Abstract
Background: In search for a mechanistic explanation of climatic treeline formation, permafrost sites in montane forests provide ideal conditions to compare soil temperature impacts on tree growth, as dwarfed trees on pockets of cold soil grow in juxtaposition with tall forest on markedly warmer soil.
Aim: The carbon supply status of dwarfed Norway spruce trees on a low elevation permafrost site in Switzerland was investigated by analysing their non-structural carbon pools. According to the sink-limitation hypothesis of alpine treeline formation, the low soil temperatures over permafrost should restrict growth processes more than photosynthesis, causing an oversupply of carbon.
Methods: Needles and sapwood of mature spruces were sampled from dwarfed trees over permafrost and from tall trees at an adjacent forest on several dates across the growing season. All tissues were analysed for non-structural carbohydrates (NSC). Branch wood was additionally analysed for lipids (acylglycerols).
Results: Comparing NSC of dwarfed trees with that of surrounding tall trees, indicated higher concentrations in trees over permafrost throughout the season, except for needles sampled in May, which likely reflects the delayed start of the growing season on permafrost. On average, NSC concentrations were about 10% higher in needles, 25% higher in stem wood and 40% higher in branch wood in dwarfed compared to tall trees. Low lipid concentrations in branch wood (< 1% d.m.) suggest a minor role for lipids as carbon reserves in spruce. Nevertheless, lipid concentrations were about 30% higher in dwarfed than in tall trees.
Conclusions: The enrichment with carbon reserves of dwarfed spruce on permafrost confirms previous findings from climatic alpine treelines. The results are therefore in line with the sink-limitation hypothesis of treeline formation, and suggest that the same mechanism applies to situations where only roots experience low temperatures.
Introduction
Permafrost soils are widely distributed in regions where sub-zero annual mean temperatures prevent thawing of the ground below the uppermost soil horizons (Davis Citation2001). Thus, permafrost is generally associated with arctic and boreal biomes, or high altitude sites within the nival and alpine belts of mountain ranges. However, peculiar situations in mountainous terrain (e.g. year-round shaded and aerated block fields) can lead to the formation of small-scale permafrost pockets at lower elevations within montane forests (Wakonigg Citation1996). In Europe, several such montane permafrost sites are known below 2000 m a.s.l. in the Alps, the Jura Mts in Switzerland and in the Black Forest, Germany (Hölzel Citation1963; Furrer Citation1966; Schindler et al. Citation1976; Wegmüller and Wegmüller Citation1985; Molenda and Gude Citation2000; Rist Citation2002; Delaloye et al. Citation2003; Körner and Hoch Citation2006). These sites, surrounded by tall montane forest, are either completely treeless or trees form dwarfed stands (‘Krummholz’), mirroring the situation found at alpine treelines.
The current study was performed at a montane permafrost site at Creux du Van in the Swiss Jura Mountains. The site is ideal to test low temperature related effects on tree growth and physiology because its extraordinarily low elevation about 800 m of altitude below the natural alpine treeline allows a comparison between dwarfed Norway spruce Picea abies (L.) KARST. Trees with tall individuals growing only a few meters from the permafrost lenses. Remarkably, the mean growing season soil temperature at 10 cm depth over permafrost is around 6.5 °C at this site (6.7 °C measured for one growing season, Körner and Hoch Citation2006), which matches the average mean growing season soil temperature calculated from 46 treeline sites across the globe (6.7 ± 0.8 °C, Körner and Paulsen Citation2004). Hence, questions related to the physiological causes of treeline formation can be addressed by comparing the dwarfed trees over permafrost with the adjacent tall trees, avoiding the disadvantage of larger distances between forest and treeline, such as in alpine treeline transects, which bear the risk of confounding site inherent effects apart from temperature. Further, trees over permafrost and the tall trees around it experience similar air temperatures. Hence, these conditions represent a sort of natural experiment perfectly suited to test the hypothesis that low soil temperatures alone can limit the growth of aboveground tree parts.
The physiological mechanisms behind alpine treeline formation have been discussed for more than a century. For an extensive review on the numerous hypotheses of treeline formation see Körner (Citation1998) and Holtmeier (Citation2003). Körner and Paulsen (Citation2004) have highlighted similar temperature regimes during the growing season at the majority of alpine treelines across the world, including tropical and sub-tropical treelines that largely lack any temperature-associated seasonality. The worldwide similarity of growing season mean temperatures (5.5–7.5 °C) at otherwise very different conditions (e.g. growing season length, air pressure, irradiance, precipitation, soil fertility), suggests a direct impact of low temperatures during the growing season to be primarily decisive for the formation of alpine treelines. The exact physiological mechanism behind this low-temperature induced decline of tree growth, however, is still unknown.
According to previous studies, it is likely that the low temperatures around 6 °C at treeline first affect growth processes (meristem activities), while carbon gain via photosynthesis continues at more than sufficient rates (Grace et al. Citation2002; Körner Citation2006). This explanation for treeline formation, which Körner (Citation1998) has referred to as the ‘sink-limitation hypothesis’, implies a higher carbon supply status of trees at the treeline than at lower elevations (warmer temperatures). The balance between carbon source- and sink-activities can be assessed by analysing the size of a plant's carbon storage pool (e.g. starch, low molecular weight sugars, lipids), since the concentrations of non-structural carbon compounds are (within a certain range) sensitive to activities of carbon in and output (Chapin et al. Citation1990; Dickson Citation1991). Carbon limited trees should thus show lower concentrations of non-structural carbon compounds than trees well supplied with carbon. On the other hand, situations of surplus carbon supply (like at sink-limitation of growth) are likely to increase the non-structural carbon pool of trees. To date, several studies that investigated non-structural carbon concentrations of trees at natural treelines in different climatic zones have shown a trend of increasing carbon reserve concentrations towards the upper end of tree growth. Those findings clearly indicate that carbon shortage of treeline trees is unlikely, but rather support the sink-limitation hypothesis (Hoch et al. Citation2002; Hoch and Körner Citation2003, Citation2005; Piper et al. Citation2006; Shi et al. Citation2006, Citation2008).
In a previous study at Creux du Van, we reported the extraordinary climatic conditions at this low elevation permafrost site (Körner and Hoch Citation2006). Within the same study we also demonstrated that although only tree roots and not the crowns are exposed to low temperatures on permafrost, the aboveground growth of Norway spruce is highly restricted by the cool soil conditions alone, leading to dwarfed tree growth. Here, I investigate the carbon supply status of spruce at the permafrost sites in comparison with adjacent tall trees by analysing non-structural carbon concentrations in different tissues over the growing season. In accordance with the situations found at alpine treelines, I hypothesise that in line with the sink-limitation hypothesis, dwarfed trees over permafrost should have higher carbon reserve pools, than trees growing in normal montane forest around the permafrost lenses.
Material and methods
Study site
The study area is situated at around 1200 m a.s.l. at the base of a 100–150 m horseshoe-shaped escarpment called Creux du Van (46° 56 ' N, 6° 44 ' E; summit at 1463 m) in the Jura Mountains, near the city of Neuchâtel in western Switzerland. The coarse debris and rocks eroded from the escarpment form a steep block field at the base of the formation (). Since the escarpment opens only to the east to north-east, a significant part of the southern half of the area below this natural ‘amphitheatre’ never receives direct sunlight during the entire year. The peculiar situation of aerated scree, which is shaded for most of the year, yields a ventilation system that results in the formation of permafrost pockets at the lower half of the block field (Wakonigg Citation1996). The net of hollow spaces within the scree obviously acts like funnels through which advective cold air drainage during winter leads to overcooling of the interior of the slope. For a detailed description of the complex processes that enable the formation of permafrost at Creux du Van see Delaloye and Reynard (Citation2001) and Delaloye et al. (Citation2003). The relatively small ice lenses (30–60 m in diameter) have a thickness of about 15–20 m overlain with approximately 2–3 m of coarse debris and rocks, which are covered with a shallow, peaty soil and raw-humus layer (Delaloye et al. Citation2003). The arctic conditions imposed by the permafrost pockets are mirrored in the vegetation with Betula pubescens Ehrh., dwarfed Picea abies and sporadic Pinus sylvestris L. trees and a ground cover dominated by Vaccinium myrtillus L. and Sphagnum mosses (Richard Citation1961).
Figure 1 Permafrost pockets on the scree slope of the Creux du Van escarpment in the Swiss Jura Mts. The permafrost sites with dwarfed trees (encircled) are located at c. 1200 m a.s.l. within a tall montane spruce forest.
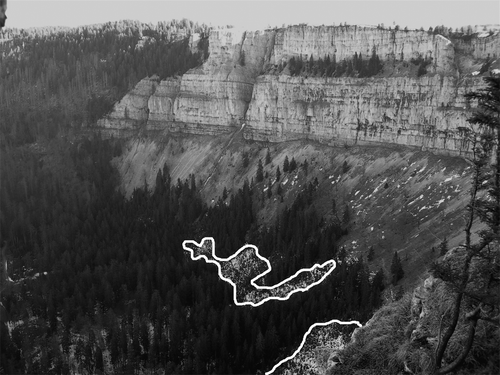
Delaloye and Reynard (Citation2001) measured an annual mean air temperature of 5.5 °C directly over a permafrost lens at 1200 m, with an average air temperature of 12.1 °C for the five warmest months (May–September). The relatively high annual precipitation of about 1600 mm (Delaloye and Reynard Citation2001) causes year-round moist soil conditions, also mirrored by the abundant distribution of Sphagnum mosses over permafrost. The c. 150 d growing season lasts from mid-May to late September (Körner and Hoch Citation2006). While permafrost reduces the mean growing season soil temperature by about 2 °C in comparison with the reference tall forest site, mean air temperatures across the growing season are even slightly higher at the dwarf forest over permafrost ().
Table 1. Soil and air temperatures during the growing season (May–September), tree age, annual tree ring width and annual lateral shoot growth (mean from 1999–2003) of P. abies at the permafrost and the reference forest sites (data from Körner and Hoch Citation2006). Temperatures were measured for one season only. Growth parameters are means of four to 10 individual trees ± standard error (see Körner and Hoch Citation2006 for details). Different letters indicate significant differences between sites at the P < 0.05 level by Student's t-test
The study site is situated within the mid-montane forest belt at the transition zone between mixed beech (Fagus sylvatica L.)/Norway spruce forests at lower altitudes and spruce dominated stands at higher altitudes. While spruce is dwarfed on permafrost and seldom reaches more than 3 m in height, trees of the same species grow tall (> 20 m) in the immediate surrounding of the permafrost pockets ( and Körner and Hoch Citation2006). The cold soil conditions over permafrost severely restrict annual tree ring and shoot increment ().
Sampling and biometric measurements
Four-year-old, dominant branches of P. abies trees were collected at the permafrost site as well as at the adjacent tall forest (about 50 m distance) on the following dates: 18 July 2003, 16 October 2003 and 13 May 2004. Three sampling dates were chosen to capture the seasonal dynamics of storage carbohydrates (especially the strong seasonal variation in starch), with the highest concentrations immediately prior to bud break and lowest concentrations at the end of the growing season in autumn (Hoch et al. Citation2003). The sampled branches were immediately processed in the field and one-year-old needles and branch xylem (one- to four-year-old) with bark and phloem, removed by knife, were collected separately. At all dates, the replication was five branches from five randomly selected trees (≥ 3 m in height) for each site (permafrost and reference forest). In addition 5 mm diameter stem cores were sampled from four trees at each site on 18 July 2003 using a hand increment corer (Suunto, Finland). The last 30 tree rings were defined as sapwood and collected for gravimetric determination of wood density and chemical analyses. Finally, five four-year-old branches per site were sampled on 13 January 2007, of which the xylem was collected for lipid analyses and needles were used for measuring needle dry weight, mean leaf area and specific leaf area (SLA). Projected areas of fresh needles were determined on a photoplanimeter (LI-3050A, Li-Cor, Lincoln, NB, USA). After the return to the laboratory (about 3 h after sampling), all samples for chemical analyses were oven dried for 48 h at 75 °C, ground to fine powder and stored over silica gel until analysis.
Chemical analyses
NSC (the sum of the three quantitatively most important low molecular weight sugars, i.e. glucose, fructose and sucrose, plus starch) were analysed after Wong (Citation1990) as described in detail in Hoch et al. (Citation2002). About 10 mg of plant powder were extracted with 2 ml distilled water at 100 °C for 30 min. An aliquot of the extract was used for the determination of low molecular carbohydrates after enzymatic conversion of fructose and sucrose to glucose. The concentration of free glucose was determined photometrically after enzymatic conversion of glucose to gluconat-6-phosphate on a 96-well multiplate reader. Following the degradation of starch to glucose by a crude fungal amylase (‘Clarase’ from Aspegillus oryzae, Enzyme Solutions Pty Ltd., Crydon South, Victoria, Australia) at 40 °C overnight, NSC was determined in a separate analysis. The concentration of starch was calculated as NSC minus the free low molecular carbohydrates.
Lipids (acylglycerols) were determined in branch wood sampled on 13 January 2007 following the method of Eggstein and Kuhlmann (Citation1974), as described in detail in Hoch et al. (Citation2002). After saponification of lipids by extraction of about 12 mg wood powder in 1 ml aqueous NaOH for 30 min, the amount of liberated glycerol was determined after enzymatic conversion of glycerol to glycerol-3-phosphate in a 96-well microplate reader. To allow for an assessment of the quantitative contribution of lipids to the whole non-structural carbon pool, the measured glyceride-glycerol concentrations were multiplied with an estimated mean molecular weight of lipids. According to Höll and Pieczonka (Citation1978), who measured the ratio of triglycerides to di- and monoglycerides to be 2:1 and identified 18:1, 18:2 and 20:0 fatty acids as the main fatty acids in acylglycerols, we assumed the average molecular weight of lipids in P. abies sapwood to be about 780.
Statistical analyses
Because values of SLA, 100-needle weight, projected needle area and sapwood density were not normally distributed, differences between the reference forest and the permafrost site were tested for significance by non-parametric Wilcoxon rank scores. All NSC and lipid concentrations were log-transformed prior to analyses. Differences of compound concentrations between sites were tested for significance by Student's t-test for single dates. Repeated measures analyses of variance (MANOVA) were used to determine possible effects of site (forest vs. permafrost) on NSC concentrations across all sampling dates, as well as for NSC concentration changes over time (among sampling dates) and for site × time interactions, indicating a potential shift in phenology between sites. All statistical analyses were performed with JMP 5.0.1.2 (SAS Institute, Cary, NC, USA).
Results
The cold soil conditions at the permafrost site strongly restricted needle development in P. abies. Mean needle weight, as well as mean projected area per needle was about half that from trees at the adjacent tall forest (). There was no difference in SLA between the two sites (). In contrast, the sapwood of dwarfed trees was significantly denser (+23 %, ) than that of tall trees. The higher wood density may be a consequence of the much smaller ring widths in trees growing over permafrost ().
Figure 2 Boxplot diagrams for specific leaf area (SLA), 100-needle weight, mean projected area per needle and sapwood density (youngest 30 tree rings) of P. abies at the tall grown reference forest and on the permafrost site. n = 5 for all parameters, except for sapwood density where n = 4. Means where compared for significant differences by Wilcoxon rank scores (n.s., not significant; ∗, P < 0.05; ∗∗, P < 0.01).
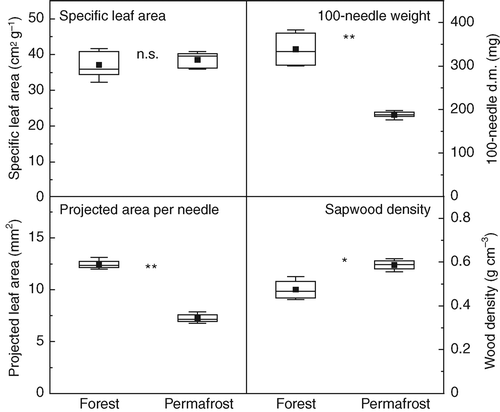
Concentrations of NSC in the needles were significantly higher in dwarfed trees in July and October (). However, at the beginning of the growing season (May) needles of trees from the reference forest showed marginally significantly higher NSC concentrations (P = 0.062, ). The site-specific differences in NSC resulted from different starch concentrations, while low molecular sugar concentrations were not significantly different between the two sites (). Free sugars and starch showed significant concentration changes over the season (time effect in ), with highest concentrations in May (prior to bud break) and lowest concentrations in October (end of growing season). The seasonal dynamics of starch concentrations in needles differed significantly between the two sites (); starch concentrations were higher in forest trees than in dwarfed trees in May, but lower at other dates (), indicating a possible phenological shift between the sites, with the vegetation period staring earlier at the reference forest.
Table 2. Repeated measures analyses for site and time effects and their interaction on free sugars, starch and total non-structural carbohydrates (NSC) concentrations in one-year old needles and branch wood of P. abies. Site effects were analysed for differences between the forest and the permafrost test sites. Time refers to the three sampling dates (May, July and October). n = 5 per site and date; P < 0.05 are in bold
Figure 3 Non-structural carbohydrate (NSC) concentrations in one-year-old needles of P. abies at the reference forest and the permafrost site at three dates across the growing season. Values are means of five replicates + standard error. For each date differences between sites were tested for significance by Student's t-test [(∗), P < 0.1; ∗, P < 0.05; ∗∗, P < 0.01].
![Figure 3 Non-structural carbohydrate (NSC) concentrations in one-year-old needles of P. abies at the reference forest and the permafrost site at three dates across the growing season. Values are means of five replicates + standard error. For each date differences between sites were tested for significance by Student's t-test [(∗), P < 0.1; ∗, P < 0.05; ∗∗, P < 0.01].](/cms/asset/1e37b8a2-bc4e-4bd9-b6ae-9d08fa49e8ee/tped_a_323246_o_f0003g.gif)
Unlike needles, NSC concentrations of young branch wood were higher at the permafrost site throughout the growing season (). Except for starch in branches sampled in May, the higher NSC pattern in branch wood of dwarfed trees resulted from both low molecular weight sugars and starch. If the NSC concentrations of branches are given as percent dry matter, only the samples from October are significantly different. However, the higher wood density of dwarfed trees () led to a higher fraction of structural compounds (cell walls) within the dry biomass compared to tall trees. Therefore, NSC concentrations of wood from trees at the permafrost site were virtually ‘diluted’ by those structural compounds if calculated on a dry matter basis. In order to eliminate the bias in NSC concentrations introduced through different wood densities between sites, NSC concentrations in branch wood were additionally calculated on a volume basis (i.e. as mg NSC cm−3), which resulted in significantly higher NSC concentrations in dwarfed trees in July and October and a marginally significant difference (P = 0.0654) in May (). The repeated measures analysis showed a significant site effect across dates for sugars and NSC (when given per volume) and a significant time effect across sites for free sugars (). The significant site × time interaction for starch in branch wood () resulted from similar concentrations between sites in May, but significantly higher concentrations at the permafrost site later in the season ().
Figure 4 Non-structural carbohydrate (NSC) concentrations in branch sapwood of P. abies at the reference forest and the permafrost site at three dates across the growing season. Concentrations are given on a percent dry matter basis on the left, and on a volume basis on the right side. Values are means of five replicates + standard error. For each date differences between sites were tested for significance by Student's t-test [n.s., not significant; ∗, P < 0.05; ∗∗, P < 0.01].
![Figure 4 Non-structural carbohydrate (NSC) concentrations in branch sapwood of P. abies at the reference forest and the permafrost site at three dates across the growing season. Concentrations are given on a percent dry matter basis on the left, and on a volume basis on the right side. Values are means of five replicates + standard error. For each date differences between sites were tested for significance by Student's t-test [n.s., not significant; ∗, P < 0.05; ∗∗, P < 0.01].](/cms/asset/20bad697-a35e-4c21-9058-d5b9acb668c5/tped_a_323246_o_f0004g.gif)
NSC concentrations in stem sapwood were measured only in samples taken in July. At both sites, NSC concentrations were about half of that in young branch wood (). As in needles and branch wood, NSC concentrations in sapwood were higher (but not significantly) in dwarfed than in tall trees. Although stem wood of trees growing at the reference forest site tended to have higher low molecular weight sugar concentrations than at the permafrost site, they exhibited exceptionally low starch concentrations ().
Figure 5 Non-structural carbohydrate (NSC) concentrations in stem sapwood (youngest 30 years) of P. abies at the reference forest and the permafrost site in July. Concentrations are given on a percent dry matter basis on the left, and on a volume basis on the right side. Values are means of four replicates + standard error. Differences between sites were not significant by Student's t-test.
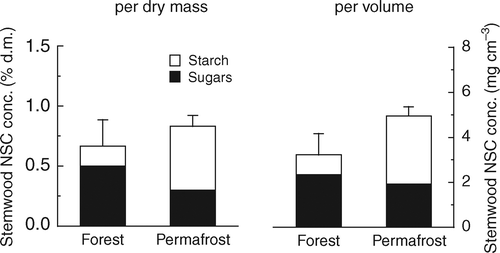
The lipid concentrations in branch wood sampled in midwinter were estimated from multiplying the molar concentration of esterified glycerol with the assumed mean molecular weight of neutral lipids in spruce sapwood (see Material and methods). However, even if the used approximation for the neutral lipid concentrations should underestimate the real value, their concentrations are still markedly lower than that of non-structural carbohydrates (1% d.m., ), indicating only a minor role of lipids as carbon reserves in P. abies. Nevertheless, the lipid concentration in branch wood of dwarfed trees was higher than in tall trees, an effect which was significant if analysed per sapwood volume, and thus, taking into account the different wood densities ().
Figure 6 Lipid (acylglycerols) concentrations in branch sapwood of P. abies sampled in midwinter (January) at the reference forest and the permafrost site as estimated from measurements of esterified glycerol (see Material and methods for details). Concentrations are given on a percent dry matter basis on the left, and on a volume basis on the right side. Values are means of five replicates + standard error. Differences between sites were tested for significance by Student's t-test (n.s., not significant; ∗, P < 0.05).
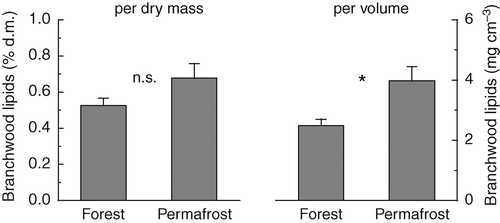
Discussion
The montane permafrost site of Creux du Van represents an extraordinary site, where low soil temperature impacts that are normally found only at alpine treelines can be investigated in a markedly warmer surrounding. The analysis of non-structural carbon pools of Norway spruce within and outside of the permafrost zone largely supported previous results from alpine treeline ecotones. Overall, dwarfed trees at the permafrost site showed a higher charging with non-structural carbon compounds than tall tress from the neighbouring forest.
This study, as well as a former study at the same site (Körner and Hoch Citation2006), indicated severe effects of low soil temperatures on aboveground growth of P. abies at the permafrost sites, although the stems and crowns were in fact exposed to even slightly warmer growing season temperatures than those of the tall forest trees. In addition to restricted annual shoot increment and tree ring growth (Körner and Hoch Citation2006), the current study documented a large decrease in size and biomass of needles from dwarfed trees. The 100-needle weight at below 200 mg for mature spruce needles at the permafrost site is lower than the minimum value recorded in a survey of P. abies needle traits across different sites in the Austrian Alps by Perterer and Körner (Citation1990). In contrast to the general trend of decreasing SLA with altitude (and thus cooler growing temperatures: Hultine and Marshall Citation2000; Körner Citation2003), the projected area SLA of spruce at the permafrost site, did not differ from that of tall trees. This may be related to the peculiar situation at the study site, where needles do not directly suffer from cold air temperatures, but are restricted in their growth through the indirect effect of cold root zone temperatures. In contrast, the very small annual tree rings in dwarfed trees obviously led to a significant increase of wood density, due to smaller cell diameters and thus a higher proportion of cell wall tissue per wood volume (Antonova and Stasova Citation1993; Camarero et al. Citation1998).
The higher NSC pools of trees at the permafrost site accord with previous studies, which demonstrated increasing sugar, starch and lipid pools with altitude across the alpine treeline ecotone (Hoch et al. Citation2002; Hoch and Körner Citation2003, Citation2005; Piper et al. Citation2006; Shi et al. Citation2006, Citation2008). The increasing carbon storage pools with lower temperature have been interpreted to signal an imbalance in the carbon source-sink-relations of trees, induced by a directly low-temperature imposed growth (i.e. sink) limitation at simultaneously sufficient carbon supply by photo-assimilation (i.e. source activity: Körner Citation1998). At natural alpine treelines the transition zone between tall closed forests and the uppermost crippled tree stands may span 100–200 m in altitude (and depending on the slope inclination, markedly longer effective distances along the slope). Assuming an average adiabatic lapse rate of 0.6 °C per 100 m altitude (Körner Citation2003), the thermal amplitude between closed forest and uppermost tree stands along natural alpine treeline ecotones is generally smaller (0.6 to 1.2 °C) than the c. 2 °C difference in mean seasonal soil temperatures between tall forest and permafrost found in this study.
Because of the sometimes substantial distances between the lower and the upper edge of the treeline ecotone, forest and treeline sites may not only differ in terms of their thermal regimes, but also with respect to other abiotic and biotic factors. Hence, factors other than temperature have also been proposed to restrict tree growth at the treeline. Tranquillini (Citation1979) and Holtmeier (Citation2003) suggested that trees at treeline frequently suffer from a higher loss rate of photosynthetic tissue (needles or leaves) compared to individuals at lower elevations, because of higher mechanical stress (e.g. wind break, ice blasting, snow pressure). Smith et al. (Citation2003) reasoned consequently, that higher carbon reserve stores at treeline would eventually reflect an ecotypic adaptation of treeline populations to those harsher environments. However, trends in increasing NSC pools with altitude have also been found at sub-tropical and tropical treelines (Hoch and Körner Citation2003, Citation2005), which lack regular strong winds, ice blasting or long-lasting snow accumulation. The dwarfed spruce trees of the current study are not exposed to higher mechanical stress than the adjacent tall trees. Since tall forests encircle them, they are likely even more sheltered from strong winds or snow pressure. Further, because of the small spatial distance between permafrost and reference forest site, all investigated trees recruit from the same population, contradicting genotypic explanations for the higher non-structural carbohydrate pools of dwarfed spruces.
Although carbon acquisition is regulated through direct and indirect feedback mechanisms of which still relatively little is known about (Smith and Stitt Citation2007), NSC pools do vary within specific limits in response to situations of enhanced or decreased carbon availability (Iglesias et al. Citation2002). The sensitivity of NSC reserves to the carbon source-sink balance of a plant could be demonstrated in numerous experimental studies in the past, since their concentrations generally departure from ‘normal’ values under situations of changed carbon supply or carbon investment activities (CO2 enrichment, e.g. Teng et al. Citation2006 and references therein; phloem girdling, e.g. Jordan and Habib Citation1996; defoliation, e.g. Canham et al. Citation1999).
Experimental studies investigating carbon reserve pools of trees in response to temperatures at or below the thermal limit of growth (about 6 °C) are scarce and were restricted to seedlings and saplings for operational reasons. Those experiments generally support the picture of cold temperature induced direct growth limitation, by revealing higher non-structural carbon pools in cold treated plants. Kontunen-Soppela et al. (Citation2002), for example, found significantly higher free sugars and starch concentrations in needles of two-year-old P. sylvestris saplings exposed to a 14-d period of 4 °C during mid-season, resulting in approximately 40% higher total non-structural carbohydrate concentrations (on a fresh weight basis) compared to seedlings grown at 18 °C during the same period.
In another study, Domisch et al. (Citation2001) described a large reduction of below-ground and above-ground biomass increment of 180-d-old Pinus sylvestris seedlings when exposed to 5 °C soil temperatures for 63 d compared to seedlings grown at 9 °C, but especially to seedlings grown at 13 °C or 17 °C. Like in our current study, the experiment by Domisch et al. (Citation2001) therefore exhibits a negative effect of cool root zone temperatures on non-cooled aboveground meristems. Although absolute non-structural carbohydrate contents were lower in the cold treated seedlings, reflecting the different total seedling masses, the concentrations on a dry matter basis tended to be higher in seedlings exposed to 5 °C and 9 °C than in those exposed to 13 and 17 °C.
Solfjeld and Johnsen (Citation2006) have documented continuous severe growth restrictions in roots and aboveground organs of Betula pendula seedlings grown at cold root zone temperatures for 56 d. Total seedlings biomass declined strongly along a thermal gradient of 17, 12, 6 and 2 °C root zone temperatures at otherwise uniformly warm crown temperatures. Non-structural carbohydrates were significantly higher in roots and leaves of seedlings treated with 2 °C soil temperatures than in those exposed to 17 °C, which Solfjeld and Johnsen (Citation2006) have attributed to the higher rate of incorporation of carbohydrates into growth in the warm treated seedlings.
In conclusion, the higher concentrations of carbon reserves found in all investigated tree tissues from the montane permafrost site at Creux du Van during most of the season, indeed support the hypothesis of enhanced carbon charging of trees at their thermal limit of growth (sink-limitation hypothesis). Since the dwarfed tress on permafrost grow directly next to tall growing trees and experience warm crown temperatures (unlike those at treelines) low soil temperatures alone can sufficiently explain the differences in growth and carbon reserve supply.
Acknowledgements
I would like to thank Prof. Christian Körner for introducing me to the permafrost site of Creux du Van and for his valuable comments on the manuscript, and Brigitta Bildstein for her assistance in the field.
References
- Antonova , GF and Stasova , VV . 1993 . Effects of environmental factors on wood formation in Scots pine stems . Trees – Structure and Function , 7 : 214 – 219 .
- Camarero , JJ , Guerrero-Campo , J and Gutiérrez , E. 1998 . Tree-ring growth and structure of Pinus uncinata and Pinus sylvestris in the central Spanish Pyrenees . Arctic and Alpine Research , 30 : 1 – 10 .
- Canham , CD , Kobe , RK , Latty , EF and Chazdon , RL . 1999 . Interspecific and intraspecific variation in tree seedling survival: effects of allocation to roots versus carbohydrate reserves . Oecologia , 121 : 1 – 11 .
- Chapin , FS , Schulze , ED and Mooney , HA . 1990 . The ecology and economics of storage in plants . Annual Review of Ecology and Systematics , 21 : 423 – 447 .
- Davis , N. 2001 . Permafrost: a guide to frozen ground in transition , Fairbanks : University of Alaska Press .
- Delaloye , R and Reynard , E. 2001 . Les eboulis gelés du Crex du Van (Chain du Jura, Suisse) . Environnements périglaciaires , 8 : 118 – 129 .
- Delaloye , R , Reynard , E , Lambiel , C , Marescot , L and Monnet , R. . Permafrost . Proceedings of the 8th International Conference on Permafrost . July 21–25 2003 , Zürich, Switzerland. Thermal anomaly in a cold scree slope (Creux du Van, Switzerland) , Edited by: Phillips , M , Springman , SM and Arenson , LU . pp. 175 – 180 . Zürich : Lisse-Balkema AA .
- Dickson , RE . 1991 . “ Assimilate distribution and storage ” . In Physiology of trees , Edited by: Raghavendra , AS . 51 – 85 . New York : John Wiley & Sons .
- Domisch , T , Finer , L and Lehto , T. 2001 . Effects of soil temperature on biomass and carbohydrate allocation in Scots pine (Pinus sylvestris) seedlings at the beginning of the growing season . Tree Physiology , 21 : 465 – 472 .
- Eggstein , M and Kuhlmann , E. 1974 . “ Triglyceride und Glycerin (alkalische Verseifung). ” . In Methoden der enzymatischen Analyse. Band II , Edited by: Bergmeyer , HU . 1871 – 1878 . Weinheim : Verlag Chemie .
- Furrer , E. 1966 . Kümmerfichtenbestände und Kaltluftströme in den Alpen der Ost- und Innerschweiz . Schweizerische Zeitschrift für das Forstwesen , 117 : 720 – 733 .
- Grace , J , Berninger , F and Nagy , L. 2002 . Impacts of climate change on the tree line . Annals of Botany , 90 : 537 – 544 .
- Hoch , G and Körner , C. 2003 . The carbon charging of pines at the climatic treeline: a global comparison . Oecologia , 135 : 10 – 21 .
- Hoch , G and Körner , C. 2005 . Growth, demography and carbon relations of Polylepis trees at the world's highest treeline . Functional Ecology , 19 : 941 – 951 .
- Hoch , G , Popp , M and Körner , C. 2002 . Altitudinal increase of mobile carbon pools in Pinus cembra suggests sink limitation of growth at the Swiss treeline . Oikos , 98 : 361 – 374 .
- Hoch , G , Richter , A and Körner , C. 2003 . Non-structural carbon compounds in temperate forest trees . Plant, Cell and Environment , 26 : 1067 – 1081 .
- Höll , W and Pieczonka , K. 1978 . Lipids in sap- and heartwood of Picea abies (L.) Karst . Zeitschrift für Pflanzenphysiologie , 87 : 191 – 198 .
- Holtmeier , FK . 2003 . “ Mountain timberlines ” . In Ecology, patchiness and dynamics , Dordrecht : Kluwer Academic Publishers .
- Hölzel , E. 1963 . Tierleben im Eiskeller der Matzen in der Karawankennordkette . Carinthia II (Klagenfurt) , 153 : 10 – 21 .
- Hultine , KR and Marshall , JD . 2000 . Altitude trends in conifer leaf morphology and stable carbon isotope composition . Oecologia , 123 : 32 – 40 .
- Iglesias , DJ , Lliso , I , Taedo , FR and Talon , M. 2002 . Regulation of photosynthesis through source: sink imbalance in citrus is mediated by carbohydrate content in leaves . Physiologia Plantarum , 116 : 563 – 572 .
- Jordan , MO and Habib , R. 1996 . Mobilizable carbon reserves in young peach trees as evidenced by trunk girdling experiments . Journal of Experimental Botany , 47 : 79 – 87 .
- Kontunen-Soppela , S , Lankila , J , Lahdesmaki , P and Laine , K. 2002 . Response of protein and carbohydrate metabolism of Scots pine seedlings to low temperature . Journal of Plant Physiology , 159 : 175 – 180 .
- Körner , C. 1998 . A re-assessment of high elevation treeline positions and their explanation . Oecologia , 115 : 445 – 459 .
- Körner , C. 2003 . Alpine plant life: functional plant ecology of high mountain ecosystems , 2nd , Berlin : Springer .
- Körner , C. 2006 . “ Significance of temperature in plant life ” . In Plant growth and climate change , Edited by: Morison , JIL and Morecroft , MD . 48 – 69 . Oxford : Blackwell Publishing .
- Körner , C and Hoch , G. 2006 . A test of treeline theory on a montane permafrost island . Arctic Antarctic and Alpine Research , 38 : 113 – 119 .
- Körner , C and Paulsen , J. 2004 . A world-wide study of high altitude treeline temperatures . Journal of Biogeography , 31 : 713 – 732 .
- Molenda , R and Gude , M. 2000 . Naturschutzfachliche Bewertung von Blockhalden mit airconditioning Effekt . Entomologia Brasiliensa , 22 : 87 – 91 .
- Perterer , J and Körner , C. 1990 . Das Problem der Bezugsgröße bei physiologisch-ökologischen Untersuchungen an Koniferennadeln . Forstwirtschaftliches Centralblatt , 109 : 220 – 241 .
- Piper , FI , Cavieres , LA , Reyes-Diaz , M and Corcuera , LJ . 2006 . Carbon sink limitation and frost tolerance control performance of the tree Kageneckia angustifolia D. Don (Rosaceae) at the treeline in central Chile . Plant Ecology , 185 : 29 – 39 .
- Richard , JL . 1961 . Le forêts acidophiles du Jura – étude phytosociologique et écologique . Commission Phytogéographique de la Société Helvétique des Sciences Naturelles Fasc , : 38
- Rist , A. 2002 . Unterkühlte Blockschutthalden mit Hexenwäldli – Abiotische Faktoren zur Charakterisierung des Phänomens , Germany : Technical University of Munich .
- Schindler , H , Kinzel , H and Burian , K. 1976 . Ökophysiologische Untersuchungen an Pflanzen der Matzen-Eisstandorte . Carinthia II (Klagenfurt) , 166 : 269 – 307 .
- Shi , P , Körner , C and Hoch , G. 2006 . End of season carbon supply status of woody species near the treeline in western China . Basic and Applied Ecology , 7 : 370 – 377 .
- Shi , P , Körner , C and Hoch , G. 2008 . A test of the growth-limitation theory for alpine treeline formation in evergreen and deciduous taxa of the Eastern Himalayas . Functional Ecology , 22 : 213 – 220 .
- Smith , AM and Stitt , M. 2007 . Coordination of carbon supply and plant growth . Plant, Cell and Environment , 30 : 1126 – 1149 .
- Smith , WK , Germino , MJ , Hancock , TE and Johnson , DM . 2003 . Another perspective on altitudinal limits of alpine timberlines . Tree Physiology , 23 : 1101 – 1112 .
- Solfjeld , I and Johnsen , O. 2006 . The influence of root-zone temperature on growth of Betula pendula Roth . Trees – Structure and Function , 20 : 320 – 328 .
- Teng , N , Wang , J , Chen , T , Wu , X , Wang , Y and Lin , J. 2006 . Elevated CO2 induces physiological, biochemical and structural changes in leaves of Arabidopsis thaliana . New Phytologist , 172 : 92 – 103 .
- Tranquillini , W. 1979 . “ Physiological ecology of the alpine timberline ” . In Tree existence at high altitudes with special references to the European Alps , Berlin : Springer .
- Wakonigg , H. 1996 . Unterkühlte Schutthalden. Arbeiten aus dem Institut für Geographie der Karl-Franzens-Universität Graz . Beiträge zur Permafrostforschung , 33 : 209 – 223 .
- Wegmüller , J and Wegmüller , S. 1985 . Ökologische Untersuchungen an Zwergfichtenbeständen im Gebit des Turnen (Niedersimmental) . Mitteilungen der Naturforschenden Gesellschaft in Bern , 42 : 87 – 104 .
- Wong , SC . 1990 . Elevated atmospheric partial-pressure of CO2 and plant-growth. 2 Nonstructural carbohydrate content in cotton plants and its effect on growth-parameters . Photosynthesis Research , 23 : 171 – 180 .