values due to the inhibitor's increased surface coverage. This is due to an increase in inhibitory efficiency. A lower local dielectric constant and/or a thicker electric double layer on the carbon steel surface could explain the decrease in Cdl value. The reduction in the reaction number and time delay (Δtm) of the four studied polymeric non-ionic surfactant inhibitor materials increases in the case of amide-based inhibitors than in glycol-based inhibitors. It was found in the following order PETTEDAA -Oli > PETTEDAA > PETTEGOli > PETTEG. The data of different studied techniques are in good agreement with each other to prove the good protection efficiency of the four prepared nonionic polymeric surfactants towards the steel surface. SEM observations of the steel surface revealed the formation of a protective coating film of inhibitor molecules on the steel surface. The protective coating film formed on the steel surface appears to be very smooth and covers the whole surface without any flaws. This confirms the observed high percentage inhibition efficiency (% I.E) of the used polymeric nonionic surfactant inhibitor compound (PETTEDAA -Oli) derived from PET plastic waste via a green recycling process.
Supplementary_Figures_clean.docx
Download MS Word (4.9 MB)Acknowledgments
This research has been funded by Deputy for Research & Innovation, Ministry of Education through Initiative of Institutional Funding at University of Ha'il- Saudi Arabia through project number IFP-22119. 'The authors extend their appreciation to the Deputyship for Research& Innovation, Ministry of Education in Saudi Arabia for funding this research work through project number IFP-22119'.
Disclosure statement
No potential conflict of interest was reported by the author(s).
References
- Abdel Hameed, R.S. Solvent Free Glycolysis of Plastic Waste as Green Corrosion Inhibitor for Carbon Steel in Sulfuric Acid. J. New Mater. Electrochem. Syst. 2017, 20, 141–149. DOI: 10.14447/jnmes.v20i3.272.
- Abdel Hameed, R.S.; Al Shafey, H.; Soliman, S.A.; Metwaly, M.S. Corrosion of C-Steel Alloy in (0.1M) Nitric Acid in the Presence of Plastic Waste as Corrosion Inhibitors. Al-Azhar Bull. Sci. 2008, 19 (1), 51–61.
- Said, R.A.H.A. Plastic Waste Recycling as Green Corrosion Inhibitors for Steel in a Variety of Corrosive Media. In Advanced Aspects of Engineering Research, Vol. 14, 2021; pp 112–125. DOI: 10.9734/bpi/aaer/v14/6673D. Published: 2021-05-20.
- Abdel Hameed, R.S. Recycling of Plastic Waste as Green Corrosion Inhibitors for Steel in Different Corrosive Media. Mater. Sci. J. 2016, 14 (12), 503–509.
- Abdel Hameed, R.S.; Qureshi, M.T.; Al-Bonayan, A.M.; Al-Mhyawi, S.R.; Abd El-Kader, M.F.H. Corrosion Inhibition of Steel in Marine Environment Using Amino Amide Derived from pet Plastic Waste. J. Optoelectron. Biomed. Mater. 2021, 13 (1), 1–10.
- Abdel Hameed, R.S.; Elmetery, N.S.; Alshemary, N.F.; Shehata, H.A. Recycling of Some Plastic Waste as Green Corrosion Inhibitors for Steel in 1 M Phosphoric Acid. Mater. Sci. J. 2016, 14 (11), 417–4250.
- Abdel Hameed, R.S.; Qureshi, M.T.; Abdallah, M. Application of Solid Waste for Corrosion Inhibition of Steel in Different Media- A Review. Int. J. Corros. Scale Inhib. 2021, 10 (6), 68–79. DOI: 10.17675/2305-6894-2021-10-1-4.
- Abdel Hameed, R.; Abd El-Kader, M.F.H.; Qureshi, M.T.; Al Elaimi, M.; Farghaly, O.A. Green Synthesis for Nonionic Surfactants from Poly(Ethylene Terephthalate) Plastic Waste. Egypt. J. Chem. 2021, 64 (2), 773–780. DOI: 10.21608/EJCHEM.2020.45554.2928.
- Abdel Hameed, R.S.; Al Shafey, H.I.; Ismail, E.A. Studies on Corrosion Inhibition of C- Steel in 1M Acetic Acid Solutions by Ethoxylated Poly(Ethylene Terphthalate) Derived from Plastic Waste. Al-Azhar Bull. Sci. 2009, 20 (1), 185–197.
- Abdel Hameed, R.S.; Alshafy, H.I.; Farghaly, O. Corrosion of Mild Steel in NaCl Solutions and Effect of Recycled Plastic Waste Inhibitors. Res. Rev. Electrochem. 2012, 3 (2), 41–49.
- Shehata, H.A.; Abdelbary, H.M.; Soliman, S.A.; Salem, A.M.; Atta, A.M.; Abdel Hameed, R.S. Evaluation of Nonionic Surfactants from Plastic Waste as Corrosion Inhibitors of Carbon Steel in 1M HCl. J. Mater. Sci. 2012, 8 (7), 289–302.
- Abdallah, M.; Al-Abdali, F.H.; Kamar, E.M.; El-Sayed, R.; Abdel Hameed, R.S. Corrosion Inhibition of Aluminum in 1.0M H2SO4 Solution by Some Nonionic Surfactant Compounds Containing Five Membered Heterocyclic Moiety. Chem. Data Coll. 2020, 28, 100407. DOI: 10.1016/j.cdc.2020.100407.
- Abdel Hameed, R.S.; Ismail, O.M.; Eissa, F.M.; Ghanem, R. New Non Ionic Polymeric Surfactants as Corrosion Inhibitors for the C- Steel Alloy in Hydrochloric Acid Corrosive Medium. Der Chemica Sinica 2012, 3 (1), 236–248.
- Abdel Hameed, R.S.; Al Elaimi, M.; Qureshi, M.T.; Nassar, A.; Abd El-Kader, M.F.H.; Aljohani, M.; Arafa, E.I. Green Recycling of Poly (Ethylene Terephthalate) Waste as Corrosion Inhibitor for Steel in Marine Environment. Egypt. J. Chem. 2021, 64 (5), 2685–2695. DOI: 10.21608/EJCHEM.2021.54262.3145.
- Abdel Hameed, R.S. Schiff’ Bases as Corrosion Inhibitor for Aluminum Alloy in Sulfuric Acid Medium. Tenside Surfactants Deterg. 2019, 56 (3), 209–215. DOI: 10.3139/113.110622.
- Abdel Hameed, R.S. Cationic Surfactant- Zn+2 System as Mixed Corrosion Inhibitors for Carbon Steel in Sodium Chloride Corrosive Medium. Port. Electrochim. Acta 2018, 36 (4), 271–283. DOI: 10.4152/pea.201804271.
- Abdel Hameed, R.S. Aminolysis of Polyethylene Terephthalate Waste as Corrosion Inhibitor for Carbon Steel in H2SO4 Corrosive Medium. Adv. Appl. Sci. Res. 2011, 2 (3), 483–499.
- Abdelhameed, R.S. Recycling of the Used Cooking Oils as Corrosion Inhibitors for Carbon Steel in Sulfuric Acid Corrosive Medium. Adv. Appl. Sci. Res. 2016, 7 (2), 92–102.
- Tantawy, A.H.; Soliman, K.A.; Abd El-Lateef, H.M. Novel Synthesized Cationic Surfactants Based on Natural Piper Nigrum as Sustainable-Green Inhibitors for Steel Pipeline Corrosion in CO2-3.5%NaCl: DFT, Monte Carlo Simulations and Experimental Approaches. J. Clean. Prod. 2020, 250, 119510. DOI: 10.1016/j.jclepro.2019.119510.
- Abd El-Lateef, H.M.; Abo-Riya, M.A.; Tantawy, A.H. Empirical and Quantum Chemical Studies on the Corrosion Inhibition Performance of Some Novel Synthesized Cationic Gemini Surfactants on Carbon Steel Pipelines in Acid Pickling Processes. Corros. Sci. 2016, 108, 94–110. DOI: 10.1016/j.corsci.2016.03.004.
- Abd El-Lateef, H.M.; Tantawy, A.H. Synthesis and Evaluation of Novel Series of Schiff Base Cationic Surfactants as Corrosion Inhibitors for Carbon Steel in Acidic/Chloride Media: Experimental and Theoretical Investigations. RSC Adv. 2016, 6 (11), 8681–8700. DOI: 10.1039/C5RA21626E.
- Ibrahim, M.M.; Abdel Hameed, R.S.; Abu-Nawwas, A.-A.H.; Mohamad, S.E. Schiff’s Bases and Their Metal Complexes as Corrosion Inhibitors for Aluminum Alloys in Corrosive Media. J. Org. Chem. 2014, 10 (7), 271–281.
- Abdel Hameed, R.S.; Abdallah, M. Corrosion Inhibition of Carbon Steel in 1M Sulfuric Acid Using Some Pyrazolo[3,4-d]Pyrimidnone Derivatives. Prot. Met. Phys. Chem. Surf. 2018, 54 (1), 113–121. DOI: 10.1134/S2070205118010239.
- Abdel Hameed, R.S.; Shamroukh, A.H. Synthesis, Characterization, and Evaluation of Some Acyclic S-Nucleosides of Pyrazolo[3,4-d] Pyrimidine-Thiones as Corrosion Inhibitors for Carbon Steel in Sulfuric Acid. Int. J. Corros. Scale Inhib. 2017, 6 (3), 333–348. DOI: 10.17675/2305-6894-2017-6-3.
- Abdel Hameed, R.S.; Al-Shafey, H.I.; Abul Magd, A.S.; Shehata, H.A. Pyrazole Derivatives as Corrosion Inhibitor for C-Steel in Sulfuric Acid Medium. J. Mater. Environ. Sci. 2012, 3 (2), 294–305.
- Abdel Hameed, R.S.; Essa, A.; Mohamed, D.; Abdallah, M.; Aljohani, M.; Al-Mhyawi, S.; Soluman, M.; Arafa, E.I. Evaluation of Prepared Augmentine Drugs as Corrosion Inhiitor for Carbon Steel Alloy in 1.0 N HCl Acidic Environment Using Analytical Techniques. Egypt. J. Chem. 2022, 65 (4), 735–745. DOI: 10.21608/EJCHEM.2021.95853.4495.
- Alfa keer, M.; Al-Habal, T.; El-Sayed, R.; Abdel-Hameed, R.; Abdallah, M. Insight into the Corrosion Inhibition and Adsorption Behavior of Synthetic Pyrazole Surfactants as an Efficient Inhibitor for Carbon Steel in 1.0 mol/L HCl Solutions. Int. J. Corros. Scale Inhib. 2023, 12 (4), 2024–2045. DOI: 10.17675/2305-6894-2023-12-4-31.
- Abdallah, M.; El Guesmi, N.; Al-Gorair, A.S.; El-Sayed, R.; Meshabi, A.; Sobhi, M. Enhancing the Anticorrosion Performance of Mild Steel in Sulfuric Acid Using Synthetic Non-Ionic Surfactants: Practical and Theoretical Studies. Green Chem. Lett. Rev. 2021, 14 (2), 382–394. DOI: 10.1080/17518253.2021.1921858.
- Abdel Hameed, R.S.; Al-bonayan, A.M. Recycling of Some Water Soluble Drugs for Corrosion Inhibition of Steel Materials: Analytical and Electrochemical Measurements. J. Optoelectron. Biomed. Mater. 2021, 13 (2), 45–55. https://chalcogen.ro/45_HameedRSA.pdf.
- Abdel Hameed, R.S.; Aljuhani, E.H.; Felaly, R.; Munshi, A.M. Effect of Prepared Paracetamol–Zn+2 System and Its Synergistic Effect Towards Iron Dissolution Inhibition and Green Inhibition Performance. J. Adhes. Sci. Technol. 2020, 34 (19), 27–43. DOI: 10.1080/01694243.2020.1826801.
- Abdel Hameed, R.S.; Mohamed, D.; Khaled, A.; Abdallah, M.; Aljohani, M.M.; Al-Mhyawi, S.R.; Soliman, M.S. Application of Prepared Co-Amoxiclav Medicinal Drugs for Corrosion Inhibition of Steel Alloy Used in Petroleum Industry in Acidic Environment. Int. J. Corros. Scale Inhib. 2021, 10 (2), 714–731. DOI: 10.17675/2305-6894-2021-10-2-15.
- Abdel Hameed, R.S.; Qureshi, M.T.; Mohamed, D.; Abdallah, M.; Aljohani, M.M.; Al-Mhyawi, S.R.; Aljuhani, E.H.; Soliman, M.S. Recycling and Application of Prepared Desloratadine Medicinal Drugs for Inhibition of Steel Corrosion in Acidic Environment: Analytical Studies. Int. J. Corros. Scale Inhib. 2021, 10 (4), 1748–1765. DOI: 10.17675/2305-6894-2021-10-4-24.
- Abdel Hameed, R.S.; Al-Bagawi, A.H.; Shehata, H.A.; Shamroukh, A.H.; Abdallah, M. Corrosion Inhibition and Adsorption Properties of Some Heterocyclic Derivatives on C- Steel Surface in HCl. J. Bio- Tribo Corros. 2020, 51 (6), 1–11. DOI: 10.1007/s40735-020-00345-y.
- Abdel Hameed, R.S.; Aleid, G.M.S.; Khaled, A.; Mohammad, D.; Aljuhani, E.H.; Al-Mhyawi, S.R.; Alshammary, F.; Abdallah, M. Expired Dulcolax Drug as Corrosion Inhibitor for Low Carbon Steel in Acidic Environment. Int. J. Electrochem. Sci. 2022, 17 (5), 220655. DOI: 10.20964/2022.06.69.
- Abdallah, M.; Alfakeer, M.; Alshareef, M.; Hawsawi, H.; Al-Juaid, S.S.; Abdel Hameed, R.S.; Sobhi, M. Natural Sweet Almond Oil as an Effective Green Inhibitor for Aluminum Corrosion in Sulfuric Acid Medium. Int. J. Electrochem. Sci. 2022, 17, 220949.
- Abdel Hameed, R.S.; Obeidat, S.; Qureshi, M.T.; Al-Mhyawi, S.R.; Aljuhani, E.H.; Abdallahd, M. Silver Nanoparticles – Expired Medicinal Drugs Waste Accumulated at Hail City for the Local Manufacturing of Green Corrosion Inhibitor System for Steel in Acidic Environment. J. Mater. Res. Technol. 2022, 21, 2743–2756. DOI: 10.1016/j.jmrt.2022.10.081.
- Abdel-Hameed, R.; Qureshi, M.T.; Abdallah, M.; Aljuhani, E.; Alzharani, A.A.; Alfarsi, A.; Bakry, A.M.; Huwaimel, B.; Farghaly, O. Recycling of Expired Lactulose Drugs as Eco-Friendly Corrosion Inhibitor for Steel Alloys in Acidic Environment: Gravimetrical and Electrochemical Studies. Int. J. Electrochem. Sci. 2022, 17, 1–20.
- Abdel-Hameed, R.; Qureshi, M.T.; Abdallah, M.; Aljuhani, E.; Alzharani, A.A.; Alfarsi, A.; Bakry, A.M.; Huwaimel, B.; Farghaly, O. Recycling of Expired Lactulose Drugs as Eco-Friendly Corrosion Inhibitor for Steel Alloys in Acidic Environment: Gravimetrical and Electrochemical Studies. Int. J. Electrochem. Sci. 2022, 17, 221270. DOI: 10.20964/2022.12.92.
- Abdel Hameed, R.S.; Essa, A.; Nassar, A.; Badr, M.; Huwaimel, B.; Al-Mhyawi, S.R.; Alshammary, F.; Seni, A.A.; Abdallah, M. Chemical and Electrochemical Studies on Expired Lioresal Drugs as Corrosion Inhibitors for Carbon Steel in Sulfuric Acid. J. New Mater. Electrochem. Syst. 2022, 25 (4), 268–276. DOI: 10.14447/jnmes.v25i4.a07.
- Abdel-Hameed, R.; Al Elaimi, M.; Qureshi, M.T.; Aleid, G.M.; Al-Shafey, H.I.; Alshammary, F.; Huwaimel, B.; Aljuhani, E.H.; Al-bonayan, A.M.; Al-Mhyaw, S. Manufacturing of Corrosion Inhibitors and Flow Improvers from Recycling of Waste at Hail City, Saudi Arabia: Physicochemical and Electrochemical Studies on Steel in Petroleum Industries. ACS Omega 2023, 8 (27), 24279–24290. DOI: 10.1021/acsomega.3c01339.
- Abdel-Hameed, R.; Aleid, G.M.S.; Ragab, H.; Alshafey, H.; Alosaimi, A.M. Synthesis, Characterization, and Evaluation of Polymeric Surfactants Derived from PET Plastic Waste as Green Corrosion Inhibitor of Steel Surfaces in Marine Environment for Heavy Industry. RSC Adv. 2023, 13 (45), 31969–31988. https://pubs.rsc.org/en/content/authorreprints.
- Ibrahim, M.M.; Abdel Hameed, R.S.; Abu-Nawwas, A.-A.H. Schiff Bases and Their Metal Complexes as Corrosion Inhibitors in Acidic Media. J. Org. Chem. 2013, 12 (9), 493–499.
- Abdel Hameed, R.S. Prepared Ranitidine Drugs as Corrosion Inhibitor for Aluminum in 1M Sulfuric Acid. Al-Azhar Bull. Sci. 2009, 20 (1), 151–163. DIO: 10.2160/8ABSB.2009.7553.
- Abdel Hameed, R.S. Ranitidine Drugs as Non-Toxic Corrosion Inhibitor for Mild Steel in Sulfuric Acid Medium. Port. Electrochim. Acta 2011, 29 (4), 273–285. DOI: 10.4152/pea.201104273">10.4152/pea.201104273.
- Abdel-Hameed, R.S. Prepared Drugs as Corrosion Inhibitors for Metals and Alloys. Phys. Chem.: Indian J. 2013, 8 (4), 146–149.
- Vaszilcsin, N.; Duca, D.-A.; Flueraş, A.; DANa, M.-L. Prepared Drugs as Inhibitors in Electrochemical Processes–A Mini-Review. Stud. Univ. Babes-Bolyai, Chemia 2019, 64 (3), 17–32. DOI: 10.24193/subbchem.2019.3.02.
- Chauhan, D.S.; Sorour, A.A.; Quraishi, M.A. An Overview of Prepared Drugs as Novel Corrosion Inhibitors for Metals and Alloys. Int. J. Chem. Pharm. Sci. 2016, 4 (1), 680–698.
- Vaszilcsin, N.; Ordodi, V.; Borza, A. Corrosion Inhibitors from Prepared Drugs. Int. J. Pharm. 2012, 15 (1), 241–253.
- Abdel Hameed, R.S.; Al Shafey, H.I.; Abu-Nawwas, A.H. 2-(2, 6-Dichloranilino) Phenylacetic Acid Drugs as Eco-Friendly Corrosion Inhibitors for Mild Steel in 1M H2SO4. Int. J. Electrochem. Sci. 2014, 9 (1), 6006–6019.
- Alshafey, H.I.; Abdel Hameed, R.S.; Ali, F.A.; Aboul-Magd, A.E.-A.S.; Salah, M. Int. J. Pharm. Sci. Rev. Res. 2014, 27 (1), 146–159.
- Haque, J.; Verma, C.; Srivastava, V.; Wan Nik, W.B. Corrosion Inhibition of Mild Steel in 1M HCl Using Environmentally Benign Thevetia Peruviana Flower Extracts. Sustain. Chem. Pharm. 2021, 19 (4), 100354. DOI: 10.1016/j.scp.2020.100354.
- Abdel Hameed, R.S.; Al Shafey, H.I.; Abu-Nawwas, A.H. Prepared Voltaren Drugs as Corrosion Inhibitor for Aluminium in Sulfuric Acid. Int. J. Electrochem. Sci. 2015, 10 (1), 2098–2109. http://www.electrochemsci.org/papers/vol10/100302098.pdf.
- Kadhim, A.; Al-Amiery, A.A.; Alazawi, R.; Al-Ghezi, M.K.S.; Abass, R.H. Corrosion Inhibitors. A Review. Int. J. Corros. Scale Inhib. 2021, 10 (1), 54–67. DOI: 10.17675/2305-6894-2021-10-1-3.
- Gupta, N.K.; Gopal, C.S.A.; Srivastava, V.; Quraishi, M.A. Application of Prepared Drugs in Corrosion Inhibition of Mild Steel. Int. J. Pharm. Chem. Anal. 2017, 4 (8), 8–12. DOI: 10.18231/2394-2797.2017.0003.
- Fouda, A.S.; El-Morsy, M.A.; El-Barbary, A.A.; Lamloum, L.E. Study on Corrosion Inhibition Efficiency of Some Quinazoline Derivatives on Stainless Steel 304 in Hydrochloric Acid Solutions. Int. J. Corros. Scale Inhib. 2016, 5 (2), 112–131. DOI: 10.17675/2305-6894-2016-5-2-2.
- Singh, A.K.; Chugh, B.; Saha, S.K.; Banerjee, P.; Ebenso, E.E.; Thakur, S.; Pani, B. Evaluation of Anti-Corrosion Performance of an Expired Semi Synthetic Antibiotic cefdinir for Mild Steel in 1 M HCl Medium: An Experimental and Theoretical Study. Results Phys. 2019, 14 (1), 102383.
- Abdallah, M.; Fawzy, A.; Al Bahir, A. The Effect of Prepared Acyclovir and Omeprazole Drugs on the Inhibition of Sabic Iron Corrosion in H2SO4 Solution. Int. J. Electrochem. Sci. 2020, 15, 1–16.
- Abdel Hameed, R.S.; Ismail, E.A.; Al-Shafey, H.I.; Abbas, M.A. Prepared Indomethacin Therapeutics as Corrosion Inhibitors for Carbon Steel in 1.0 M Sulfuric Acid Media. J. Bio Tribo Corros. 2020, 6 (1), 114. DOI: 10.1007/s40735-020-00403-5.
- Abdel Hameed, R.S.; Aljohani, M.M.; Essa, A.B.; Khaled, A.; Nassar, A.M.; Badr, M.M.; Al-Mhyawi, S.R.; Soliman, M.S. Electrochemical Techniques for Evaluation of Prepared Megavit Drugs as Corrosion Inhibitor for Steel in Hydrochloric Acid. Int. J. Electrochem. Sci. 2021, 16 (1), 1–13. DOI: 10.20964/2021.04.15.
- Abdallah, M.; Hawsawi, H.; Al-Gorair, A.S.; Alotaibi, M.T.; Al-Juaid, S.S.; Abdel Hameed, R.S. Appraisal of Adsorption and Inhibition Effect of Prepared Micardis Drug on Aluminum Corrosion in Hydrochloric Acid Solution. Int. J. Electrochem. Sci. 2022, 17 (5), 220462. DOI: 10.20964/2022.04.61.
- Baari, M.J.; Sabandar, C.W. A Review on Prepared Drug-Based Corrosion Inhibitors: Chemical Composition, Structural Effects, Inhibition Mechanism, Current Challenges, and Future Prospects. Indones. J. Chem. 2021, 21 (5), 1316–1336. DOI: 10.22146/ijc.64048.
- Abdel Hameed, R.S.; Abdallah, M. Inhibiting Properties of Some Heterocyclic Amide Derivatives as Potential Nontoxic Corrosion Inhibitors for Carbon Steel in 1.0 M Sulfuric Acid. Surf. Eng. Appl. Electrochem. 2018, 54 (6), 599–606. DOI: 10.3103/S1068375518060054.
- Abdel Hameed, R.S.; Al-Shafey, H.I.; Abul Magd, A.S.; Shehata, H.A. Thiosemicarbazone Derivatives as Corrosion Inhibitor for C- Steel in HCl Corrosive Medium. Mater. Sci. 2012, 8 (5), 213–220.
- Abdel Hameed, R.S.; Abu-Nawwas, A.-A.H.; Shehata, H.A. Nano-Composite as Corrosion Inhibitors for Steel Alloys in Different Corrosive Media: Review Article. Adv. Appl. Sci. Res. 2013, 4 (3), 126–129.
- Abdel Hameed, R.S.; Aljuhani, E.H.; Al-Bagawi, A.H.; Shamroukh, A.H.; Abdallah, M. Study of Sulfanyl Pyridazine Derivatives as Efficient Corrosion Inhibitors for Carbon Steel in 1.0 M H2SO4 Using Analytical Techniques. Int. J. Corros. Scale Inhib. 2020, 9 (2), 623–643. DOI: 10.17675/2305-6894-2020-9-2-16.
- Abdallah, M.; Al-Gorair, A.S.; Fawzy, A.; Hawsawi, H.; Abdel Hameed, R.S. Enhancement of Adsorption and Anticorrosion Performance of Two Polymeric Compounds for the Corrosion of SABIC Carbon Steel in Hydrochloric Acid. J. Adhes. Sci. Technol. 2021, 36 (1), 35–53. DOI: 10.1080/01694243.2021.1907041.
- Al-Gorair, A.S.; Hawsawi, H.; Fawzy, A.; Sobhi, M.; Alharbi, A.; Abdel Hameed, R.S.; Abd El Wanees, S.; Abdallah, M. Evaluation of the Anticorrosion and Adsorption Properties of Polyethylene Glycol and Polyvinyl Alcohol for Corrosion of Iron in 1.0 M NaCl Solution. Int. J. Electrochem. Sci. 2021, 16 (4), 211119. DOI: 10.20964/2021.11.03.
- Alfakeera, M.; Abdallah, M.; Abdel Hameed, R.S. Propoxylated Fatty Esters as Safe Inhibitors for Corrosion of Zinc in Sulfuric Acid. Prot Met. Phys.Chem. Surf. 2020, 56 (1), 225–232. DOI: 10.1134/S2070205120010025.
- Atta, A.M.; Al-Hodan, H.A.; Abdel Hameed, R.S. Preparation of Green Cardanol-Based Epoxy and Hardener as Primer Coatings for Petroleum and Gas Steel in Marine Environment. Prog. Org. Coat. 2017, 111 (1), 283–293. DOI: 10.1016/j.porgcoat.2017.06.002.
- Abdel Hameed, R.S.; El-Zomrawy, A.; Abdallah, M.; Abed El Rehim, S.S.; AlShafey, H.I.; Nour Edin, S. Polyoxyethylene Stearate of Molecular Weight 6000 as Corrosion Inhibitor for Mild Steel in 2.0 M Sulphuric Acid. Int. J. Corros. Scale Inhib. 2017, 6 (2), 196–208. DOI: 10.17675/2305-6894-2017-6-2-8.
- Abdallah, M.; Soliman, K.A.; Alfakeer, M.; Al-bonayan, A.M.; Alotaibi, M.T.; Hawsawie, H.; Hazazia, O.A.; Abdel Hameed, R.S.; Sobhib, M. Mitigation Effect of Natural Lettuce Oil on the Corrosion of Mild Steel in Sulfuric Acid Solution: Chemical, Electrochemical, Computational Aspects. Green Chem. Lett. Rev. 2023, 16, 1. DOI: 10.1080/17518253.2023.2249019.
- Abdel Hameed, R.S.; Aleid, G.M.S.; Mohammad, D.; Badr, M.M.; Huwaimel, B.; Suliman, M.S.; Alshammary, F.; Abdallah, M. Spinacia Oleracea Extract as Green Corrosion Inhibitor for Carbon Steel in Hydrochloric Acid Solution. Int. J. Electrochem. Sci. 2022, 17, 221017. DOI: 10.20964/2022.10.31.
- Sabet, M.; Tanreh, S.; Khosravi, A.; Astaraki, M.; Rezvani, M.; Ganji, M.D. Theoretical Assessment of the Solvent Effect on the Functionalization of Au32 and C60 Nanocages with Fluorouracil Drug. Diam. Relat. Mater. 2022, 126 (6), 109142. DOI: 10.1016/j.diamond.2022.109142.