ABSTRACT
The sociality of the Arctic fox has been extensively studied during the breeding season, so that its summer territorial and generally monogamous social systems are now well described. A key knowledge gap remains, however, during the winter season, when logistic challenges preclude detailed observation of individuals. We have studied the socio-spatial winter dynamics of Arctic fox pairs to determine: (1) winter fidelity of Arctic fox pair mates to their summer home range; (2) the degree to which extraterritorial movements are simultaneous between pair mates; and (3) spatial proximity between pair mates when they perform simultaneous extraterritorial movements. To meet these objectives, 15 Arctic fox pairs from Bylot Island (Nunavut, Canada) were tracked during at least one winter in 2007–2011, using Argos satellite collars, for a total of 21 pair-years. Arctic foxes were generally faithful to their summer home ranges during winter, but some variation occurred among pairs. The degree of territory fidelity was highly correlated between pair mates. When foxes did extraterritorial movements, they performed excursions that were short in duration and generally not synchronized among pair mates. When pair mates were outside the territory at the same time, they did not travel together and rather foraged independently. We discuss some ecological implications of our findings, and suggest that different patterns may be observed in other Arctic fox populations. If such is the case, replicating our study in other parts of the species range will allow productive hypothesis testing regarding the determinants of Arctic fox winter sociality.
Introduction
Many canids are socially monogamous, with groups typically including a dominant mated pair and one or more related but reproductively suppressed subordinates (Kleiman Citation1977; Asa & Valdespino Citation1998; Baker et al. Citation2004). This social system implies long-lasting pair bonds that persist across multiple breeding seasons, extensive overlap between home ranges of the pair mates and cooperation in the care of the young (Geffen & Macdonald Citation1992; Pauw Citation2000). Social monogamy may optimize individual fitness by enhancing survival and reproductive success (Ralls et al. Citation2007). It is, however, still unclear why pair mates in some species associate closely year-round, whereas in others they only meet once a year for breeding (Kappeler Citation2013). A long-lasting pair bond may have some fitness benefits during the non-breeding season. Those benefits can be related to the importance for individuals of maintaining their well-known territory once acquired (Ralls et al. Citation2007) or to cooperative territory defence (Dobson et al. Citation2010). Higher benefits may also be obtained the next breeding season by remaining with a successful and compatible mate. Annually searching for a new mate can increase predation risk and energy cost while travelling through unfamiliar territory, and also increases the risk of mate incompatibility (Kleiman Citation1977; Ralls et al. Citation2007). Investigating the social structure during the non-breeding season is important because in many monogamous species the non-breeding season is far longer than the breeding one and sociality during fall–winter could influence dispersal and mortality (Patterson & Messier Citation2001), with potential effects on population dynamics.
The degree to which activities are simultaneous between pair mates and the spatial proximity of pair mates are two key variables used to describe social monogamy and infer its costs and benefits (Kleiman Citation1977; Dietz Citation1984). The degree of simultaneity depends largely on the strength of the pair bond and the need to coordinate activities such as feeding, breeding, resting and territory defence (Kleiman Citation1981; Kitchen et al. Citation2005; Bandeira de Melo et al. Citation2007). Predator avoidance and parental care promote proximity between pair mates (Kleiman Citation1977, Citation1981; Pusey Citation2005), whereas foraging for small prey promotes separation (Parker & Ruttan Citation1988; White et al. Citation2000; Kitchen et al. Citation2005). Territoriality is a common feature in monogamous mammals (Kleiman Citation1981). Spatial fidelity incurs repetitive or continuous use of previously occupied space (Kitchen et al. Citation2000). Continued use of a site by an individual is positively related to the cost of changing territory, to the previous reproductive success, and to the probability of mortality in this habitat (Switzer Citation1993). Range fidelity offers benefits such as knowledge of foraging areas, dens and breeding opportunities (Kitchen et al. Citation2000). Spatially faithful individuals can make short-term excursions outside of their home range (EMs) to search for mates, resources or better living conditions (Lidicker & Stenseth Citation1992; Soulsbury et al. Citation2011). Those movements are different from dispersal movements, which are one-way movements of individuals away from their home range (Lidicker & Stenseth Citation1992).
We used the Arctic fox (Vulpes lagopus) to study spatial fidelity of pair mates and the degree to which simultaneous activities occur between pair mates during the winter season. Year-round partnership and year-round territoriality are observed in many canids such as the kit fox (Vulpes macrotis; Ralls et al. Citation2007), the swift fox (Vulpes velox; Kitchen et al. Citation2005), the maned wolf (Chrysocyon brachyurus; Bandeira de Melo et al. Citation2007), the coyote (Canis latrans; Hennessy et al. Citation2012) and the grey wolf (Canis lupus; Mech & Boitani Citation2010). Arctic foxes form socially monogamous pairs (Norén et al. Citation2011) and have been reported to mate for life (Audet et al. Citation2002). Pairs display a strong territorial behaviour, especially during the denning season (April to August), when the pair mates share an overlapping home range (Eberhardt et al. Citation1982; Prestrud Citation1992). However, even during this period, pair mates rarely interact (Garrot et al. Citation1984). Both actively care for the young at the den (Strand et al. Citation2000), but the pair bond has been reported to gradually weaken over the course of the summer and to break when the young leave the natal den (Fay & Follmann Citation1982). Winter movements of Arctic foxes are poorly documented. Some telemetry studies found that Arctic foxes tend to remain close to their denning site during winter (Anthony Citation1997; Landa et al. Citation1998), whereas field observations suggested that they show extensive seasonal movements, with a movement towards the coast and onto the sea ice during fall and early winter and a return to the home range at the onset of the next breeding season (Chitty & Chitty Citation1945; Chesemore Citation1968; Wrigley & Hatch Citation1976). Pair mates leaving the territory may remain largely solitary (Fay & Follmann Citation1982; Audet et al. Citation2002). During winter, terrestrial resources are scarcer because migratory birds have left (Gauthier et al. Citation2011). When sea ice is available, it offers additional food resources to Arctic foxes (Smith Citation1976; Tarroux et al. Citation2012). Roth (Citation2002) showed that marine food became more important during terrestrial food shortage, accounting for nearly half of the winter protein intake. Sea ice thus offers Arctic foxes the possibility to move to a completely new foraging habitat located outside their terrestrial home range during winter, which is usually not the case for other canids. The tension between two conflicting forces – on one hand, remaining with the mate and keeping the territory outside of the breeding season; on the other hand, having to search for food resources outside of the home range – may shape the degree of spatial proximity of pairs during winter.
During the summers 2007-2011, we fitted 74 adult Arctic foxes with 91 satellite collars in the Canadian High Arctic to monitor their year-round movements. We analysed the movements of 30 adults forming 21 pair-years to answer three objectives. First, we assessed the degree of fidelity of pair mates to their summer home range during winter and the similarity of this fidelity between pair mates. Considering that marine resources are important during winter and that Arctic foxes can be highly mobile, we expected a low fidelity of Arctic fox pairs to their summer home range. Second, we assessed the degree to which simultaneous EMs occur between pair mates. Because of the solitary nature of Arctic foxes and the benefits derived from maintaining territory defence, we expected a low degree of simultaneous EMs in Arctic fox pair mates. Third, we assessed the spatial proximity between pair mates when they were simultaneously outside of their home range. We expected pair mates to move separately because Arctic foxes are not known to incur benefits from group foraging.
Methods
Study area
We worked in a 600 km2 study area located in the southern plain of Bylot Island (73°N, 80°W), which is part of Sirmilik National Park of Canada, Nunavut (). The Arctic fox is the main terrestrial predator of the island. The red fox (Vulpes vulpes), a potential predator and a competitor for Arctic fox (Tannerfeldt et al. Citation2002; Pamperin et al. Citation2006), is only present in low numbers in the region (Gagnon & Berteaux Citation2009; Gauthier et al. Citation2013). Two species of lemmings are present: the brown lemming (Lemmus trimucronatus) and the collared lemming (Dicrostonyx groenlandicus). Populations of brown lemmings show typical fluctuations of large amplitude with a periodicity of three to four years, whereas populations of collared lemmings show low-amplitude cycles (Gruyer et al. Citation2008, Citation2010). The southern plain of the island is the most important breeding site for the greater snow goose (Chen caerulescens atlantica) in the Canadian High Arctic (Bêty et al. Citation2001). Sea ice on the south coast of Bylot Island forms in late October and breaks up in late July (Canadian Ice Service Citation2013). Inuit traditional knowledge indicates that Arctic foxes in the area forage on the sea ice and hunt seal pups in their birth lairs (Gagnon & Berteaux Citation2009).
Figure 1. Arctic fox study area (black contour) on Bylot Island, Nunavut, Canada. Fox dens are represented with triangles, with filled triangles indicating dens occupied by fox pairs followed during the study. Identification of numbered dens is as follows: den identification-last two digits of year-fox pair identification.
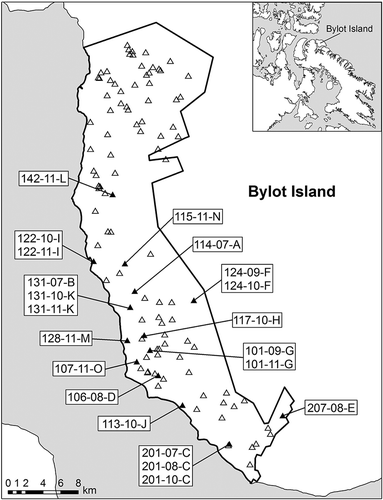
Captures and satellite tracking
The Bylot Island Arctic fox population has been monitored opportunistically since 1993 and systematically since 2003. Every summer, all known dens (ca. 100) are visited to assess presence of young and adults. We captured adults using padded foot traps (model Softcatch # 1, Oneida Victor). When needed, we anaesthetized animals using a combination of Medetomidine (0.05 ml kg–1) and Ketamine (0.03 ml kg–1). Atipemazole (0.05 ml kg–1) was used as an antidote to the anaesthetic before releasing individuals at their capture site. We marked individuals using a unique combination of coloured and numbered ear tags (Rototags, Dalton Supplies). Since 2007, we have been fitting individuals with collars bearing Argos Platform Transmitter Terminals (Model Kiwisat 202, Sirtrack). Collars weighed 2.6–3.5% of body mass and we used three different collar settings. The collars transmitted for a three- to four-hour period (13:00–17:00 UTC, corresponding to 07:00–11:00 local time) daily or every two days (), with a repetition rate of 60 seconds for all collars. We therefore obtained locations only during the morning, potentially generating sampling bias, considering that fox activity can vary throughout the 24-hour cycle (Eberhardt et al. Citation1982; Cypher Citation2003). This should not, however, affect our comparisons within and between pair mates since all individuals were sampled in a similar way. Data were recovered through the Argos System. We used data from May 2007 to April 2012.
Table 1. Description of the Argos Platform Terminal Transmitter settings for 40 collars fitted on 30 adult Arctic foxes tracked during winter (2007–2011) in the Canadian High Arctic (for two individuals, the same collars transmitted in both 2007 and 2008, giving a total of 42 fox-years).
In this study, we defined the winter season as the period between sea-ice formation (25 October) and end of the mating period (30 April), whereas we considered the summer season to start with the beginning of pup rearing (1 May) and end with juvenile dispersal (1 October) (Audet et al. Citation2002). We selected for analyses 30 Arctic foxes forming 15 pairs, for a total of 21 pair-years. Mated pairs were confirmed by visual field observations or pictures taken at dens (Silent Image and RapidFire Professional, Reconyx) and were monitored for ≥ 2–6 months during winter (see monitoring length in Supplementary Table S1).
In order to have a suitable level of accuracy for the analyses, we only kept positions with a Location Class 3, 2 or 1, corresponding respectively to positioning errors having a 68% probability of being < 250 m, < 500 m, and < 1500 m (CLS Citation2011). We removed all positions with a Location Class 0, A, B or Z, corresponding to large (> 1500 m) or unquantifiable positioning errors (CLS Citation2011). Using coordinates projected in the Universal Transverse Mercator, North American Datum 83 system, we filtered Argos data through R 3.0.1 software (R Core Team Citation2013) in order to exclude locations resulting in unrealistic speed and distance values (> 7 km h−1 cruising speed, with possible 12-minute acceleration bouts of 10 km h−1; see S1 File in Christin et al. Citation2015). We previously evaluated Argos telemetry accuracy on Bylot Island and reported some of the lowest published errors for this system (Christin et al. Citation2015), likely on account of increased satellite coverage at high latitudes.
Home range fidelity of pair mates
A preliminary analysis based on an Utilization Distribution Overlap Index (Fieberg & Kochanny Citation2005) showed that the summer home range of males and females overlapped by 82% ± 13% (mean ± standard deviation), therefore we combined male and female locations to obtain the pair’s summer home range. We estimated home ranges using the fixed kernel method with a 90% isopleth (Seaman & Powell Citation1996; Borger et al. Citation2006) using the adehabitatHR library in R (Calenge Citation2006). We selected the 90% isopleth to represent the full range of the pairs while excluding exploratory movements (Borger et al. Citation2006). To decrease data temporal autocorrelation when estimating home ranges, we kept one location per day of tracking, based on the smallest location error. Using the 12 pairs with the highest number of locations during the summer period (n > 77 locations per individual), we plotted the summer home range size of the pairs with respect to the number of locations. Locations were randomly added in increments of five until all locations of a pair were assessed, and the process was repeated 99 times for each pair (Harris et al. Citation1990). Kernel estimates reached an asymptote at 44 ± 10 locations on average. All pairs used in this study have a number of locations higher than 44. When calculating fixed kernels, we chose the smoothing parameter by first allowing R to allocate specific smoothing parameters to each pair using the reference bandwidth (href) in adehabitatHR. We then ran a second analysis in which we manually calculated a global smoothing factor as the median smoothing parameter (0.656) from the first analysis (Dahl & Willebrand Citation2005). We used the href method because it is suitable for central place foragers showing unimodal utilization distribution (Bowman Citation1985; Worton Citation1989; Hemson et al. Citation2005), such as the Arctic fox in summer.
We created an FI to estimate the winter fidelity of each member of the pair relative to the pair’s summer home range:
Individuals always remaining in their summer home range during the winter have an FI of 100, whereas individuals never using their summer home range during winter have an FI of 0. We calculated FI for each pair mate.
To complement our analysis of the winter fidelity of fox pairs to their summer home range, we also compared the size of each pair’s summer home range to their winter home range after removing locations outside Bylot Island (hereafter the ‘terrestrial winter home range’). In addition, as each member of the pair may move independently during winter, we also estimated the winter ranging area of each fox separately using all of their winter locations (on land and on the sea ice) with the specific smoothing parameters allocated by href for each individual.
Extraterritorial movements and spatial proximity of pair mates
EMs could be considered as all movements beyond the boundary of the summer home range. However, to take positioning errors into account and avoid confusions between EM and seasonal changes in position or size of home ranges, we identified EM as all movements ≥ 2 km from the summer home range boundary followed by a return. This 2 km distance represents approximately 30% of a home range diameter and is consistent with the threshold used by Nicholson et al. (Citation2007) to study EM in swift foxes. A movement ≥ 2 km from the summer home range boundary not followed by a return was identified as a dispersal movement (permanent departure) and not considered in the analysis of EMs. When pair mates were both outside their home range during an EM for a given collar transmission period, we considered that they did a simultaneous EM for that day. We created an SI to estimate the tendency of pair mates to perform simultaneous EM:
Pair mates always doing simultaneous EMs have an SI of 100, whereas pair mates never doing simultaneous EMs have an SI of 0. For each pair, we used for analysis data obtained during the period over which both pair mates were tracked. When an adult dispersed, we considered dispersal to break the pair bond and therefore only analysed locations obtained before dispersal. The daily or bi-daily duty cycle of the collars may have caused us to miss some short-term EMs, but we likely detected most of the longest excursions, which were also the most relevant in terms of pair bonding. When we observed a simultaneous EM and both pair mates were located within an hour, we measured spatial proximity between pair mates by calculating the Euclidian distance between pair mate locations.
Statistical analyses
Because transmission rate varied across collars, with some pair mates sampled daily and others sampled every second day (), we checked whether SI or FI was affected by sampling effort. Using data subsampling and paired t-tests, we found that retaining only locations obtained every second day for the pairs that had been located daily did not affect FI (FI24 h = 74.4 ± 24.5; FI48 h = 74.4 ± 24.5) but decreased slightly SI (SI24 h = 15.5 ± 14.8; SI48 h = 11.8 ± 14.8). None of these differences, however, were significant (FI: t = −0.019, p = 0.99, df = 41; SI: t = 2.07, p = 0.07, df = 19) so we report values obtained from the original data sets. To test whether males and females had a similar FI, we used a Pearson’s product-moment correlation after normalizing our data using the arcsine square root transformation. To compare the size of the pairs’ summer home ranges with the size of the pairs’ terrestrial winter home ranges, we used paired t-tests after normalizing our data using the log transformation. We similarly compared the size of summer home ranges with the size of the winter ranging areas of each pair mate.
To assess whether SI was different than expected by chance, we performed the following randomization procedure. For each pair, we first calculated the observed SI. Then, the days with EM for each pair mate were mixed over the tracking period of the pair and a new SI was determined from this random rearrangement of days with EM. This procedure was repeated 999 times for each pair. If the observed SI was significantly different from the mean SI obtained by randomization (p < 0.05), the observed SI was considered to be significantly different from the one expected by chance.
To assess the level of spatial proximity of pair mates when they were simultaneously outside of their home range, we compared distances between pair mates when they were both located inside their home range to distances between pair mates during simultaneous EM, using a Student’s t-test. Sample sizes were higher inside the home range than outside, so to have the same sample size as distances outside we carried a sampling without replacement of distances inside the home range 10 000 times. We performed the Student’s t-test 10 000 times for each pair (once for each sampling without replacement). If 95% of the observed t-values were higher than or equal to the critical t-value, we considered that the mean distance inside the home range was significantly different from the mean distance during simultaneous EM.
We present summary statistics as means ± standard deviation. We performed all statistical analyses in R 3.0.1.
Results
We obtained 6128 locations (after filtering) for the 42 fox-years, with a mean of 4.7 ± 2.5 locations per day of tracking per individual. During the 2007–2011 study period, nine collars stopped transmitting before 30 April and four females and three males (from five pairs) dispersed during winter (Supplementary Table S1).
Home range fidelity of pair mates
The size of fox pairs’ summer home ranges (34.9 ± 14.6 km2; range: 16.9–84.2) was not significantly different (paired t-test, t = 1.07, p = 0.298, df = 20) from the size of pairs’ terrestrial winter home ranges (34.2 ± 20.8 km2; range: 16.9–86.6). In contrast, the winter ranging areas (including locations on land and sea ice) of pair mates were significantly larger than their summer home ranges (paired t-test, t = −5.97, p < 0.001, df = 41) and varied widely from 15.7 to 9615.9 km2 (median = 102.1 km2). The mean FI of individuals was 74 ± 25% (range: 19–100%), indicating that during winter foxes still spent a large proportion of their time in their summer home range. Within a given pair, FI of the male and female were highly correlated (r = 0.73, p < 0.001, df = 19). Only five out of 21 pair-years had a FI ≤ 60% for at least one of the pair mates. In four of them, one or both pair mates dispersed during winter ().
Figure 2. Relationship between the FI of males and females within Arctic fox pairs tracked during winter (2007–2011) in the Canadian High Arctic. Each data point represents a given pair during a given winter. The diagonal indicates a similar FI for both pair mates. Data come from 15 pairs, including one tracked for three years and four tracked for two years (21 pair-years).
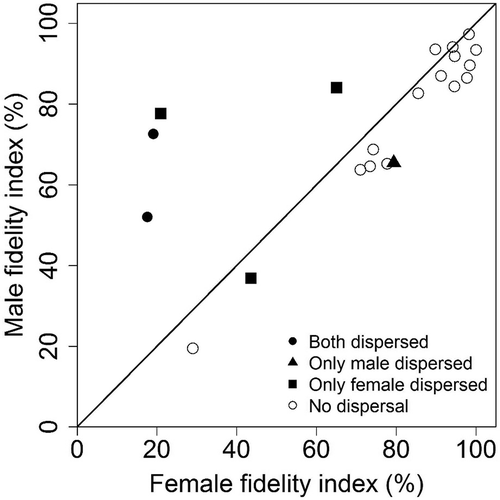
Simultaneous extraterritorial movements between pair mates
We excluded from this analysis one pair tracked in 2008 because the collars of the pair mates were not transmitting the same day so we could not determine simultaneous EM. The mean SI for the remaining 20 pair-years was 15.5 ± 14.8% (range: 0–58.8%), indicating that pair mates performed rather few simultaneous EMs. EMs were generally of short duration (median = 1 day, mean = 2.0 ± 4.8 days, n = 652) and most simultaneous EMs occurred in December–January (Supplementary Fig. S1). We observed no simultaneous EM at all in six out of 20 pair-years. In eight of the 14 pair-years with simultaneous EMs, the observed SI was significantly higher than the mean SI obtained by randomization (). Overall, these results thus indicate no clear tendency of Arctic fox pair mates to seek or avoid simultaneous EMs.
Table 2. Observed SI and mean SI (±standard deviation) obtained through randomization for Arctic fox pairs studied by satellite tracking in the Canadian High Arctic. Data are from 14 pairs tracked for one to three years (20 pair-years). EMs were defined as movements ≥ 2 km of the summer home range boundaries. Values of p in boldface indicate statistical significance at the 0.05 level.
Spatial proximity of pair mates during extraterritorial movements
We obtained 33.1 ± 24.5 distances per pair-year of pair mates located within one hour while performing simultaneous EMs (range = 6–71, median = 23.5, n = 14 pair-years). Pair mates were further apart during simultaneous EMs (18.9 ± 21.4 km) than when inside the boundaries of their summer home range (2.5 ± 0.8 km). The difference was significant in 12 of the 14 pair-years showing simultaneous EMs ().
Figure 3. Comparison of the mean (± standard deviation) distance between Arctic fox pair mates when they were both inside (grey bars) and outside (black bars) of the boundaries of their summer home range. Each pair is identified by a capital letter. Data come from 12 Arctic fox pairs tracked during winter (2007–2011) in the Canadian High Arctic, including pairs C and F that were tracked for two years each, for a total of 14 pair-years. Distances inside and outside the home range were significantly higher for 12 pair-years (asterisks), and not significantly different for two pair-years, as determined through randomization t-tests.
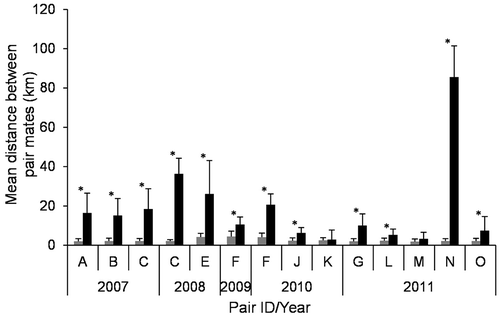
Discussion
Our study of space use by Arctic fox pairs during winter is, to our knowledge, the first for the species based on a relatively large sample of individuals. It shows that individuals were highly faithful to their summer home range during the winter period, which contrasts with our first prediction. Although we observed that individuals could roam over relatively wide areas when leaving their terrestrial winter home ranges, home range fidelity remained generally high for both pair mates, suggesting interactions between them (Hennessy et al. Citation2012) and year-round maintenance of the pair bond. Arctic foxes in our study area therefore display the typical social structure of canids, which consists of a long-lived pair bond, with pair mates that may continue to interact even after the breeding season (Kleiman & Eisenberg Citation1973; Kleiman Citation2011). Interestingly, the dispersal of one pair mate, male or female, did not necessarily lead to the dispersal of the other, indicating that either sex may hold and maintain the territory after a mate’s departure. Consistent with our two other predictions, we also found a low degree of simultaneous EMs in Arctic fox pair mates, and solitary movements when pair mates were simultaneously outside of their territory, indicating that they appear to behave independently once they leave the territory.
Home range fidelity of pair mates
The patterns of social monogamy and perennial pair bond found in canids, unusual in other mammals, have been hypothesized to represent phylogenetically old traits within the Canidae family (Kleiman & Eisenberg Citation1973; Dietz Citation1984). The Arctic fox was the only canid for which it was assumed, based on relatively old reports, that pair mates would separate and leave the breeding grounds to relocate on the coast or the sea ice until the following breeding season (Soper Citation1944; Wrigley & Hatch Citation1976; Fay & Follmann Citation1982). Previous telemetry studies showed conflicting results regarding adult winter movements in Arctic foxes. Lifelong mate and home range fidelity were reported for Arctic foxes living in areas with no access to sea ice, such as Iceland, Sweden and Norway (Tannerfeldt & Angerbjörn Citation1996; Landa et al. Citation1998; Strand et al. Citation2000). In regions with access to the sea ice, such as Alaska (Anthony Citation1997; Pamperin Citation2008; Lehner Citation2012) and Svalbard (Frafjord & Prestrud Citation1992), different residency patterns were observed, with some foxes staying resident and others performing long-distance movements, possibly reflecting the flexibility of the species, but the trend remained unclear on account of the difficulty of relocating the individuals regularly all year or very small sample sizes per year. Possible differences in seasonal home range fidelity and pair bonding across Arctic fox populations may reflect local ecological conditions. Individuals from populations inhabiting inland areas where the sea ice is accessible but further away than for our coastal population may be more prone to leave their mate and territory to move onto the sea ice for the winter, as short EMs would then be too costly. Tracking other populations inhabiting sea-ice areas would be needed to resolve this question.
Attempting to remain with the mate during the winter season has several potential benefits, including the maintenance of a well-known territory once acquired (Ralls et al. Citation2007) and cooperative territory defence (Dobson et al. Citation2010). Arctic foxes live in a constraining environment (Fuglei et al. Citation2004) and store food in summer and fall for retrieval when prey are less abundant (Prestrud Citation1991; Careau et al. Citation2007; Careau et al. Citation2008). This caching behaviour may increase the value of the territory and promote its maintenance year-round. Home range fidelity in winter also directly connects to the mating strategy of individuals. In our study area, multiple paternity and extra-pair paternity are frequent (Carmichael et al. Citation2006; Cameron et al. Citation2011), therefore complex interactions within and between fox pairs likely occur in March–April, when females have their unique three-to-five-day-long oestrus. Higher temporal and spatial resolution of data are needed to test causal hypotheses on this important topic.
Simultaneous extraterritorial movements between pair mates
While Arctic fox pairs remained highly faithful to their summer home range during winter, they often made short-term movements to the sea ice and sometimes inland. Overall, the degree of simultaneous EMs was low. This could result from two strategies that are not mutually exclusive. First, when canids forage primarily on small prey such as rodents, they usually hunt alone and avoid their mate in order to increase foraging efficiency, as reported in the red fox (Poulle et al. Citation1994), the swift fox (Kitchen et al. Citation2005), the kit fox (White et al. Citation2000) and the maned wolf (de Almeida Jácomo et al. Citation2009). Arctic foxes, as lemming specialists, may also exhibit this solitary foraging behaviour, whether they forage inside or outside their territory, resulting into a low degree of simultaneous EMs. Alternatively, this low degree of simultaneity could reflect a coordination of home range defence, with one mate guarding the territory while the other is foraging outside (Krebs Citation1982; Davies & Houston Citation1983). However, EMs of Arctic fox pairs last for only a few days and this short time lapse may not be long enough to lose the territory (Tsukada Citation1997).
In more than half of the pairs making simultaneous EMs, these were more simultaneous than expected by chance (although the degree of simultaneity remained low). Despite the need to defend the territory year-round, which could prevent long or frequent EMs (explaining low level of simultaneity), the need to find food outside the territory could be triggered by some environmental conditions shared at the same time by the pair mates (explaining simultaneous EMs). Indeed, simultaneous EMs of pairs peaked in December–January, matching the peak of individual EMs (Supplementary Fig. S1), which could indicate that food is less available in their tundra territory at this time of the year, or that food is more available on the sea ice.
Spatial proximity of pair mates during extraterritorial movements
The distance between pair mates was clearly lower when they were inside the territory than when they were outside, except for two pairs. The distance between both pair mates may depend on the main stimuli leading to EMs. The distance between pair mates outside of the home range may be high during foraging trips triggered by lack of resources inside the home range. An example of a stimulus outside the home range in our study area is a clumped food resource such as a marine mammal carcass, which can attract several foxes from their territory to the same spot on the sea ice (Lai et al. Citation2015). Pair mates may sometimes detect these hot spots simultaneously and therefore forage in close proximity during an EM. Such circumstances are probably too rare, however, to strongly influence average distance between pair mates when they are out of the territory.
Conclusion
Seasonal movements and solitary winter life are often assumed for Arctic foxes living in sea ice areas (Wrigley & Hatch Citation1976; Eberhardt et al. Citation1982; Audet et al. Citation2002), yet little quantitative information is available to support this. The technological advances in wildlife tracking (e.g., increased precision of location data, miniaturization of tracking devices, remote retrieval of data through satellites) have now overcome the logistic challenges associated with following relatively small mammals over long distances in Arctic winter conditions, providing new knowledge about their movement patterns. These developments, which apply to other species living in remote regions, will allow a better understanding of the ecology of these species and the impact of their movements on ecosystem functioning.
Supplementary material
Download PDF (210.3 KB)Acknowledgements
We are grateful to all field assistants for their help in the field. We thank Dr Carl Soulsbury and an anonymous reviewer for their comments on an earlier draft. Capture techniques and immobilization procedures were approved by the Université du Québec à Rimouski Animal Care Committee, and field research was approved by the Joint Park Management Committee of Sirmilik National Park of Canada.
Disclosure statement
No potential conflict of interest was reported by the authors.
Supplemental Material
Supplemental data for this article can be accessed here.
Additional information
Funding
References
- Anthony R.M. 1997. Home ranges and movements of Arctic fox (Alopex lagopus) in western Alaska. Arctic 50, 1–10.
- Asa C.S. & Valdespino C. 1998. Canid reproductive biology: an integration of proximate mechanisms and ultimate causes. American Zoologist 38, 251–259.
- Audet A.M., Robbins C.B. & Larivière S. 2002. Alopex lagopus. Mammalian Species 713, 1–10.
- Baker J.P., Funk S.M., Bruford M.W. & Harris S. 2004. Polygynandry in a red fox population: implications for the evolution of group living in canids? Behavioral Ecology 15, 766–778.
- Bandeira de Melo L.F., Lima Sabato M.A., Vaz Magni E.M., Young R.J. & Coelho C.M. 2007. Secret lives of maned wolves (Chrysocyon brachyurus Illiger 1815): as revealed by GPS tracking collars. Journal of Zoology 271, 27–36.
- Bêty J., Gauthier G., Giroux J.F. & Korpimaki E. 2001. Are goose nesting success and lemming cycles linked? Interplay between nest density and predators. Oikos 93 388–400.
- Borger L., Franconi N., De Michele G., Gantz A., Meschi F., Manica A., Lovari S. & Coulson T. 2006. Effects of sampling regime on the mean and variance of home range size estimates. Journal of Animal Ecology 75, 1393–1405.
- Bowman A.W. 1985. A comparative study of some kernel-based nonparametric density estimators. Journal of Statistical Computation and Simulation 21, 313–327.
- Calenge C. 2006. The package adehabitat for the R software: a tool for the analysis of space and habitat use by animals. Ecological Modelling 197 516–519.
- Cameron C., Berteaux D. & Dufresne F. 2011. Spatial variation in food availability predicts extrapair paternity in the Arctic fox. Behavioral Ecology 22, 1364–1373.
- Canadian Ice Service 2013. Archives; weekly regional ice charts; eastern Arctic region. Accessed on the internet http://iceweb1.cis.ec.gc.ca/Archive/page1.xhtml?lang=en on 1 November 2013.
- Careau V., Giroux J.-F. & Berteaux D. 2007. Cache and carry: hoarding behavior of Arctic fox. Behavioral Ecology and Sociobiology 62, 87–96.
- Careau V., Lecomte N., Bêty J., Giroux J.-F., Gauthier G. & Berteaux D. 2008. Hoarding of pulsed resources: temporal variations in egg-caching by Arctic fox. Ecoscience 15, 268–276.
- Carmichael L.E., Szor G., Berteaux D., Giroux M.A. & Strobeck C. 2006. Free love in the Far North: plural breeding and polyandry of Arctic foxes (Alopex lagopus) on Bylot Island, Nunavut. Canadian Journal of Zoology 85, 338–343.
- Chesemore D.L. 1968. Distribution and movements of white foxes in northern and western Alaska. Canadian Journal of Zoology 46, 849–854.
- Chitty H. & Chitty D. 1945. Canadian Arctic wild life enquiry, 1942-43. The Journal of Animal Ecology 14, 37–45.
- Christin S., St-Laurent M.-H. & Berteaux D. 2015. Evaluation of Argos telemetry accuracy in the High-Arctic and implications for the estimation of home-range size. Plos ONE 10(11), article no: e0141999, doi: 10.1371/journal.pone.0141999
- CLS 2011. Argos user’s manual. Accessed on the internet at http://www.argos-system.org/manual/on on 1 November 2013.
- Cypher B.L. 2003. Foxes, Vulpes species, Urocyon species, and Alopex lagopus. In G.A. Feldhamer et al. (eds.): Wild mammals of North America: biology, management, and conservation. Pp. 511–546. Baltimore: John Hopkins University Press.
- Dahl F. & Willebrand T. 2005. Natal dispersal, adult home ranges and site fidelity of mountain hares Lepus timidus in the boreal forest of Sweden. Wildlife Biology 11, 309–317.
- Davies N.B. & Houston A.I. 1983. Time allocation between territories and flocks and owner-satellite conflict in foraging pied wagtails, Motacilla alba. The Journal of Animal Ecology 52, 621–634.
- de Almeida Jácomo A.T., Kashivakura C.K., Ferro C., Furtado M.M., Astete S.P., Tôrres N.M., Sollmann R. & Silveira L. 2009. Home range and spatial organization of maned wolves in the Brazilian grasslands. Journal of Mammalogy 90, 150–157.
- Dietz J.M. 1984. Ecology and social organization of the maned wolf (Chrysocyon brachyurus). Smithsonian Contributions to Zoology 392. Washington D.C.: Smithsonian Institution Press.
- Dobson F.S., Way B.M. & Baudoin C. 2010. Spatial dynamics and the evolution of social monogamy in mammals. Behavioral Ecology 21, 747–752.
- Eberhardt L.E., Hanson W.C., Bengtson J.L., Garrott R.A. & Hanson E.E. 1982. Arctic fox home range characteristics in an oil-development area. The Journal of Wildlife Management 46, 183–190.
- Fay F.H. & Follmann E.H. 1982. The Arctic fox (Alopex lagopus). Species Account 3917. Juneau: US Department of Commerce, National Oceanic and Atmospheric Administration, Outer Continental Shelf Environmental Assessment Program.
- Fieberg J. & Kochanny C.O. 2005. Quantifying home-range overlap: the importance of the utilization distribution. Journal of Wildlife Management 69, 1346–1359.
- Frafjord K. & Prestrud P. 1992. Home range and movements of Arctic foxes Alopex lagopus in Svalbard. Polar Biology 12, 519–526.
- Fuglei E., Mustonen A.M. & Nieminen P. 2004. Effects of season, food deprivation and re-feeding on leptin, ghrelin and growth hormone in Arctic foxes (Alopex lagopus) on Svalbard, Norway. Journal of Comparative Physiology B 174, 157–162.
- Gagnon C.A. & Berteaux D. 2009. Integrating traditional ecological knowledge and ecological science: a question of scale. Ecology and Society 14, 19–58.
- Garrot R.A., Eberhardt L.E. & Hanson W.C. 1984. Arctic fox denning behavior in northern Alaska. Canadian Journal of Zoology 62, 1636–1640.
- Gauthier G., Berteaux D., Bêty J., Tarroux A., Therrien J.-F., Mckinnon L., Legagneux P. & Cadieux M.-C. 2011. The tundra food web of Bylot Island in a changing climate and the role of exchanges between ecosystems. Ecoscience 18, 223–235.
- Gauthier G., Bêty J., Cadieux M.-C., Legagneux P., Doiron M., Chevallier C., Lai S., Tarroux A. & Berteaux D. 2013. Long-term monitoring at multiple trophic levels suggests heterogeneity in responses to climate change in the Canadian Arctic tundra. Philosophical Transactions of the Royal Society B 368, article no. UNSP 20120482, doi:10.1098/rstb.2012.0482
- Geffen E. & Macdonald D.W. 1992. Small size and monogamy: spatial organization of Blanford’s foxes, Vulpes cana. Animal Behaviour 44, 1123–1130.
- Gruyer N., Gauthier G. & Berteaux D. 2008. Cyclic dynamics of sympatric lemming populations on Bylot Island, Nunavut, Canada. Canadian Journal of Zoology 86, 910–917.
- Gruyer N., Gauthier G. & Berteaux D. 2010. Demography of two lemming species on Bylot Island, Nunavut, Canada. Polar Biology 33, 725–736.
- Harris S., Cresswell W.J., Forde P.G., Trewhella W.J., Woollard T. & Wray S. 1990. Home-range analysis using radio-tracking data – a review of problems and techniques particularly as applied to the study of mammals. Mammal Review 20, 97–123.
- Hemson G., Johnson P., South A., Kenward R., Ripley R. & Macdonald D. 2005. Are kernels the mustard? Data from global positioning system (GPS) collars suggests problems for kernel home-range analyses with least-squares cross-validation. Journal of Animal Ecology 74, 455–463.
- Hennessy C.A., Dubach J. & Gehrt S.D. 2012. Long-term pair bonding and genetic evidence for monogamy among urban coyotes (Canis latrans). Journal of Mammalogy 93, 732–742.
- Kappeler P.M. 2013. Why male mammals are monogamous. Science 341, 469–470.
- Kitchen A.M., Gese E.M., Karki S.M. & Schauster E.R. 2005. Spatial ecology of swift fox social groups: from group formation to mate loss. Journal of Mammalogy 86, 547–554.
- Kitchen A.M., Gese E.M. & Schauster E.R. 2000. Long-term spatial stability of coyote (Canis latrans) home ranges in southeastern Colorado. Canadian Journal of Zoology 78, 458–464.
- Kleiman D.G. 1977. Monogamy in mammals. The Quarterly Review of Biology 52, 39–69.
- Kleiman D.G. 2011. Canid mating systems, social behavior, parental care and ontogeny: are they flexible? Behavior Genetics 41, 803–809.
- Kleiman D.G. 1981. Correlations among life history characteristics of mammalian species exhibiting two extreme forms of monogamy. In R. D. Alexander & D. W. Tinkle (eds.): Natural selection and social behavior. Pp. 332–344. New York: Chiron Press.
- Kleiman D.G. & Eisenberg J.F. 1973. Comparisons of canid and felid social systems from an evolutionary perspective. Animal Behaviour 21, 637–659.
- Krebs J.R. 1982. Territorial defence in the great tit (Parus major): do residents always win? Behavioral Ecology and Sociobiology 11, 185–194.
- Lai S., Bêty J. & Berteaux D. 2015. Spatio–temporal hotspots of satellite–tracked Arctic foxes reveal a large detection range in a mammalian predator. Movement Ecology 3, 1–10.
- Landa A., Strand O., Linnell J.D.C. & Skogland T. 1998. Home-range sizes and altitude selection for Arctic foxes and wolverines in an alpine environment. Canadian Journal of Zoology 76, 448–457.
- Lehner N.S. 2012. Arctic fox winter movement and diet in relation to industrial development on Alaska’s North Slope. M.Sc. thesis. University of Alaska Fairbanks.
- Lidicker W.Z. & Stenseth N.C. 1992. To disperse or not to disperse: who does it and why? In N.C. Stenseth & W.Z. Lidicker (eds.): Animal dispersal: small mammals as a model. Pp. 28–36. London: Chapman & Hall.
- Mech L. D., & Boitani L. 2010. Wolves: behavior, ecology, and conservation. Chicago: University of Chicago Press.
- Nicholson K.L., Ballard W.B., McGee B.K. & Whitlaw H.A. 2007. Dispersal and extraterritorial movements of swift foxes (Vulpes velox) in northwestern Texas. Western North American Naturalist 67, 102–108.
- Norén K., Carmichael L., Fuglei E., Eide N.E., Hersteinsson P. & Angerbjörn A. 2011. Pulses of movement across the sea ice: population connectivity and temporal genetic structure in the Arctic fox. Oecologia 166, 973–984.
- Pamperin N.J. (2008). Winter movements of Arctic foxes in northern Alaska measured by satellite telemetry. M.Sc. thesis, University of Alaska Fairbanks.
- Pamperin N.J., Follmann E.H. & Petersen B. 2006. Interspecific killing of an Arctic fox by a red fox at Prudhoe Bay, Fairbanks, Alaska. Arctic 59, 361–364.
- Parker C. & Ruttan L. 1988. The evolution of cooperative hunting. The American Naturalist 132, 159–198.
- Patterson B.R. & Messier F. 2001. Social organization and space use of coyotes in Eastern Canada relative to prey distribution and abundance. Journal of Mammalogy 82, 463–477.
- Pauw A. 2000. Parental care in a polygynous group of bat-eared foxes, Otocyon megalotis (Carnivora: canidae). African Zoology 35, 139–145.
- Poulle M.-L., Artois M. & Roeder J.-J. 1994. Dynamics of spatial relationships among members of a fox group (Vulpes vulpes: Mammalia: Carnivora). Journal of Zoology 233, 93–106.
- Prestrud P. 1991. Adaptations by the Arctic fox (Alopex lagopus) to the polar winter. Arctic 44, 132–138.
- Prestrud P. 1992. Denning and home-range characteristics of breeding Arctic foxes in Svalbard. Canadian Journal of Zoology 70, 1276–1283.
- Pusey A.E. 2005. Social systems. In J.J. Bolhuis & L.-A. Giraldeau (eds.): The behavior of animals: mechanisms, function and evolution. Pp. 313–341. Oxford: Blackwell Publishing.
- R Core Team 2013. R: A language and environment for statistical computing. Vienna: R Foundation for Statistical Computing.
- Ralls K., Cypher B. & Spiegel L.K. 2007. Social monogamy in kit foxes: formation, association, duration, and dissolution of mated pairs. Journal of Mammalogy 88, 1439–1446.
- Roth J.D. 2002. Temporal variability in Arctic fox diet as reflected in stable-carbon isotopes; the importance of sea ice. Oecologia 133, 70–77.
- Seaman D.E. & Powell R.A. 1996. An evaluation of the accuracy of kernel density estimators for home range analysis. Ecology 77, 2075–2085.
- Smith T.G. 1976. Predation of ringed seal pups (Phoca hispida) by the Arctic fox (Alopex lagopus). Canadian Journal of Zoology 54, 1610–1616.
- Soper J.D. 1944. The mammals of southern Baffin Island, Northwest Territories, Canada. Journal of Mammalogy 25, 221–254.
- Soulsbury C.D., Iossa G., Baker P.J., White P.C.L. & Harris S. 2011. Behavioral and spatial analysis of extraterritorial movements in red foxes (Vulpes vulpes). Journal of Mammalogy 92, 190–199.
- Strand O., Landa A., Linnell J.D.C., Zimmermann B. & Skogland T. 2000. Social organization and parental behavior in the Arctic fox. Journal of Mammalogy 81, 223–233.
- Switzer P.V. 1993. Site fidelity in predictable and unpredictable habitats. Evolutionary Ecology 7, 533–555.
- Tannerfeldt M. & Angerbjörn A. 1996. Life history strategies in a fluctuating environment: establishment and reproductive success in the Arctic fox. Ecography 19, 209–220.
- Tannerfeldt M., Elmhagen B. & Angerbjörn A. 2002. Exclusion by interference competition? The relationship between red and Arctic foxes. Oecologia 132, 213–220.
- Tarroux A., Bêty J., Gauthier G. & Berteaux D. 2012. The marine side of a terrestrial carnivore: intra-population variation in use of allochthonous resources by Arctic foxes. Plos ONE 7, e42427, doi: 10.1371/journal.pone.0042427
- Tsukada H. 1997. A division between foraging range and territory related to food distribution in the red fox. Journal of Ethology 15, 27–37.
- White P.J., Ralls K. & Siniff D.B. 2000. Nocturnal encounters between kit foxes. Journal of Mammalogy 81, 456–461.
- Worton B. 1989. Kernel methods for estimating the utilization distribution in home range studies. Ecology 70, 164–168.
- Wrigley R.E. & Hatch D.R.M. 1976. Arctic fox migrations in Manitoba. Arctic 29, 147–158.