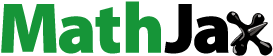
ABSTRACT
Slope stability is one of the most important topics of engineering geology with a background of more than 300 years. So far, various stability assessment techniques have been developed which include a range of simple evaluations, planar failure, limit state criteria, limit equilibrium analysis, numerical methods, hybrid and high-order approaches which are implemented in two-dimensional (2D) and three-dimensional (3D) space. In the meantime, limit equilibrium methods due to their simplicity, short analysis time, coupled with probabilistic and statistics functions to estimate the safety factor (F.S), probable slip surface, application on different failure mechanisms, and varied geological conditions has been received special attention from researchers. The presented paper provides a review to limit equilibrium methods used for discontinuous rock slope stability analyses with different failure mechanisms of natural and cut slopes. The article attempted to provide a systematic review for rock slope stability analysis outlook based on limit equilibrium approaches.
1. Introduction
The slope stability is considered as a most extensive description for soil and rock (or combination of both) slope masses under various failures (i.e. Akgün and Koçkar Citation2004; Bertolini Citation2010; Johari, Fazeli, and Javadi Citation2013, Citation2015, Citation2016, Citation2017; Zahri et al. Citation2016; Dahoua, Savenko, and Hadji Citation2017; El-Mekki, Hadji, and Fehdi Citation2017; Dahoua et al. Citation2018; Zeqiri et al. Citation2019; Saadoun et al. Citation2020; Fredj et al. Citation2020). These movements can cause damages under certain conditions (Bromhead Citation1992; Azarafza, Akgün, and Asghari-Kaljahi Citation2016; Yang and Liu Citation2018; Yamaguchi, Takeuchi, and Hamasaki Citation2018; Zhu and Yang Citation2018; Hou et al. Citation2019). These conditions can be classified related to geometry and geo-material status which determine the behavior and the critical slip surface expansion. shows various types of possible slip surfaces based on limit equilibrium analysis approaches which are directly depending on the geological conditions of the slope mass (Huang Citation2014). The existence of such a slip surface complicates the stability analysis and demands to consider more assumptions for covering the existing uncertainties. Therefore, the methods used in stability analysis have undergone a number of changes and improvements. In this task, the theoretical foundations and a literature review of stability analysis using the limit equilibrium method are presented herein which are successfully used for accurate description of slope failure mechanisms and stability analysis.
Figure 1. Various types of slip surfaces in slopes (Huang Citation2014).
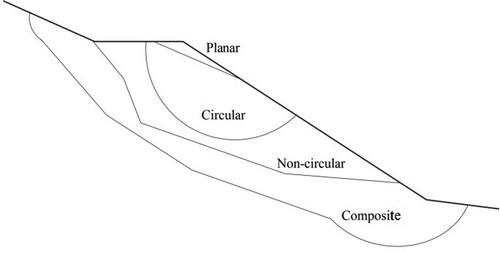
In general, the type of slope failure directly depends on the geological units and constituent geo-materials, geometric characteristics, stress–strain history, structural and tectonic conditions, geomorphology status, regional climate, seismic activity, water conditions (surface and underground), vegetation, weathering, drainage pattern, construction activities and special occasions (Qin and Chian Citation2018; Ersöz and Topal Citation2018; Li, Yang, and Li Citation2020; Yang et al. Citation2020; Ansari et al. Citation2021). According to the nature of the slippery mass, it can be stated that the slope failure mode in isotropic and homogeneous masses such as soils is mostly rotational (or massive failure). If there is a rigid surface or resistant layer, they occur in planar form (Abramson et al. Citation2001). Depending on the discontinuity network, joint orientation, infilling material, the rock masses undergo a wide range of failures such as wedge failure, planar failure, rotational failure, and toppling failure (Hoek Citation2006). Most commonly, failure occurrence in slopes can be classified into the main and secondary groups. The main group consists of wedge failure, toppling failure (rock slopes), planar failure, rotational failure (rock and earth slopes) and the second group consists of composite slips, special cases, glacial slips, etc. (Bromhead Citation1992).
Wedge failure: This failure type is one of the most common failure types in the discontinuous rock masses and it occurs based on the slip geometry in wedge form leading to tilt towards the slope face with a deviation degree less than the free face of the slope which slides along the intersection of the discontinuities downwards the slope(Zhou and Wang Citation2017).
Planar failure: This failure type is also a special case of slip that occurs because of the geological conditions and the geometry of the discontinuities, where slip occurs along a surface on the main discontinuity or a layer that is more related to the geotechnical conditions from the upper mass (Wyllie and Mah Citation2004).
Toppling failure: Occurs in rock slopes where the discontinuity orientation with a deviation degree close to the vertex and opposite to the excavation orientation/slope faces occurs. Because of the sliding gravity geometric center positioning, the blocks are extruded along the slope driven by gravity, which is referred to as toppling (Nikoobakht and Azarafza Citation2016).
Rotational failure: This type of slope movement is observed inhomogeneous and isotropic masses such as soils, severely discontinuous, and weak rock masses. The occurrence mechanism of rotational movement is to form a shear surface that engulfs the entire mass as a curvature or circle and may involve several smaller rotational slips in the mass body (Wyllie and Mah Citation2004).
The objective of this paper is to provide a review of limit equilibrium-based methods for discontinuous rock slope stability analyses (i.e. wedge, planar, toppling failure) and for weak rock or soil-like lithologies (i.e. mass failure) of natural slopes as well as of cut slopes (i.e. Azarafza, Feizi-Derakhshi, and Jeddi Citation2017; Bagheri Shendi and Azarafza Citation2018; Deng Citation2020; Shariati and Fereidooni Citation2021). The prepared article provides information about the limit equilibrium procedure to estimate the failure mechanisms in rock slopes. The main focuses on this matter are to provide principles and applications of stability analysis procedures especially, of the most recently developed methods named ‘Block theory’.
2. Slope stability analysis by limit equilibrium methods
Limit equilibrium analysis methods (LEMs) are one of the basic and old analytical approaches for slope stability analyses that are widely used in slope stability studies because of their simplicity, low complexity in the formulation, and less analysis time. LEMs based on massive analysis or slices investigate a possible slippery mass at the top of the assumed slip surface, and the polyhedral force vector closure or incurring moments in equilibrium state which are capable to be utilized in static and dynamic conditions for two-dimensional and three-dimensional space. If these polyhedral forces are closed and all assumptions/requirements are provided, this implies that the mass is in equilibrium and that the analysis is valid. The non-closure of the polyhedral forces/moments indicates the lack of balance or lack of satisfaction of some effective parameters in it.
(1)
(1)
The various equilibrium methods utilized are presented in . In this table, the basic failure mechanisms are considered and categorized for all states. Many of the LEMs provide close results in calculating the Factor of Safety (F.S) and the difference in the estimated values is usually less than 6% (Duncan 1996). The majority of the limit equilibrium approaches presented in this table use the Mohr-Coulomb relation to estimating the shear stress and resistance across the slip surface in all types of failures, where this criterion is considered as one of the most important failure criteria for stability analyses in geo-materials. As presented in , the limit equilibrium methods for various failure mechanisms are under the heading of wedge failure, planar failure, toppling failure, and rotational failure. This implies that various assumptions are introduced into the limit equilibrium stability analyses depending on the failure types.
Table 1. The most important limit equilibrium methods used in slope stability analysis.
Abramson et al. (Citation2001) have presented three methods, namely limit method, force equilibrium method, and moment equilibrium method to define the F.S coefficient in different slip surfaces based on a limit equilibrium where shear stress /resistance is considered as total resistance-stress or effective resistance-stress. In the force equilibrium method, the ratio of the resisting forces to the mobilized forces at the possible slip surface is investigated and in the moment method, through comparing the resistant moments to the overturning moments, the reliability of the slope is estimated. According to their achievements, these methods are capable to be applied to various types of slope failures or complex movements.
2.1. Wedge failure stability analysis
Wedge failure in specific geometric and geological conditions moves downward from the cairn along the intersection of two discontinuity planes where the discontinuity status with respect to the slope is oblique. This movement is due to the geometric three-dimensional structure and discontinuity mechanics in the rock mass. In , the geometrical conditions of a wedge failure are presented (Wyllie and Mah Citation2004). The values of the tendency αi and intersection angle ψi in the wedge sliding can be calculated as follows:
(2)
(2)
(3)
(3) where αA and αB are the dip directions, and ψA and ψB are the dips of the two joint planes. To define the confidence coefficient for the slipping wedge using the limit equilibrium method can be estimated:
(4)
(4)
Figure 2. Geometrical conditions in wedge failure analysis (Wyllie and Mah Citation2004).
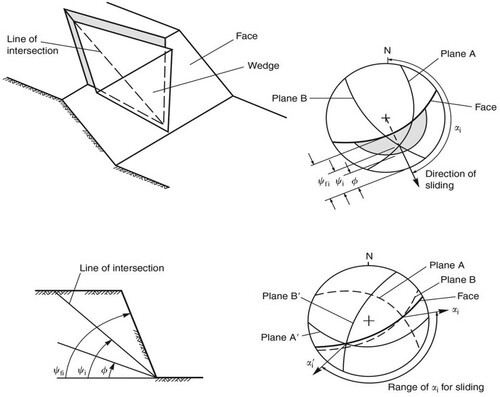
In the above relation, ϕ is friction; ξ is vectored distance from discontinuity to weight component, β and ψi are evaluated by Equation (3) as well as illustrated in .
Figure 3. Limit equilibrium analysis and force polyhedral in wedge failure (Wyllie and Mah Citation2004).
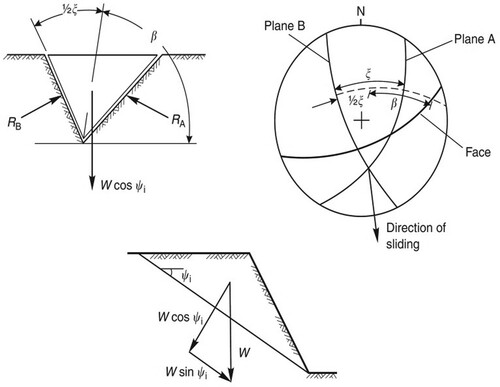
2.2. Planar failure stability analysis
Planar failure can be considered as a special case of wedge failure, in which the joint plane is aligned along 180 degrees. For planar failure to occur – as shown in (Wyllie and Mah Citation2004).
Figure 4. Geometrical conditions in planar failure analysis (Wyllie and Mah Citation2004).
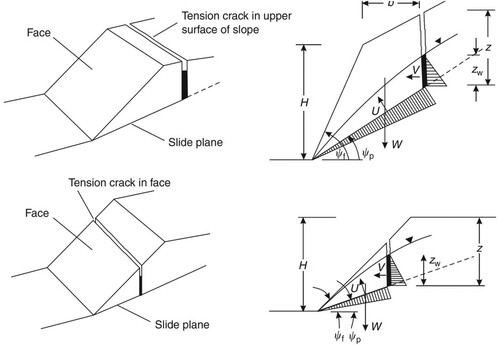
To analyze the stability and to calculate the confidence coefficient for the planar failure shown in , using the limit equilibrium approach, we have:
(5)
(5)
(6)
(6)
(7)
(7)
Figure 5. Limit equilibrium analysis and force polyhedral in planar failure (Wyllie and Mah Citation2004).
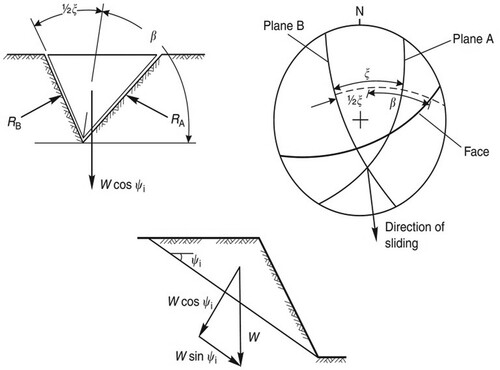
In the above relations, c is cohesion, A is the area, H is slope height, z is tension crack depth, U is the uplift water pressure, γw water specific weight. The other parameters can be estimated from .
2.3. Toppling failure stability analysis
Toppling failure occurs as a column or rock block rotation around its constant base. Since for toppling failure, the resistance and shear stress embattled at the base surface does not play any role in mass stability, it is not practically possible to evaluate this type of failure by limit equilibrium approaches. Hence, to evaluate this type of failure, a kinematic analysis method is usually used. Müller (Citation1964, Citation1968) was the first researcher who studied the occurrence of overturning phenomenon in the Vaiont Dam Lake. Ashby (Citation1971), for the first time, introduced this type of failure as ‘toppling’. Goodman and Bray (Citation1976) investigated various types of toppling failures, classified and presented mathematical solutions for themes (Sageseta, Sánchez, and Cañizal Citation2001). The various types of toppling failures are displayed as, flexural failure, block failure, and block-flexural failure were used to analyze different cases(i.e. Alejano, Gómez-Márquez, and Martínez-Alegría Citation2010, Citation2015, Citation2018; Zheng et al. Citation2018a, Citation2018b, Citation2018c, Citation2019). Block-toppling failure occurs in rock masses with high resistance and acute dip angles due to proper geometric conditions in the formation of suspended rock blocks moving outwards towards the bottom of the slope. Therefore, in such a case, there occurs slipping and rotation phenomenon in the rock columns (Amini, Majdi, and Aydan Citation2009; Aydan and Amini Citation2009).
In the flexural-toppling failure, during the flexure phenomenon, rock columns with acute dip angle separate from each other and break during bending downwards the slope. In the occurrence of such a slip, heel erosion, leakage, and wash down cause the slip trend to begin, and form deep tensile cracks as the flexural separation continues on the upstream side of the slope. At last, producing a rough surface, it slips downward of the slope (Amini, Majdi, and Aydan Citation2009). These types of failures provide favorable conditions for column separation and flexure phenomenon and crack diffusion in the section under the rocky columns (Amini, Ardestani, and Khosravi Citation2017). Block-flexural failure is presented as a combination of two states, in which the rocky columns are continuously under flexure, which, by several latitudinal gaps, cause their slip and movement on the gaps. This state of failure is very complicated and involves many uncertainties. Therefore, it is necessary to consider possible assumptions and to solve various differential equations in order to obtain an acceptable answer. Amini, Majdi, and Veshadi (Citation2012), considering two different states, have proposed a close approximation computational approach to analyze slippery single column and potential block-flexural toppling.
2.4. Rotational failure stability analysis
In soil masses or weak or weathered rock masses, it is not possible to determine the slipping surface by one or several discontinuities or gaps, and this slipping surface passes through the path that has the least resistance. Studies on such masses have shown that most of the occurred failures in such situations are rotational. Sliding surfaces with a spherical form that are controlled by the geological conditions and domain body are observed in the weathered masses with low cohesion/low friction angle and high erosion rate. Limit equilibrium analyses based on rotational failure are known to be the oldest and simplest approaches for instability analysis of the slopes which are implemented in two ways namely, massive analysis methods (common and final sliding surfaces are considered for the entire mass) and slice methods (the slippery mass is divided into a number of slices and evaluated). In and , examples of simplified slice methods are presented.
Figure 6. A rotational failure stability analysis using Bishop’s method (Hoek and Bray Citation1981; Wyllie and Mah Citation2004).
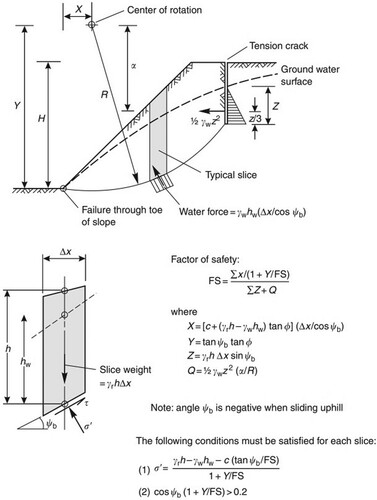
Figure 7. A rotational failure stability analysis using Janbu’s method (Hoek and Bray Citation1981; Wyllie and Mah Citation2004).
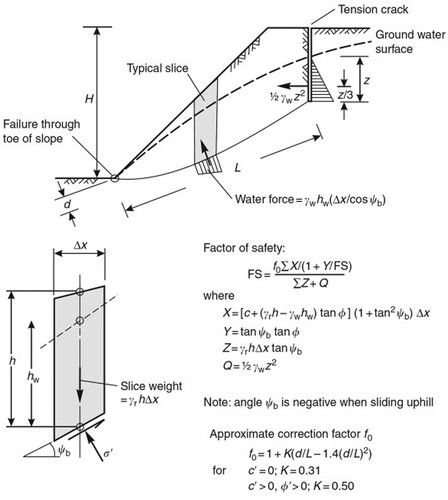
2.5. Composite failure stability analysis
Complex failures represent a combination of two or more different types of slope failure mechanisms that occur at different scales. If these instabilities are at a large scale, they can be considered as landslides (Highland and Bobrowsky Citation2008). Varnes (Citation1978) has classified the large-scale instabilities in soil or rock slopes as landslides that contain slides, flows, falls, toppling, creeps, debris, lateral separation, and complex movement types. Application of such classification for small-scale instabilities which are categorized in slope stability evaluations must be localized and modified for special cases. Nevertheless, in complex failure stability assessment, the recognition of the failure type is important to access the failure mechanisms (Kumar et al. Citation2021; Lee and Pietruszczak Citation2021). Goodman and Bray (Citation1976), Wyllie and Mah (Citation2004), Alejano, Gómez-Márquez, and Martínez-Alegría (Citation2010, Citation2011), Havaej et al. (Citation2014), and Sun et al. (Citation2019, Citation2020) have illustrated several types of complex slope failures. Although complex instabilities in slopes occur based on specific localized conditions, the geological characteristics, tectonic states, discontinuity network orientations, layered structure, and sedimentary formations are the main causes for complex failures. presents several identified complex failures that have occurred in discontinuous rock slopes. The complex instabilities must be simplified as a basic failure mechanism to evaluate the stability status. For example, Alejano, Gómez-Márquez, and Martínez-Alegría (Citation2010) used Bishop’s simplified method for circular (Bishop Citation1955) and Goodman and Bray (Citation1976) method for block toppling failure assessment to obtain the toppling-circular complex instability in claystone–sandstone sedimentary formation in Valencia, Spain. These procedures are applied for all types of complex failures in rock slopes.
Figure 8. Several types of complex failures in discontinuous rock slopes (Alejano, Gómez-Márquez, and Martínez-Alegría Citation2010, Citation2011; Havaej et al. Citation2014; Sun et al. Citation2019, Citation2020).
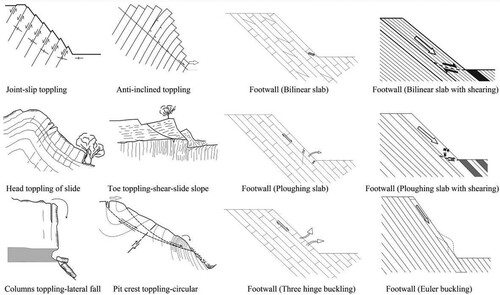
3. Generalized framework in limit equilibrium analyses
In the last century, over 10 remarkable methods have been developed based on the slice or massive analysis and the type of circular or general slip surface. The general shape of the slip surface in many of these analyses is as presented in . In order to analyze the exact stability by LSMs, specifying a generalized framework can improve the conditions and cover the uncertainties in slope stability analysis (Singh, Banka, and Verma Citation2019a, Citation2019b). Zhu, Lee, and Jiang (Citation2003) mentioned that in the two-dimensional stability analysis in the cross-sectional area, the slope is restricted by the ground surface (y = g[x]) and sliding surfaces (y = s[x]). Assuming that the coefficient is constant and equals the F.S for the entire sliding surface, the expansion of the slipping surface on the slider surface is determined as a function of the mass weight element in the static state W(x). Considering the validity of the Mohr-Coulomb failure criterion, it can be stated as:
(8)
(8)
Figure 9. Limit equilibrium stability analyses considerations: (a) graphical figure of massive failure, (b) force status that incur on a slice (Zhu, Lee, and Jiang Citation2003).
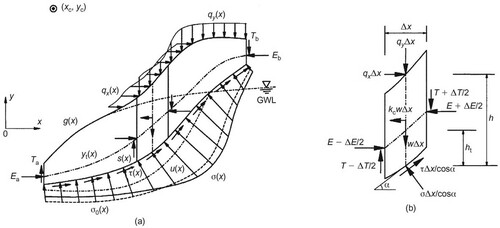
In this expression, σ(x) and τ(x) are normal and shear stresses, c(x) is cohesion, ϕ(x) is effective internal friction angle and u(x) is pore water pressure. Zhou and his colleagues proposed a parameter x as a probability distribution function that can be expanded for each piece. It should be noted that when the total stress is used, u(x) will be equal to zero and therefore, the total expression should be considered (Zhu, Lee, and Jiang Citation2003).
All of the slice analysis methods, except the ordinary method of slices, OMS (Fellenius Citation1936), use several relations to estimate the confidence coefficient. Hence, the accomplished stability analysis will be unpredictable at both sides of the equation, which requires assuming values of F.S and decreasing the uncertainties of the analytical equations (Zhu, Lee, and Jiang Citation2003).
However, Zhu and his colleagues provide the differential equation as presented in Equation (9) were used as generalized LEMs-based stability assessment (Zhu, Lee, and Jiang Citation2003):
(9)
(9)
The above equation can be generalized and extended for all limit equilibrium methods. These generalizations are proposed for a number of limit equilibrium methods as briefly presented in . Other methods such as Morgenstern and Price (Citation1965), Sarma (Citation1979), USACE (Citation2003) methods have been introduced to improve and reduce the uncertainties. Zhu, Lee, and Jiang (Citation2003) performed a comparison of the accuracy of the confidence coefficient values based on the type of slip surface in different ways. The last and the most updated limit equilibrium method introduced, which has been used extensively and successfully for rock slope stability analysis (for all forms of slip surfaces), is called ‘Block theory’. Block theory is widely considered by many researchers because of considering the rock mass geometric conditions and the possibility of probabilistic expansion and statistical function application (Wang et al. Citation2018; Azarafza et al. 2020b).
Table 2. Specification of F.S using the limit equilibrium method (Jie, Chen, and Zhang Citation1999; Paparo Citation2014; Agam et al. Citation2016).
The conventional limit equilibrium methods produce extra loops that make the assessment of the progressive instability possible, due to the lack of restrictions on the assumed surfaces to estimate slip parameters. This phenomenon is covered by Goodman’s theory. The most important advantages of this theory are restriction of the given slip surfaces, determination of the element key blocks, the definition of progressive failure, the possibility of expanding and employing statistical and probability functions, continuous three-dimensional and two-dimensional analysis (Goodman and Shi Citation1985). This theory is considered as a basis for a novel analysis for using the limit equilibrium method for the stability of slopes and blocks (Kulatilake et al. Citation2011).
4. Dimensional evaluations based on limit equilibrium methods
The main procedure of the LEMs methodologies are developed by two-dimensional aspects to evaluate the F.S and probable slide surface with the lowest F.S, but the actual condition of slopes (rock or earth) is a three-dimensional concept (Azarafza, Asghari-Kaljahi, and Akgün Citation2017b). In this regard, several scholars have attempted to introduce the generalized limit equilibrium models for three-dimensional slope stability analysis. Baligh and Azzouz (Citation1975), Hovland (Citation1977), Michalowski (Citation1980), Chen and Chameau (Citation1982), Ugai (Citation1985), Leshchinsky, Baker, and Silver (Citation1985), Leshchinsky and Baker (Citation1986), Hungr (Citation1987), Zhang (Citation1988), Hungr, Salgado, and Byrne (Citation1989), Leshchinsky and Huang (Citation1992), and Lam and Fredlund (Citation1993) are the pioneers of the three-dimensional LEMs based analysis on soil slopes. Lam and Fredlund (Citation1993) state that circular failure can be divided into several columns which represent a three-dimensional equivalent for slices that are normally used in circular failure stability assessment (see ). Afterward, the mass above the slip surface is divided into columns, and the forces acting on the various faces of each column are computed or assumed according to the LEMs methodologies (e.g. OMS, Bishop’s simplified, Janbu’s simplified). presented Lam and Fredlund’s work on the preparation of a three-dimensional slice method for earth slope circular failure.
Figure 10. Three-dimensional modelling of circular failure in earth slope (Lam and Fredlund Citation1993).
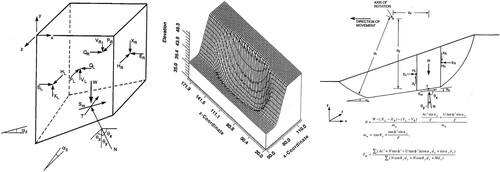
Huang and Tsai (Citation2000) have stated that two-dimensional slope stability analyses are typically considered to be more conservative than three-dimensional analyses in slope stability assessments, but they are more simplified to describe actual slope instability conditions. Huang and Tsai (Citation2000) used the basic work of Lam and Fredlund (Citation1993) to develop the F.S variation contours for asymmetrical slopes which were modified for toe-slide and deep-slide for a uniform soil slope in 2003 (Huang, Chen, and Chang Citation2003). Chen et al. (Citation2003) have presented a simplified method for stability assessment of wedge and circular failures. Li, Wang, and Deng (Citation2003) have presented a modification for three-dimensional slope stability. Albataineh (Citation2006) has established a review study on slope stability methods conducted on two and three-dimensional circular, wedge, and cylindrical failures based on previous tasks. Zheng (Citation2007), Zhou and Chen have used conservative LEMs (quasi-rigorous) for the three-dimensional stability assessment of slopes which is built on uniform circular failure mechanism evaluations by column progress.
After the introduction of Goodman’s Block Theory, the application of the three-dimensional concept to evaluate slope stability received much attention. Noroozi, Jalali, and Yarahmadi-Bafghi (Citation2011) and Azarafza, Asghari-Kaljahi, and Akgün (Citation2017b) used Block theory to develop procedures to simulate rock block geometry which led to the analysis of discontinuous rock slope stability. Wang, Sun, and Li (Citation2019) have utilized the LEMs methods for three-dimensional stability analysis which was mainly conducted on toe-failure, face-failure, and base-failure mechanisms involving uniform soil slopes based on the studies of Michalowski and Drescher (Citation2009). presents the failure mechanism concept by Wang and his colleagues. Zhou and Qin (Citation2020) have presented the lower bound limit coupled with block element method for three-dimensional slope stability analysis based on linear programming optimization technique which is applicable to both unique and non-unique direction models.
Figure 11. Three-dimensional stability models based on LEMs (Wang, Sun, and Li Citation2019).
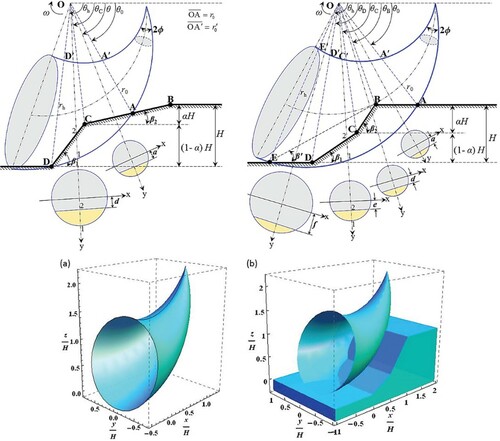
5. Block theory
Block theory or Goodman’s theory that was proposed by Goodman and Shi (Citation1985) is the newest limit equilibrium method that analyses the stability of rock blocks by two basic mechanisms of failure called ‘structural failure’ because of discontinuities and ‘stress-based failure’ because of the presence of high stresses. This theory by considering cairn geometrical status provides a logical relationship between cairn geometrical and critical failure potential in contact with released surfaces (during drilling operations) or rock outcrops (natural outcrop of the slope surface). The geometric position of the blocks, the emplacement of discontinuities in the space (which causes block division), spreading and continuity, and discontinuity spacing are considered parameters in assumptions of the analysis using Block theory. The movement of blocks is along the gapping geometric direction and is based on the resisting and driving forces on the discontinuity surface. This theory, by classifying block geometry, identifies the main causes of structural failure and finds the possible slip surface in the range of element (key) blocks. presents a classification of a rock slope by using Block theory.
Figure 12. Rock block classification based on Block theory (Kulatilake et al. Citation2011).
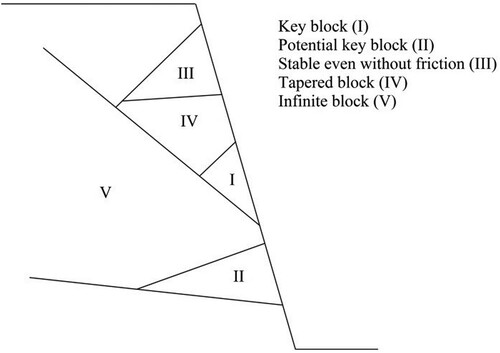
This theory by using the ‘finiteness theorem’ and ‘removability theorem’, attempts to analyze spatial geometry and defines line and plane equations/inequalities in space (discontinuities), which can easily convert the recorded information from polar coordinates (dip/dip direction of discontinuities) to Cartesian coordinates. The removability of the blocks is also defined based on the convex polyhedral geometry convergence in space. Using the finiteness theorem, joint pyramid, JP (the common spaces between half-spaces of discontinuity planes that form part of the block pyramid), the excavation pyramid, EP (a group of extraction half-spaces (excavation half-spaces) that are displaced to form a block pyramid), space pyramid, SP (complementary half-space of excavation pyramid) and block pyramid, BP (the factor for determining the convexity and concavity of blocks) can be defined (Azarafza, Asghari-Kaljahi, and Akgün Citation2017a, Citation2017b). These continuous equations give a mathematical definition for block geometry and its stability analysis.
This advantage has made the focus on this theorem very robust in stability analysis (Zhang et al. Citation2012; Wang et al. Citation2018). Goodman and Shi (Citation1985), by using the removability theorem, have expressed the tendency trend and motion of the block where a finite block is removable if it can displace in a particular direction without encountering the neighbor cairn. They also defined the conical block as a non-removable block that cannot be moved in any direction without encountering the neighbor cairn. Thus, the failure potential and slip in rock masses are very limited (Shi Citation1988). This restriction in the assumption of the initial slip surfaces (in the limit equilibrium stability analysis) provides the estimation of the results much faster, more accurately, and in less time than the available approaches (manual methods and common software). In the recent decades (i.e. specifically after the year 2000), Block theory has received widespread attention from various scholars because of its strong computational and analytical foundations. Ling (Citation2001) has presented the seismic/static application of this theory to different geotechnical structures. Um and Kulatilake (Citation2001) have utilized kinematic and block theory analyses for rock slope stability evaluations in three Gorges dam sites in China. Eberhardt (Citation2003) has conducted several analytical/numerical methodologies for rock slope stability assessments. Huang, Chen, and Chang (Citation2003) used the discontinuity network description of the key-block concept for identifying the potential key blocks in surficial excavation. Yarahmadi-Bafghi and Verdel (Citation2003) introduced the novel work based on Goodman’s theory named as ‘key-group method’. They also used probabilistic approaches to modify the key-group method (Yarahmadi-Bafghi and Verdel Citation2004). Pötsch and Schubert (Citation2004) have used a computer-based process referred to as JointMetriX3D to determine rock mass behavior and stability. Yarahmadi-Bafghi and Verdel (Citation2005) have conducted the Sarma limit-equilibrium procedure for re-ordering the key-group approach. Jimenez-Rodriguez, Sitar, and Chacón (Citation2006) presented the systematic quantitative methodology for the reliability analysis of rock slope stability based on key-block theory. Jimenez-Rodriguez and Sitar (Citation2007) applied Block theory for rock wedge stability analysis by system reliability approach given by the Monte-Carlo simulation technique. Haswanto and Abd-Ghani (Citation2008) performed a kinematic evaluation and Block theory for rock slope stability assessment in Fraser’s Hill Pahang, Malaysia. Haswanto and Abd-Ghani (Citation2010) completed the project on Fraser’s Hill Pahang, Malaysia by using several unstable slopes.
Kulatilake et al. (Citation2011) used the Block theory concept to evaluate fractured rock slope instabilities in the Yujian River dam site. Tian and Fu (Citation2011) used Goodman’s block theory for introducing the modified joints random probability model which is applied to analyze key block slide probability in discontinuous rock slope stability. Noroozi, Jalali, and Yarahmadi-Bafghi (Citation2011) presented the three dimensions key-group method which is developed by Yarahmadi-Bafghi and Verdel in Citation2003. Ma, Li, and Hong (Citation2013) applied a coupled procedure based on Block theory; kinematic vector analysis and discontinuous deformational analysis numerical method (DDA) for rock slope stability analysis. Greif and Vlčko (Citation2013) applied a key-block analyses system to evaluate the static stability of rock slopes for 45 cases in medieval castles in Slovakia. Wang and Ni (Citation2014) presented the geotechnical structure and model analysis (GeoSMA-3D) software for rock slope stability analysis. Nguyen and Phi (Citation2014) applied Block theory and probabilistic approaches for investigating the stability concerns in national road No.6, Vietnam. Sun, Zheng, and Huang (Citation2014) applied an indeterminate key-block method for static assessment in the Jinping-I hydropower station, China. Shi (Citation2014) presented the application of Block theory and the DDA technique for the reliability studies of slopes and underground powerhouses. Zheng et al. (Citation2014, Citation2015) developed a new formulation/computer code based on probabilistic Block theory analysis named PBTAC.
Li, Zhou, and Wang (Citation2016) proposed a framework for rock mass sliding blocks in surface cuts based on block-group and the Sarma integrated methodology. Azarafza, Asghari-Kaljahi, and Akgün (Citation2017a) applied the three-dimensional discontinuity geometrical modeling (3DDGM) algorithm for three-dimensional simulation of discontinuity network in rock slopes. Liu et al. (Citation2017) provided a new semi-deterministic Block theory method (NSDBT) based on digital photogrammetry techniques and applied it to the Changhe dam that is located in the Sichuan province, southwest China. Jia et al. (Citation2017) used discontinuity spatial distribution for underground geotechnical structures. They provided the extended key block theory in three dimensions which attempted to identify and classify the key-blocks. Azarafza, Asghari-Kaljahi, and Akgün (Citation2017b) presented the key block method based computational methodology for critical rock block recognition and evaluation of the sliding mechanisms.
Turanboy, Ülker, and Küçüksütçü (Citation2018) described a new method to model a discontinuity that intersects rock bodies. They used the k-means vector quantization cluster as an unsupervised machine learning technique to extract the three-dimensional discontinuity emplacements in surface rock cuts. He et al. (Citation2018) presented the couple method as a nodal variable-based discontinuous deformation analysis, named NDDA based on DDA and finite-element method (FEM) which was developed according to kinematic and block principles. Wang et al. (Citation2018) used a framework based on block theory multi-level rock slope characterization by the analytic hierarchy process (AHP) and GeoSMA-3D. Mohebbi et al. (Citation2019) developed an analytical approach based on the key-group method (named TFS_KGM) for the investigation of toppling free fall-sliding events. They stated that the computer evaluation results of this method significantly helped to describe the free fall toppling conditions in slope cuts. Mohammad et al. (Citation2020a) have presented a fuzzy logical decision-making method based on block theory to effectively determine discontinuous rock slope reliability under various wedge and planar slip scenarios. The method is capable to investigate the reliability (or stability-instability) degree to prepare the response operations without the extensive requirements. Mohammad et al. (Citation2020b) established the new methodology based on simplified semi-distinct element and block theory to estimate the stability conditions for main toppling failures (block, flexural and block-flexural types).
6. Rock slope stability analysis outlook by limit equilibrium approaches
Slope stability (earth or rock) is one of the most important issues in the geotechnical engineering field with a background of more than 300 years since the construction and development projects have always been faced with side-hill instabilities in different scales which were classified as slopes or landslides (Frasheri Citation2006). Generally, various approaches used for slope stability assessment can be classified as simple evaluations, planar failures, limit state criteria for limit equilibrium analysis, numerical methods, hybrid, and high-order approaches (Kliche Citation2018). In the meantime, limit equilibrium approaches due to their simplicity, rapid implementation; closed-form analysis, continuous access, easy assumptions, and providing multiple answers (e.g. F.S and probable sliding surface), the capability of being coupled or re-activated with other procedures are considered as the most flexible methodologies. Development of novel or hybrid procedures (rebuilt based on traditional progress) is conducted to achieve more accurate stability results, to cover more uncertainties, reduce the errors and establish generalized procedures for instability assessments as the main goal during the last decades. Application of the LEMs for stability analyses in the last recent 300 years indicate that these approaches are highly flexible to be integrated with high-level programming which is capable to cover more geomechanical features and geometrical properties. This advantage helps to reduce the uncertainties in the slope mass by considering the deterministic formulations.
7. Conclusions
These papers present a systematic review study of kinematical and limit equilibrium-based methods (LEMs) that are utilized for discontinuous rock stability analyses as well as for limit-equilibrium methods for heavy jointed rock or soil-like lithologies which are implemented in two-dimensional and three-dimensional spaces. During as decades, various approaches were developed by scholars to estimate the slope stabilities. To this end, the main methodologies of LEMs have been examined and their approaches through history have been described. The LEMs have been improved and modified to cover applications ranging from simplified circular failure analysis to high-order solutions of complex failures. Finally, the most advanced method of rock slope stability assessment that has been widely used by researchers nowadays and named as ‘Block theory’ that is based on strong computational and analytical foundations has been considered. In this regard, a brief overview of the new decade’s achievements on Goodman’s theory, which reflects modification of Block theory over time has been mentioned and discussed. As outlook of the LEMs, these approaches due to the flexibility can be coupled with the new procedure like numerical and hybrid methods and provide the simple and fast implementation for slope stability assessments. On the other hand, the application of new theories such as Block theory provides simple mathematical decryption for both structural and stress-field failures which is highly efficient in combination with numerical procedures like distinct of finite elements. In terms of the future scope for LEMs-based slope stability especially Block theory, it can be notified that Goodman’s theorem can be considered as the strongest approach to quantify the discontinuous rock slope and estimate the slope’s stability condition.
Disclosure statement
No potential conflict of interest was reported by the author(s).
Data availability statement
The data of the literature review for this study are available within the article.
References
- Abramson, Lee W., Thomas S. Lee, Sunil Sharma, and Glenn M. Boyce. 2001. Slope Stability Concepts: Slope Stabilisation and Stabilisation Methods. 2nd ed. Millburn, NJ: Wiley-Interscience.
- Agam, M. W., M. H. M. Hashim, M. I. Murad, and H. Zabidi. 2016. “Slope Sensitivity Analysis Using Spencer’s Method in Comparison with General Limit Equilibrium Method.” Procedia Chemistry 19: 651–658.
- Akgün, Haluk, and Mustafa K. Koçkar. 2004. “Design of Anchorage and Assessment of the Stability of Openings in Silty, Sandy Limestone: A Case Study in Turkey.” International Journal of Rock Mechanics and Mining Sciences 41: 37–49.
- Albataineh, Nermeen. 2006. Slope Stability Analysis Using 2D and 3D Methods. Ohio: University of Akron.
- Alejano, Leandro, C. Carranza-Torres, G. P. Giani, and J. Arzúa. 2015. “Study of the Stability Against Toppling of Rock Blocks with Rounded Edges Based on Analytical and Experimental Approaches.” Engineering Geology 195: 172–184.
- Alejano, Leandro, A. M. Ferrero, Pedro Ramírez-Oyanguren, and M. I. Álvarez Fernández. 2011. “Comparison of Limit-Equilibrium, Numerical and Physical Models of Wall Slope Stability.” International Journal of Rock Mechanics and Mining Sciences 48: 16–26.
- Alejano, Leandro, Iván Gómez-Márquez, and Roberto Martínez-Alegría. 2010. “Analysis of a Complex Toppling-Circular Slope Failure.” Engineering Geology 114: 93–104.
- Alejano, Leandro, Carlota Sánchez-Alonso, Ignacio Pérez-Rey, Javier Arzúa, E. Alonso, and Javier González. 2018. “Block Toppling Stability in the Case of Rock Blocks with Rounded Edges.” Engineering Geology 234: 192–203.
- Amini, Mehdi, Akbar Ardestani, and Mohammad H. Khosravi. 2017. “Stability Analysis of Slide-toe-Toppling Failure.” Engineering Geology 228: 82–96.
- Amini, Mehdi, Abbas Majdi, and Ömer Aydan. 2009. “Stability Analysis and the Stabilisation of Flexural Toppling Failure.” Rock Mechanics and Rock Engineering 42: 751–782.
- Amini, Mehdi, Abbas Majdi, and Mohammad Amin Veshadi. 2012. “Stability Analysis of Rock Slopes Against Block Flexure Toppling Failure.” Rock Mechanics and Rock Engineering 45: 519–532.
- Ansari, T., Ashutosh Kainthola, K. H. Singh, T. N. Singh, and M. Sazid. 2021. “Geotechnical and Micro-Structural Characteristics of Phyllite Derived Soil; Implications for Slope Stability, Lesser Himalaya, Uttarakhand, India.” Catena 196: 104906.
- Ashby, J. 1971. Sliding and Toppling Modes of Failure in Models and Jointed Rock Slopes. London: Imperial College, University of London.
- Aydan, Ömer, and Mehdi Amini. 2009. “An Experimental Study on Rock Slopes Against Flexural Toppling Failure Under Dynamic Loading and Some Theoretical Considerations for Its Stability Assessment.” Journal of Tokai University School of Marine Science and Technology 7: 25–40.
- Azarafza, Mohammad, Haluk Akgün, and Ebrahim Asghari-Kaljahi. 2016. “Assessment of Rock Slope Stability by Slope Mass Rating (SMR): A Case Study for the Gas Flare Site in Assalouyeh, South of Iran.” Geomechanics and Engineering 13: 571–584.
- Azarafza, Mohammad, Ebrahim Asghari-Kaljahi, and Haluk Akgün. 2017a. “Assessment of Discontinuous Rock Slope Stability with Block Theory and Numerical Modeling: A Case Study for the South Pars Gas Complex, Assalouyeh, Iran.” Environmental Earth Sciences 76 (11): 397.
- Azarafza, Mohammad, Ebrahim Asghari-Kaljahi, and Haluk Akgün. 2017b. “Numerical Modeling of Discontinuous Rock Slopes Utilizing the 3DDGM (Three-Dimensional Discontinuity Geometrical Modeling) Method.” Bulletin of Engineering Geology and the Environment 76: 989–1007.
- Azarafza, Mehdi, Mohammad-Reza Feizi-Derakhshi, and Ali Jeddi. 2017. “Blasting Pattern Optimization in Open-pit Mines by Using the Genetic Algorithm.” Journal of Geotechnical Geology 13: 75–81.
- Bagheri Shendi, Mousa, and Mehdi Azarafza. 2018. “A Case Study for Utilization of Image Processing in Jointed Network Detection in Open-pit Mining.” Journal of Geotechnical Geology 14: 197–202.
- Baligh, Mohsen M., and Amr S. Azzouz. 1975. “End Effects on Stability of Cohesive Slopes.” Journal of the Geotechnical Engineering Division 101: 1105–1117.
- Bertolini, Giovanni. 2010. “Large Earth Flows in Emilia-Romagna (Northern Apennines, Italy): Origin, Reactivation and Possible Hazard Assessment Strategies.” Zeitschrift der Deutschen Gesellschaft für Geowissenschaften 161: 139–162.
- Bishop, Alan W. 1955. “The use of Failure Circle in the Stability Analysis of Slopes.” Geotechnique 5: 7–17.
- Brady, Barry H. G., and E. T. Brown. 2005. Rock Mechanics for Underground Mining. Dordrecht, The Netherlands: Springer.
- Bromhead, Eddie. 1992. The Stability of Slopes. New York: Spon Press.
- Chen, R. H., and J. L. Chameau. 1982. “Three-dimensional Limit Equilibrium Analysis of Slopes.” Géotechnique 32: 31–40.
- Chen, Zuyu, Hongliang Mi, Faming Zhang, and Xiaogang Wang. 2003. "A Simplified Method for 3D Slope Stability Analysis." Canadian Geotechnical Journal 40: 675–683.
- Correia, R. M. 1988. “A limit equilibrium method of slope stability analysis.” Paper presented at the Proceedings of the 5th International Symposium on Landslides, Lausanne, Switzerland.
- Dahoua, Lamri, Viatcheslav Yakovitch Savenko, and Riheb Hadji. 2017. “GIS-based Technic for Roadside-Slope Stability Assessment: An Bivariate Approach for A1 East-West Highway, North Algeria.” Mining Science 24: 117–127.
- Dahoua, Lamri, Olena Usychenko, Viatcheslav Yakovitch Savenko, and Riheb Hadji. 2018. “Mathematical Approach for Estimating the Stability of Geotextile-Reinforced Embankments During an Earthquake.” Mining Science 25: 207–217.
- Deng, Dong-ping. 2020. “Limit Equilibrium Solution for the Rock Slope Stability Under the Coupling Effect of the Shear Dilatancy and Strain Softening.” International Journal of Rock Mechanics and Mining Sciences 134: 104421.
- Eberhardt, Erik. 2003. “Rock Slope Stability Analysis – Utilization of Advanced Numerical Techniques.” Earth and Ocean Sciences UBC: 1–41.
- El-Mekki, Abdelouahad, Riheb Hadji, and Chemseddine Fehdi. 2017. “Use of Slope Failures Inventory and Climatic Data for Landslide Susceptibility, Vulnerability, and Risk Mapping in Souk Ahras Region.” Mining Science 24: 237–249.
- Ersöz, Timur, and Tamer Topal. 2018. “Assessment of Rock Slope Stability with the Effects of Weathering and Excavation by Comparing Deterministic Methods and Slope Stability Probability Classification (SSPC).” Environmental Earth Sciences 77: 547.
- Fellenius, Wolmar. 1936. “Calculation of the Stability of Earth Dams.” Paper presented at the Proceedings of the 2nd Transaction Congress on Large Dams (ICOLD), Washington.
- Frasheri, Alfred. 2006. “Outlook on the Possibility for Slope Stability Evaluation According to Petrophysical and Integrated Geophysical Data.” Paper presented at the Proceedings of the 6th International Conference & Exposition on Petroleum Geophysics, Kolkata.
- Fredj, Mohamad, Hafsaoui Abdellah, Riheb Hadji, Boukarm Riadh, and Saadoun Abderrazak. 2020. “Back-analysis Study on Slope Instability in an Open Pit Mine (Algeria).” Scientific Bulletin of National Mining University 2: 24–29.
- Fredlund, Delwyn D., J. Krahn, and D. E. Pufahl. 1981. “The relationship between limit equilibrium slope stability methods.” Paper presented at the Proceedings of the International Conference on Soil Mechanics and Foundation Engineering, Stockholm, Sweden.
- Freitas, M. H., and R. J. Watters. 1973. “Some Field Examples of Toppling Failure.” Géotechnique 23: 495–514.
- Goodman, Richard E., and J. W. Bray. 1976. “Toppling of Rock Slopes.” Paper presented at the Proceedings of the ASCE Speciality Conference on Rock Engineering, Foundation and Slopes, Boulder, Colorado.
- Goodman, Richard E., and Gen-hua Shi. 1985. Block Theory and Its Application to Rock Engineering. Englewood Cliffs, NJ: Prentice-Hall.
- Greif, Vladimír, and Ján Vlčko. 2013. “Key Block Theory Application for Rock Slope Stability Analysis in the Foundations of Medieval Castles in Slovakia.” Journal of Cultural Heritage 14: 359–364.
- Haswanto, Adinata W., and R. Abd-Ghani. 2010. “Stability Analysis of Rock Slopes using Block Theory.” Paper presented at the Proceedings of the American Rock Mechanic Associations Annual Conference (ARMA), Salt Lake.
- Haswanto, Wangsa Adinata, and Rafek Abdul-Ghani. 2008. “Kinematic and Block Theory Applications to Rock Slope Stability Analysis at Fraser’s Hill, Pahang, Malaysia.” Electronic Journal of Geotechnical Engineering 13: 1–8.
- Havaej, Mohsen, Doug Stead, Erik Eberhardt, and Brendan R. Fisher. 2014. “Characterization of bi-Planar and Ploughing Failure Mechanisms in Footwall Slopes Using Numerical Modelling.” Engineering Geology 178: 109–120.
- He, Lei, Qian Tian, Zhiye Zhao, Xiaobao Zhao, Xiaobao Zhao, and Jian Zhao. 2018. “Rock Slope Stability and Stabilization Analysis Using the Coupled DDA and FEM Method: NDDA Approach.” International Journal of Geomechanics (ASCE) 18: 04018044.
- Highland, Lynn M., and Peter Bobrowsky. 2008. The Landslide Handbook—a Guide to Understanding Landslides. Reston: US Geological Survey Circular press.
- Hoek, Evert. 2006. “Practical Rock Engineering.” https://www.rocscience.com/documents/hoek/corner/Practical-Rock-Engineering-Full-Text.pdf.
- Hoek, Evert, Bray, Jonathan W. 1981. Rock Slope Engineering. 3rd ed. London: The Institution of Mining and Metallurgy, Maney Publishing.
- Hou, Chaoqun, Tingting Zhang, Zhibin Sun, Daniel Dias, and Jianfei Li. 2019. “Discretization Technique for Stability Analysis of Complex Slopes.” Geomechanics and Engineering 17: 227–236.
- Hovland, H. John. 1977. “Three-dimensional Slope Stability Analysis Method.” Journal of Geotechnical Engineering Division 103: 971–986.
- Huang, Yang H. 2014. Slope Stability Analysis by the Limit Equilibrium Method. Reston: ASCE Publications.
- Huang, Tien-Kuen, Jaw-Chern Chen, and Chein-Chi Chang. 2003. “Stability Analysis of Rock Slopes Using Block Theory.” Journal of the Chinese Institute of Engineers 26: 353–359.
- Huang, Ching-Chuan, and Cheng-Chen Tsai. 2000. “New Method for 3D and Asymmetrical Slope Stability Analysis.” Journal of Geotechnical & Geoenvironmental Engineering 126: 917–927.
- Hungr, Oldrich. 1987. “An Extension of Bishop’s Simplified Method of Slope Stability Analysis to Three Dimensions.” Géotechnique 37: 113–117.
- Hungr, Oldrich, Francisco Manuel Salgado, and P. M. Byrne. 1989. “Evaluation of a Three-Dimensional Method of Slope Stability Analysis.” Canadian Geotechnical Journal 26: 679–686.
- Janbu, N. 1954. “Application of Composite Slip Surface for Stability Analysis.” Paper presented at the Proceedings of the European Conference on Stability Analysis, Stockholm, Sweden.
- Janbu, N., L. Bjerrum, and B. Kjaernsli. 1956. Soil Mechanics Applied to Some Engineering Problems. Oslo: Norwegian Geotechnical Institute Publication.
- Jia, Chao, Yong Li, Mingyuan Lian, and Xiaoyong Zhou. 2017. “Jointed Surrounding Rock Mass Stability Analysis on an Underground Cavern in a Hydropower Station Based on the Extended Key Block Theory.” Energies 10: 563.
- Jie, M., C. Y. Chen, and J. J. Zhang. 1999. “General Stability of Two-Dimensional Slopes Based on Sarma’s Method.” International Journal for Numerical and Analytical Methods in Geomechanics 23: 413–426.
- Jimenez-Rodriguez, R., and Nicholas Sitar. 2007. “Rock Wedge Stability Analysis Using System Reliability Methods.” Rock Mechanics and Rock Engineering 40: 419–427.
- Jimenez-Rodriguez, R., Nicholas Sitar, and José Chacón. 2006. “System Reliability Approach to Rock Slope Stability.” International Journal of Rock Mechanics and Mining Sciences 43: 847–859.
- Johari, Ali, Ali Fazeli, and A. A. Javadi. 2013. “An Investigation Into Application of Jointly Distributed Random Variables Method in Reliability Assessment of Rock Slope Stability.” Computers and Geotechnics 47: 42–47.
- Johari, Ali, and A. Mehrabani Lari. 2016. “System Reliability Analysis of Rock Wedge Stability Considering Correlated Failure Modes Using Sequential Compounding Method.” International Journal of Rock Mechanics and Mining Sciences 82: 61–70.
- Johari, Ali, and A. Mehrabani Lari. 2017. “System Probabilistic Model of Rock Slope Stability Considering Correlated Failure Modes.” Computers and Geotechnics 81: 26–38.
- Johari, Ali, Mohammad Momeni, and A. A. Javadi. 2015. “An Analytical Solution for Reliability Assessment of Pseudo-Static Stability of Rock Slopes Using Jointly Distributed Random Variables Method.” Iranian Journal of Science and Technology 39 (C2): 351–363.
- Kliche, Charles A. 2018. Rock Slope Stability. 2nd ed. Englewood: Society for Mining, Metallurgy, and Exploration Press.
- Kulatilake, Pinnaduwa H. S. W., Liangqing Wang, Huiming Tang, and Ye Liang. 2011. “Evaluation of Rock Slope Stability for Yujian River Dam Site by Kinematic and Block Theory Analyses.” Computers and Geotechnics 38: 846–860.
- Kumar, Vinay, Avijit Burman, Navneet Himanshu, and Behrouz Gordan. 2021. “Rock Slope Stability Charts Based on Limit Equilibrium Method Incorporating Generalized Hoek–Brown Strength Criterion for Static and Seismic Conditions.” Environmental Earth Sciences 80: 212.
- Lam, Lkj, and Lkj Fredlund. 1993. “A General Limit Equilibrium Model for Three-Dimensional Slope Stability Analysis.” Canadian Geotechnical Journal 30: 905–919.
- Lee, Youn-Kyou, and S. Pietruszczak. 2021. “Limit Equilibrium Analysis Incorporating the Generalized Hoek–Brown Criterion.” Rock Mechanics and Rock Engineering 54: 4407–4418.
- Leshchinsky, Dov, and Rafael Baker. 1986. “Three-dimensional Slope Stability: End Effects.” Soils and Foundations 26: 98–110.
- Leshchinsky, Dov, Rafael Baker, and M. L. Silver. 1985. “Three-dimensional Analysis of Slope Stability.” International Journal for Numerical and Analytical Methods in Geomechanics 9: 199–223.
- Leshchinsky, Dov, and Ching-Chuan Huang. 1992. “Generalized Three-Dimensional Slope-Stability Analysis.” Journal of Geotechnical Engineering, v 118: 1748–1764.
- Li, T. L., Y. X. Wang, and H. K. Deng. 2003. “An Improved Method for Three-Dimensional Slope Stability Analysis.” Chinese Journal of Geotechnical Engineering 25: 611–614.
- Li, Zheng-Wei, Xiao-Li Yang, and Tian-Zheng Li. 2020. “Static and Seismic Stability Assessment of 3D Slopes with Cracks.” Engineering Geology 265: 105450.
- Li, Mingchao, Sibao Zhou, and Gang Wang. 2016. “3D Identification and Stability Analysis of key Surface Blocks of Rock Slope.” Transactions of Tianjin University 22: 317–323.
- Ling, Hoe I. 2001. “Recent Applications of Sliding Block Theory to Geotechnical Design.” Soil Dynamics and Earthquake Engineering 21: 189–197.
- Liu, Tiexin, Jianhui Deng, Jun Zheng, Lu Zheng, Zhenghu Zhang, and Hongchun Zheng. 2017. “A new Semi-Deterministic Block Theory Method with Digital Photogrammetry for Stability Analysis of a High Rock Slope in China.” Engineering Geology 216: 76–89.
- Lowe, J., and L. Karafiath. 1960. “Stability of Earth Dams Upon Drawdown.” Paper presented at the Proceedings of the 1st Pan American Conference on Soil Mechanic and Foundation Engineering, São Paulo, Mexico.
- Ma, Qiang, Guang Jie Li, Yuan Hong. 2013. “The Evaluation of Stability of Rock Mass Slope Based on the Block Theory and DDA Numerical.” Applied Mechanics and Materials 353–356: 1051–1056.
- Michalowski, R. L. 1980. “Three-dimensional Analysis of Locally Loaded Slopes.” Géotechnique 39: 27–38.
- Michalowski R. L., and A. Drescher. 2009. “Three-Dimensional Stability of Slopes And Excavations.” Géotechnique 59 (10): 839–850.
- Mohammad, Azarafza, Haluk Akgün, Mohammad-Reza Feizi-Derakhshi, Mehdi Azarafza, Jafar Rahnamarad, and Reza Derakhshani. 2020a. “Discontinuous Rock Slope Stability Analysis Under Blocky Structural Sliding by Fuzzy key-Block Analysis Method.” Heliyon 6: e03907.
- Mohammad, Azarafza, Haluk Akgün, Akbar Ghazifard, and Ebrahim Asghari-Kaljahi. 2020b. “Key-block Based Analytical Stability Method for Discontinuous Rock Slope Subjected to Toppling Failure.” Computers and Geotechnics 124: 103620.
- Mohebbi, Mohsen, Alireza Yarahmadi-Bafghi, Mohammad F. Marji, and Javad Gholamnejad. 2019. “Discontinued Rock Slope Analysis Through a new TFS-KGM Analytical Method.” Journal of Numerical Methods in Mining Engineering 18: 1–18.
- Morgenstern, N. R., and V. E. Price. 1965. “The Analysis of the Stability of General Slip Surfaces.” Geotechnique 15: 79–93.
- Müller, L. 1964. “The Rock Slide in the Vajont Valley.” Rock Mechanics and Engineering Geology 2: 148–212.
- Müller, L. 1968. “New Considerations on the Vaiont Slide.” Rock Mechanics and Engineering Geology 6: 1–91.
- Nguyen, QuocPhi, and TruongThanh Phi. 2014. “Rock Slope Stability Analysis using Block Theory and Probabilistic Approach: An Application at National Road No.6, Vietnam.” Paper presented at the Proceedings of the International Conference on GeoInformatics for Spatial-Infrastructure Development in Earth & Allied Sciences, Danang, Vietnam.
- Nikoobakht, Shahrzad, and Mohammad Azarafza. 2016. “Stability Analysis and Numerical Modelling of Toppling Failure of Discontinuous Rock Slope (A Case Study).” Journal of Geotechnical Geology 12: 169–178.
- Nonveiller, Erwin. 1965. “The Stability Analysis of Slopes with a Slide Surface of General Shape.” Paper presented at the Proceedings of the 6th International Conference on Soil Mechanics and Foundation Engineering, Montreal, Canada.
- Noroozi, Mehdi, Seyed Esmaeil Jalali, and Alireza Yarahmadi-Bafghi. 2011. “3D key-Group Method for Slope Stability Analysis.” International Journal for Numerical and Analytical Methods in Geomechanics 36: 1780–1792.
- Paparo, Maria Ausilia. 2014. Evaluation of Slope Stability Under Water and Seismic Load Through the Minimum Lithostatic Deviation Method. Bologna: University of Bologna.
- Pötsch, Markus, and Wulf Schubert. 2004. “Computer Based Stability Analysis of Rock Slopes in a Blocky Rock Mass.” Paper presented at the Proceedings of the Eurock 2004 & 53rd Geomechanics Colloquy, Schubert, Austria.
- Qin, C. B., and S. C. Chian. 2018. “Kinematic Analysis of Seismic Slope Stability with a Discretisation Technique and Pseudo-Dynamic Approach: A New Perspective.” Géotechnique 68 (6): 492–503.
- Saadoun, Abderrazak, Isik Yilmaz, Abdallah Hafsaoui, Riheb Hadji, Mohamed Fredj, Riadh Boukarm, and Radouane Nakache. 2020. “Slope Stability Study in Quarries by Different Approaches: Case Chouf Amar Quarry, Algeria.” IOP Conference Series: Materials Science and Engineering 960: 042026.
- Sageseta, C., J. M. Sánchez, and J. Cañizal. 2001. “A General Analytical Solution for the Required Anchor Force in Rock Slopes with Toppling Failure.” International Journal of Rock Mechanics and Mining Sciences 38: 421–435.
- Sarma, Sarada K. 1973. “Stability Analysis of Embankments and Slopes.” Geotechnique 23: 423–433.
- Sarma, Sarada K. 1979. “Stability Analysis of Embankments and Slopes.” Journal of the Geotechnical Engineering Division (ASCE) 105: 1511–1524.
- Shariati, Mohsen, and Davood Fereidooni. 2021. “Rock Slope Stability Evaluation Using Kinematic and Kinetic Methods Along the Kamyaran-Marivan Road, West of Iran.” Journal of Mountain Science 18 (3): 779–793.
- Shi, Gen-hua. 1988. Discontinuous Deformation Analysis: A New Numerical Method for the Statics and Dynamics of Block Systems. University of California, Berkeley.
- Shi, G. H. 2014. “Application of Discontinuous Deformation Analysis on Stability Analysis of Slopes and Underground Power Houses.” Geomechanics and Geoengineering 9: 80–96.
- Singh, Jayraj, Haider Banka, and Amit Kumar Verma. 2019a. “A BBO-Based Algorithm for Slope Stability Analysis by Locating Critical Failure Surface.” Neural Computing and Applications 31: 6401–6418.
- Singh, Jayraj, Haider Banka, and Amit Kumar Verma. 2019b. “Locating Critical Failure Surface Using Meta-Heuristic Approaches: A Comparative Assessment.” Arabian Journal of Geosciences 12: 307.
- Spencer, E. 1967. “A Method of Analysis of the Stability of Embankments Assuming Parallel Inter-Slice Forces.” Geotechnique 17: 11–26.
- Sun, Chaoyi, Congxin Chen, Yun Zheng, and Kaizong Xia. 2020. “A Limit Equilibrium Analysis of the Stability of a Footwall Slope with Respect to bi-Planar Failure.” International Journal of Geomechanics 20: 04019137.
- Sun, Chaoyi, Congxin Chen, Yun Zheng, Wei Zhang, and Feng Liu. 2019. “Numerical and Theoretical Study of bi-Planar Failure in Footwall Slopes.” Engineering Geology 260: 105234.
- Sun, Guanhua, Hong Zheng, and Yaoying Huang. 2014. “Stability Analysis of Statically Indeterminate Blocks in key Block Theory and Application to Rock Slope in Jinping-I Hydropower Station.” Engineering Geology 186: 57–67.
- Tian, Qingyan, and Helin Fu. 2011. “Block Theory Rock Joint Length Modifying and Slope Stability Analysis.” Paper presented at the Proceedings of the GeoHunan International Conference, Hunan, China.
- Turanboy, A., E. Ülker, and C. B. Küçüksütçü. 2018. “A new Approach for Assessing Stability of Rock Slopes Considering Centroids of Weak Zones.” Journal of Mining and the Environment 9: 1–18.
- Ugai, Keizo. 1985. “Three-dimensional Stability Analysis of Vertical Cohesive Slopes.” Soils and Foundations 2: 41–48.
- Um, Jeongi-Gi, and Pinnaduwa H. S. W. Kulatilake. 2001. “Kinematic and Block Theory Analyses for Shiplock Slopes of the Three Gorges Dam Site in China.” Geotechnical and Geological Engineering 19: 21–42.
- US Army Corps of Engineers, USACE. 2003. Slope Stability: Engineering and Design. US Army Corps of Engineers Publications, Washington.
- Varnes, David J. 1978. Slope Movement Types and Processes: Landslide Analysis and Control. Washington: Transportation Research Board, National Academy Sciences.
- Wang, Shuhong, and Pengpeng Ni. 2014. “Application of Block Theory Modeling on Spatial Block Topological Identification to Rock Slope Stability Analysis.” International Journal of Computational Methods 11: 1350044.
- Wang, Long, De’an Sun, and Lin Li. 2019. “Three-dimensional Stability of Compound Slope Using Limit Analysis Method.” Canadian Geotechnical Journal 56: 116–125.
- Wang, Feili, Shuhong Wang, Muhammad Zaffar Hashmi, and Zhanguo Xiu. 2018. “The Characterization of Rock Slope Stability Using key Blocks Within the Framework of GeoSMA-3D.” Bulletin of Engineering Geology and the Environment 77: 1405–1420.
- Wyllie, Duncan C., and Chris Mah. 2004. Rock Slope Engineering. 4th ed. London: Spon Press.
- Yamaguchi, Kiyomichi, Norio Takeuchi, and Eisaku Hamasaki. 2018. “Three-dimensional Simplified Slope Stability Analysis by Hybrid-Type Penalty Method.” Geomechanics and Engineering 15: 947–955.
- Yang, Jianhua, Jinhao Dai, Chi Yao, Shuihua Jiang, Chuangbing Zhou, and Qinghui Jiang. 2020. “Estimation of Rock Mass Properties in Excavation Damage Zones of Rock Slopes Based on the Hoek-Brown Criterion and Acoustic Testing.” International Journal of Rock Mechanics and Mining Sciences 126: 104192.
- Yang, X. L., and Z. A. Liu. 2018. “Reliability Analysis of Three-Dimensional Rock Slope.” Geomechanics and Engineering 15: 1183–1191.
- Yarahmadi-Bafghi, Alireza, and Thierry Verdel. 2003. “The Key-Group Method.” International Journal for Numerical and Analytical Methods in Geomechanics 27: 495–511.
- Yarahmadi-Bafghi, Alireza, and Thierry Verdel. 2004. “The Probabilistic Key-Group Method.” International Journal for Numerical and Analytical Methods in Geomechanics 28: 899–917.
- Yarahmadi-Bafghi, Alireza, and Thierry Verdel. 2005. “Sarma-Based Key-Group Method for Rock Slope Reliability Analyses.” International Journal for Numerical and Analytical Methods in Geomechanics 29: 1019–1043.
- Zahri, Farid, Mohammed Laid Boukelloul, Riheb Hadji, and Kourichi Talhi. 2016. “Slope Stability Analysis In Open Pit Mines Of Jebel Ustar Career, Ne Algeria – A Multi-Steps Approach.” Mining Science 23: 137––1146.
- Zeqiri, Rafet, Riheb Hadji, Zighmi Karim, Guesmi Younes, Rania Boudjellal, and Mahleb Anissa. 2019. “Analysis of Safety Factor of Security Plates in the Mine “Trepça” Stantërg.” Mining Science 26: 221–229.
- Zhang, Xing. 1988. “Three-dimensional Stability Analysis of Concave Slopes in Plan View.” Journal of Geotechnical Engineering 114: 658–671.
- Zhang, Yingbin, Guangqi Chen, Jian Wu, Lu Zheng, and Xiaoying Zhuang. 2012. “Numerical Simulation of Seismic Slope Stability Analysis Based on Tension-Shear Failure Mechanism.” SEAGS-AGSSEA Journal 43: 18–28.
- Zhang, Guangcheng, Fei Wang, Hu Zhang, Huiming Tang, Xianghui Li, and Yuan Zhong. 2018c. “New Stability Calculation Method for Rock Slopes Subject to Flexural Toppling Failure.” International Journal of Rock Mechanics and Mining Sciences 106: 319–328.
- Zheng, Hong. 2007. “A Rigorous Three-Dimensional Limit Equilibrium Method.” Chinese Journal of Rock Mechanic Engineering 26: 1529–1537.
- Zheng, Yun, Congxin Chen, Tingting Liu, Kaizong Xia, and Xiumin Liu. 2018a. “Stability Analysis of Rock Slopes Against Sliding or Flexural-Toppling Failure.” Bulletin of Engineering Geology and the Environment 77: 1383–1403.
- Zheng, Y., C. Chen, T. Liu, H. Zhang, and C. Sun. 2019. “Theoretical and Numerical Study on the Block-Flexure Toppling Failure of Rock Slopes.” Engineering Geology 263: 105309.
- Zheng, Yun, Congxin Chen, Tingting Liu, Haina Zhang, Kaizong Xia, and Feng Liu. 2018b. “Study on the Mechanisms of Flexural Toppling Failure in Anti-Inclined Rock Slopes Using Numerical and Limit Equilibrium Models.” Engineering Geology 237: 116–128.
- Zheng, Jun, H. S. W. Pinnaduwa, B. Biao Shu, Taghi Sherizadeh, and Jh Deng. 2014. “Probabilistic Block Theory Analysis for a Rock Slope at an Open pit Mine in USA.” Computers and Geotechnics 61: 254–265.
- Zheng, Jun, H. S. W. Pinnaduwa, and Jh Deng. 2015. “Development of a Probabilistic Block Theory Analysis Procedure and Its Application to a Rock Slope at a Hydropower Station in China.” Engineering Geology 188: 110–125.
- Zhou, Jian-feng, and Chang-bing Qin. 2020. “A Novel Procedure for 3D Slope Stability Analysis: Lower Bound Limit Analysis Coupled with Block Element Method.” Bulletin of Engineering Geology and the Environment 79: 1815–1829.
- Zhou, Jianfeng, and Junxing Wang. 2017. “Lower Bound Limit Analysis of Wedge Stability Using Block Element Method.” Computers and Geotechnics 86: 120–128.
- Zhu, D. Y., C. F. Lee, and H. D. Jiang. 2003. “Generalised Framework of Limit Equilibrium Methods for Slope Stability Analysis.” Geotechnique 53: 377–395.
- Zhu, J. Q., and X. L. Yang. 2018. “Probabilistic Stability Analysis of Rock Slopes with Cracks.” Geomechanics and Engineering 16: 655–667.