Abstract
The functional traits of plants in regions of the world with a Mediterranean climate have been shaped to tolerate periods of water deficit. These species are adapted to summer droughts but may not be able to cope with future increases in drought intensity, duration, and/or frequency. Here, we review the mechanisms and traits of drought resistance and recovery of the well-studied holm oak (Quercus ilex), which we propose as a model species for Mediterranean-type ecosystems. Our aim was to understand the differences and links between the responses of Q. ilex to summer droughts, extreme droughts, and long-term drought experiments. A main goal was to provide an integral picture of drought responses across organisational and temporal scales for identifying the most relevant processes that are likely to contribute to determining the future of Mediterranean vegetation. Evidence from long-term drought experiments showed that acclimation processes from the molecular (e.g. epigenetic changes) to the ecosystem level (e.g. reductions in stand density) mitigate the effects of drought. Changes in leaf morphology and hydraulics, leaf-to-shoot allometry, and root functioning are among the key mechanisms for overcoming increasing drought. The duration of drought determines its severity in terms of canopy loss and stem mortality. Although Q. ilex can vigorously resprout after such episodes, its resilience may be subsequently reduced. In the future, higher frequency of return of extreme droughts will challenge thus the capacity of these forests to recover. The insights provided by this review of the complex interplay of processes that determine the response of trees to droughts of different duration, intensity, and frequency will also help us to understand the likely responses of other resprouting angiosperms in seasonally dry ecosystems that share similar functional traits with Q. ilex.
Introduction
The increasing length and intensity of droughts is producing dieback in all forested regions of the world (Allen et al. Citation2010, Citation2015). The vulnerability of forests to droughts of a certain length and intensity depends largely on the climate of the region to which the structure and function of the forests are adapted (Vicente-Serrano et al. Citation2013). Tree species have evolved to cope with climatic variability (including the variability of drought duration), so the mechanisms to resist drought have been adjusted to the climatic variability during the evolutionary histories of the trees. Longer, more intense droughts are forcing trees to display their phenotypic plasticity to its maximum (Matesanz and Valladares Citation2013). Severe modifications of tree function or forest structure may often occur, however, when drought reaches a certain threshold of length and/or intensity. Furthermore, the capacity of recovery after such a disturbance may also be influenced by prior disturbances and by the length of the period until a potential subsequent disturbance (Anderegg et al. Citation2015). The mechanisms of drought resistance, the responses to severe and extreme droughts, and the subsequent recovery include processes from the molecular to the stand level, which operate at different timescales. A focus on the temporal dimension of both the abiotic (duration and intensity of droughts) and biotic (drought-resistance mechanisms and drought effects on plants) factors would thus improve our ability to predict potential changes in the structure and function of vegetation.
In this review, we discuss the effect of drought on a tree species, from the tissue level to stand or ecosystem or level, at range of timescales. Our chosen model tree is the holm oak (Quercus ilex L.), an extensively studied Mediterranean forest tree. Q. ilex possesses some adaptations to drought which the species shares with many other species of Mediterranean-type ecosystems. It is a keystone species in vast areas of the Western Mediterranean Basin, and its dominance is expected to increase to the detriment of other forest species that appear to be more sensitive to aridity, and in response to land abandonment, followed by secondary regrowth by Q. ilex (Carnicer et al. Citation2014). The numerous studies on the responses of Q. ilex to drought have allowed us to construct a case study to understand the drought-induced physiological and ecological processes that all vary in their response times. We explore changes in forest structure and function, including the different feedbacks that result from extreme drought episodes and a sustained increase in aridity.
The World Meteorological Organization (Citation1992) provides two different definitions of drought. The first one defines drought as a prolonged absence or marked deficiency of precipitation. In the regions with a Mediterranean climate, the period with the lowest precipitation coincides with the warmest temperatures every year. This is referred to summer drought in this review, and implies weather conditions similar to the climatic average for a given site. However, drought is also defined as a period of abnormally dry weather, sufficiently long to cause a serious hydrological imbalance. We call this second definition an extreme drought in this study. We also review the response of holm oak forests to drought periods in which the aridity is created or exacerbated by abnormally warm weather (Allen et al. Citation2015).
We first discuss the resistance mechanisms that operate during the summer droughts that typically occur in Mediterranean regions and which last 3–4 months. Second, we review the effects of droughts that exceed the average climatic variability of a given location in terms of duration, seasonality, intensity, or coincide with heatwaves. Third, we report the effects of persistent droughts, such as those produced by experimental rain exclusions. We demonstrate how this information is not only useful for a better understanding of the response of Q. ilex to environmental change but also to show how the temporal scale is crucial for predicting the responses of plants to the increases in drought projected by global circulation models (IPCC Citation2013). The mechanisms and traits that Q. ilex possesses to resist and recover from droughts () are shared by many plant species from Mediterranean-type ecosystems and other drought-prone ecosystems, especially by resprouting angiosperms (Zeppel et al. Citation2015). Therefore, the insights provided by this review are, to some extent, representative of the ecology of Mediterranean vegetation under current climate and can serve for indicating expected climate change responses.
Table 1. Adaptive morphological, physiological, and phenological mechanisms and/or traits of Quercus ilex for withstanding summer droughts.
Quercus ilex as a paradigmatic Mediterranean species
The insights from published studies provided by the present synthesis on the effect of drought at different timescales may be relevant not only for Q. ilex conservation and management but also for woody Mediterranean flora, especially resprouting angiosperms. Q. ilex shares many morphological, physiological, and phenological traits related to the response to water stress () with other species from regions with a Mediterranean climate, such as in California, Chile, Australia, or South Africa. The maximum rooting depth of holm oak is similar to the global average of species in sclerophyllous forests and shrublands (Canadell et al. Citation1996) whereby high investments in below-ground biomass are common in these seasonally dry ecosystems. Similarly, sclerophyllous leaves are also a typical feature of Mediterranean vegetation, indicating the convergent evolution of plant form by several selective forces, including drought (Mooney and Dunn Citation1970). Mediterranean summer drought stops growth in Q. ilex, resulting in bimodal secondary growth patterns (Lempereur et al. Citation2015). A transient quiescence of growth has also been reported for Mediterranean conifers (Camarero et al. Citation2010; Vieira et al. Citation2014) and forbs (Olano et al. Citation2013). Large below- and above-ground bud banks are also characteristically associated with Mediterranean vegetation (Canadell and Lopez-Soria Citation1998; Klimešová and Klimeš Citation2007) and its capacity to recover from fires but are also relevant after extreme droughts (Zeppel et al. Citation2015). Furthermore, resprouting species share a suite of ecophysiological and anatomical traits (Hernández et al. Citation2011; Vilagrosa et al. Citation2014), so their response to drought should be similar, albeit with differences in sensitivity.
Mediterranean summer droughts and the adaptive mechanisms and traits of Q. ilex
Plants in Mediterranean ecosystems have to cope with a double limitation or stressful period: summer drought and winter cold (Terradas Citation1999). The high summer temperatures increase evapotranspiration, which, together with the low precipitation, produces periods with a negative water balance (the difference between precipitation and water losses through evapotranspiration and run-off). The summer drought, within the distributional range of forests dominated by Q. ilex, typically lasts 3–4 months (). The duration and intensity of the summer droughts, however, are highly variable between years (Piñol et al. Citation1991), and some Q. ilex forests thrive in circum-Mediterranean areas where summer drought is rare, such as the coastal areas of the Bay of Biscay (Niinemets Citation2015), and evidence suggests that colonisation in areas with an oceanic climate is recent (Delzon et al. Citation2013)(). Pollen records indicate that Q. ilex persisted during the last glacial period in climatic refugia in the wettest part of its current distribution and gained early dominance in the Holocene in more semi-arid areas of current Morocco and Southern Spain (Reille and Pons Citation1992). Human activity may have contributed to the expansion of Q. ilex in the wettest areas of its distribution during the second half of the Holocene (Reille and Pons Citation1992). Furthermore, this tree species will likely expand its distribution in the future as the climate becomes warmer and the availability of water decreases.
Figure 1. Monthly average difference between the monthly precipitation and the monthly potential evapotranspiration (the climatic water balance) for two forests dominated by Quercus ilex. Data were obtained from meteorological stations located at Torners Valley in the Prades Mountains (930 m a.s.l; 1998–2014) and at Viladrau in the Montseny Mountains (950 m a.s.l. 1995–2014). Potential Evapotranspiration is calculated with the SPEI package in R, based on monthly temperature and incoming solar radiation. Error bars are the standard errors of the means.
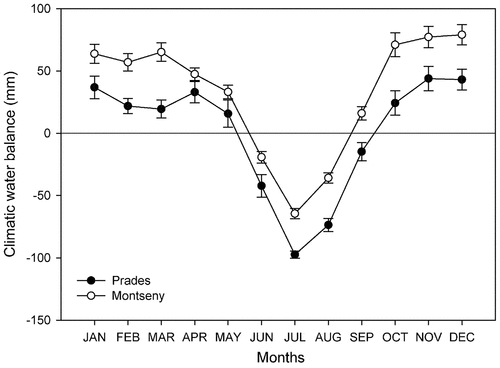
Figure 2. Map of the current distributional range of holm oak (Quercus ilex) in the Mediterranean Basin.
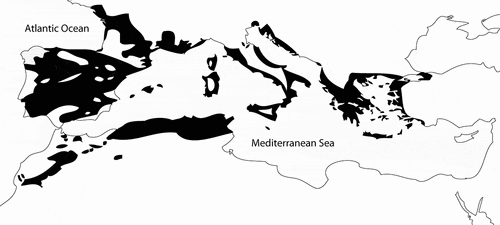
Q. ilex possesses important ecotypic variation (Gratani et al. Citation2003; Peguero-Pina et al. Citation2014) but is generally considered as a species that has several morphological and physiological adaptations for surviving summer droughts (). Summer drought depletes the water in the topsoil, but moisture can still be found in deeper soil layers. Because water is taken up by roots, the development of a deep root system in Q. ilex has been regarded as a morphological adaptation to summer drought (Canadell et al. Citation1999). Woody species in sclerophyllic forests and shrublands (Canadell et al. Citation1996) also have deeper roots than plants in ecosystems without seasonal drought. The effect of drought at sites with shallow soils is determined by the capacity of roots to exploit fissures in the bedrock to access moisture pockets (Lloret et al. Citation2004; Barbeta et al. Citation2015). Deep roots are thus a crucial morphological feature of holm oaks, conferring a competitive advantage over sympatric species, such as Mediterranean pines (Ferrio et al. Citation2003; Comas et al. Citation2015). This ability to tap deep water tables and thus have permanent access to water has also been confirmed at the ecosystem level by data for synchronous eddy covariance and soil water content (Reichstein et al. Citation2002). The possession of this morphological character has led to the categorisation of Q. ilex as a drought-avoiding species (David et al. Citation2004). Tapping deep water table is maximised where soils lay on permeable or fractured bedrock in a relatively flat terrain and with sufficient groundwater recharge during winter months (David et al. Citation2004). In addition to the depth of the root system, the ratio of total root biomass relative to above-ground biomass is associated with water availability. Holm oaks in xeric sites have a higher percentage of below-ground biomass than those in mesic sites; roots account for more than half the total tree biomass in some cases (Canadell et al. Citation1999). Fine roots have been shown to have a higher relative area in the drier sites of Q. ilex (Martin-Stpaul et al. Citation2013). The variability in the length of summer drought is thus offset by the allocation of resources for the growth of below-ground structures to the detriment of aerial organs. As a result, Q. ilex trees withstand the typical summer droughts by ensuring access to water through their extensive root systems and also by maintaining a sufficiently low ratio of above-ground to below-ground biomass.
The morphology of aerial organs has been evolutionarily shaped by summer drought to promote a conservative use of water. Xylem conduits in Q. ilex are narrower than in deciduous Quercus tree species (Villar-Salvador et al. Citation1997), resulting in lower conductivity, although this may be an adaptation to winter cold (Cavender-Bares et al. Citation2005). In contrast, Q. ilex has wider xylem conduits compared to sympatric Mediterranean evergreen species from other genera (Martínez-Vilalta et al. Citation2002), and thus transpire at higher rates than those. Sap flow during summer drought, however, must be substantially reduced (Martínez-Vilalta et al. Citation2003; Barbeta et al. Citation2012) to avoid xylem cavitation, because the xylem anatomy of Q. ilex is not more resistant to embolism than that of deciduous Quercus species in temperate forests (Choat et al. Citation2012). Once water reaches the canopy, sclerophyllous leaves with high specific mass and thickness promote the conservation of cellular water (Bartlett et al. Citation2012). Together, the morphologies of roots, xylems, and leaves partly determine the water-use strategy of Q. ilex and thus its performance under drought.
When morphological adaptations to dry environments are not sufficient to avoid completely any physiological water stress, plants activate several physiological mechanisms, most relevantly stomatal control (Martínez-Vilalta et al. Citation2014). Q. ilex may be the most isohydric species of the genus, and more isohydric than sympatric sclerophyllous shrubs, according to a recent review (Martínez-Vilalta et al. Citation2014). Accordingly, it will reduce stomatal conductance to avoid conductivity losses at less negative water potentials than other congeneric or co-occuring species. Indeed, Q. ilex uses water very conservatively during summer drought, with almost completely closed stomata at midday (Ogaya, Llusià, et al. Citation2014), and the trees consequently have a lower risk of xylem embolism. Nevertheless, embolism in Q. ilex xylem is not rare and can occur either during summer drought (Aguadé, Poyatos, Rosas, et al. Citation2015) or winter frost (Cavender-Bares et al. Citation2005). The soluble fraction of non-structural carbohydrates (NSC) has been hypothesised to be used for maintaining the integrity of the vascular system by osmotic regulation (Sala et al. Citation2012). While it has not been observed in Q. ilex, the unloading of sugars from the phloem to embolised xylem vessels has been proposed as a possible mechanism of embolism repair (Nardini et al. Citation2011). Xylem refilling in Mediterranean plants such as Olea europaea and Laurus nobilis has recently been shown to be ion mediated (Trifilò et al. Citation2014). Interestingly, the proportion of soluble sugars increases during summer drought in the branches (Rosas et al. Citation2013) and leaves (Rivas-Ubach et al. Citation2014) of Q. ilex, suggesting a role for these solutes in drought resistance. Rivas-Ubach et al. (Citation2014) have also found an increase in foliar potassium concentration in summer, which may also be related to osmotic regulation (Babita et al. Citation2010).
Common with a number of other species, in Q. ilex thresholds of water availability (van der Werf et al. Citation2007) and temperature (Deslauriers et al. Citation2008) constrain cambial activity and thus govern the phenology of growth (Mitrakos Citation1980; Lempereur et al. Citation2015). The phenology of primary, secondary, and reproductive growth are thus linked to summer drought and winter cold. A bimodal pattern of stem growth that peaks in spring and autumn is common in Q. ilex (Montserrat-Martí et al. Citation2009; Gutiérrez et al. Citation2011). The onset of the summer cessation of growth has been correlated with spring rainfall (Lempereur et al. Citation2015). This quiescence might be an adaptation to summer drought, but growth would be expected to be plastic enough to continue when summers are sufficiently wet (Albuixech et al. Citation2012). Primary growth, including foliar flushing (Ogaya and Peñuelas Citation2006) and shoot growth (Camarero, Franquesa, et al. Citation2015), occurs mostly before the summer drought, although foliar maturation and shoot growth are completed in summer (Montserrat-Martí et al. Citation2009). Q. ilex thus takes advantage of periods with favourable conditions for growth (Albuixech et al. Citation2012). In contrast to deciduous Mediterranean oaks, it has a peak of growth in autumn, either of the stems (Corcuera et al. Citation2004; Gutiérrez et al. Citation2011; Albuixech et al. Citation2012) or the leaves (Ogaya and Peñuelas Citation2006). In addition, several cohorts of buds up to 3–4 years old are maintained in the branches, which may burst in years with favourable conditions (Alla et al. Citation2012). Overall, Q. ilex has large phenological plasticity of growth-related traits to cope with the marked seasonality in water supply of Mediterranean ecosystems.
The various morphological, physiological adaptations to drought and phenological patterns in response to water shortage manifest themselves at a range of timescales (). Above-ground to below-ground biomass ratios reflect the compound effects of environmental conditions experienced during the lifetime of individuals. For example, contrasting microtopographical conditions can produce morphological differentiation between genetically closely related populations in terms of allocation to roots and above-ground organs (Canadell et al. Citation1999). The environmental conditions experienced during an individual’s lifetime thus contribute to defining its structure and functioning. However, foliar and xylem morphology are determined mainly by the environmental conditions of the periods relevant for development – spring for leaves (Ogaya and Peñuelas Citation2006) and summer for xylem (Abrantes et al. Citation2013). Physiological mechanisms can be activated at even shorter times; the degradation of starch to sugars occurs on a daily basis (Patakas et al. Citation2002), and stomatal response, mediated by … is almost instantaneous (Mcadam and Brodribb Citation2012). Most of these mechanisms are transient responses and can be reversed when the limitation disappears (sensu Wolkovich et al. (Citation2014), ). For example, at the leaf level, stomata can open fully again once soil moisture availability increases, or leaves initiated in wet years may grow less sclerophyllic than those in dry years. However, other features, such as modified xylem anatomy (e.g. the vessel area and density) are persistent responses. Xylem anatomy fluctuates in response to the environmental conditions during the growing season (Campelo et al. Citation2010), but it influences future water transport because water is conducted by the xylem formed over several years (Martínez-Vilalta et al. Citation2003). In those traits that form over longer periods, the plasticity to respond to sudden climatic extremes is thus limited.
Response of Q. ilex to extreme drought
Summer droughts in Mediterranean ecosystems occur every year but with high inter-annual variability in intensity and duration. However, as climatic change is increasing temperatures and their variability (IPCC Citation2013), a positive trend effect (Jentsch et al. Citation2007) is likely to increase the intensity of summer droughts. Extreme droughts, in addition to the cyclic summer droughts, are also expected to become more frequent in the Mediterranean Basin (IPCC Citation2013; Wolkovich et al. Citation2014). Droughts occurring in or extending into the typically wet seasons in the Mediterranean Basin (spring and autumn) have recently increased and these extreme drought events may have larger impacts than more intense summer droughts, because spring and autumn are the optimum periods for growth in holm oaks (Camarero, Franquesa, et al. Citation2015).
Extreme droughts are episodes that (i) represent statistically rare events regarding their intensity, duration, and/or timing and that (ii) cause negative effects that involve at least partial crown damage or an increase in tree mortality rates above the baseline levels. The available literature on drought-induced dieback of holm oaks has reported a great diversity of drought characteristics. Several extreme droughts have been recorded recently in the Iberian Peninsula. For example, the 1993–1995 drought in the North-eastern Iberian Peninsula lasted for 10 months, during which time precipitation was only half of the historical average (Peñuelas et al. Citation2000; Lloret et al. Citation2004). In 2005, drought in the Southern Pyrenees was very intense and aseasonal, peaking in the spring, while another in the same region in 2012 was characterised by less intense but lasted longer (Camarero, Franquesa, et al. Citation2015). Other, more local droughts – such as the drought in Southern Catalonia in 2011 – had severe effects on the vegetation but were restricted to smaller geographic areas (Poyatos et al. Citation2013; Ogaya, Barbeta, et al. Citation2014). All these events had in common the rarity of the historical climatic series, either in drought duration, intensity, and/or timing. Q. ilex populations will suffer important alterations in their use of water and carbon, phenology, primary and secondary growth rates, or mortality rates, depending on the characteristics of the drought.
Drought-induced forest dieback of Q. ilex
Leaf shedding occurs in Q. ilex simultaneously with budburst (Ogaya and Peñuelas Citation2006), whereby some of the old leaves are abscised and substituted by new leaves. Leaf shedding can also be a short-term response to drought, whereby plants adjust their transpiratory area to decreased water availability. Leaf abscission may be able to act as a safety valve to avoid extensive embolism in the xylem of stems, where carbon investment is more expensive (Bucci et al. Citation2012; Pivovaroff et al. Citation2014). Some level of leaf shedding during a summer drought is thus not necessarily indicative of strong water stress and could be added to as a mechanism for regulating the plant–water relations. High foliage loss, however, reduces photosynthetic capacity and may compromise the survival of trees in the long term (Galiano et al. Citation2012). Extensive canopy loss is thus the first indicator of drought-induced forest dieback.
The crown defoliation of Q. ilex stands in the last 15 years has been correlated with a progressive increase in water stress (Carnicer et al. Citation2011) and with extreme droughts (Peñuelas et al. Citation2000; Lloret et al. Citation2004; Galiano et al. Citation2012; Ogaya, Barbeta, et al. Citation2014; Barbeta et al. Citation2015). These events were noticeable by the browning of leaves and the desiccation of entire branches, and, in some cases, of all above-ground organs of an individual. Temperature has increased in most parts of the world over the last 100 years, but no such consistent trend has been observed for precipitation (IPCC Citation2013). As a result, the vulnerability of forests to drought is increasing because hotter temperatures enhance drought severity (Allen et al. Citation2015). Therefore, the inclusion of temperature in the calculation of a drought index is required for comparing the conditions of current and past droughts (Vicente-Serrano et al. Citation2010).
The timing of crown defoliation or stem mortality triggered by drought has been variable (); some events (1994 and 2011) were produced by long-lasting water deficits (Lloret et al. Citation2004; Barbeta et al. Citation2015), whereas others caused a much more negative water balance but for shorter periods (2005) (Barbeta et al. Citation2013). Stem mortality after the event reported by Barbeta et al. (Citation2013) was 5.4% (short-term acute water shortage), but the prolonged drought in 1994 caused 51.3% of stem mortality in stands over the same bedrock, suggesting that Q. ilex has more tolerance to short-term and acute droughts. Confirming this premise will require increasing observational efforts and understanding other factors inherent to forest structure and function that interact with drought to determine the magnitude of its effects. Furthermore, the lack of standardisation between the units to measure the effects of drought on vegetation (crown defoliation at plot or individual level, or mortality rates at stem- or individual-level) prevents a straightforward comparison among events. For example, Barbeta et al. (Citation2013) assessed mortality at the stem level as the lack of resprouting the year after the drought event, because the isolation and identification of genets was not possible. In contrast, Galiano et al. (Citation2012) categorised trees (multi-stemmed individuals) into classes of crown damage and assessed mortality at the genet level in another site. Harmonising the metrics of the effects of drought on forests to provide comparable data sets would be desirable in future studies and useful for assessing large-scale patterns.
Table 2. Characteristics of reported drought-induced dieback in Quercus ilex forests involving crown defoliation and/or stem mortality based on the values the drought index SPEI, calculated as defined in the text. CD, crown defoliation, SM, stem mortality.
Drought characteristics alone (represented in by the multi-scalar drought index) do not appear to fully explain the variability in crown defoliation or stem mortality. The effects of a drought event depend on forest structure (Carnicer et al. Citation2011). Trees in denser stands are more likely to die or be defoliated (Carnicer et al. Citation2011; Galiano et al. Citation2012). Having more stems increases the survival of a multi-stemmed individual but increases individual stem mortality and defoliation (Galiano et al. Citation2012). Taller trees (Lloret et al. Citation2004) with larger basal areas (Galiano et al. Citation2012; Barbeta et al. Citation2013) are the least affected during these events. The form of tree growth (multi-stems, in particular) is affected by management practices and thus has an impact on drought survival. Topography is another critical factor that affects the levels of mortality and defoliation in Q. ilex. The fluctuations of climate are offset, to some extent, by the deep root system of Q. ilex (Canadell et al. Citation1999), but rooting depth can be spatially heterogeneous. Soil is rockier, shallower, and has a lower capacity to store water on steep slopes than on flat ground or in valley bottoms, so the survival of the trees varies across the landscape (Galiano et al. Citation2012). Similarly, access to groundwater is critical for surviving summer drought (Barbeta et al. Citation2015), and the mortality rates during an extreme drought are substantially higher on bedrock types that do not allow the penetration of roots in fissures (Lloret et al. Citation2004).
Trends of crown defoliation and their relationship with drought characteristics
To identify the more critical drought characteristics (in terms of duration and timing) for crown defoliation (i.e. for Q. ilex resistance to drought), we carried out a correlative analysis, using 25 years of data on crown defoliation in the Iberian Peninsula (ICP – International Co-operative Programme on Assessment and Monitoring of Air Pollution Effects on Forests), (Carnicer et al. Citation2011; de la Cruz et al. Citation2014) and the global Standardised Precipitation Evapotranspiration Index (SPEI) database (Vicente-Serrano et al. Citation2010). Using an updated version of the data analysed by Carnicer et al. (Citation2011) but only for Q. ilex, we correlated plot-level data on the annual relative change of crown defoliation with gridded (0.25°) SPEI values at all different months and temporal scales (periods ranging from 1 to 24 months). The correlation heat map presented in indicates that the SPEI and the annual relative change in defoliation level were best correlated for SPEI scales longer than 7 months and during summer months (June, July, and August). More particularly, June SPEI-23 (which is calculated using the water balance, P-PET, of the 23 months prior to June) was best (negatively) correlated, followed by July SPEI-21 and July SPEI-15. These data were collected from 25 years (1987–2013) and were based on 233 plots distributed throughout the range of Q. ilex in the Iberian Peninsula.
Figure 3. Correlation heat map between the annual relative change in crown defoliation and the SPEI values for Iberian Quercus ilex forests (1987–2013, 233 plots). The SPEI duration on the x-axis represents the number of months used to calculate the water balance (e.g. an SPEI duration of 3 in June is calculated using the water balance of the previous April and May and the June itself). The y-axis is the month in which the calculation of SPEI departs from. The panel on the right top indicates the correspondence of the colours in the heat map with the Person’s r coefficient of the correlation between SPEI and defoliation.
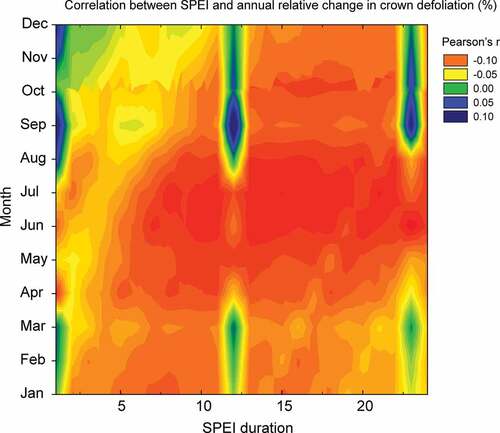
The sensitivity of Q. ilex to drought indicated by crown defoliation was similar to the sensitivity of secondary growth, indicated by tree rings (Pasho et al. Citation2011; Camarero, Franquesa, et al. Citation2015). The correlation between crown defoliation and the SPEI became stronger by June and when accounting for the water balance of the previous winter and spring (). In those tree-ring studies (Gea-Izquierdo et al. Citation2009; Pasho et al. Citation2011; Camarero, Franquesa, et al. Citation2015), growth rates were correlated highest with the water balance in summer. In contrast, crown defoliation was better correlated with longer periods of the water balance (i.e. timescales of the SPEI longer than 7 months), spanning nearly 2 years. This is in agreement with previously reported drivers of defoliation in Iberian tree species at the large scale, which found that the duration of drought is a statistically significant factor (de la Cruz et al. Citation2014). The good correlations of defoliation with the SPEI over long periods are likely to be a result of tapping of groundwater by Q. ilex (Reichstein et al. Citation2002; David et al. Citation2004; Barbeta et al. Citation2015). Certainly, the level of groundwater reserves responds to the accumulated water balance of longer periods than the level of surface soil moisture (Vicente-Serrano et al. Citation2010). Surface soil moisture is subject to strong short-term fluctuations responding to single events of rainfall and to the atmospheric evaporative demand. In contrast, deeper soil layers containing groundwater are recharged during wet and cold periods and are less affected by evaporation. Growth is mainly dependent on the climate of the growing climate that determines the surface soil moisture; whereas, groundwater reserves that have accumulated over periods of more than 1 year are crucial to survive those droughts that deplete surface soil moisture completely.
Winter rainfall may also be related to the replenishment of tree carbon reserves. Sap flow is higher in winter than in summer, but trees do not allocate carbon to above-ground growth in winter (Sánchez-Costa et al. Citation2015). Thus, carbon assimilated during winter is likely to be directed to other functions, such as defence or cold hardening. Such winter carbon could be also stored in the form of starch that may then be remobilised and used during summer drought events to maintain cell turgor and the integrity of the vascular system ().
The sustained increase in crown defoliation in Q. ilex stands since the early 1990s peaked during the droughts of 1994–1995 (). The defoliation levels have since stabilised at around 20%. The SPEI values indicated that droughts of similar intensity occurred after this peak (e.g. 2001 and 2005) but did not trigger larger increases in defoliation than those in the mid-1990s, indicated by the relative change in crown defoliation (). These data not only suggest that increasing aridity has led to a diminished canopy cover but also that once a certain level of defoliation was reached, droughts of equal intensity did not further decrease leaf area. A reduction in leaf area to sapwood area and/or in the ratio of above- and below-ground biomass prompted by droughts could be maintained in the long term. Forests have been hypothesised to acclimate through stabilising processes at the individual (Barbeta et al. Citation2015) and community levels (Lloret et al. Citation2012; Barbeta et al. Citation2013). Other studies, however, have suggested that consecutive droughts can weaken trees and compromise their survival in the long term (Anderegg et al. Citation2015). The results of long-term drought experiments (see below) and long-term studies on the recovery of affected stands following extreme drought events can help to elucidate whether it is the case of stabilising processes or rapid ecosystem change. In addition to the likely acclimation of forest leaf area as a result of reduced water availability, a potential sampling effect on the quantification of defoliation should not be ruled out. Defoliation within the ICP forests programme (Carnicer et al. Citation2011; de la Cruz et al. Citation2014) is estimated in relation to a reference tree supposed to be completely healthy. Over the years, these reference trees may have also suffered some defoliation while still being the relatively healthiest at the plot level. This would have led to the underestimation of defoliation in the most recent years.
Figure 4. Time courses of (a) accumulated crown defoliation and (b) annual change in crown defoliation relative to defoliation from the previous years (red lines). Blue bars are the June SPEIs calculated at a timescale of 23 months, which was the best correlated index. Error bars are the standard errors of the means (N = 233).
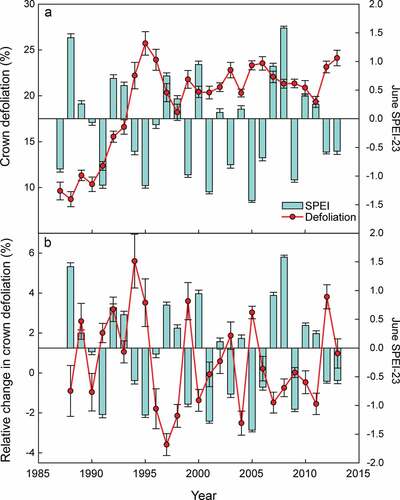
Resprouting after drought-induced biomass loss and the legacy of droughts
Q. ilex has the capacity to resprout from pre-existing buds after a disturbance that causes the loss of biomass (Espelta et al. Citation1999). Resprouts can emerge either from above-ground (canopy) or below-ground (lignotuber) buds, allowing a rapid recovery of the photosynthetic area after a disturbance. Fire, overgrazing, firewood extraction, and coppicing have been the most common disturbances triggering resprouting in Q. ilex stands historically, and extreme droughts will likely increasingly frequent in the coming years, and Q. ilex will likely resprout in response. Extreme droughts cause low tree mortality compared to other disturbances, such as wildfires (Espelta et al. Citation1999). However, extreme droughts can impact roots by causing hydraulic dysfunction (Anderegg et al. Citation2012; Meinzer and McCulloh Citation2013) and deplete below-ground reserves of NSC (Galiano et al. Citation2012) and weaken the resprouting capacity of trees. Still, Q. ilex has shown full canopy recovery within a year after an extreme drought (Ogaya, Barbeta, et al. Citation2014) that induced extensive branch desiccation (Liu et al. Citation2015). The presence of long-lived apical buds confers Q. ilex the capacity to resprout from stems and twigs (, Alla et al. Citation2012). The resilience of trees to drought relies on these above-ground meristemic tissues that are not usually damaged by drought.
Despite the higher resistance to drought-induced mortality of resprouting plants relative to non-resprouting plants (Zeppel et al. Citation2015), the resilience of the former can be weakened if the frequency of extreme droughts increases. Lloret et al. (Citation2004) reported that plants that resprouted weakly after the 1985 drought were more likely to die in the drought of 1994. Forty percent of the plants that completely lost their green foliage in 1994 had not recovered 1 year after the end of the drought. In contrast, only 11.2% of the trees that maintained some of their leaves after the 1994 drought were completely defoliated the year after (Lloret et al. Citation2004). Past droughts thus have a legacy, a carry-over effect, which has been associated with the levels of NSC in lignotubers, the below-ground swollen structures that accumulate starch and form buds (Canadell et al. Citation1999). Galiano et al. (Citation2012) were the first to demonstrate that the resilience of crown condition 7 years after an extreme drought was associated with the amount of NSC in the lignotubers. NSC reserves were depleted 60% more in highly damaged than in healthy trees. Rosas et al. (Citation2013) observed significantly lower lignotuber NSC levels in the most defoliated trees after the drought of 2011, confirming this organ as an important NSC reservoir for overcoming the effects of drought. The period of return of extreme drought appears to be a key factor for determining the resilience of Q. ilex to these droughts. If lignotuber NSC levels are not fully recovered by the following drought, the survival of the tree will be compromised. Q. ilex needs an estimated 20 years to fully replenish the NSC levels of its lignotubers (López et al. Citation2009), so the current frequency of extreme droughts (see time-lapse between droughts of similar intensity in ) is already threatening the future resilience of Q. ilex stands. These extreme droughts will have tangible effect on the levels of NSC reserves only if they result in extensive or total loss of the photosynthetic area, impairing the use of newly assimilated carbon. It is known that the levels of NSC reserves are not constrained by the source activity (i.e. the photosynthetic activity) and that carbon acquisition can be maintained during periods of drought or low temperatures (Körner Citation2003). Therefore, droughts that drastically reduce or stop growth but do not produce leaf shedding will have little effect on the NSC reserves and consequently, the capacity of a tree to resprout in response to drought will remain intact.
The hydraulic deterioration produced by drought-induced xylem cavitation accumulates and persists over several years in aspen trees (Anderegg et al. Citation2013). This so-called cavitation fatigue has been shown to increases the vulnerability of the xylem to embolism of previously drought-affected stems in several species (e.g. Populus angustifolia, P. tremuloides or Helianthus annuus), but in others (e.g. Acer negundo and Alnus incana) stems do not suffer any change in vulnerability (Hacke et al. Citation2001). Other woody plants (Ligustrum vulgare and Viburnum lantana) are even able to acclimate to drought by increasing their resistance to embolism (Beikircher and Mayr Citation2009). This does not seem to be the case of Q. ilex, as was shown by a long-term drought experiment where its vulnerability of xylem to cavitation has slightly but not significantly increased (Limousin, Longepierre, et al. Citation2010). During extremely dry years, such as 1994 in the Iberian Peninsula, the vessels produced by Q. ilex present a smaller diameter than during average years (Corcuera et al. Citation2004). Smaller diameters imply a reduction in the hydraulic conductance and this persists for several years (, Martínez-Vilalta et al. Citation2003). It is highly likely thus that Q. ilex trees will carry over a drought legacy not only in their transpiring area and the levels of NSC but also in their hydraulic function. This legacy will negatively predispose trees to maintain an adequate hydraulic functioning by reducing the potential conductance and increasing the vulnerability to embolism of xylem in the stems.
In addition to the drought legacies related to carbon and water use, drought may also predispose trees to suffer attacks from insects and fungal pathogens (Desprez-Loustau et al. Citation2006; Oliva et al. Citation2014). Importantly, biotic agents are considered a contributing factor in drought-induced forest dieback (Worrall et al. Citation2010). The combined effect of fungal infections and drought-induced defoliation has been associated with lower growth, sapwood depth, and reduced conductivity in Pinus sylvestris individuals (Aguadé, Poyatos, Gomez, et al. Citation2015). Consequently, infected trees will have a lower capacity to recover from drought, exacerbating the hydraulic dysfunction and the NSC depletion. A common fungal pathogen that causes defoliation and mortality in Q. ilex is Phytophthora cinnamomi (Sánchez et al. Citation2002). There is yet no information available on the potential initiating or contributing role of this fungal pathogen to drought-induced forest dieback of Q. ilex or during the recovery after these events. Nonetheless, an experimental study conducted with Q. ilex seedlings demonstrated that plants subjected to drought were more likely to die after being inoculated with this pathogen (Corcobado et al. Citation2014). Interestingly, the most damaging treatment included a prior water-logging treatment, followed first by the inoculation and then by drought treatment. Hence, the sequence of the meteorological extremes matters, as it may interact with the activity of biotic agents and amplify the damage of droughts. For this reason, it is required to conduct experimental and field studies to understand the role of pathogens previously, during and after drought-induced forest dieback of Q. ilex.
The role of forest structure in episodes of forest dieback
The spatial variation in growth and mortality rates of some Mediterranean tree species is largely related to forest structure, more so than to climate (Vilà-Cabrera et al. Citation2011). Indeed, the demographic response by forests to climate disturbances (e.g. extreme droughts) is determined by structural characteristics, such as tree density and stand basal area (Lloret et al. Citation2012; Carnicer et al. Citation2014). The abandonment of forest management practices over the last decades has resulted higher stand densities, which, in turn, increased competition for resources (Vayreda et al. Citation2012). The widely distributed Q. ilex forests are a good example of this process. Coll et al. (Citation2013) showed competition constraints on growth illustrated by lower growth rates of Q. ilex in stands with high basal area, which is supported by less noticeable effects of drought on tree water use in low-density stands compared to high-density stands (Moreno and Cubera Citation2008). In the Iberian Peninsula, many forests are recovering from overexploitation during the 1950s and 1960s, so they are relatively young forests that are increasing in biomass (Vayreda et al. Citation2012). Along with the accumulation of biomass, there is a progressive but slow self-thinning (López et al. Citation2009). Extreme droughts may partly accelerate self-thinning by killing stems of multi-stemmed trees and by suppressing the smaller individuals (Galiano et al. Citation2012; Barbeta et al. Citation2013). Consequently, the stands with high stem density and basal area (and thus with a potential larger degree competition for water) are a priori more prone to suffering large impacts during extreme drought episodes. These impacts may involve extensive biomass loss and the death of individuals and thus, the surviving trees will have less stems per individual, and the stand basal area will be concentrated in a reduced number of individuals. It is likely therefore that the forest structure resulting from a drought impact will present more resistance to drought episodes in the long-term. The convergence towards structures adapted to the new, lower availability of water and more recurrent drought episodes can be expected. Research addressed to predict which the most suitable forest structure is for each subtype of climate and topography should be a primary goal in order to adapt management practices to the ongoing climate change.
Sequence of responses of Q. ilex to long-term field experimental drought
Two long-term drought experiments have been established in natural forests of Q. ilex; the first in 1998 in Prades (North-eastern Iberian Peninsula) and the other in 2003 in the Puébachon State Forest (Southern France). Both experimental designs excluded throughfall over ca. 30% of the plot surface (Limousin, Misson, et al. Citation2010), and run-off at the Prades site was intercepted by ditches along the top edges of the plots (Ogaya and Peñuelas Citation2003). These experiments have produced a large body of information on the responses of Q. ilex forests to imposed moderate but continuous drought, from leaf-level physiology to ecosystem-level demography and fluxes of energy and matter. In fact, the drought imposed by this throughfall exclusion matches the predictions of increasingly drier conditions and the decrease in soil moisture for the Western Mediterranean Basin for the next decades (IPCC Citation2013). Severe drought treatments have often been applied to seedlings and/or saplings, providing a useful mechanistic understanding of the sequence of responses to drought. The still relatively short duration of these experiments, however, compromises the implementation of their results in long-term coupled climate–vegetation models. Seedlings and adults from the same species may also have different water-use strategies (Mediavilla and Escudero Citation2004), so the results of experiments on seedlings may not be comparable with those on adults. Furthermore, the strength of the effect on ecosystems of the same level of stress induced by the pulse-type experimental treatment (e.g. warming, drought, or nutrient addition) decreases over time (Leuzinger et al. Citation2011). In contrast, the results of the long-term drought experiments in Prades and Puébachon would identify more stable effects and responses that can be expected in natural environments. In this section, we review the findings from these two experimental systems (; ).
Table 3. Summary of the effects identified by studies of long-term experimental drought in Quercus ilex forests.
Figure 5. Conceptual scheme of the most relevant effects of the long-term drought experiments in Quercus ilex forests at the tree and ecosystemic levels. The span of the rectangles defines the duration of the effects. The rectangles that surpass 12 years indicate effects that lasted till the present day of the experiment. The position on the x-axis represents the approximate time of appearance. Rectangles with dashed contour lines indicate potential effects that require further study.
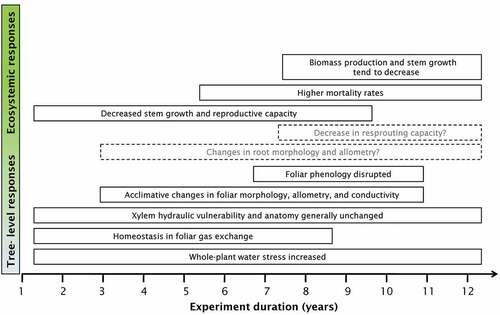
The removal of ca. 30% of the throughfall reduced soil moisture by an average of 13% in the drought-treatment plots in Prades (Liu et al. Citation2015). An analogous reduction of ca. 28% throughfall significantly increased water stress in the drought plots of Puébachon, as it induced a consistent decrease in predawn leaf water potentials of droughted plants (Limousin et al. Citation2009, Citation2012; Limousin, Misson, et al. Citation2010). Predawn water potential is often considered a surrogate of soil water potential (Ritchie and Hinckley Citation1975; Barbeta et al. Citation2012). Midday leaf water potentials, though, did not generally differ between treatment and control (Limousin et al. Citation2009; Ogaya, Barbeta, et al. Citation2014; Barbeta et al. Citation2015). As a short-term response to an increase in xylem tension produced by lower soil water potentials, plants down-regulated their canopy conductance and daily transpiration rates (Limousin et al. Citation2009). Water stress caused by lower amounts of soil moisture thus clearly entailed a decrease in the soil-plant-atmosphere water flow (transpiration), shortly after the treatment had commenced (Limousin et al. Citation2009), showing a rapid acclimation of plant funtioning to the new drier conditions. The consistent decrease in total transpiration was not accompanied by significant modifications of foliar gas exchange, neither the short term (after 1–4 years of drought treatment (Limousin, Misson, et al. Citation2010; Ogaya, Llusià, et al. Citation2014), nor the long term (after 14 years (Ogaya, Llusià, et al. Citation2014; Sperlich et al. Citation2015), indicating physiological homeostasis (Martin-Stpaul et al. Citation2013). It should be noted, however, that measurements of leaf-level gas exchange are instantaneous. The overall decrease in transpiration may not be detectable by gas-exchange analysers that measure at a scale of seconds. Daily (or a longer timescale) integrals of gas-exchange measurements might be able to identify differences between treatments, as do measurements of sap flow (Limousin et al. Citation2009). The concept of leaf-level physiological homeostasis has been challenged in a recent study by the measurement of mesophyllic conductance and daytime respiration (Sperlich et al. Citation2015). These authors have provided evidence that mesophyllic conductance, in addition to stomatal conductance, can acclimate to drought conditions by facilitating the diffusion of CO2 to chloroplasts and that, in the long term, the droughted plants can increase the plasticity of their photosynthetic traits to take advantage of environmentally favourable periods.
The decrease in transpiration reported by Limousin et al. (Citation2009) may seem inconsistent with the results of other drought treatments (Martin-Stpaul et al. Citation2013; Ogaya, Llusià, et al. Citation2014), but the droughted plants at both the Prades and Puébachon experimental sites had reduced leaf areas after 3 years (Ogaya and Peñuelas Citation2006) and 7 years (Limousin et al. Citation2012) of treatment. This reduction was not accompanied by a reduction in sapwood area, and thus the ratio of leaf to sapwood area was decreased at the shoot level (Limousin, Longepierre, et al. Citation2010; Limousin et al. Citation2012), indicating that tree-level transpiration was reduced by decreasing the transpiring (leaf) area, which is also in agreement with the long-term increase in leaf litterfall in the drought treatment observed in Prades (Liu et al. Citation2015). Indeed, leaf-specific conductivity has increased in the long term in droughted plants as a consequence of a decrease in the ratio of leaf to sapwood area (Martin-Stpaul et al. Citation2013). In parallel with the changes in leaf to shoot allometry, individual leaf area and hydraulic conductivity and stem anatomy and hydraulic properties have changed little. Root and branch xylem vulnerability and stem-specific conductivity did not differ significantly in the trees at Puébachon after 6 years of the drought treatment (Limousin, Longepierre, et al. Citation2010). Vessel diameter, frequency, and distribution also did not vary in the same study. Even though wood anatomy is not necessarily associated with xylem vulnerability, the larger picture indicates that the effects of mid- to long-term experimental drought are restricted to foliar anatomy, foliar hydraulic properties, and shoot-to-leaves allometry.
The acclimation of tree-level transpiration (Limousin et al. Citation2009), leaf area (Ogaya and Peñuelas Citation2006), and leaf-to-shoot allometry (Limousin, Longepierre, et al. Citation2010) to drier conditions entails a reduction in total photosynthetic area. Despite the lack of significant differences in rates of carbon assimilation per unit leaf area, the amount of carbon assimilated at the individual or stem level should be reduced. The amount of carbon allocated to growth decreased in Prades after 2 years (Ogaya et al. Citation2003) and 5 years (Ogaya and Peñuelas Citation2007a) of treatment, indicated by significantly lower rates of stem growth in the drought treatments. Likewise, the allocation of carbon to reproduction was also reduced, indicated by a decrease in fruit litterfall (Ogaya and Peñuelas Citation2007b). In contrast, stem growth was never affected in Puébachon after 9 years of drought treatment. Indeed, a recent analysis of a longer data series (11 years) in Prades found that the differences in stem growth tended to dampen over time (Barbeta et al. Citation2013), which has been linked to a potential increase of resources as a consequence of higher mortality rates in the drought plots (Lloret et al. Citation2012; Barbeta et al. Citation2013) and to epigenetic acclimation (Rico et al. Citation2013). That is, the relatively larger decrease in stem density produced by a higher mortality in the drought plots has led to lower competition for water. Furthermore, the physiological and morphological mechanisms of acclimation described above may have provided to droughted plants a greater resistance to naturally occurring extreme droughts, such as those in 2005–2006 and 2011 in Prades (Ogaya, Barbeta, et al. Citation2014). Additionally, the self-thinning related to extreme drought and the drought treatment kills those stems that had previously lower growth rates (Barbeta et al. Citation2013). Consequently, stems with higher growth rates would have been selected by self-thinning in the drought plots, influencing the difference between treatments.
The responses to drought after a decade or more after the onset of the experiments differed slightly from those early in the experiments. Q. ilex has shown it to able to display some mechanisms of acclimation to drought, which would be useful for its persistence under a future drier climate. Overall forest productivity tended to decrease (Liu et al. Citation2015) with the forests reducing their biomass in accordance with the artificially imposed ca. 30% reduction in throughfall. Less dense forests with sparser canopies would thrive under the projected 15% decrease in soil moisture in the Mediterranean Basin (IPCC Citation2013), but as we described above, the effects of extreme droughts are more severe than those of drought experiments that substantially reduce the water availability permanently. Because both an increase in the frequency of extreme drought events and generally lower water availability are likely to occur in the future, continuing investigating the interaction between extreme drought and the acclimation mechanisms of Q. ilex identified by the long-term experiments is necessary. One could expect that a forest adapted to lower water availability would be more resistant to an extreme drought. Trees suffering the effects of a long-term drought experiment, however, stored similar NSC than the control trees (Rosas et al. Citation2013) so their resilience would be equivalent after droughts that cause total canopy loss. In contrast, groundwater contributed less to transpiration in the trees in the control plot during the drought of 2011 (after 12 years of drought treatment), which indicated a relatively stronger depletion of groundwater reserves (Barbeta et al. Citation2015). These reserves respond to the water balance over longer periods, so the chronic water stress imposed by the experiment would prevent them from being ready and available to minimise the negative effects of drought.
Concluding remarks
The objective of this review was to shed light on the key factors that determine potential changes in the distribution, structure, and function of Q. ilex forests in response to increasing droughts, either in the form of extreme events or as a sustained decrease in water availability. This is a critical issue not only for Q. ilex forests but also for other ecosystems dominated by resprouting angiosperms in all the Mediterranean regions of the world that share many of the life-history traits and physiological mechanisms presented in . Analysing the responses to droughts along different timescales, some of the key determinants of drought responses have been described. Gaps in our knowledge that warrant further research, however, still exist. From the dichotomy shown in between developmental time and duration of drought-adaptive traits or mechanisms, we can conclude that not all of these traits or mechanisms could be similarly relevant in the future. In particular, the capacity to modify the phenology of growth and carbon assimilation (Lempereur et al. Citation2015) is a promising compensatory strategy for Q. ilex. In this sense, the limits of plasticity in primary and secondary growth in relation to future drier and warmer conditions may be determinants for the persistence of some populations in their current structure and function. We need to determine if an increase in winter photosynthetic activity and an earlier spring budburst could offset the deleterious effects of a longer summer cessation of growth (Carnicer et al. Citation2013). This seasonal compensation is not only relevant for species sharing similar phenological patterns and life-history traits with Q. ilex, such as Mediterranean woody angiosperms, but also for winter- and drought-deciduous species in temperate and subtropical environments.
The results of field drought experiments have indicated that trees are able to adjust their tree- and stand-level leaf area to a lower availability of water. This adjustment increases their resistance to drought, but we still do not know if they are also more resilient. The depletion of NSC reserves in resprouting organs, such as lignotubers, indicates that the capacity to resprout could be weakened. This weakened resprouting capacity has been observed in the field following episodes of extreme drought. However, the permanent reduction of water availability imposed by field drought experiments does not seem to reduce lignotuber reserves and thus the resprouting capacity (Rosas et al. Citation2013). Still, this does not directly imply that the resistance to extreme drought events associated with the current climatic change remains unchanged after these permanent reductions of water availability. Indeed, a drought in 2011 had a more negative impact on the drought plots (Barbeta et al. Citation2015), and a recent global-scale study evaluating the legacy of extreme droughts on trees has estimated that growth could be reduced for 4 years after a single event (Anderegg et al. Citation2015). In addition, the broad-scale analysis of the drought characteristics that triggered Q. ilex forest dieback presented here shows that the duration of drought is the key parameter in determining the severity of drought effects on Q. ilex forests. The multi-year dynamics of NSC and/or the accumulated hydraulic dysfunction may be behind the fact that persistent droughts cause more crown defoliation and mortality on Q. ilex forests than droughts of short duration regardless of their intensity.
Individuals and plant communities have an extraordinary capacity to accommodate to environmental changes. This is especially true for Quercus ilex and the forests that it dominates. Such long-lived woody species have thrived in the same geographical area at least since the Middle Würm (Reille and Pons Citation1992), facing contrasting climatic conditions. A characteristic that may have allowed such plants to survive these climatic periods is their extensive root system, but when the access to below-ground water pools is impaired by long-lasting droughts forest dieback may occur (Barbeta et al. Citation2015). To sum up, it is still not clear whether the pace of the current climatic change can be matched by acclimation processes and mechanisms showed here. More particularly, shorter periods of return of extreme droughts may be decisive in determining the fate of Q. ilex forests and perhaps other Mediterranean vegetation types. We recommend that future research should keep on addressing the combined effect of consecutive extreme droughts and drier average conditions on the structure and function of plant communities, but with a special emphasis on the resilience after crown damage and on the access to the vital long-lasting deep water pools.
Acknowledgements
The authors thank Jofre Carnicer, Víctor Resco de Dios, Laszlo Nagy and three anonymous reviewers for the insightful comments on earlier versions of this manuscript.
Disclosure statement
No potential conflict of interest was reported by the authors.
Correction Statement
This article has been republished with minor changes. These changes do not impact the academic content of the article.
Additional information
Funding
Notes on contributors
Adrià Barbeta
Adrià Barbeta is a postdoctoral researcher. He is an ecologist, interested in the response of ecosystems to environmental changes. His research focuses on plant ecophysiology, dendroecology, and forest dynamics.
Josep Peñuelas
Josep Peñuelas is a research professor of the National Research Council of Spain and director of the Global Ecology Unit, CREAF-CSIC-UAB, Barcelona. His recent subjects of study include global change, climate change, atmospheric pollution, emission of biogenic volatile organic compounds, remote sensing, plant ecophysiology, and the structure and functioning of terrestrial plants and ecosystems.
References
- Abrantes J, Campelo F, García-González I, Nabais C. 2013. Environmental control of vessel traits in Quercus ilex under Mediterranean climate: relating xylem anatomy to function. Trees - Structure and Function 27:655–662.
- Aguadé D, Poyatos R, Gomez M, Oliva J, Martinez-Vilalta J. 2015. The role of defoliation and root rot pathogen infection in driving the mode of drought-related physiological decline in Scots pine (Pinus sylvestris L.). Tree Physiology 35:229–242.
- Aguadé D, Poyatos R, Rosas T, Martínez-vilalta J. 2015. Comparative drought responses of Quercus ilex L. and Pinus sylvestris L. in a Montane forest undergoing a vegetation shift. Forests 6:2505–2529.
- Albuixech J, Camarero JJ, Montserrat-Marti G. 2012. Dinámica estacional del crecimiento secundario y anatomía del xilema en dos Quercus mediterráneos que coexisten. Forest Systems 21:9–22.
- Alla AQ, Camarero JJ, Maestro-Martínez M, Montserrat-Martí G. 2012. Acorn production is linked to secondary growth but not to declining carbohydrate concentrations in current-year shoots of two oak species. Trees - Structure and Function 26:841–850.
- Allen CD, Breshears DD, McDowell NG. 2015. On underestimation of global vulnerability to tree mortality and forest die-off from hotter drought in the Anthropocene. Ecosphere 6:1–55.
- Allen CD, Macalady AK, Chenchouni H, Bachelet D, McDowell N, Vennetier M, Kitzberger T, Rigling A, Breshears DD, Hogg EH(, et al. 2010. A global overview of drought and heat-induced tree mortality reveals emerging climate change risks for forests. Forest Ecology and Management 259:660–684.
- Anderegg W, Schwalm C, Biondi F, Camarero JJ, Koch G, Litvak M, Ogle K, Shaw JD, Shevliakova E, Williams AP, et al. 2015. Pervasive drought legacies in forest ecosystems and their implications for carbon cycle models W. Science 349:528–532.
- Anderegg WRL, Berry JA, Field CB. 2012. Linking definitions, mechanisms, and modeling of drought-induced tree death. Trends in Plant Science 17:693–700.
- Anderegg WRL, Plavcová L, Anderegg LDL, Hacke UG, Berry JA, Field CB. 2013. Drought’s legacy: multiyear hydraulic deterioration underlies widespread aspen forest die-off and portends increased future risk. Global Change Biology 19:1188–1196.
- Babita M, Maheswari M, Rao LM, Shanker AK, Rao DG. 2010. Osmotic adjustment, drought tolerance and yield in castor (Ricinus communis L.) hybrids. Environmental and Experimental Botany 69:243–249.
- Barbeta A, Mejía-Chang M, Ogaya R, Voltas J, Dawson TE, Peñuelas J. 2015. The combined effects of a long-term experimental drought and an extreme drought on the use of plant-water sources in a Mediterranean forest. Global Change Biology 21:1213–1225.
- Barbeta A, Ogaya R, Peñuelas J. 2012. Comparative study of diurnal and nocturnal sap flow of Quercus ilex and Phillyrea latifolia in a Mediterranean holm oak forest in Prades (Catalonia, NE Spain). Trees 26:1651–1659.
- Barbeta A, Ogaya R, Peñuelas J. 2013. Dampening effects of long-term experimental drought on growth and mortality rates of a Holm oak forest. Global Change Biology 19:3133–3144.
- Bartlett MK, Scoffoni C, Sack L. 2012. The determinants of leaf turgor loss point and prediction of drought tolerance of species and biomes: A global meta-analysis. Ecology Letters 15:393–405.
- Beikircher B, Mayr S. 2009. Intraspecific differences in drought tolerance and acclimation in hydraulics of Ligustrum vulgare and Viburnum lantana. Tree Physiology 29:765–775.
- Bucci SJ, Scholz FG, Campanello PI, Montti L, Jimenez-Castillo M, Rockwell FA, Manna LL, Guerra P, Bernal PL, Troncoso O, et al. 2012. Hydraulic differences along the water transport system of South American Nothofagus species: do leaves protect the stem functionality? Tree Physiology 32:880–893.
- Camarero JJ, Franquesa M, Sangüesa-Barreda G. 2015. Timing of drought triggers distinct growth responses in holm oak: implications to predict warming-induced forest defoliation and growth decline. Forests 6:1576–1597.
- Camarero JJ, Olano JM, Parras A. 2010. Plastic bimodal xylogenesis in conifers from continental Mediterranean climates. The New Phytologist 185:471–480.
- Campelo F, Nabais C, Gutiérrez E, Freitas H, García-González I. 2010. Vessel features of Quercus ilex L. growing under Mediterranean climate have a better climatic signal than tree-ring width. Trees - Structure and Function 24:463–470.
- Canadell J, Djema A, López B, Lloret F, Sabate S, Siscart D, Gracia CA. 1999. Structure and dynamics of the root system. In: Rodà F, Retana J, Gracia CA, Bellot J, editors. Ecology of Mediterranean evergreen oak forests Berlin: Springer. p. 47–59.
- Canadell J, Jackson RB, Ehleringer JB, Mooney HA, Sala OE, Schulze E-D. 1996. Maximum rooting depth of vegetation types at the global scale. Oecologia 108:583–595.
- Canadell J, Lopez-Soria L. 1998. Lignotuber reserves support regrowth following clipping of two Mediterranean shrubs. Functional Ecology 12:31–38.
- Carnicer J, Barbeta A, Sperlich D, Coll M, Peñuelas J. 2013. Contrasting trait syndromes in angiosperms and conifers are associated with different responses of tree growth to temperature on a large scale. Frontiers in Plant Science 4:409.
- Carnicer J, Coll M, Ninyerola M, Pons X, Sanchez G, Penuelas J. 2011. Widespread crown condition decline, food web disruption, and amplified tree mortality with increased climate change-type drought. Proceedings of the National Academy of Sciences of the United States of America 108:1474–1478.
- Carnicer J, Coll M, Pons X, Ninyerola M, Vayreda J, Peñuelas J. 2014. Large-scale recruitment limitation in Mediterranean pines: the role of Quercus ilex and forest successional advance as key regional drivers. Global Ecology and Biogeography 23:371–384.
- Cavender-Bares J, Cortes P, Rambal S, Joffre R, Miles B, Rocheteau A. 2005. Summer and winter sensitivity of leaves and xylem to minimum freezing temperatures: a comparison of co-occurring Mediterranean oaks that differ in leaf lifespan. New Phytologist 168:597–612.
- Choat B, Jansen S, Brodribb TJ, Cochard H, Delzon S, Bhaskar R, Bucci SJ, Feild TS, Gleason SM, Hacke UG, et al. 2012. Global convergence in the vulnerability of forests to drought. Nature 491:752–755.
- Coll M, Peñuelas J, Ninyerola M, Pons X, Carnicer J. 2013. Multivariate effect gradients driving forest demographic responses in the Iberian Peninsula. Forest Ecology and Management 303:195–209.
- Comas C, Castillo J, Voltas J, Ferrio JP. 2015. Point processes statistics of stable isotopes : analysing water uptake patterns in a mixed stand of Aleppo pine and Holm oak. Forest Systems 24:1–13.
- Corcobado T, Cubera E, Juárez E, Moreno G, Solla A. 2014. Drought events determine performance of Quercus ilex seedlings and increase their susceptibility to Phytophthora cinnamomi. Agricultural and Forest Meteorology 192-193:1–8.
- Corcuera L, Camarero JJ, Gil-Pelegrín E. 2004. Effects of a severe drought on Quercus ilex radial growth and xylem anatomy. Trees - Structure and Function 18:83–92.
- David TS, Ferreira MI, Cohen S, Pereira JS, David JS. 2004. Constraints on transpiration from an evergreen oak tree in southern Portugal. Agricultural and Forest Meteorology 122:193–205.
- de la Cruz AC, Gil PM, Fernández-Cancio Á, Minaya M, Navarro-Cerrillo RM, Sánchez-Salguero R, Grau JM. 2014. Defoliation triggered by climate induced effects in Spanish ICP forests monitoring plots. Forest Ecology and Management 331:245–255.
- Delzon S, Urli M, Samalens JC, Lamy JB, Lischke H, Sin F, Zimmermann NE, Porté AJ. 2013. Field evidence of colonisation by holm oak, at the Northern margin of its distribution range, during the anthropocene period. PLoS ONE 8:1–8.
- Deslauriers A, Rossi S, Anfodillo T, Saracino A. 2008. Cambial phenology, wood formation and temperature thresholds in two contrasting years at high altitude in southern Italy. Tree Physiology 28:863–871.
- Desprez-Loustau M-L, Marçais B, Nageleisen L-M, Piou D, Vannini A. 2006. Interactive effects of drought and pathogens in forest trees. Annals of Forest Science 63:597–612.
- Espelta J, Sabaté S, Retana J. 1999. Resprouting dynamics. In: Rodà F, Retana J, Gracia CA, Bellot J, editors. Ecology of Mediterranean evergreen oak forests Berlin: Springer. p. 61–73.
- Ferrio JP, Florit A, Vega A, Serrano L, Voltas J. 2003. Delta(13)C and tree-ring width reflect different drought responses in Quercus ilex and Pinus halepensis. Oecologia 137:512–518.
- Galiano L, Martinez-Vilalta J, Sabate S, Lloret F. 2012. Determinants of drought effects on crown condition and their relationship with depletion of carbon reserves in a Mediterranean holm oak forest. Tree Physiology 32:478–489.
- Gea-Izquierdo G, Martín-Benito D, Cherubini P, Cañellas I. 2009. Climate-growth variability in Quercus ilex L. west Iberian open woodlands of different stand density. Annals of Forest Science 66:802.
- Gratani L, Meneghini M, Pesoli P, Crescente MF. 2003. Structural and functional plasticity of Quercus ilex seedlings of different provenances in Italy. Trees - Structure and Function 17:515–521.
- Gutiérrez E, Campelo F, Camarero J, Ribas M, Muntán E, Nabais C, Freitas H. 2011. Climate controls act at different scales on the seasonal pattern of Quercus ilex L. stem radial increments in NE Spain. Trees Structure and Function 25:637–646.
- Hacke UG, Stiller V, Sperry JS, Pittermann J, McCulloh KA. 2001. Cavitation Fatigue. Embolism and refilling cycles can weaken the cavitation resistance of xylem. Plant Physiology 125:779–786.
- Hernández EI, Pausas JG, Vilagrosa A. 2011. Leaf physiological traits in relation to resprouter ability in the Mediterranean Basin. Plant Ecology 212:1959–1966.
- IPCC. 2013. Climate change 2013: the physical science basis. Contribution of working group I to the fifth assessment report of the intergovernmental panel on climate change Cambridge, UK: Cambridge University Press.
- Jentsch A, Kreyling J, Beierkuhnlein C. 2007. A new generation of events, not trends experiments. Frontiers in Ecology and the Environment 5:365–374.
- Klimešová J, Klimeš L. 2007. Bud banks and their role in vegetative regeneration - A literature review and proposal for simple classification and assessment. Perspectives in Plant Ecology, Evolution and Systematics 8:115–129.
- Körner C. 2003. Carbon limitation in trees. Journal of Ecology 91:4–17.
- Lempereur M, Martin-stpaul NK, Damesin C, Joffre R, Ourcival J, Rocheteau A, Rambal S. 2015. Growth duration is a better predictor of stem increment than carbon supply in a Mediterranean oak forest : implications for assessing forest productivity under climate change. New Phytologist 207:579–590.
- Leuzinger S, Luo Y, Beier C, Dieleman W, Vicca S, Körner C. 2011. Do global change experiments overestimate impacts on terrestrial ecosystems? Trends in Ecology & Evolution 26:236–241.
- Limousin J-M, Longepierre D, Huc R, Rambal S. 2010. Change in hydraulic traits of Mediterranean Quercus ilex subjected to long-term throughfall exclusion. Tree Physiology 30:1026–1036.
- Limousin J-M, Misson L, Lavoir A, Martin NK. 2010. Do photosynthetic limitations of evergreen Quercus ilex leaves change with long-term increased drought severity ? Plant Cell and Environment 33:863–875.
- Limousin JM, Rambal S, Ourcival JM, Rocheteau A, Joffre R, Rodríguez-Cortina R. 2009. Long-term transpiration change with rainfall decline in a Mediterranean Quercus ilex forest. Global Change Biology 15:2163–2175.
- Limousin J-M, Rambal S, Ourcival J-M, Rodríguez-Calcerrada J, Pérez-Ramos IM, Rodríguez-Cortina R, Misson L, Joffre R. 2012. Morphological and phenological shoot plasticity in a Mediterranean evergreen oak facing long-term increased drought. Oecologia 169:565–577.
- Liu D, Ogaya R, Barbeta A, Yang X, Peñuelas J. 2015. Contrasting impacts of continuous moderate drought and episodic severe droughts on the aboveground-biomass increment and litterfall of three coexisting Mediterranean woody species. Global Change Biology 21:4196–4209.
- Lloret F, Escudero A, Iriondo JM, Martínez-Vilalta J, Valladares F. 2012. Extreme climatic events and vegetation: the role of stabilizing processes. Global Change Biology 18:797–805.
- Lloret F, Siscart D, Dalmases C. 2004. Canopy recovery after drought dieback in holm-oak Mediterranean forests of Catalonia (NE Spain). Global Change Biology 10:2092–2099.
- López BC, Gracia CA, Sabaté S, Keenan T. 2009. Assessing the resilience of Mediterranean holm oaks to disturbances using selective thinning. Acta Oecologica 35:849–854.
- Martínez-Vilalta J, Mangirón M, Ogaya R, Sauret M, Serrano L, Peñuelas J, Piñol J. 2003. Sap flow of three co-occurring Mediterranean woody species under varying atmospheric and soil water conditions. Tree Physiology 23:747–758.
- Martínez-Vilalta J, Poyatos R, Aguadé D, Retana J, Mencuccini M. 2014. A new look at water transport regulation in plants. New Phytologist 204:105–115.
- Martínez-Vilalta J, Prat E, Oliveras I, Piñol J. 2002. Xylem hydraulic properties of roots and stems of nine Mediterranean woody species. Oecologia 133:19–29.
- Martin-Stpaul NK, Limousin J-M, Vogt-Schilb H, Rodríguez-Calcerrada J, Rambal S, Longepierre D, Misson L. 2013. The temporal response to drought in a Mediterranean evergreen tree: comparing a regional precipitation gradient and a throughfall exclusion experiment. Global Change Biology 19:2413–2426.
- Matesanz S, Valladares F. 2013. Ecological and evolutionary responses of Mediterranean plants to global change. Environmental and Experimental Botany 103:53–67.
- Mcadam SA, Brodribb TJ. 2012. Stomatal innovation and the rise of seed plants. Ecology Letters 15:1–8.
- Mediavilla S, Escudero A. 2004. Stomatal responses to drought of mature trees and seedlings of two co-occurring Mediterranean oaks. Forest Ecology and Management 187:281–294.
- Meinzer FC, McCulloh KA. 2013. Xylem recovery from drought-induced embolism: where is the hydraulic point of no return? Tree Physiology 33:331–334.
- Mitrakos K. 1980. A theory for Mediterranean plant life. Acta Oecologica 1:245–252.
- Montserrat-Martí G, Camarero JJ, Palacio S, Pérez-Rontomé C, Milla R, Albuixech J, Maestro M. 2009. Summer-drought constrains the phenology and growth of two coexisting Mediterranean oaks with contrasting leaf habit: implications for their persistence and reproduction. Trees - Structure and Function 23:787–799.
- Mooney HA, Dunn EL. 1970. Convergent evolution of Mediterranean-climate evergreen sclerophyll shrubs. Evolution 24:292–303.
- Moreno G, Cubera E. 2008. Impact of stand density on water status and leaf gas exchange in Quercus ilex. Forest Ecology and Management 254:74–84.
- Nardini A, Lo Gullo MA, Salleo S. 2011. Refilling embolized xylem conduits: is it a matter of phloem unloading? Plant Science: An International Journal of Experimental Plant Biology 180:604–611.
- Niinemets Ü. 2015. Is there a species spectrum within the world-wide leaf economics spectrum ? Major variations in leaf functional traits in the Mediterranean sclerophyll Quercus ilex. New Phytologist 205:79–96.
- Ogaya R, Barbeta A, Başnou C, Peñuelas J. 2014. Satellite data as indicators of tree biomass growth and forest dieback in a Mediterranean holm oak forest. Annals of Forest Science 72:135.
- Ogaya R, Llusià J, Barbeta A, Asensio D, Liu D, Alessio GA, Peñuelas J. 2014. Foliar CO2 in a holm oak forest subjected to 15 years of climate change simulation. Plant Science 226:101–107.
- Ogaya R, Peñuelas J. 2003. Comparative field study of Quercus ilex and Phillyrea latifolia: photosynthetic response to experimental drought conditions. Environmental and Experimental Botany 50:137–148.
- Ogaya R, Peñuelas J. 2004. Phenological patterns of Quercus ilex, Phillyrea latifolia, and Arbutus unedo growing under a field experimental drought. Écoscience 11:263–270.
- Ogaya R, Peñuelas J. 2006. Contrasting foliar responses to drought in Quercus ilex and Phillyrea latifolia. Biologia Plantarum 50:373–382.
- Ogaya R, Peñuelas J. 2007a. Tree growth, mortality, and above-ground biomass accumulation in a holm oak forest under a five-year experimental field drought. Plant Ecology 189:291–299.
- Ogaya R, Peñuelas J. 2007b. Species-specific drought effects on flower and fruit production in a Mediterranean holm oak forest. Forestry 80:351–357.
- Ogaya R, Peñuelas J, Asensio D, Llusià J. 2011. Chlorophyll fluorescence responses to temperature and water availability in two co-dominant Mediterranean shrub and tree species in a long-term field experiment simulating climate change. Environmental and Experimental Botany 71:123–127.
- Ogaya R, Peñuelas J, Martínez-Vilalta J, Mangirón M. 2003. Effect of drought on diameter increment of Quercus ilex, Phillyrea latifolia, and Arbutus unedo in a holm oak forest of NE Spain. Forest Ecology and Management 180:175–184.
- Olano JM, Almería I, Eugenio M, von Arx G. 2013. Under pressure: how a Mediterranean high-mountain forb coordinates growth and hydraulic xylem anatomy in response to temperature and water constraints. Functional Ecology 27:1295–1303.
- Oliva J, Stenlid J, Martínez-Vilalta J. 2014. The effect of fungal pathogens on the water and carbon economy of trees : implications for drought-induced mortality included in theoretical models for drought induced mortality. New Phytologist 203:1028–1035.
- Pasho E, Camarero JJ, de Luis M, Vicente-Serrano SM. 2011. Impacts of drought at different time scales on forest growth across a wide climatic gradient in north-eastern Spain. Agricultural and Forest Meteorology 151:1800–1811.
- Patakas A, Nikolaou N, Zioziou E, Radoglou K, Noitsakis B. 2002. The role of organic solute and ion accumulation in osmotic adjustment in drought-stressed grapevines. Plant Science 163:361–367.
- Peguero-Pina JJ, Sancho-Knapik D, Barrón E, Camarero JJ, Vilagrosa A, Gil-Pelegrín E. 2014. Morphological and physiological divergences within Quercus ilex support the existence of different ecotypes depending on climatic dryness. Annals of Botany 114:301–313.
- Peñuelas J, Filella I, Lloret F, Piñol J, Siscart D. 2000. Effects of a severe drought on water and nitrogen use by Quercus ilex and Phillyrea latifolia. Biologia Plantarum 43:47–53.
- Piñol J, Lledó MJ, Escarré A. 1991. Hydrological balance of two Mediterranean forested catchments (Prades, Northeast Spain). Hydrological Sciences Journal 36:95–107.
- Pivovaroff AL, Sack L, Santiago LS. 2014. Coordination of stem and leaf hydraulic conductance in southern California shrubs: a test of the hydraulic segmentation hypothesis. New Phytologist 203:842–850.
- Poyatos R, Aguadé D, Galiano L, Mencuccini M, Martínez-Vilalta J. 2013. Drought-induced defoliation and long periods of near-zero gas exchange play a key role in accentuating metabolic decline of Scots pine. New Phytologist 200:388–401.
- Reichstein M, Tenhunen JD, Roupsard O, Rambal S, Miglietta F, Peressotti A. 2002. Severe drought effects on ecosystem CO2 and H2O fluxes at three Mediterranean evergreen sites: revision of current hypotheses? Global Change Biology 8:999–1017.
- Reille M, Pons A. 1992. The ecological significance of slerophyllous oak forests in the western part of the Mediterranean basin: a note on pollen analytical data. In: Romane F, Terradas J, editors. Quercus ilex L. ecosystems: function, dynamics and management Dordrecht: Kluwer; p. 13–18.
- Rico L, Ogaya R, Barbeta A, Peñuelas J. 2013. Changes in DNA methylation fingerprint of Quercus ilex trees in response to experimental field drought simulating projected climate change. Plant Biology 16:419–427.
- Ritchie GA, Hinckley TM. 1975. The pressure chamber as an instrument for ecological research. Advances in Ecological Research 9:165–254.
- Rivas-Ubach A, Gargallo-Garriga A, Sardans J, Oravec M, Mateu-Castell L, Pérez-Trujillo M, Parella T, Ogaya R, Urban O, Peñuelas J. 2014. Drought enhances folivory by shifting foliar metabolomes in Quercus ilex trees. New Phytologist 202:874–885.
- Rodríguez-Calcerrada J, Martin-Stpaul NK, Lempereur M, Ourcival JM, del Rey MC, Joffre R, Rambal S. 2014. Stem CO2 efflux and its contribution to ecosystem CO2 efflux decrease with drought in a Mediterranean forest stand. Agricultural and Forest Meteorology 195–196:61–72.
- Rosas T, Galiano L, Ogaya R, Peñuelas J, Martínez-Vilalta J. 2013. Dynamics of non-structural carbohydrates in three Mediterranean woody species following long-term experimental drought. Frontiers in Plant Science 4:1–16.
- Sala A, Woodruff DR, Meinzer FC. 2012. Carbon dynamics in trees: feast or famine? Tree Physiology 32:764–775.
- Sánchez ME, Caetano P, Ferraz J, Trapero A. 2002. Phytophtora disease of Quercus ilex in south-western Spain. Forest Pathology 32:5–18.
- Sánchez-Costa E, Poyatos R, Sabaté S. 2015. Contrasting growth and water use strategies in four co-occurring Mediterranean tree species revealed by concurrent measurements of sap flow and stem diameter variations. Agricultural and Forest Meteorology 207:24–37.
- Sperlich D, Barbeta A, Ogaya R, Sabate S, Penuelas J. 2015. Balance between carbon gain and loss under long-term drought: impacts on foliar respiration and photosynthesis in Quercus ilex L. Journal of Experimental Botany 67:821–833.
- Terradas J. 1999. Holm oak and holm oak forests: an introduction. In: Rodà F, Retana J, Gracia C, Bellot J, editors. Ecology of Mediterranean evergreen oak forests Berlin: Springer Berlin /Heidelberg. p. 3–14.
- Trifilò P, Barbera PM, Raimondo F, Nardini A, Lo Gullo MA, Meinzer F. 2014. Coping with drought-induced xylem cavitation: Coordination of embolism repair and ionic effects in three Mediterranean evergreens. Tree Physiology 34:109–122.
- Van Der Werf GW, Sass-Klaassen UGW, Mohren GMJ. 2007. The impact of the 2003 summer drought on the intra-annual growth pattern of beech (Fagus sylvatica L.) and oak (Quercus robur L.) on a dry site in the Netherlands. Dendrochronologia 25:103–112.
- Vayreda J, Martinez-Vilalta J, Gracia M, Retana J. 2012. Recent climate changes interact with stand structure and management to determine changes in tree carbon stocks in Spanish forests. Global Change Biology 18:1028–1041.
- Vicente-Serrano SM, Beguería S, López-Moreno JI. 2010. A multiscalar drought index sensitive to global warming: the standardized precipitation evapotranspiration index. Journal of Climate 23:1696–1718.
- Vicente-Serrano SM, Gouveia C, Camarero JJ, Beguería S, Trigo R, López-Moreno JI, Azorín-Molina C, Pasho E, Lorenzo-Lacruz J, Revuelto J, et al. 2013. Response of vegetation to drought time-scales across global land biomes. Proceedings of the National Academy of Sciences of the United States of America 110:52–57.
- Vieira J, Rossi S, Campelo F, Freitas H, Nabais C. 2014. Xylogenesis of Pinus pinaster under a Mediterranean climate. Annals of Forest Science 71:71–80.
- Vilà-Cabrera A, Martínez-Vilalta J, Vayreda J, Retana J. 2011. Structural and climatic determinants of demographic rates of scots pine forests across the Iberian Peninsula. Ecological Applications: a Publication of the Ecological Society of America 21:1162–1172.
- Vilagrosa A, Hernández EI, Luis VC, Cochard H, Pausas JG. 2014. Physiological differences explain the co-existence of different regeneration strategies in Mediterranean ecosystems. New Phytologist 201:1277–1288.
- Villar-Salvador P, Castro-Díez P, Pérez-Rontomé C, Montserrat-Martí G. 1997. Stem xylem features in three Quercus (Fagaceae) species along a climatic gradient in NE Spain. Trees 12:90–96.
- Wolkovich EM, Cook BI, McLauchlan KK, Davies TJ. 2014. Temporal ecology in the Anthropocene. Ecology Letters 17:1365–1379.
- World Meteorological Organization. 1992. International meteorlogical vocabulary. Geneva: Secretariat of the World Meteorological Organization. No. 182.
- Worrall JJ, Marchetti SB, Egeland L, Mask RA, Eager T, Howell B. 2010. Effects and etiology of sudden aspen decline in southwestern Colorado, USA. Forest Ecology and Management 260:638–648.
- Zeppel MJB, Harrison SP, Adams HD, Kelley DI, Li G, Tissue DT, Dawson TE, Fensham R, Belinda E, Palmer A, et al. 2015. Drought and resprouting plants. New Phytologist 206:583–589.