ABSTRACT
In 2015, the United Nations created a framework comprising 17 aspirational goals known as the Sustainable Development Goals (SDGs), with a view toward peace and prosperity by 2030. Green Chemistry (GC) has always been recognized as a pathway to sustainability. However, the best solutions cannot be found if we look at each of these separately, but rather it is important to apply a systems thinking approach and recognize the interconnected nature of the systems being studied. In this paper, we present activities that allow learners to visualize synergies and trade-offs with the SDGs when applying a systems thinking approach compared with a narrower perspective. We applied the approach to an industrial example (Nike) and to concerning chemicals such as bisphenols and per-and polyfluoroalkyl substances (PFAS or ‘forever chemicals’). We believe this approach can be modified and used widely for various chemical processes in an educational context.
Introduction
The Brundtland Report, released in 1987, defined Sustainable Development as ‘meeting the needs of the present without compromising the ability of future generations to meet their own needs’ (Citation1). In September 2000 the United Nations (UN) put out the Millennium Development Goals (MDGs). It consisted mainly of 8 time-bound development goals supported by 18 targets and 60 indicators. In 2015 all United Nations member nations agreed on a global mission to create a sustainable future. At that time, the 17 UN Sustainable Development Goals (SDGs) were adopted (). The 2030 Agenda for Sustainable Development lists these goals in detail. The United Nations members state these goals as ‘an urgent call for action by all countries – developed and developing – in a global partnership’ (Citation2, Citation3). Due to the interconnectedness of the SDGs, the UN recognized that true progress can only be achieved if we are able to promote situations where multiple goals strengthen one another (synergies) and reduce situations where goals hinder one another (trade-offs) (Citation4, Citation5).
Figure 1. The 17 UN Sustainable Development Goals adopted in 2015. ‘The content of this publication has not been approved by the United Nations and does not reflect the views of the United Nations or its officials or Member States.’
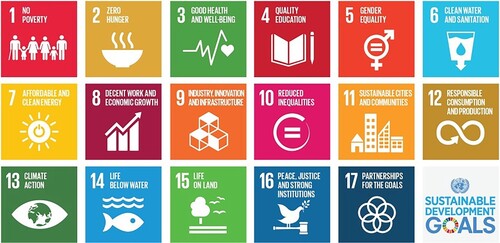
Green Chemistry is defined as ‘the utilization of a set of principles that reduces or eliminates the use or generation of hazardous substances in the design, manufacture and application of chemical products’ (Citation6) and has been described as pollution prevention at the molecular level. Because green chemistry is not related to a specific discipline in chemistry, any chemist can use the twelve principles associated with it to design molecular processes. The twelve principles provide a framework to assess whether a process is benign and can also show areas for progress. Green chemistry can reduce pollution at its source rather than requiring remediation at the end of a process (Citation7,Citation8).
Chemicals, and chemical processes, are clearly an integral part of achieving the SDGs (Citation5, Citation9). However, due to the complexity of these interconnected systems, it would be simplistic to create a list of places where green chemistry and the UN SDGs intersect and expect meaningful progress to follow (Citation9). For example, fertilizers are key to achieving two of the SDGs: #1, No Poverty and #3, Zero Hunger. Despite their promise of achieving these goals, they are also major contributors to climate change and produce several downstream water quality issues (Citation10). Even though a chemical solution addressed an SDG effectively, it did not ensure sustainable development. By focusing only on chemical solutions, we run the risk of introducing unintended consequences through regrettable substitutions (Citation11–13). For holistic sustainable solutions, we must consider factors beyond chemistry; we must explore the interconnected nature of the SDGs and Green Chemistry and Sustainability. ‘In order for the potential of using chemistry to achieve SDGs, there are challenges that must be addressed within the chemical enterprise and within the society that this enterprise impacts’ (Citation9). We must therefore define ‘Systems Thinking.’
Instead of the traditional ‘silo’ approach to problem solving, systems thinking considers and emphasizes the interdependence of the complex challenges posed by dynamic systems (Citation14, Citation15). If we put chemistry into this context, we can move beyond isolated consideration of reactions. Questions about where materials come from and how they are transformed and used must be addressed. By examining the entire life cycle, we can also consider what happens to a product at the end of its usable life span. In addition, a systems thinking approach must consider the balance between the benefits and impacts of chemical substances and look at the role they play in society and the environment. Any solution should include an understanding of the economics involved and consider whether the solution proposed is equitable to all stakeholders, particularly within communities that have historically borne a higher burden of environmental harm. Collaboration across a variety of stakeholders is essential to address these complex issues and should be inclusive of race, ethnicity, class, gender and nationality. This is important because the same issue or solution is not necessarily viewed in the same way due to the lived experiences of individual stakeholders. A broader, systems-thinking approach will help show links between issues that might not be initially obvious (Citation9, Citation16–18).
Recently, there have been several examples of incorporation of systems thinking into the curriculum. These include a special issue on the incorporation of systems thinking into classes, a class that introduces systems thinking into the introductory chemistry curriculum level and a card game designed so students can learn about recycling processes through a systems approach. These approaches allow for effective learning in chemistry and highlight the role of chemistry and the development of partnerships in the creation of a sustainable society (Citation2, Citation19–25). However, a systems thinking approach, while beneficial, adds an additional level of complexity which requires further tools to aid student learning. Graphical tools such as a systems-oriented concept map are one way to tackle complexity (Citation26–28). If we want to truly tackle the American Chemical Society Green Chemistry Institute learning goals of ‘Students will be able to use life cycle thinking to reduce the environmental footprint of chemicals and design chemicals and materials for sustainability’ we need more educational tools and case studies to help students comprehend and utilize systems thinking in their decision making (Citation29). Herein, we present a hands-on educational tool that can be used alone or in combination with other approaches to specifically highlight synergies and tradeoffs when discussing chemical substitutions, green process development and life cycle analysis. We used the approach for three independent examples, which are described in detail below.
Overall, the students were asked to apply a systems approach and consider the examples using a broader lens that incorporated the Four E categories: Economics, Equity, Empowerment and the Environment (Citation9). By explicitly stating each of these four categories, students were able to understand the interactions of Green Chemistry and the UN SDGs as causes and consequences. Leveraging such interdependencies can offer integrated and complementary solutions, thus leading to truly sustainable solutions. These exercises are hands-on and done in small groups and have no absolute ‘correct’ answer, which can motivate students to discuss each case at length among themselves and consider each case in broader terms than if they were only examining a chemical solution. By using industry-related examples, we can train future employees to use a broad approach to solving complex chemical problems, such as those concerning bisphenols and the so-called ‘forever chemicals’ (per-and polyfluoroalkyl substances, PFAS). We believe this approach can be modified and used widely for various chemical processes in an educational context.
Format of activities
The activities described were tested in various fora ranging from a 4-day workshop for graduate students in Poznan, Poland, a 4-hour virtual seminar for graduate students in Thailand, a 2-hour seminar for industrial and government chemists in Thailand, and 2.5 hour seminars for two different high school groups in Thailand. We will describe the 4-day workshop activities as they provide the greatest representation of the concepts described in this paper. We mention the other formats and audiences to demonstrate the versatility of these activities.
Students in the 4-day workshop were pursuing Ph.D. studies at the Poznan University of Technology in Poznan, Poland and were familiar with the principles of green chemistry. The group consisted of a mix of chemistry, chemical technology and environmental engineering students. The aims of the workshop and activities described included:
Students are introduced to the concepts of Green Chemistry and the U.N. Sustainable Development Goals,
Students build the skills needed to adopt a systems-thinking approach, thus allowing them to look at the entire life cycle of products
Students learn how to recognize the interconnections between the complex systems being studied.
Students are able to assess the importance of the broader ‘Four E’ categories: Economics, Equity, Empowerment and the Environment in assessing the sustainability of a solution.
Students applied a systems thinking approach to industrial-related examples specifically to understand the interconnections between the various UNDP goals: synergies and tradeoffs.
Three of the activities are described below.
Example 1: Nike case study
Once the introductory material about the UN SDGs and Green Chemistry had been covered, students were placed into groups of 3–4 and given two sets of preprinted 2 × 2 inch cardboard tiles, with each tile representing a single UN SDG or a single Green Chemistry principle. They were also given a sheet of paper with three concentric circles drawn on it, which we are referring to as ‘Circles of Sustainability’ ().
Figure 2. UN SDG tiles and green chemistry principles tiles along with ‘concentric circles’ setup provided to students.
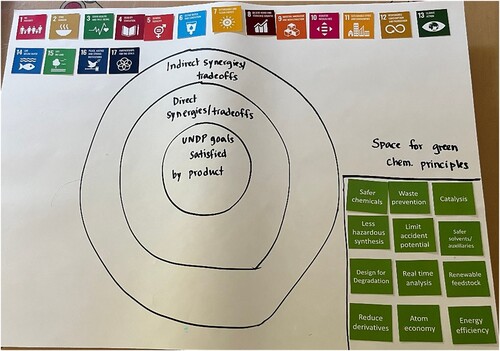
The first part of the exercise asked the students to consider Nike, a shoe company, and think about which (if any) UN SDGs could be met by a shoe company and to place those tiles in the center of the paper. They eventually came up with #3 (Good Health and Well-Being) and #9 (Industry and Infrastructure) being satisfied by Nike manufacturing shoes (). Prompts to keep things simple, particularly with an advanced group who want to immediately apply greener thinking, is important at this stage. Students need only to think broadly about how the manufacturing of shoes can satisfy any UN SDGs.
At this point, the lecture continued to discuss the robust Green Chemistry program that Nike has as part of its sustainability commitment and the effects that this program has had (Citation30); GREEN CHEMISTRY: CHEMICAL PRODUCTS & PROCESSES THAT REDUCE OR ELIMINATE THE USE OF HAZARDOUS SUBSTANCES.; Nike Restricted Substances List (RSL) and Sustainable Chemistry Guidance (SCG), (Citation31). Students were then asked to look through the Green Chemistry tiles and to link the lecture materials (Nike RSL database, the use of recycled fibers, use of wind energy, etc.) with the actual Green Chemistry Principles that were satisfied. Once they discussed the possible principles satisfied, they were then asked to choose additional UN SDGs that are directly satisfied by Nike’s use of Green Chemistry. These tiles were placed in the next circle and are Direct Links between Green Chemistry and the UN SDGs. These new tiles are #12 (Responsible Consumption and Production), #13 (Climate Action), #14 (Life Below Water) and #15 (Life on Land). shows the direct links between the use of green chemistry and the UN SDGs satisfied.
The students were then asked if it is enough to only consider Green Chemistry solutions. The lecture continued to introduce ‘systems thinking’ and how to solve complex problems by looking at the whole rather than the parts. They were introduced to the idea of expanding solutions to include Economics, Equity, Empowerment and the Environment. Nike is used as the corporate example for this workshop because it has a robust Green Chemistry program in addition to their support for women – and minority-owned businesses, girls in sports, support for women in the workforce, etc.
Once these topics were introduced, the students were then asked to apply any remaining tiles to expand the circle of sustainability by looking at topics beyond a simple Green Chemistry application (e.g. Nike’s efforts to include women in the workforce or their efforts at providing a fair wage to their workers). At this point, UN SDGs #1 (No Poverty), #5 (Gender Equality), #8 (Decent Work and Economic Growth), #10 (Reduced Inequalities), #11 (Sustainable Cities and Communities) and #17 (Partnerships for the Goals) can be satisfied. These tiles were placed in the outer circle, which indicates Indirect Links (). It was important at this time to discuss that Green Chemistry was not involved in satisfying the UN SDGs in the outer circle and the importance of the 4Es at this stage in widening the circle. The students were reminded that a systems thinking approach was important to satisfy more UN SDGs that would be satisfied by focusing purely on chemical solutions.
Figure 5. UN SDG goals satisfied by Nike after applying select green chemistry solutions + wider sustainability/ethical solutions.
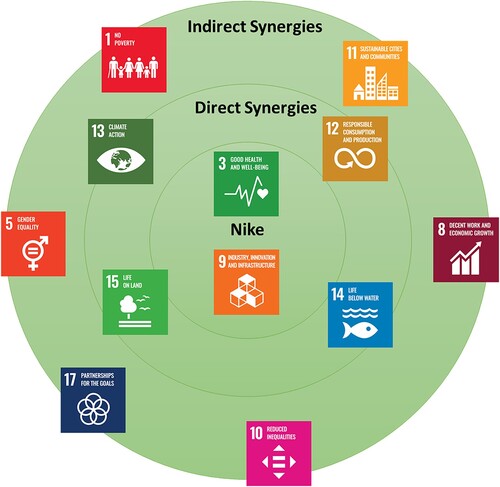
The students were given time to discuss the topics and to consider what each tile actually meant in the context of the exercise. It created considerable discussion within the groups! The goal was to create a case study that would train students to think about several different issues when faced with making complex decisions. Even though Green Chemistry is a framework designed to help scientists create more benign chemical processes, the exercise of expanding the circles of sustainability demonstrates that additional considerations are required to avoid making regrettable substitutions. The case study also illustrates that while it is hard for a large company to execute a complex sustainability strategy, it is necessary when it pays dividends in terms of profits. The use of physical tiles provides a play-like feel to the activity and provides tactile feedback that encourages the groups to stay engaged in discussions and complete the activity.
Instructors should allocate 2–3 hours for this case study depending on the level of audience. Having the students work on one case study is sufficient to teach the concept and is recommended for audiences with less chemistry experience. Presentations and keys for all the activities presented can be found in the supplemental information. The Nike case study is the most versatile of the three presented in this paper and with the brand being internationally recognizable, it is easy to get the entire audience immediately involved. The next two exercises show how we expanded this model to industrial examples to truly drive home the concepts of systems thinking and interdependencies.
Example 2: trade-offs of PFAS and alternatives
Per – and polyfluoroalkyl substances (PFAS) are widely used industrial chemicals that essentially never break down. They are inert and designed for durability, so not surprisingly they have been deemed ‘forever chemicals’ (Citation32). Due to their inert nature, they are difficult to destroy in incinerators and can leach from landfills into groundwater and soil. They can accumulate in blood, fish, food products and the environment and have different modes of action based on their structure. Ongoing studies worldwide suggest multiple health issues are possible with low-level exposure. Exposure can happen at manufacturing sites as well as further down the line when used in other products (Citation33–36). This example used the Circles of Sustainability model described in the previous section and applied it to PFAS use.
The lecture materials covered PFAS use in consumer, commercial and industrial products and discussed health impacts related to PFAS exposure. The students were asked to categorize many different consumer products into one of three categories: Non-essential, substitutable or essential. The 1987 Montreal Protocol for the reduction of ozone depleting chemicals provides a model for a way to effectively ‘triage’ chemicals into these categories. In 2019, a proposal based on the Montreal Protocol demonstrated a way to categorize PFAS chemicals into these same categories. Using this model, PFAS that are easier to replace or eliminate (PFAS in dental floss and eye mascara) vs. those that serve essential functions (certain medical devices, protective gear worn in the oil and gas industry) can be effectively categorized (Citation35).
Once the basic framework had been established, the students were provided with two sequential exercises to develop Circles of Sustainability related to PFAS and waterproof raincoats. Similar to the Nike exercise, the first question asked was to determine what UN SDGs were satisfied by having a waterproof raincoat (#3, Good Health and Well-Being). This tile was placed in the center circle. Unlike the Nike example, the students were then asked to think about conflicts instead of synergies. If this raincoat was made using PFAS, what were the direct conflicts with the UN SDGs? At this point, the students should realize that #3 is now in direct conflict due to having the raincoat made with PFAS. All of the direct conflicts are then placed in the second circle (#3, #6 Clean Water and Sanitation, #14 Life Below Water and #15 Life on Land). The outer circle is for indirect conflicts. These include #8 (Decent Work and Economic Growth), #10 (Reduced Inequalities, due to evidence that people of lower socioeconomic status are disproportionately impacted by environmental pollutants) (Citation37), #11 (Sustainable Cities and Communities), #12 (Responsible Consumption and Production) and #13 (Climate Action, due to the release of HCFC-22 in the production of PFAS) () (Citation38).
Figure 6. UN SDG goals satisfied by using a raincoat made with a PFAS alternative. There are no green principles used since chemical information was not easily available.
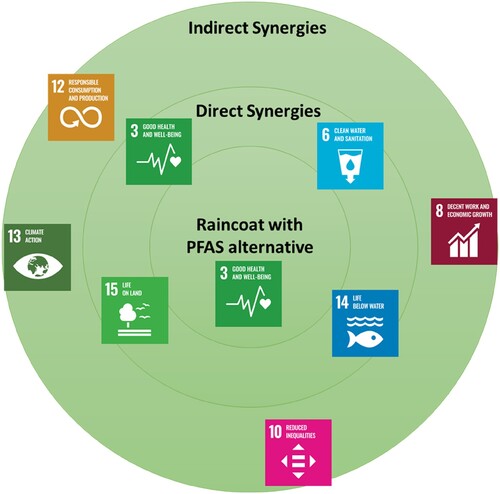
The second part of this activity was to think about a raincoat made from a PFAS alternative that has been evaluated for hazard (Citation39). The direct synergies were #3, #6, #13, #14 and #15. Indirect synergies were #8 (Decent Work and Economic Growth), #10 (Reduced Inequalities) and #12 (Responsible Consumption and Production). The indirect synergies included discussions about where the manufacturing is done, conditions for the workers, the gender distribution of the workers in these companies and what the supply chain looks like (transportation, local availability of feedstocks) (Citation37). In this example, we were unable to discuss any related green chemistry since we don’t know what the alternative is chemically or how it is manufactured due to intellectual property protections.
Depending on the level of participating students, the instructor may choose to focus on more of the chemistry of PFAS, such as the polarity and bond strengths of PFAS, environmental and bioaccumulation based on structure, lack of biodegradability and challenges of manufacture and disposal. The exercise can be tailored depending on the level of participating students.
Example 3: tradeoffs of Bisphenol-A and lessons to avoid ‘regrettable substitutions’
Bisphenol-A (BPA) is a chemical compound that has been used in the production of certain plastics and epoxy resins since the 1960s. It is commonly found in food and beverage containers, such as water bottles, food storage containers, and the lining of metal cans (Citation40). An introductory lecture to provide basic chemistry and context for this exercise was done prior to the student activity.
Once the introductory framework was provided, the students were specifically asked to focus only on epoxy resins used to line cans for food storage. Similar to previous examples, they were asked to think of the UN SDG goals satisfied by using an epoxy liner for canned food. The students were encouraged to think about the role of the liner in ensuring extended shelf life of canned products, which prevents risks associated with the development of life-threatening conditions such as botulism. At this point, the students easily came up with SDGs #2 (Zero Hunger) and #3 (Good Health and Well-Being) to place in the center of the rings.
The students were then introduced to the synthesis of a polycarbonate plastic and asked to place tiles on their papers for each of the green chemistry principles that were satisfied or not satisfied in the synthesis. They were also asked to reflect on the toxicity information for all the reactants/products and amend their answers. There are several resources that can be used to obtain toxicity information for BPA and this area can be vast and intimidating (Citation40–42). As an approachable review and primer for students as well as instructors Stephen Ritter’s article collection in the Chemical and Engineering News is recommended (Citation43). The lecture continued to introduce the fact that the BPA used in the synthesis of these resins can leach into food and beverages, and there has been concern about its potential health effects, specifically as an endocrine disruptor.
At this point, students are familiar with the Circles of Sustainability as described in previous exercises and were asked to use the same concept to generate direct and indirect conflicts between the use of BPA-lined cans and the UN SDGs. The direct conflicts were identified as BPA being detrimental to #3 (Good Health and Well-Being), #6 (Clean Water and Sanitation) #14 (Life Below Water) and #15 (Life on Land), due to the toxic effects of BPA. The less obvious direct conflict is that of #5, Gender Equality. While BPA can impact both genders, there are specific consequences of BPA exposure in women. These may manifest as obesity, recurrent miscarriages, and polycystic ovarian syndrome, among other problems, leading to the inclusion of SDG #5 – Gender Equality as a major direct conflict. The indirect conflicts were identified as BPA being detrimental to #13 (Climate action, due to production emissions), #11 (Sustainable cities and communities) #8 (Decent work and economic growth, due to specific effects on workers who are exposed to BPA during production) and #12 (Responsible consumption and production) and #10 (Reduced inequalities, reiterated again due to the gender inequities and general inequities in who bears the burden of toxic substances in society).
The students were taught that BPA was replaced by Bisphenol-S (BPS) and similar analogs, and a discussion about when a replacement was identified as perhaps even more problematic than the problem it sought to solve was conducted. The common term for this occurrence is ‘Regrettable Substitution.’ The chemicals that were introduced as BPA alternatives are structurally similar to BPA and share a common structure of two hydroxyphenyl functionalities and are collectively referred to as bisphenol analogs. Due to their being analogs, several of the substitutes share similar toxicological profile and are as likely to leach from linings as BPA, thus not reducing any of the conflicts previously identified (Citation44, Citation45).
In order to create a more sustainable solution, the students were introduced to Tetramethyl Bisphenol F (TMBPF), a new coating intended as a safer replacement for Bisphenol A (Citation46). TMBPF was developed to be used in epoxy linings of aluminum cans and steel cans. In the next part of the exercise, students were asked to make a green chemistry assessment and to consider the direct and indirect synergies resulting from the substitution of resins made using TMBPF vs. BPA. One of the ways in which TMBPF is different from BPA as a precursor for epoxy and/or polycarbonates is that TMBPF is not used in the final polymerization reaction, which means TMBPF is not detected in final products and thus the migration potential of TMBPF is reduced (Citation47–49). In the systems thinking approach, we can discuss protecting workers and communities from substances known to carry health risks which can lead to greater gender equity and decent work and economic growth (Citation41, Citation42). A full assessment of the direct and indirect synergies can be seen in .
Figure 7. UN SDG goals satisfied by using a can made with epoxy resin that is BPA free. The green principles used are also highlighted.
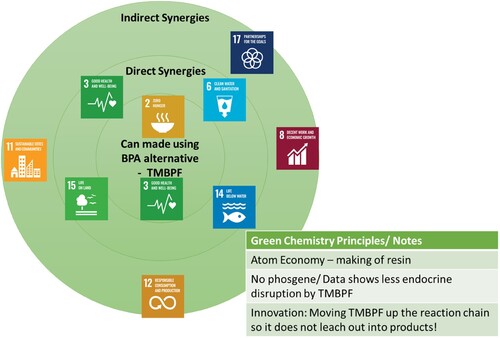
The substitution of TMBPF for BPA is interesting in that there was a strategic design by Sherwin Williams/Valspar as the new material was developed. The students were shown how a responsible alternatives assessment can be done. This included specific actions during the assessment phase that allowed TMBPF to become successful and not fall into the trap of a regrettable and costly substitution. These actions included testing the migration potential of TMBPF from the polymeric coating, minimizing a key exposure route and minimizing overall risks; use of intellectual property protection prior to the hazard assessment stage, which allowed transparency; involvement of stakeholders in the assessment process to inspire confidence; and consideration of additional hazard criteria, regulatory agency clearances and sustainable supply chain availability. A result of this strategic design was that the company realized a competitive advantage by offering a drop-in alternative to fill a market need (Citation49, Citation50).
In this example, we were able to discuss green chemistry principles since there was transparency in the chemical synthesis and toxicity data. The alternatives assessment approach discussed provided a pathway for students to think about how responsible substitutes can be developed. Because there is information about how the TMBPF development considered existing infrastructure and capital costs, some discussion about the economics of chemical substitution could be done. While there still continues to be research on the toxicity of TMBPF we focus on the approach and the fact that the TMBPF was moved up the synthesis chain to prevent leaching into the ultimate product (Citation47, Citation51). The students were also urged to think further about the equity implications, land use, workforce development, etc. that would make the implementation even more sustainable that are not evident in this case.
Conclusion
For a chemical process to be truly sustainable, practitioners must apply a systems thinking approach prior to the development of new chemicals. Sustainable chemistry requires out-of-the box thinking that is sensitive to functionality as well as environmental and human systems. The SDGs proposed by the UN are interconnected by design and interact in multiple ways. These interactions are dynamic and not always positive. The ultimate goal of truly sustainable chemistry would be synergistic – where the accomplishment of one goal will also help fulfill others. A systems thinking approach can help anticipate hidden negative effects, leading to better-informed decisions.
A systems thinking approach can be complex for students, creating a need for multiple tools to help visualize and tackle difficult issues. We have presented in this paper a graphical tool that specifically highlights synergies, conflicts and trade-offs when discussing chemical substitutions, green process development and life cycle analysis. In all the examples discussed, the students were urged to consider looking beyond chemistry and to include economics, equity, empowerment and the environment as they worked through the case studies. Through the structured Circles of Sustainability exercises we have developed; we are able to incorporate green chemistry and the UN SDGs into a platform that allows students to look at solutions that are not only related to chemistry. As a result, students can develop an appreciation of the intimate linkages between chemical systems and various other systems such as human health, the environment, regulatory systems and social systems. During the activities, students were engaged and the format of the activities allowed several discussions and points of view being shared between group members as well as between group members and instructor. Informal comments indicated that students universally enjoyed the activities and learned from them. We believe the examples provided can offer instructors opportunities to extend student comprehension of chemistry and provide a context to students on the central role that chemistry plays in solving global problems.
Supplemental Information for Circles Paper.docx
Download MS Word (2.6 MB)Disclosure statement
No potential conflict of interest was reported by the authors.
References
- Brundtland, C. G. (1987). Our Common Future: Report of the World Commission on Environment and Development. http://www.un-documents.net/ocf-ov.htm
- Mahaffy, P.G.; Matlin, S.A.; Holme, T.A.; MacKellar, J. Systems Thinking for Education About the Molecular Basis of Sustainability. Nature Sustainability 2019, 2 (5), 362–370. doi:10.1038/s41893-019-0285-3.
- Transforming Our World: The 2030 Agenda for Sustainable Development. https://sdgs.un.org/2030agenda (accessed Mar 28, 2024)
- Matlin, S.A.; Mehta, G.; Hopf, H.; Krief, A. The Role of Chemistry in Inventing a Sustainable Future. Nat. Chem. 2015, 7 (12), 941–943. doi:10.1038/nchem.2389.
- Welton, T. Editorial Overview: Un Sustainable Development Goals: How Can Sustainable/Green Chemistry Contribute? There Can Be More Than one Approach. Current Opinion in Green and Sustainable Chemistry 2018, 13, A7–A9. doi:10.1016/j.cogsc.2018.09.005.
- Anastas, P.T.W.; John, C. Green Chemistry Theory and Practice; Oxford University Press: Oxford, UK, 1998.
- Anastas, P.T.; Kirchhoff, M.M. Origins, Current Status, and Future Challenges of Green Chemistry. Acc. Chem. Res. 2002, 35 (9), 686–694. doi:10.1021/ar010065m.
- Beach, E.S.; Cui, Z.; Anastas, P.T. Green Chemistry: A Design Framework for Sustainability. Energy Environ. Sci. 2009, 2 (10), 1038. doi:10.1039/b904997p.
- Anastas, P.T.; Zimmerman, J.B. The United Nations Sustainability Goals: How Can Sustainable Chemistry Contribute? Current Opinion in Green and Sustainable Chemistry 2018, 13, 150–153.
- Byrnes, B.H. Environmental Effects of N Fertilizer Use ? An Overview. Fertilizer Research 1990, 26 (1), 209–215. doi:10.1007/BF01048758.
- Jacobs Molly, M.; Malloy Timothy, F.; Tickner Joel, A.; Edwards, S. Alternatives Assessment Frameworks: Research Needs for the Informed Substitution of Hazardous Chemicals. Environ. Health Perspect. 2016, 124 (3), 265–280. doi:10.1289/ehp.1409581.
- Lavoie, E.T.; Heine, L.G.; Holder, H.; Rossi, M.S.; Lee, R.E.; II, Connor, E.A.; Vrabel, M.A.; DiFiore, D.M.; Davies, C.L. Chemical Alternatives Assessment: Enabling Substitution to Safer Chemicals. Environ. Sci. Technol. 2010, 44 (24), 9244–9249. doi:10.1021/es1015789.
- Zimmerman, J.B.; Anastas, P.T. Toward Substitution with No Regrets. Science 2015, 347 (6227), 1198–1199. https://doi.org/10.1126/science.aaa0812.
- Arnold, R.D.; Wade, J.P. A Definition of Systems Thinking: A Systems Approach. Procedia. Comput. Sci. 2015, 44, 669–678. doi:10.1016/j.procs.2015.03.050.
- Lane, D.C. What We Talk About When We Talk About ‘Systems Thinking’. J. Oper. Res. Soc. 2016, 67 (3), 527–528. doi:10.1057/jors.2015.10.
- Anastas, P.T.; Zimmerman, J.B. Creating Cascading Non-Linear Solutions for the UN Sustainable Development Goals Through Green Chemistry. Chem 2021, 7 (11), 2825–2828. doi:10.1016/j.chempr.2021.10.025.
- Hutchison, J.E. Systems Thinking and Green Chemistry: Powerful Levers for Curricular Change and Adoption. J. Chem. Educ. 2019, 96 (12), 2777–2783. doi:10.1021/acs.jchemed.9b00334.
- Liu, J.; Hull, V.; Godfray, H.C.J.; Tilman, D.; Gleick, P.; Hoff, H.; Pahl-Wostl, C.; Xu, Z.; Chung, M.G.; Sun, J.; Li, S. Nexus Approaches to Global Sustainable Development. Nature Sustainability 2018, 1 (9), 466–476. doi:10.1038/s41893-018-0135-8.
- D’eon, J.; Silverman, J.R. Using Systems Thinking to Connect Green Principles and United Nations Sustainable Development Goals in a Reaction Stoichiometry Module. Green Chemistry Letters and Reviews 2023, 16 (1), 2185109. doi:10.1080/17518253.2023.2185109.
- Dicks, A.P.; D’eon, J.C.; Morra, B.; Kutas Chisu, C.; Quinlan, K.B.; Cannon, A.S. A Systems Thinking Department: Fostering a Culture of Green Chemistry Practice among Students. J. Chem. Educ. 2019, 96 (12), 2836–2844. doi:10.1021/acs.jchemed.9b00287.
- Mahaffy, P.G.; Matlin, S.A.; Whalen, J.M.; Holme, T.A. Integrating the Molecular Basis of Sustainability Into General Chemistry Through Systems Thinking. J. Chem. Educ. 2019, 96 (12), 2730–2741. doi:10.1021/acs.jchemed.9b00390.
- Miller, J.L.; Wentzel, M.T.; Clark, J.H.; Hurst, G.A. Green Machine: A Card Game Introducing Students to Systems Thinking in Green Chemistry by Strategizing the Creation of a Recycling Plant. J. Chem. Educ. 2019, 96 (12), 3006–3013. doi:10.1021/acs.jchemed.9b00278.
- Nagarajan, S.; Overton, T. Promoting Systems Thinking Using Project- and Problem-Based Learning. J. Chem. Educ. 2019, 96 (12), 2901–2909. doi:10.1021/acs.jchemed.9b00358.
- Paschalidou, K.; Salta, K.; Koulougliotis, D. Exploring the Connections Between Systems Thinking and Green Chemistry in the Context of Chemistry Education: A Scoping Review. Sustainable Chemistry and Pharmacy 2022, 29, 100788. doi:10.1016/j.scp.2022.100788.
- Talanquer, V.; Szozda, A.R. An Educational Framework for Teaching Chemistry Using a Systems Thinking Approach. J. Chem. Educ. 2024, 101 (5), 1785–1792. doi:10.1021/acs.jchemed.4c00216.
- Aubrecht, K.B.; Bourgeois, M.; Brush, E.J.; MacKellar, J.; Wissinger, J.E. Integrating Green Chemistry in the Curriculum: Building Student Skills in Systems Thinking, Safety, and Sustainability. J. Chem. Educ. 2019, 96 (12), 2872–2880. doi:10.1021/acs.jchemed.9b00354.
- Aubrecht, K.B.; Dori, Y.J.; Holme, T.A.; Lavi, R.; Matlin, S.A.; Orgill, M.; Skaza-Acosta, H. Graphical Tools for Conceptualizing Systems Thinking in Chemistry Education. J. Chem. Educ. 2019, 96 (12), 2888–2900. doi:10.1021/acs.jchemed.9b00314.
- Sustainability and Systems Thinking In Chemistry Education. https://sastice.com/ (accessed Mar 28, 2024)
- MacKellar, J.J.; Constable, D.J.C.; Kirchhoff, M.M.; Hutchison, J.E.; Beckman, E. Toward a Green and Sustainable Chemistry Education Road Map. J. Chem. Educ. 2020, 97 (8), 2104–2113. doi:10.1021/acs.jchemed.0c00288.
- Council, G. C. a. C. Considered Chemistry at Nike: Creating Safer Products through the Evaluation and Restriction of Hazardous Chemicals. https://www.greenchemistryandcommerce.org/downloads/Nike_final.pdf
- Nike Restricted Substances List (RSL) and Sustainable Chemistry Guidance (SCG). (2017). https://www.greenscreenchemicals.org/static/ee_images/uploads/resources/NIKE_INC_Restricted_Substances_Guidance_Aug_2011.pdf (accessed Mar 13, 2024)
- Evich, M.G.; Davis, M.J.B.; McCord, J.P.; Acrey, B.; Awkerman, J.A.; Knappe, D.R.U.; Lindstrom, A.B.; Speth, T.F.; Tebes-Stevens, C.; Strynar, M.J.; Wang, Z.; Weber, E.J.; Henderson, W.M.; Washington, J.W. Per- and Polyfluoroalkyl Substances in the Environment. Science 2022, 375, 6580. doi:10.1126/science.abg9065.
- Cousins, I.T.; Johansson, J.H.; Salter, M.E.; Sha, B.; Scheringer, M. Outside the Safe Operating Space of a New Planetary Boundary for Per- and Polyfluoroalkyl Substances (PFAS). Environ. Sci. Technol. 2022, 56 (16), 11172–11179. doi:10.1021/acs.est.2c02765.
- Glüge, J.; Scheringer, M.; Cousins, I.T.; Dewitt, J.C.; Goldenman, G.; Herzke, D.; Lohmann, R.; Ng, C.A.; Trier, X.; Wang, Z. An Overview of the Uses of Per- and Polyfluoroalkyl Substances (PFAS). Environmental Science: Processes & Impacts 2020, 22, 2345–2373. doi:10.1039/D0EM00291G.
- Kwiatkowski, C.F.; Andrews, D.Q.; Birnbaum, L.S.; Bruton, T.A.; DeWitt, J.C.; Knappe, D.R.U.; Maffini, M.V.; Miller, M.F.; Pelch, K.E.; Reade, A.; Soehl, A.; Trier, X.; Venier, M.; Wagner, C.C.; Wang, Z.; Blum, A. Scientific Basis for Managing PFAS as a Chemical Class. Environ. Sci. Technol. Lett. 2020, 7 (8), 532–543. doi:10.1021/acs.estlett.0c00255.
- Ng, C.; Cousins, I.T.; DeWitt, J.C.; Glüge, J.; Goldenman, G.; Herzke, D.; Lohmann, R.; Miller, M.; Patton, S.; Scheringer, M.; Trier, X.; Wang, Z. Addressing Urgent Questions for PFAS in the 21st Century. Environ. Sci. Technol. 2021, 55 (19), 12755–12765. doi:10.1021/acs.est.1c03386.
- Schreder, E., Beth K.. (2021). Path of Toxic Pollution. Retrieved 3-29-24 from https://toxicfreefuture.org/wp-content/uploads/2022/08/Report-Daikin-Path-of-Toxic-Pollution-new-logo.pdf
- Dalmijn, J.; Glüge, J.; Scheringer, M.; Cousins, I.T. Emission Inventory of PFASs and Other Fluorinated Organic Substances for the Fluoropolymer Production Industry in Europe. Environmental Science: Processes & Impacts 2024, 26 (2), 269–287. doi:10.1039/d3em00426k.
- Find Alternatives to PFAS. https://chemsec.org/knowledge/all-you-need-to-know-about-pfas/#find-alternatives-to-pfas (accessed Mar 29, 2024)
- Michałowicz, J. Bisphenol A – Sources, Toxicity and Biotransformation. Environ. Toxicol. Pharmacol. 2014, 37 (2), 738–758. doi:10.1016/j.etap.2014.02.003.
- N'Dri, L.A.; White-Newsome, J.L.; Corbin-Mark, C.D.; Shepard, P.M. The Invisible Threat: Bisphenol-A and Phthalates in Environmental Justice Communities. Environmental Justice 2015, 8 (1), 15–19. doi:10.1089/env.2014.0015.
- van Woerden, I.; Bruening, M.; Montresor-López, J.; Payne-Sturges, D.C. Trends and Disparities in Urinary BPA Concentrations among U.S. Emerging Adults. Environ. Res. 2019, 176, 108515. doi:10.1016/j.envres.2019.05.046.
- Ritter, S. (2011). Bisphenol A. Chemical and Engineering News, 23. https://pubsapp.acs.org/cen/coverstory/89/8923cover.html
- Bittner, G.D.; Yang, C.Z.; Stoner, M.A. Estrogenic Chemicals Often Leach from BPA-Free Plastic Products That Are Replacements for BPA-Containing Polycarbonate Products. Environ. Health 2014, 13 (1), 41. doi:10.1186/1476-069X-13-41.
- Chen, D.; Kannan, K.; Tan, H.; Zheng, Z.; Feng, Y.-L.; Wu, Y.; Widelka, M. Bisphenol Analogues Other Than BPA: Environmental Occurrence, Human Exposure, and Toxicity—A Review. Environ. Sci. Technol. 2016, 50 (11), 5438–5453. doi:10.1021/acs.est.5b05387.
- Cornwall, W. To Replace Controversial Plastic Additive BPA, a Chemical Company Teams Up with Unlikely Allies. Science. doi:10.1126/science.aba9956
- Maffini, M.V.; Canatsey, R.D. An Expanded Toxicological Profile of Tetramethyl Bisphenol F (TMBPF), a Precursor for a New Food-Contact Metal Packaging Coating. Food Chem. Toxicol. 2020, 135, 110889. doi:10.1016/j.fct.2019.110889.
- Soto, A.M.; Schaeberle, C.; Maier, M.S.; Sonnenschein, C.; Maffini, M.V. Evidence of Absence: Estrogenicity Assessment of a New Food-Contact Coating and the Bisphenol Used in Its Synthesis. Environ. Sci. Technol. 2017, 51 (3), 1718–1726. doi:10.1021/acs.est.6b04704.
- Tickner, J.A.; Simon, R.V.; Jacobs, M.; Pollard, L.D.; van Bergen, S.K. The Nexus Between Alternatives Assessment and Green Chemistry: Supporting the Development and Adoption of Safer Chemicals. Green Chemistry Letters and Reviews 2021, 14 (1), 23–44. doi:10.1080/17518253.2020.1856427.
- Valspar: How We Engaged Stakeholders to Solve the BPA Dilemma. https://www.greenbiz.com/article/valspar-how-we-engaged-stakeholders-solve-bpa-dilemma
- Hyun, M.; Rathor, L.; Kim, H.-J.; McElroy, T.; Hwang, K.H.; Wohlgemuth, S.; Curry, S.; Xiao, R.; Leeuwenburgh, C.; Heo, J.-D.; Han, S.M. Comparative Toxicities of BPA, BPS, BPF, and TMBPF in the Nematode Caenorhabditis Elegans and Mammalian Fibroblast Cells. Toxicology 2021, 461, 152924. doi:10.1016/j.tox.2021.152924.
- Green Chemistry: Chemical Products and Processes that Reduce or Eliminate the Use of Hazardous Substances. https://circulardesign.nike.com/green-chemistry (accessed Mar 13, 2024)