Abstract
Background: Forest boundaries at sites of natural limitation of tree growth (‘treelines’) offer a valuable opportunity for studying responses and adaptation mechanisms of tree species to extreme site conditions under recent and future climate.
Aim: The study investigated above- and below-ground structure and leaf and root morphology in a Nothofagus pumilio forest in south Patagonia along an elevation gradient from the natural xeric forest–steppe treeline to the alpine treeline with intervening mesic tall forest.
Methods: We analysed the stands' above- and below-ground structure and leaf production, as well as leaf and root morphological and chemical traits. We also measured microclimate and soil chemical properties of the forest stands.
Results: Microclimate measurements showed a remarkably high temperature lapse rate (c. 1 K 100 m−1) along the elevation transect. Soil moisture was most favourable in the mid-altitude tall forest and least favourable at the xeric treeline. Thermal conditions at the alpine treeline were in line with data reported to coincide with the upper treeline position globally. The changes in above- and below-ground structure from the tall forest to the treelines were similar: tree height, stem diameter, and leaf production decreased, while stem density (number of trees per area) increased towards the treelines indicating a reduction in above-ground biomass investment. However, leaf area index was only reduced at the xeric treeline. In contrast, living and dead fine root mass, as well as the surface area of the fine root system increased markedly towards both treeline sites. Leaf and root morphology did not differ among the stands, but leaves at the treelines had higher nitrogen contents, while foliar N:P ratio decreased with elevation.
Conclusions: The findings suggest that the above- and below-ground response to growth conditions at predominantly drought-affected and temperature-driven treelines can be principally similar. However, the identification of the underlying driving factors causing the marked shift in C allocation towards the root system is more obvious at the xeric than at the alpine treeline.
Introduction
The upper treeline in montane–alpine ecotones is the most widely recognised vegetation limit on all continents. Hence, numerous studies have been conducted on this upper forest boundary. The obvious changes in above-ground structure, morphology, and carbon cycle are predominantly influenced by the decrease in temperature with elevation (e.g. George et al. Citation1974; Tranquillini Citation1979; Ohsawa Citation1990; Körner Citation1998). Recent studies have indicated that treelines globally are associated with a mean growing season root zone temperature of 6.7 °C (Körner and Paulsen Citation2004). However, there is still debate on the actual mechanisms that limit tree growth above a certain elevation (data reviewed by Stevens and Fox Citation1991; Körner Citation1998, Citation2003a; Grace et al. Citation2002). Apart from the alpine treeline, distribution boundaries of forests also occur under dry conditions at the transition to grassland habitats (Sarmiento Citation1984; Walter Citation1985; Stevens and Fox Citation1991). Beside drought stress and the associated physiological constraints, tree growth at such xeric boundaries might also be affected by the occurrence of fire (e.g. Huntley and Walker Citation1982; Hennenberg et al. Citation2006; Müller et al. Citation2007; Prior et al. Citation2007). Additionally, tree seedling establishment and success in the open land is often restricted by unfavourable moisture conditions, fire, and intense competition with the grassland vegetation (e.g. Fensham and Kirkpatrick Citation1992; Hoffman et al. Citation2004; Müller et al. Citation2007). Although the changes in plant form and above-ground stand structure show some similarities between the alpine and the xeric forest–grassland transition zone, there has been little attempt to compare the structural and morphological responses of the tree vegetation to the prevailing growth conditions at the upper and the lower treeline (e.g. Stevens and Fox Citation1991). Moreover, most research to date has been made on the above-ground parts of the forests, neglecting the responses of the root system of the trees.
In the southern Andes (> c. 35° S) the alpine treeline consists of deciduous tree species of the genus Nothofagus (Schmaltz Citation1991; Donoso Citation1993; Veblen et al. Citation1996). Nothofagus pumilio, in particular, forms tall forests at middle elevation in north Patagonia (c. 800–1400 m at c. 41° S) and at lower elevation in south Patagonia (c. 600–1000 m at c. 50° S). At higher elevations, trees of N. pumilio form dense prostrate Krummholz stands at the treeline (Kalela Citation1941; Veblen et al. Citation1996; Lara et al. Citation2005). Due to the persistent wind direction, the Patagonian region west of the Andes receives high amounts of rainfall, while east of the Andes a steep precipitation gradient is present within 20–50 km from the main Andean chain to the Patagonian steppe (Ljungner Citation1939; Kalela Citation1941; Schmaltz Citation1991; Daniels and Veblen Citation2004). In this region, forest grows within a narrow range near the mountains. In south-east Patagonia, where the alpine treeline is present at relatively low altitudes, there are some locations where the down-slope transition from the alpine treeline to tall Nothofagus forests and further to the forest–steppe boundary occurs within a short elevation distance. Such a location was selected in our study to test the hypotheses that (1) changes in forest structure and leaf morphology of N. pumilio towards the lower treeline can be primarily interpreted as responses to increased drought stress, while (2) structural and morphological patterns of the same species at the upper (alpine) treeline are predominantly caused by low temperature conditions. Hence, we aimed to analyse (i) how the prevailing climatic growth conditions in the forests stands differed among the xeric treeline, the mid-altitude tall forest, and the alpine treeline, (ii) what differences in above- and below-ground structure of the N. pumilio stands could be found along the transition from the lower to the upper treeline, and (iii) if N. pumilio trees responded by modifying the morphology and nutrient status of their leaves and fine roots to the different growth conditions.
Material and methods
Study site
The study was conducted near El Chalten (49° 23′ S, 72° 55′ W), north of Lake Viedma in the Los Glaciares National Park, Santa Cruz, Argentina between 2002 and 2005. This area is situated in one of the steepest precipitation gradients of the world: annual precipitation is 6000–7500 mm year−1 of water equivalent on the Southern Patagonian Ice Field c. 20–30 km west of the study area (Escobar et al. Citation1992) and decreases sharply on the lee side of the Andes to c. 440 mm year−1 at the steppe–forest boundary in the area of El Chalten (data from the weather station of the ranger station of the Los Glaciares National Park) and to 200–300 mm year−1 in the treeless Patagonian steppe few kilometres east of the site (Villalba et al. Citation2003). We selected three representative forest stands of mature Nothofagus pumilio trees along an elevation transect between 750 and 1050 m a.s.l., representing a small-scale climatic gradient from the steppe-facing xeric treeline at the lowest elevation followed by mid-altitude (920 m a.s.l.) tall forest of mesic micro-climatic condition to the relatively moist alpine treeline at the highest elevation (). All forests grew on gentle (5°) to moderately steep (10–15°) east-facing slopes. Following the definition given by Körner and Paulsen (Citation2004), we interpret the upper forest stand at 1050 m a.s.l. as representing the upper treeline in this region (i.e. the upper distribution limit of connected forest patches of trees > 2–3 m in height in the area). All forest stands had a closed canopy without any gaps. A second layer of juvenile trees or a shrub layer was absent. The xeric treeline stand had a largely graminaceous herb layer with Festuca together with individuals of the herb Osmorhiza chilensis and some Nothofagus seedlings (ground cover c. 20%). The herb layer in the mid-altitude forest was sparse at < 5% cover by Osmorhiza and Nothofagus seedlings. No herb layer was present in the alpine treeline stand. All three Nothofagus stands grew on fine-textured (sandy–loamy) mineral soils derived from granite and schist bedrocks.
Figure 1 View from the Patagonian steppe west to the Andes near El Chalten (49° 23′ S 72° 55′ W). The narrow strip of Nothofagus forest between the xeric treeline and the alpine treeline is visible in the left part of the picture. Note the typical weather conditions of this area with the highest peaks of the Andes in the background (e.g. Mt Fitzroy, 3406 m; Cerro Torre, 3133 m) being covered in rain and snow clouds and the increasingly drier areas towards the steppe (Photo: D. Hertel).
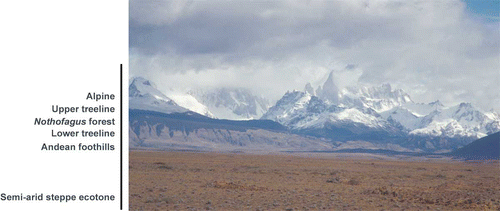
Soil properties
In order to compare the soil chemical conditions among the three stands, four soil samples were randomly taken during the middle of the vegetation period. Fresh soil samples (organic layer and 0–20 cm of mineral soil) were analysed for pH(H2O) and pH(KCl) values. Total organic carbon and nitrogen in the organic layer and the mineral soil were determined by a C/N elemental analyser (Vario EL III, elementar, Hanau, Germany). C:N ratios are given in mol mol−1. The concentrations of salt-extractable cations in both soil depths were determined by percolating 2.5 g of soil with 100 ml 0.2 N BaCl2 solution for 4 h. The solution concentrations of Na, K, Mg, Ca, Mn, Al and Fe were analysed by atomic absorption spectroscopy (vario 6, analytik Jena, Germany). The concentration of hydrogen ions at the soil cation exchangers was calculated from the observed pH change during the percolation process. The effective cation exchange capacity (CECe) was recorded as the sum of all extractable cations in the BaCl2 extraction. The base saturation was calculated as relative proportion (%) of Na, K, Mg and Ca in CECe. As an estimate of plant-available phosphorus, P was extracted by resin bags that were placed for 16 h in a solution of 1 g of soil material suspended in 30 ml of water (‘Presin’, Sibbesen Citation1977; Bowman and Cole Citation1978). P was then re-exchanged by NaCl and NaOH solutions and analysed colorimetrically after blue-dyeing. The thickness of the organic layer was measured from 10 additional soil samples randomly taken for root analysis (see below).
Climatic measurements
Microclimate conditions of the three stands were measured over a year using Hobo data loggers (Onset, US) for continuous records of air temperature and relative humidity 120 cm above the ground, and mini-dataloggers (iButtons, Maxim Dallas, US) for records of air and soil temperature at +120 cm and −10 cm, respectively. Sensors in air were screened against direct radiation with ventillated white plastic caps. Occasional observation showed that even a thin layer of few centimetres of snow led to an effective thermal isolation of the soil corresponding to an immediate temperature measure signal of 0 °C at −10 cm. Hence, we used this indication in the logger data to measure snow cover duration. Length of the growing season was estimated following the protocol given in Körner and Paulsen (Citation2004), who indicated that a soil temperature of 3.2 °C at −10 cm is strongly correlated with an air temperature of 0 °C and, thus, marks the threshold between periods suitable or unsuitable for tree physiological activity. Since soil temperature data are less susceptible to bias of the records due to effects of direct or indirect insolation, we used this threshold to calculate growing season length as the period when mean daily soil temperature was continuously > 3.2 °C.
Above-ground forest structure
Measurements were made of diameter at breast height (dbh) of all tree stems and stem density was recorded in plots of 16 m2 at the uppermost site, while plot size was 225 m2 at the mid-altitude and at the lowermost sites, to include a sufficient number of dominant tree individuals per plot. In the case of the uppermost stand, dbh values of prostrate tree individuals were measured at 130 cm distance from the stem base. These data were used to calculate the stand basal area of the forest plots. Mean tree height of the stands was measured from 10 randomly selected tree individuals per stand using a Vertex III Ultrasonic device (Hagloef, Sweden).
Leaf and seed production, leaf morphology and chemistry
To investigate annual leaf and seed production, eight litter traps were installed randomly in each stand. The traps were made of a plastic net bag of c. 2 mm mesh-size with a circular opening of 29 cm in (inner) diameter suspended in a metal rack c. 1 m above the ground. One trap was damaged in the uppermost stand; thus, data from only seven litter traps are available for this stand. The complete litter material was collected after leaf fall in autumn, air dried, and transported to the laboratory in Mendoza. There, litter material was separated into leaves, seeds, and other material (e.g. twigs) and the mass was measured after drying for 48 h at 70 °C. Prior to drying, 20 leaves were selected randomly from each litter trap to analyse leaf morphology, resulting in a total of 140–180 leaves per stand. Digital images of the rehydrated leaves were made by using a scanner. Leaf areas were measured from scanned images of the leaves using WinFolia software (Régent Instruments Inc., Canada) and the specific leaf area (SLA) was determined. Leaf area index (LAI) was calculated by multiplying SLA by the total leaf mass of each litter trap and relating this value to ground area covered by the trap net.
To analyse leaf chemical composition, leaves were sampled from twigs of the upper sun-lit crown of eight different Nothofagus trees; 20 leaves were picked per twig and were pooled for further analyses, resulting in a total of 80 leaves per stand and eight leaf samples. Leaves were stored in paper bags, air dried, and transported to the laboratory in Göttingen. After drying for 48 h at 70 °C, the leaves were ground. Total carbon and nitrogen concentrations were analysed with a C/N elemental analyser (Vario EL III, elementar, Hanau, Germany). Total phosphorus concentration was determined photometrically with a staining procedure (p-yellow), after digestion of leaf tissue with 65% HNO3 at 195 °C.
Structure of the fine root system
Root samples were taken with a soil corer (3.5 cm in diameter) from the organic layer and the upper mineral soil (0–20 cm) at 10 randomly selected sampling locations per study plot. The soil samples were transferred to plastic bags and stored under cold conditions (c. 6 °C) and transported to the laboratory at the University of Göttingen within a few days, where the samples were processed within 30 days. The samples were cleaned in water from soil residues using a sieve with a mesh size of 0.25 mm. Large root fractions (> 10 mm in length) were extracted by hand with tweezers. Only fine roots (roots < 2 mm in diameter) of trees were considered for the analysis. Living (biomass) and dead rootlets (necromass) were distinguished under a stereo-microscope by means of colour, root elasticity, and the degree of cohesion of cortex, periderm and stele (e.g. Persson Citation1978; Hertel and Leuschner Citation2002). This fine root fraction represents the majority of living fine root mass, but a large portion of the whole fine root necromass, typically formed by small root fractions (< 10 mm length), is hardly recovered with this method (Bauhus and Bartsch Citation1996; Hertel Citation1999). Hence, one-third of the samples were subjected to an additional, more detailed analysis of finest root necromass particles (< 10 mm in length) applying a method introduced by van Praag et al. (Citation1988) and modified by Hertel (Citation1999). After extraction of the large root fractions, the residue of the sample was evenly spread on a large sheet of filter paper (730 cm2) with 36 squares marked on it. Six of the squares were randomly selected and analysed under a stereo-microscope for even the smallest dead fine root fragments. Total mass of small dead rootlets was extrapolated by regression analysis of the ratio of small rootlets to large dead roots (> 10 mm in length) recorded in the same sub-sample. Fine root biomass and necromass of each sample were dried at 70 °C for 48 h and weighed. The data were expressed as fine root dry mass of the respective soil depth, and as profile fine root mass total (g m−2).
Root morphology and nitrogen content
One living rootlet was selected randomly per soil sample for analysing mean fine root diameter and root specific surface area (SRA, in cm2 g−1) by using scanned images and the WhinRhizo (Régent Instruments Inc., Québec, Canada) image analysis system. Fine root area index (RAI, in m2 root surface area per m2 ground area) was calculated from SRA values and fine root biomass of a respective horizon. The abundance of live fine root tips was determined by counting under a stereo-microscope in the same fine root sample used for analysis of SRA. Tip abundance was expressed on a dry mass basis (specific root tip abundance, n mg−1) or on a stand ground area basis (root tip frequency, or total number of root tips m−2 stand area). The degree of ectomycorrhizal infection in these root samples (%) was recorded (Glenn et al. Citation1991; Godbold et al. Citation1997). Nitrogen content of the selected rootlets was analysed with a C/N elemental auto-analyser (Vario EL III, elementar, Hanau, Germany).
Statistical analysis
All data sets were analysed for normality of distribution using a Shapiro and Wilk test. Normally distributed data were tested for significant differences using an ANOVA or GLM procedure followed by pair-wise comparison after Scheffé (P < 0.05). Other data sets were tested with one-way Kruskal–Wallis single factor analyses of differences among groups (P < 0.05). Subsequently, a non-parametric Mann–Whitney two-sample test (U-test) was applied to identify the differences (P < 0.05). All calculations were performed using SAS, version 8.01 (SAS Institute, Cary, NC, USA).
Results
Soil characteristics
The organic layer covering the mineral soil was thicker in the mid-altitude forest and tended to be thinner in the xeric and the alpine treeline forests (). Differences in chemical soil conditions were less pronounced: pH(H2O) values ranged between 5.4 and 6.6 in the organic layer and between 5.0 and 5.5 in the mineral soil; pH(KCl) values were about one unit lower and decreased significantly with elevation. Values of the C:N ratio were relatively high in both the organic layer and the mineral soil. Cation exchange capacity was low in the mineral soil, but showed rather high values in the organic layer of the xeric treeline stand compared to the mid-altitude and alpine treeline stands. Base saturation ranged from 21 to 34% in the organic layer and the upper mineral soil, indicating moderate to slightly low availability of K, Ca, and Mg elements in all stands. Plant-available phosphorus (according to resin-bag extraction method by Bowman and Cole Citation1978) was similar between the three stands in the organic layer, but increased significantly in the upper mineral soil with elevation ().
Table 1. Thickness of the organic layer and soil chemical characteristics in three Nothofagus pumilio stands in southern Patagonia. Values are means ± SE; n = 10 for organic layer thickness and n = 4 for soil chemical properties. Different letters indicate statistically significant differences (P < 0.05)
Microclimatic conditions
There was a strong decrease in mean annual air temperature from the xeric treeline stand (5.7 °C) to the alpine treeline site (2.8 °C, ). Hence, temperature lapse rate reached high values of 1.06 K and 0.85 K 100 m−1 at the lower and the upper elevation ranges of the three stands. However, the absolute air temperature minimum was lowest (−13.5 °C) at the xeric site. Changes in mean annual soil temperature with elevation were similar to those in air temperature with 4.0 °C at the xeric treeline compared to 2.1 °C at the alpine treeline. Mean growing season soil temperature differences were small, with a somewhat lower soil temperature in the mid-altitude forest compared to the treeline stand (). However, growing season soil temperature sum (‘day degrees’) in the lowermost stand was 30% higher than in the mid-altitude forest, and over 80% higher than in the alpine treeline stand; temperature sum in the mid-altitude forest was 37% higher compared to the alpine treeline. This was mainly a result of large differences in growing season length with a period of 211 d at the lower treeline and 175 d in the mid-altitude forest in contrast to 119 d at the upper treeline. The number of days with snow cover on the soil, on the other hand, was nearly double at the alpine treeline (202 d) compared to the mid-altitude forest (104 d); only 37 d of snow cover were recorded at the lowermost site. As a consequence, many more days with low soil temperatures (< 3.2 °C) and no protective snow cover occurred at the lowermost site (117 d) compared to the mid-altitude stand (86 d) and the alpine treeline site (44 d).
Table 2. Micro-climatic conditions inside three Nothofagus pumilio stands. Soil moisture contents are mean values ± SE; n = 10. Different letters indicate statistically significant differences (P < 0.05)
Mean daytime vapour pressure deficit (VPD) from November to April was much higher in the lowermost stand compared to the mid-altitude forest (). VPD values at the alpine treeline were on average somewhat higher than in the mid-altitude tall forest. Short-term field measurements of soil moisture during the summer period in 2004 showed similar differences among the stands as in VPD: mean water content in the upper mineral soil (−10 cm) of the xeric treeline stand was almost half of that in the mid-altitude stand. Soil moisture in the alpine treeline stand was also markedly lower compared to the tall forest in middle elevation ().
Above-ground stand structure
A clear response of above-ground structural parameters to the growth conditions of the three Nothofagus stands was found. Mean tree height was largest in the mid-altitude forest and decreased in both upslope and down-slope towards the treeline sites (). Mean tree height was about 11 m at the xeric treeline and 3.5 m at the alpine treeline. Similarly, mean stem diameter was largest in the mid-altitude forest and lowest at the alpine treeline (). Stem density showed the opposite trend with lowest values in the middle stand, followed by the xeric treeline site and a very high number of trees in the alpine treeline stand (). Stand basal area increased from the lowermost to the uppermost stand ().
Figure 2 Tree height and diameter at breast height (dbh) of the three Nothofagus pumilio stands. Values are means ± SE; n = 10 for mean tree height and n = 27, 18, and 9 for dbh of the xeric treeline (XTL), the mid-altitude tall forest (MTF), and the alpine treeline (ATL) stand, respectively. Different letters indicate statistically significant differences between the forests (P < 0.05).
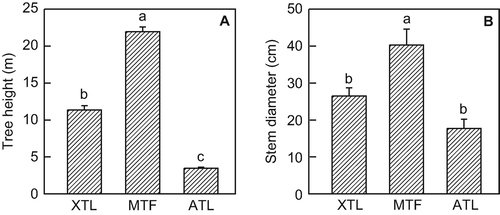
Table 3. Stem density and basal area in three Nothofagus pumilio stands in south Patagonia
Leaf morphology and chemistry
Individual mean leaf area was lowest at the alpine treeline stand (P < 0.10), and tended to increase slightly towards the lowermost xeric site, but this was not significant (). However, specific leaf area (SLA) at the alpine treeline was significantly higher compared to the trees at lower elevations.
Table 4. Structural and chemical leaf traits of the Nothofagus pumilio trees in three stands in southern Patagonia. Values are means ± SE; n = 8 for specific leaf area and leaf nutrient status, and n = 164, 182, and 140 for leaf area in the xeric treeline, mid-altitude tall forest, and alpine treeline stand, respectively. Different letters indicate statistically significant differences (P < 0.05)
Concentration of nitrogen per unit leaf dry mass was lowest in the mid-altitude forest and increased significantly towards both the xeric and the alpine treeline stands (). In contrast, leaf phosphorus concentration decreased strongly from the lowermost stand to the mid-altitude forest and tended to be lowest at the alpine treeline. Hence, N:P ratio of the N. pumilio leaves increased continuously with elevation and reached the highest mean value at the alpine treeline site (P < 0.10).
Leaf and seed production, and leaf area index
Annual leaf production was similar in the xeric and the alpine treeline stands (c. 155 g m−2 year−1), and was about 25% higher in the mid-altitude tall forest (). Conversely, annual production of seeds of N. pumilio in the mid-altitude forest was very low, while the xeric treeline stand showed a markedly higher annual seed production. The highest seed productivity was found in the alpine treeline stand, but due to a large spatial variability, the difference from the xeric treeline forest was not statistically significant.
Table 5. Mean (± SE) annual leaf and seed production in three Nothofagus pumilio stands. n = 8 for specific leaf area and leaf nutrient status and n = 164, 182, and 140 for leaf area for xeric treeline, mid-altitude tall forest, and alpine treeline, respectively. Different letters indicate statistically significant differences (P < 0.05)
Leaf area index of the three stands ranged between 2.0 and 2.6 m2 m−2 (). The two upper Nothofagus stands had nearly identical LAI values, while LAI of the lowermost stand was 25% lower.
Fine root mass and morphology
Standing mass of living and dead fine roots differed strongly between the three Nothofagus stands. Fine root biomass was lowest in the mid-altitude forest while the lowermost xeric stand had a 73% higher fine root biomass (). In the alpine treeline stand, fine root biomass exceeded that of the xeric and the mid-altitude forests by factors of 1.4 and 2.4, respectively. Fine roots in the organic layer of the mid-altitude and the uppermost stand accounted for 10% or 11.4% of the total fine root biomass in the soil profile, while contribution of fine roots in this soil layer was only 4.2% in the lowermost stand.
Figure 4 Fine root biomass (live roots) and fine root necromass (dead roots) in the organic layer and the upper mineral soil of the three Nothofagus pumilio stands. Values are means ± SE; n = 10. Different letters indicate statistically significant differences among the forests in terms of profile totals (P < 0.05). XTL: xeric treeline, MTL: mid-altitude tall forest, ATL: alpine treeline. Note that the scales of the Y axes are different.
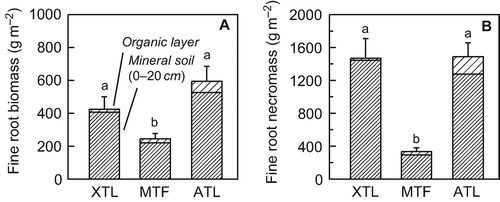
Values of fine root necromass were much higher than those of fine root biomass in all stands (). Similarly to fine root biomass, mean standing fine root necromass was lowest in the mid-altitude forest and increased conspicuously towards the xeric and the alpine treeline sites, where the mass of dead fine roots exceeded that of the mid-altitude forest by a factor of 4.4. Although no differences in fine root necromass was found between the xeric and the alpine treeline stands, the live:dead ratio of fine roots was significantly lower at the xeric treeline (0.26) compared with alpine treeline (0.42). In contrast, the ratio of live to dead fine root mass in the mid-altitude showed a significantly higher value (0.80) compared to both treeline stands.
Differences in fine root morphology between the three stands were small (). Mean fine root diameter, specific root tip abundance, and the degree of ectomycorrhizal infection of the fine root tips showed no statistically significant differences between the three stands. Specific fine root area (SRA) in the organic layer tended to decrease from the mid-altitude forest towards the xeric and the alpine treelines, but not significantly. On the other hand, differences in morphology between fine roots in the organic layer and the mineral soil were more pronounced. Fine roots in the mineral soil had a larger mean root diameter and smaller specific root area, specific root tip abundance, and ectomycorrhizal infection compared to fine roots in the organic layer.
Table 6. Morphological and chemical traits of the fine roots in three Nothofagus pumilio forest stands in southern Patagonia. Values are means ± SE; n = 10 for root morphological parameters and n = 5, 5, and 10 in the organic layer and n = 10, 10, and 9 in the mineral soil for root nutrient status of the xeric treeline, mid-altitude tall forest, and alpine treeline stands, respectively. Different letters indicate statistically significant differences (P < 0.05)
Nitrogen content of the Nothofagus fine roots was more variable among the stands compared to fine root morphological parameters and showed significantly higher values in both the organic layer and the mineral soil of the mid-altitude forest (). At both treeline stands, fine root N content was 30–40% lower compared with the middle tall forest.
Root area index and root tip frequency
The surface area of the fine root system was markedly different between the three Nothofagus stands (). The root area index (RAI) in the xeric treeline stand was twice as high as in the mid-altitude tall forest, and values at the alpine treeline exceeded the RAI of the mid-altitude forest by a factor of > 2.5.
Figure 5 Root area index (RAI) and root tip frequency in the organic layer and the upper mineral soil of the three Nothofagus pumilio stands. Values are means ± SE; n = 10. Different letters indicate statistically significant differences between the forests in terms of profile totals (P < 0.05). XTL: xeric treeline, MTL: mid-altitude tall forest, ATL: alpine treeline.
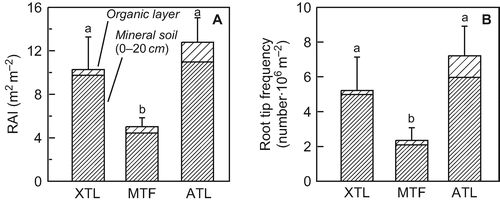
Similarly, root tip frequency was highest at the alpine treeline (7.2 ¥ 106 tips m−2), followed by the xeric treeline stand (5.2 ¥ 106 tips m−2), while the mid-altitude forest had the lowest amount of root tips by far (2.3 ¥ 106 tips m−2, ). However, the recorded differences between the two treeline stands were not significant due to high variability within the stands.
Discussion
Climatic growth conditions
Marked differences in the climatic growth conditions of the Nothofagus pumilio stands were measured along the elevation transect. While mean annual air and soil temperature decreased linearly with elevation, air humidity and soil moisture conditions were found to be most favourable in the tall forest at middle elevation. The temperature lapse rate of 0.97 K 100 m−1 was remarkably high compared to other studies, e.g. 0.55 K 100 m−1 (to 0.70 K 100 m−1) measured at Ushuaia, Tierra del Fuego, by Barrera et al. (Citation2000). The large differences in the thermal regime between the stands might not only be a result of the different elevation but also of the sharp decrease in precipitation (with less cloud cover and higher solar irradiance) from the mountain range towards the steppe in our study region.
Mean annual air temperature of 3.9 °C inside the mid-altitude forest was lower compared to the values measured in tall N. pumilio forests of other studies. Frangi and Richter (Citation1994) and Frangi et al. (Citation1997) have reported a mean annual air temperature of 5.1 °C and Barrera et al. (Citation2000) of 5–6 °C for N. pumilio tall forests near Ushuaia. Mosqueda and Caldentey (Citation1995) and Moll (Citation1981) measured temperature values of 6.0 and 6.6 °C, respectively, for N. pumilio tall forests at the Chilean part of Tierra del Fuego. Similarly, the mean annual air temperature of 5.7 °C of the xeric forest in our study appeared to be comparably low: Schlatter (Citation1994) has given a temperature range of 6.5–7.0 °C for N. pumilio forests at the low elevation distribution limit in Chile. The relatively low air temperatures in our stands might be a result of higher continentality, with temperatures being lower in winter and higher in summer at the Argentine side of the Patagonian Ice Field compared to the Chilean side (Villalba et al. Citation2003).
The thermal conditions at the alpine treeline in our elevation transect, on the other hand, were well in accordance with other studies. The annual mean air temperature in our study was 2.9 °C while Puigdefabregas et al. (Citation1988) have measured 2.4 °C and Barrera et al. (Citation2000) 2.5 °C for alpine treeline stands of N. pumilio near Ushuaia. However, all three records are below the temperature range of 3.5–4.0 °C given by Schlatter (Citation1994) for the upper limit of N. pumilio along the Chilean Andes, again emphasising the importance of continentality on the Argentine side of the Andes. In the past, several thresholds other than a specific annual mean temperature value have been proposed to describe the altitudinal position of the alpine treeline, e.g. air temperature isotherms of a certain month or the growing season (e.g. Köppen Citation1931) or the 3–6 °C summer (growing season) air temperature isotherm at tropical or subtropical mountains (Körner Citation1998, 2003a). More recently, soil temperatures have been advanced to circumscribe alpine treeline positions (e.g. Körner and Hoch Citation2006). Interestingly, Körner and Paulsen (Citation2004) have reported mean growing season root zone temperatures of 9.2 °C in the south-hemispheric cool temperate regions (four Nothofagus sites from New Zealand and Chile), much higher at c. 2.5 K than their global mean of 6.7 °C. They argued that these unusually high temperatures should be considered genus-specific rather than being representative for the climatic treeline position in this region. This cannot be confirmed by the results of our study, where mean growing season soil temperature at the alpine treeline was 6.7 °C and therefore meets exactly the global temperature threshold given by Körner and Paulsen (Citation2004).
In this context, it is noteworthy that the growing season soil temperature of the mid-altitude tall forest in our study was lower by 0.4 K than the alpine treeline site despite a higher mean annual temperature. This was due to the differences in growing season length and number of days with snow cover. Hence, the soil temperature sum of the growing season more likely reflects the change in the thermal conditions with elevation. In our study, soil temperature sum of the growing season in the alpine treeline stand was 28% less compared to the mid-altitude Nothofagus stand.
Above- and below-ground changes towards the xeric Nothofagus site
The xeric Nothofagus pumilio stand had a much lower above-ground biomass than the site at mid elevation, in accordance with various allometric models given in the literature for N. pumilio forests (e.g. Cuevas Citation1979; Pastur et al. Citation1993; Weber Citation1999; Barrera et al. Citation2000; Carabelli et al. Citation2004). Similar trends of above-ground structural changes were documented in other studies on forest–dryland boundaries, for example by De Castro and Kauffman (Citation1998) along a grassland–savanna–forest transect in Brazil. These changes in above-ground forest structure were concordantly assessed as the result of decreased carbon gain due to a higher rate of stomatal limitation at drier sites. In our study LAI, and foliar nitrogen and phosphorus concentrations were different between the mesic and the xeric Nothofagus stands, but not SLA. In their review on above- and below-ground forest attributes of different climatic biomes, Coomes and Grubb (Citation2000) have described low LAI and sclerophyllous leaves as characteristic of dry/arid regions. Li et al. (Citation2000) also found a decrease in SLA in Eucalyptus microtheca seedlings exposed to experimental drought. Analysing leaf morphology of c. 30 Quercus species in the Eastern USA, Abrams (Citation1996) found that species from drier provenances had thicker leaves with lower SLA and higher foliar nitrogen contents than those from mesic regions. Concordant findings were also reported by Killingbeck and Whiteford (Citation1996) and Cunningham et al. (Citation1999). Wright and Westoby (Citation2002) have presented a model in their meta-analysis to disentangle the relationship between costs and benefits of tree leaves with low SLA and high N concentration at low rainfall sites. According to their explanation, the higher leaf N concentration at the xeric treeline in our study could be interpreted as a mechanism to maximise both the use of radiation and water conservation, although the SLA remained unchanged and the LAI decreased from the mesic to the xeric stand.
The changes in the fine root system of the Nothofagus pumilio stands from the mesic to the xeric site were different from those observed above-ground. The carbon investment into the fine root system was markedly higher at the xeric treeline than in the mid-altitude stand. The reports on the below-ground response of trees to soil drought in the literature are not fully consistent. While De Castro and Kauffman (Citation1998) have found a significant increase in fine root biomass along a forest–savanna gradient, Konopka et al. (Citation2007) reported a decrease in fine root biomass of Cryptomeria japonica in response to simulated drought stress. On the other hand, Bakker et al. (Citation2006) found much higher fine root biomass and root tip frequency in drier compared to moister stands of Pinus pinaster. Despite these contradicting findings, there seems to be more consistency in that even though fine root biomass might also decrease towards drier sites in some cases, the root:shoot ratio increases as a response to drier conditions. This was documented in a study on mature Eucalyptus populnea forests along a rainfall gradient in Australia. Similar increases in the root:shoot ratio of tree seedlings as a reaction on drier growth conditions were found by Landhäusser et al. (Citation1996), Li et al. (Citation2000), and Thomas et al. (Citation2000). This converse carbon investment in the above- and the below-ground tree part may reflect an adaptation mechanism to improve the ratio of trees organs in water absorption and water loss.
Above- and below-ground changes towards the alpine Nothofagus treeline
The changes in above- and below-ground stand structure as well as in leaf and fine root morphology of N. pumilio from the mid-altitude to the alpine treeline site were, in principle, similar to the changes towards the xeric treeline. The findings indicated a pronounced decrease in C investment into above-ground tree organs and a marked increase of the size of the fine root system towards the alpine treeline. In contrast, leaf and root morphology changed little. Comparable changes in above-ground structure towards the alpine treeline were reported frequently. Most commonly, this phenomenon has been explained by a reduced carbon gain due to a limitation in photosynthetic activity and/or an imbalance of annual C fixation vs. respiratory loss as a consequence of the short growing season (e.g. Stevens and Fox Citation1991; Körner Citation1998, Citation2003a; Grace et al. Citation2002). However, there is evidence from various studies that photosynthetic activity of trees near the upper treeline is not significantly reduced (Slatyer Citation1978; Häsler Citation1982; Garcia-Nunez et al. Citation2004; Brodersen et al. Citation2006; see also review by Körner Citation2003b). Relatively high concentrations of non-structural carbohydrates (NSC) have been reported from stem and leaf tissues at the treeline (Hoch et al. Citation2002; Hoch and Körner Citation2003, Citation2005; Piper et al. Citation2006; Shi et al. Citation2006), leading to the conclusion that tree growth under cool growth conditions is sink- rather than source-limited. Other hypotheses holding for effects of strong winds, blowing ice, large snow charge, or winter drought are based on data from upper treeline forests of the temperate, boreal, and arctic/antarctic regions (Stevens and Fox Citation1991; Sveinbjörnsson Citation2000) and hence cannot explain the altitudinal trends globally found in mountains (Körner Citation2003a).
For Nothofagus pumilio it is well known that the height of mature trees can vary greatly between > 30 m and < 1 m depending on the environment (e.g. Kalela Citation1941; Schmaltz Citation1991). Changes with altitude in the above-ground stand structure of N. pumilio similar to that found in this study have been reported by Hildebrand-Vogel et al. (Citation1990), Fernández and Pastur (Citation1993), Barrera et al. (Citation2000), and Cuevas (Citation2003).
In contrast, investigations on the fine root system of N. pumilio forests are very few. To our knowledge, the only record in the literature represents a relatively high fine root biomass (1310 g m−2) in a mature tall forest in southern Patagonia (Richter and Frangi Citation1992). Unfortunately, the authors did not report the method they used, hence, no comparison can be made. From the few other studies on elevation effects on the fine root system of forests (Kitayama and Aiba (Citation2002) in Malaysia; Leuschner et al. (Citation2007) in Ecuador; Hertel and Wesche (Citation2008) in Bolivia > 4000 m), it appears that an increase in fine root biomass with elevation (under mesic conditions), as observed in our study, is a general phenomenon. Overall, our study neither confirms the source limitation nor the sink limitation hypothesis. This is true even though the LAI at the treeline stand was not significantly lower than that at the mid-altitude tall forest. Considering that the growing season at the treeline is one-third shorter compared to what the tall forest experiences, it is most likely that the carbon gain of the treeline stand is also lower. Hence, the reduction in above-ground biomass with elevation could be at least partly attributed to this fact; however, it cannot explain the simultaneous increase in fine root biomass. As in the steppe–forest treeline stand, such an enhanced carbon investment into the root system could reflect a mechanism to compensate for missing resources such as water or nutrients. Although several authors have stated that soils at high elevations are often water-saturated (e.g. Vitousek and Sanford Citation1986; Crawford Citation2000), there are studies reporting a significant influence of low water availability on stem growth of Nothofagus pumilio at the treeline (Lara et al. Citation2001, Citation2005; Daniels and Veblen Citation2004). Despite the treeline stand in our study showing some signs of periodically drier conditions in summer, it is unlikely that drought stress is a dominant factor, considering the steep precipitation gradient in the study region.
Another possible explanation for the increased size of the root system could be seen in reduced nutrient availability at the treeline. It is known that nutrient mineralisation slows down under cool soil conditions (e.g. Meentemeyer Citation1977; Sveinbjörnsson et al. Citation1995; Timoney Citation1995; Sveinbjörnsson Citation2000). Although there are some indications that nutrient availability (especially of N and P) could be reduced at the upper treeline site in our study, the differences in soil chemical properties between the mid-altitude forest and the alpine treeline stands were not statistically significant.
The marked increase in carbon investment into the root system at the treeline could also be explained by constraints in water and related nutrient uptake from soil to root and across root cells at low temperatures due to purely physical reasons (Stevens and Fox Citation1991; Sveinbjörnsson Citation2000). Hence, the long-distance transport of water and nutrients in trees requires a disproportional large carbon investment in the root system in cold compared to warmer environments (Stevens and Fox Citation1991). This idea is confirmed by studies of Karlsson and Nordell (Citation1996) and Weih and Karlsson (Citation2001). These authors demonstrated that N uptake of birch seedlings under cold conditions might not only be affected by low nutrient availability, but also by reduced nutrient supply due to the physiological inability of root nutrient uptake.
Whatever mechanisms might be effective, our results highlight the ecological importance of the fine root system at the alpine Nothofagus treeline in Patagonia.
Conclusions
The close proximity of the xeric (steppe–forest) treeline and the alpine treeline, separated by the mesic tall forest offered the unique opportunity in our study region to compare the response of the same tree species to the respective growth conditions. The changes in above- and below-ground forest structure towards the different treelines were surprisingly similar: in both cases, above-ground carbon allocation was reduced while the size of the fine root system increased markedly. However, the extent of the actual responses differed at the two ends of the studied gradient. The decrease in above-ground biomass at the xeric treeline was less pronounced compared to the alpine treeline stand although the xeric stand had a lower LAI. The strong decrease in above-ground biomass at the alpine treeline was paralleled by a larger increase in the size of the fine root system compared to the xeric treeline. The fine roots at the lower treeline, on the other hand, had a markedly lower live:dead ratio indicating a higher fine root mortality compared to the alpine treeline stand. While the differences in leaf and fine root morphology among the stands were surprisingly small, foliar nitrogen contents were higher at both treeline stands compared to the mid-altitude tall forest, but there were signs for lower phosphorus availability at the alpine treeline. Most of our findings indicate clear effects of a drought stress on the above- and below-ground structure of the N. pumilio forest at the xeric treeline. However, it remains open if the altered C allocation towards the root system can solely explain the physiognomy and structure of the xeric treeline or if is limited by seedling establishment and survival in the steppe. At the alpine treeline, the identification of the driving factors that cause the conspicuous shift in carbon investment towards the root system is less clear. In addition to likely temperature constraints on above-ground growth, we conclude from our findings that periodically low soil moisture during the growing season and retarded mineralisation processes may explain enhanced root growth, further influenced by cold-imposed physiological constraints on water and nutrient uptake.
Acknowledgements
This study was funded by the German Ministry of Education and Research (BMBF, project ARG02/025) and the Inter-American Institute for Global Change Research (IAI, CRNI03 and CRNII047), which is supported by the US National Science Foundation (Grant GEO-0452325). We gratefully acknowledge the provision of Ana Srur (IANIGLA, Mendoza) for her support of the leaf morphology analysis as well as Heinz Coners (University of Göttingen) for his help during one of the field campaigns. We also gratefully acknowledge the kind permission by the authorities of the Los Glaciares National Park to conduct our studies.
References
- Abrams , MD . 1996 . Distribution, historical development and ecophysiological attributes of oak species in the eastern United States . Annales des Sciences Forestières , 53 : 487 – 512 .
- Bakker , MR , Augusto , L and Achat , DL . 2006 . Fine root distribution of trees and understory in mature stands of maritime pine (Pinus pinaster) on dry and humid sites . Plant and Soil , 286 : 37 – 51 .
- Barrera , MD , Frangi , JL , Richter , LL , Perdomo , MH and Pinedo , LB . 2000 . Structural and functional changes in Nothofagus pumilio forests along an altitudinal gradient in Tierra del Fuego, Argentina . Journal of Vegetation Science , 11 : 179 – 188 .
- Bauhus , J and Bartsch , N . 1996 . Fine-root growth in beech (Fagus sylvatica) forest gaps . Canadian Journal of Forest Research , 26 : 2153 – 2159 .
- Bowman , RA and Cole , CV . 1978 . An exploratory method for fractionation of organic phosphorus from grassland soils . Soil Science , 25 : 95 – 101 .
- Brodersen , CR , Germino , MJ and Smith , WK . 2006 . Photosynthesis during an episodic drought in Abies lasiocarpa and Picea engelmannii across an alpine treeline . Arctic, Antarctic and Alpine Research , 38 : 34 – 41 .
- Carabelli , F , Bava , J , Momberg , F and Cordone , V . 2004 . Estimación de volumen maderable en bosques de Lenga (Nothofagus pumilio) en la región patagónico de Argentina . Bosque , 25 : 3 – 9 .
- Coomes , DA and Grubb , PJ . 2000 . Impacts of root competition in forests and woodlands: a theoretical framework and review of experiments . Ecological Monographs , 70 : 171 – 207 .
- Crawford , RMM . 2000 . Ecological hazards of oceanic environments . New Phytologist , 147 : 257 – 281 .
- Cuevas , JG . 2003 . Gap characteristics in relation to forest structure and implications for southern beech forest dynamics . Canadian Journal of Forest Research , 33 : 1915 – 1922 .
- Cuevas , RM . 1979 . Determinacion de indice de sitio y variables de produccion para bosques de lenga en Magellanes . Ciencias Forestales , 1 : 47 – 56 .
- Cunningham , SA , Summerhayes , B and Westoby , M . 1999 . Evolutionary divergences in leaf structure and chemistry, comparing rainfall and soil nutrient gradients . Ecological Monographs , 69 : 569 – 588 .
- Daniels , LD and Veblen , TT . 2004 . Spatiotemporal influences of climate on altitudinal treeline in northern Patagonia . Ecology , 85 : 1284 – 1296 .
- De Castro , EA and Kauffman , JB . 1998 . Ecosystem structure in the Brazilian Cerrado: a vegetation gradient of above-ground biomass, root mass and consumption by fire . Journal of Tropical Ecology , 14 : 263 – 283 .
- Donoso , C . 1993 . “ Los bosques templados de Chile y Argentina ” . In Variación, estructura y dinámica , Santiago (Chile) : Santiago University Press .
- Escobar , F , Vidal , F , Garin , C and Naruse , R . 1992 . “ Water balance in the Patagonia Icefield ” . In Glaciological researches in Patagonia, 1990 , Edited by: Naruse , R and Aniya , M . 109 – 119 . Japanese Society of Snow and Ice, Nagoya, Data Center for Glacier Research .
- Fensham , RJ and Kirkpatrick , JB . 1992 . The Eucalypt forest-grassland/grassy woodland boundary in Central Tasmania . Australian Journal of Botany , 40 : 123 – 138 .
- Fernández , C and Pastur , GM . 1993 . Funciones de altura total y area de copa para lenga Nothofagus pumilio (Poepp. et Endl.) (Krasser) en Lago General Vintter – Cerro Colorado, Provincia de Chubut – Argentina . Alcances por clases de exposicion y altitud. Ciencias de Investigaciones Forestales , 7 : 313 – 337 .
- Frangi , JL and Richter , LL . 1994 . Balances hídricos de bosques de Nothofagus de Tierra del Fuego, Argentina . Revista de la Facultad de Agronomia La Plata , 70 : 65 – 79 .
- Frangi , JL , Richter , LL , Barrera , MD and Aloggia , M . 1997 . Decomposition of Nothofagus fallen woody debris in forests of Tierra del Fuego, Argentina . Canadian Journal of Forest Research , 27 : 1095 – 1102 .
- García-Nunez , C , Rada , F , Boero , C , Gonzalez , J , Gallardo , M , Azocar , A , Libermann-Cruz , M , Hilal , M and Prado , F . 2004 . Leaf gas exchange and water relations in Polylepis tarapacana at extreme altitudes in the Bolivian Andes . Photosynthetica , 42 : 133 – 138 .
- George , MF , Burke , MJ , Pellet , HM and Johnson , AG . 1974 . Low temperature exotherms and woody plant distribution . Horticultural Science , 9 : 519 – 522 .
- Glenn , MG , Wagner , WS and Webb , SL . 1991 . Mycorrhizal status of mature red spruce (Picea rubens) in mesic and wetland sites of northwestern New Jersey . Canadian Journal of Forest Research , 21 : 741 – 749 .
- Godbold , DL , Berntson , GM and Bazzaz , FA . 1997 . Growth and mycorrhizal colonization of three North American tree species under elevated atmospheric CO2 . New Phytologist , 137 : 433 – 440 .
- Grace , J , Berninger , F and Nagy , L . 2002 . Impacts of climate change on the tree line . Annals of Botany , 90 : 537 – 544 .
- Häsler , R . 1982 . Net photosynthesis and transpiration of Pinus montana on east and north facing slopes at alpine timberline . Oecologia , 54 : 14 – 22 .
- Hennenberg , KJ , Fischer , F , Kouadio , K , Goetze , D , Orthmann , B , Linsenmair , KE , Jeltsch , F and Porembski , S . 2006 . Phytomass and fire occurrence along forest–savanna transects in the Comoe National Park, Ivory Coast . Journal of Tropical Ecology , 22 : 303 – 311 .
- Hertel , D . 1999 . “ Das Feinwurzelsystem von Rein- und Mischbeständen der Rotbuche: Struktur, Dynamik und interspezifische Konkurrenz ” . In Dissertationes Botanicae 317 , Stuttgart : Gebrüder Borntraeger .
- Hertel , D and Leuschner , C . 2002 . A comparison of four different fine root production estimates with ecosystem carbon balance data in a Fagus–Quercus mixed forest . Plant and Soil , 239 : 237 – 251 .
- Hertel , D and Wesche , K . 2008 . Tropical-moist Polylepis stands at the treeline in E-Bolivia: the effect of elevation on above- and below-ground structure, and regeneration . Trees , 22 : 303 – 315 .
- Hildebrand-Vogel , R , Godoy , R and Vogel , A . 1990 . Subantarctic-Andean Nothofagus pumilio forests . Vegetatio , 89 : 55 – 68 .
- Hoch , G and Körner , C . 2003 . The carbon charging of pines at the climatic treeline: a global comparison . Oecologia , 135 : 10 – 21 .
- Hoch , G and Körner , C . 2005 . Growth, demography and carbon relations of Polylepis trees at the world's highest treeline . Functional Ecology , 19 : 941 – 951 .
- Hoch , G , Popp , M and Körner , C . 2002 . Altitudinal increase of mobile carbon pools in Pinus cembra suggest sink limitation of growth at the Swiss treeline . Oikos , 98 : 361 – 374 .
- Hoffmann , WA , Orthen , B and Franco , AC . 2004 . Constraints to seedling success of savanna and forest trees across the savanna–forest boundary . Oecologia , 140 : 252 – 260 .
- Huntley , BJ and Walker , BH . 1982 . Ecology of tropical savannas , Berlin : Springer .
- Kalela , EK . 1941 . “ Über die Holzarten und die durch klimatischen Verhältnisse verursachten Holzartenwechsel in den Wäldern Ostpatagoniens ” . In Suomalaisen Tiedeakatemian Toimituksia, Helsinki (Series A) IV , Biologica No. 2
- Karlsson , PS and Nordell , KO . 1996 . Effects of soil temperature on nitrogen economy and growth of mountain birch near its presumed low temperature distribution limit . Écoscience , 3 : 183 – 189 .
- Killingbeck , KT and Whitford , WG . 1996 . High foliar nitrogen in desert shrubs: an important ecosystem trait or defective desert doctrine? . Ecology , 77 : 1728 – 1737 .
- Kitayama , K and Aiba , S-I . 2002 . Ecosystem structure and productivity of tropical rain forests along altitudinal gradients with contrasting soil phosphorus pools on Mount Kinabalu, Borneo . Journal of Ecology , 90 : 37 – 51 .
- Konopka , B , Noguchi , K , Sakarta , T , Takahashi , M and Konopkova , Z . 2007 . Effects of simulated drought stress on the fine roots of Japanese cedar (Cryptomeria japonica) in a plantation forest on the Kanto Plain, eastern Japan . Journal of Forest Research , 12 : 143 – 151 .
- Köppen , W . 1931 . Grundriss der Klimakunde , Berlin : De Gruyter .
- Körner , C . 1998 . A re-assessment of high elevation treeline positions and their explanation . Oecologia , 115 : 445 – 459 .
- 2003a , Körner C. Alpine plant life: functional plant ecology of high mountain ecosystems , Berlin : Springer .
- 2003b , Körner C. Carbon limitation in trees . Journal of Ecology , 91 4 – 17 .
- Körner , C and Hoch , G . 2006 . A test of treeline theory on a montane permafrost island . Arctic, Antarctic and Alpine Research , 38 : 113 – 119 .
- Körner , C and Paulsen , J . 2004 . A world-wide study of high altitude treeline temperatures . Journal of Biogeography , 31 : 713 – 732 .
- Landhäusser , SM , Wein , RW and Lange , P . 1996 . Gas exchange and growth of three arctic tree-line tree species under different soil temperature and drought preconditioning regimes . Canadian Journal of Botany , 74 : 686 – 693 .
- Lara , A , Aravena , JC , Villalba , R , Wolodarsky-Franke , A , Luckman , B and Wilson , R . 2001 . Dendroclimatology of high-altitude Nothofagus pumilio forests at their northern distribution limit in the central Andes of Chile . Canadian Journal of Forest Research , 31 : 925 – 936 .
- Lara , A , Villalba , R , Wolodarsky-Franke , A , Aravena , JC , Luckman , BH and Cuq , E . 2005 . Spatial and temporal variation in Nothofagus pumilio growth at tree line along its latitudinal range (35°40′–55′ S) in the Chilean Andes . Journal of Biogeography , 32 : 879 – 893 .
- Leuschner , C , Moser , G , Bertsch , C , Röderstein , M and Hertel , D . 2007 . Large altitudinal increase in tree root/shoot ratio in tropical mountain forests of Ecuador . Basic and Applied Ecology , 8 : 219 – 230 .
- Li , CY , Berninger , F , Koskela , J and Sonninen , E . 2000 . Drought responses of Eucalyptus microtheca provenances depend on seasonality of rainfall in their place of origin . Australian Journal of Plant Physiology , 27 : 231 – 238 .
- Ljungner , E . 1939 . “ A forest section through the Andes of Northern Patagonia ” . In Mision scientifica sueca a la Patagonia 1932–34, informe No. 3. Svensk Botanisk Tidskrift 33
- Meentemeyer , V . 1977 . “ Climatic regulation of decomposition rates of organic matter in terrestrial ecosystems ” . In Environmental chemistry and cycling processes , United States Dept. Energy Symp. Series CONF-760429 Edited by: Adrians , DC and Brisbin , IL . 779 – 789 . Washington : District of Columbia, USA .
- Moll , AU . 1981 . Struktur- und Zuwachsuntersuchungen in magellanischen Nothofagus pumilio-Urwäldern als Grundlage eines Modells der waldbaulichen Überführungsplanung [Dissertation]. , [Göttingen] : University of Göttingen .
- Mosqueda , C and Caldetey , J . 1995 . Aplicación de rodales de Lenga (Nothofagus pumilio) en Magellanes . Ciencias Forestales , 11 : 37 – 49 .
- Müller , SC , Overbeck , GE , Pfadenhauer , J and Pillar , VD . 2007 . Plant functional types of woody species related to fire disturbance in forest-grassland ecotones . Plant Ecology , 189 : 1 – 14 .
- Ohsawa , M . 1990 . An interpretation of latitudinal patterns of forest limits in south and east-Asian mountains . Journal of Ecology , 78 : 326 – 339 .
- Pastur , GM , Fernandez , C , Peri , P and Boyeras , F . 1993 . Ecuaciones estandar de volumen total para la lenga (Nothofagus pumilio) de la margen sur del lago General Vintter y Cerro Colorado, (Provincia de Chubut), Argentina . Revista de la Facultad de Agronomia La Plata , 69 : 5 – 12 .
- Persson , H . 1978 . Root dynamics in a young Scots pine stand in Central Sweden . Oikos , 30 : 508 – 519 .
- Piper , FI , Cavieres , LA , Reyes-Diaz , M and Corcuera , LJ . 2006 . Carbon sink limitation and frost tolerance control performance of the tree Kageneckia angustifolia D. Don (Rosaceae) at the treeline in central Chile . Plant Ecology , 185 : 29 – 39 .
- Prior , LD , Bowman , DMJS and Brook , BW . 2007 . Growth and survival of two north Australian relictual tree species, Allosyncarpia ternata (Myrtaceae) and Callitris intratropica (Cupressaceae) . Ecological Research , 22 : 228 – 236 .
- Puigdefabregas , J , del Barrio , G and Iturraspe , R . 1988 . Regimen termico estacional de un ambiente montanoso en la Tierra del Fuego, con especial atencion al limite superior del bosque . Pirineos , 132 : 37 – 48 .
- Richter , LL and Frangi , JL . 1992 . Bases ecológicas para el manejo del bosque de Nothofagus pumilio de Tierra del Fuego . Revista de la Facultad de Agronomia La Plata , 68 : 35 – 52 .
- Sarmiento , G . 1984 . The ecology of neotropical savannas , Cambridge : Harvard University Press .
- Schlatter , JE . 1994 . Requirimientos de sitio para la lenga, Nothofagus pumilio (Poepp. et Endl.) Krasser . Bosque , 15 : 3 – 10 .
- Schmaltz , J . 1991 . “ Deciduous forests of southern South America ” . In Temperate deciduous forests. Ecosystems of the World 7. , Edited by: Röhrig , E and Ulrich , B . 557 – 578 . Amsterdam : Elsevier .
- Shi , PL , Körner , C and Hoch , G . 2006 . End of season carbon supply status of woody species near the treeline in western China . Basic and Applied Ecology , 7 : 370 – 377 .
- Sibbesen , E . 1977 . A simple ion-exchange resin procedure for extracting plant-available elements from soil . Plant and Soil , 46 : 665 – 669 .
- Slatyer , RO . 1978 . Altitudinal variation in photosynthetic characteristics of snow gum, Eucalyptus pauciflora Sieb. ex Spreng. Relationship between gradients of field temperature and photosynthetic temperature optima in snowy mountains area . Australian Journal of Botany , 26 : 111 – 121 .
- Stevens , GC and Fox , JF . 1991 . The causes of treeline . Annual Review of Ecology and Systematics , 22 : 177 – 191 .
- Sveinbjörnsson , B . 2000 . North American and European treelines: external forces and internal processes controlling position . Ambio , 29 : 388 – 395 .
- Sveinbjörnsson , B , Davis , J , Abadie , W and Butler , A . 1995 . Soil carbon rind nitrogen mineralization at different elevations in the Chugach Mountains of south-central Alaska, USA . Arctic and Alpine Research , 27 : 29 – 37 .
- Thomas , DS , Eamus , D and Shanahan , S . 2000 . Influence of season, drought and xylem ABA on stomatal responses to leaf-to-air vapour pressure difference of trees of the Australian wet–dry tropics . Australian Journal of Botany , 48 : 143 – 151 .
- Timoney , K . 1995 . Tree and tundra cover anomalies in the subarctic forest tundra of northwest Canada . Arctic , 48 : 13 – 21 .
- Tranquillini , W . 1979 . “ Physiological ecology of the alpine timberline ” . In Tree existence at high altitudes with special reference to the European Alps , Ecological Studies 31 Berlin : Springer .
- Van Praag , HJ , Sougnez-Remy , S , Weissen , F and Carletti , G . 1988 . Root tunover in a beech stand of the Belgian Ardennes . Plant and Soil , 105 : 87 – 103 .
- Veblen , TT , Hill , RS and Read , J . 1996 . The ecology and biogeography of Nothofagus forests , New Haven : Yale University Press .
- Villalba , R , Lara , A , Boninsegna , JA , Masiokas , M , Delgado , S , Aravena , JC , Roig , FA , Schmelter , A , Wolodarsky , A and Ripalta , A . 2003 . Large-scale temperature changes across the southern Andes: 20th-century variations in the context of the past 400 years . Climate Change , 59 : 177 – 232 .
- Vitousek , PM and Sanford , RL . 1986 . Nutrient cycling in moist tropical forest . Annual Review of Ecology and Systematics , 17 : 137 – 167 .
- Walter , H . 1985 . Vegetation of the earth, and ecological systems of the geo-biosphere , New York : Springer .
- Weber , M . 1999 . Kohlenstoffvorräte eines Nothofagus-Primarwaldes auf Feuerland . Forstwissenschaftliches Centralblatt , 118 : 156 – 166 .
- Weih , M and Karlsson , PS . 2001 . Growth response of mountain birch to air and soil temperature: is increasing leaf-nitrogen content an acclimation to lower air temperature? . New Phytologist , 150 : 147 – 155 .
- Wright , IJ and Westoby , M . 2002 . Leaves at low versus high rainfall: coordination of structure, lifespan and physiology . New Phytologist , 155 : 403 – 416 .