Abstract
Background: Prescribed burning in peatlands is controversial due to concerns over damage to their ecological functioning, particularly regarding their key genus Sphagnum. However, empirical evidence is scarce.
Aims: The aim of the article is to quantify Sphagnum recovery following prescribed burns.
Methods: We completed nine fires at a raised bog in Scotland, achieving a range of fire severities by simulating drought in some plots. We measured Sphagnum cover and chlorophyll fluorescence Fv/Fm ratio (an estimate of photosynthetic capacity) up to 36 months post-fire.
Results: Cover of dominant Sphagnum capillifolium was similar in unburnt and burnt plots, likely due to its high moisture content which prevented combustion. Burning decreased S. capillifolium Fv/Fm 5 months after fire from 0.67 in unburnt plots to 0.44 in low fire severity plots and 0.24 in higher fire severity (drought) plots. After 22 months, Fv/Fm in burnt plots showed a healthy photosynthetic capacity of 0.76 and no differences between severity treatments. Other Sphagnum species showed similar post-fire recovery though their low overall abundance precluded formal statistical analysis.
Conclusions: S. capillifolium is resilient to low–moderate fire severities and the same may be true for a number of other species. This suggests that carefully applied managed burning can be compatible with the conservation of peatland ecosystem function.
Introduction
Peatlands deliver important ecosystem services such as the provision of fresh water and regulation of its quality and supporting services such as carbon sequestration, soil (peat) formation, nutrient cycling and primary production (Joosten and Clarke Citation2002; Millennium Ecosystem Assessment Citation2005). Peatlands also provide habitat for specialist species, the maintenance of which ensures that humans can continue to directly benefit from these ecosystems (Rydin and Jeglum Citation2013). For instance, Sphagnum species play a central ecological role in many northern (>45°N) peatlands; they are adapted to, and also mediate, the characteristic waterlogged and acidic conditions that promote peat accumulation (Hayward and Clymo Citation1982; Van Breemen Citation1995; Verhoeven and Liefveld Citation1997; Turetsky Citation2003). The formation of peat has resulted in a large global carbon store of ca. 600 Pg C (Page et al. Citation2011; Yu Citation2012). This is equivalent to around a third of the total soil carbon, estimated at 1500 Pg in the upper 1 m and 2400 Pg in the upper 2 m (Stockmann et al. Citation2013; Scharlemann et al. Citation2014).
Fire is a key disturbance in many peatlands in northern and boreal ecosystems (Turetsky et al. Citation2004). Wildfire activity is projected to increase due to changes in temperature and rainfall patterns arising from climate change (Krawchuk et al. Citation2009; De Groot et al. Citation2013), possibly leading to more severe disturbances in peatland ecosystems. In some regions such as the British Isles, open peat bogs (sensu Rydin and Jeglum Citation2013), including blanket and raised bogs, are also subjected to managed burning to promote suitable habitat for livestock, game and wildlife and to manage the risk and severity of wildfires (Fernandes and Botelho Citation2003; Harris et al. Citation2011; Allen et al. Citation2016). Managed burning is a controversial practice on peat bogs (Davies et al. Citation2016a,Citationb). This is at least partly due to limited experimental evidence of its ecological consequences and a lack of research on the ability of Sphagnum species to recover following managed burns. We do however have a number of studies which suggest that certain Sphagnum species are resilient to the effects of fire. For instance, previous field research on the North York Moors (northern England) reported greater Sphagnum abundance in plots with a 10-year managed burning rotation compared to plots that had remained unburnt for 47 years (Lee et al. Citation2013), whilst other studies of managed burning have shown greater abundance in more recently burnt areas (3–7 years) compared to areas unburnt for 25 years (Burch Citation2009). In peatlands in Alberta (Canada), Sphagnum abundance was highest at medium time spans post-wildfire (20–80 years) compared to shorter (10 years) or longer time spans (>80 years) (Benscoter and Vitt Citation2008). Taken together, these studies suggest that whilst burning may temporarily set back Sphagnum populations, changes to environmental conditions post-fire, such as increased substrate and light availability, can favour Sphagnum regeneration (Heijmans et al. Citation2002). Unfortunately, the direct impacts of high temperatures during managed burning on Sphagnum are little understood. To our knowledge, only a single study (Taylor et al. Citation2017) has been published and this reported that the degree of recovery of Sphagnum capillifolium (Ehrh.) Hedw (assessed by chlorophyll fluorescence, CO2 exchange and physical damage) was related to the temperature treatment, post-treatment environmental conditions and pre-treatment stem moisture content. The slowest recovery was found when samples were heated to 400°C for 30 s (Taylor et al. Citation2017). Nevertheless, all temperature treatments showed signs of recovery. The study of Taylor et al. was conducted under semi-controlled conditions and thus longer term evidence in the field remains lacking.
There is considerable concern that environmental changes throughout the distribution range of peatlands could increase soil carbon losses leading to increased greenhouse gas emissions to the atmosphere, altered radiative forcing and exacerbated climate change (Heimann and Reichstein Citation2008; Dorrepaal et al. Citation2009). The unique biodiversity of peatlands and their restricted global distribution (ca. 3% of land cover; Yu et al. Citation2010), make them particularly important for global conservation (European Commission Citation1992). As such, and given the key role of peatland ecosystems for global carbon cycling, it is important to understand their responses to major natural and anthropogenic disturbances so they can be effectively conserved and sustainably managed. There is therefore an urgent need for further investigations of the response of Sphagnum to fire to provide an evidence base to ensure that debates about managed burning are informed rather than opined (Davies et al. Citation2016a) and that management practices are sustainable. Moreover, a sound understanding of Sphagnum’s response to variation in fire severity can help evaluate potential impacts of the projected alteration to fire regimes throughout peatlands’ range on globally substantial carbon stores. Here, we aimed to study the effect of prescribed burning on Sphagnum cover and photosynthetic capacity at a raised bog. Our general aim was to quantify Sphagnum recovery following burns at a range of fire severities and for up to 3 years post-fire. Specifically, our objectives were to determine whether
managed burning led to sustained reductions in Sphagnum cover,
loss of Sphagnum photosynthetic capacity arising from thermal shock was recovered and
post-fire Sphagnum recovery was related to fire severity.
Materials and methods
The experiment was carried out at Braehead Moss, a raised bog in southern Scotland (latitude 55.740°N, longitude 3.658°W, elevation 270 m). Declared site of special scientific interest in 1997 (Scottish Natural Heritage Citation2012), Braehead Moss is known to have been subjected to low levels of grazing, peat cutting and some limited drainage. Maximum peat depth is >9 m at the centre of the bog (Scottish Natural Heritage, personal communication; 21 September 2016). Data from Drumalbin weather station (1981–2010; 13 km south of the site) indicate mean annual precipitation to be 900 mm, a mean summer temperature of 13.2°C and a mean winter temperature of 2.8°C (Met Office Citation2012). The experiment was completed in the drier southern part of the bog, where vegetation, dominated by continuous stands of mature Calluna vulgaris (L.) Hull (hereafter Calluna) with Eriophorum vaginatum (L.) also present, reflects past disturbance and altered hydrology. The bryophyte layer is dominated by the pleurocarpous moss Hypnum jutlandicum Holmen & E. Warncke. However, Sphagnum mosses are common, with S. capillifolium being the most abundant species.
We completed nine ca. 20 m × 25 m experimental fires, burnt as headfires between October 2013 and November 2014. We achieved a range of fire severities by lowering the moisture content of 2 m × 2 m plots using rain-out shelters (Yahdjian and Sala Citation2002). The shelters were left in the field for 2–4 months before removing them immediately before the fires. In each fire, we established two treated plots (‘drought’, higher fire severity plots) and two untreated plots (‘no-drought’, lower fire severity plots). Additionally, we delimited two unburnt plots outside each fire. Before burning, we took three surface (top 2 cm) moss and litter samples from random locations in each plot to measure fuel moisture content (FMC). Results of drought-induced changes in vegetation moisture content and subsequent fire severity are described in full in Grau-Andrés et al. (Citation2017). Briefly, the drought treatment significantly decreased pre-fire FMC of the moss and litter layer from 365 ± 248% (mean ± standard deviation) to 112 ± 101% (dry base). Lower FMC significantly increased fire severity as estimated by moss and litter consumption (0.1 ± 0.3 cm in no-drought; 1.4 ± 1.1 cm in drought plots) and soil heating (e.g. total heat at the soil surface was 40 ± 62 °C-min in no-drought and 146 ± 146 °C-min in drought plots).
To measure moss consumption, we assessed changes in moss layer depth using five metal “duff spikes” (Davies et al. Citation2010) per plot. A visual estimation of the cover of individual Sphagnum species (to the nearest 1%, using a 1 m × 1 m quadrat placed centrally in each plot) was estimated on September 2015. Depending on the plot/burn date, plots were sampled at 306–705 (mean = 514) days after the fire had been completed. Depending on availability, 4–15 (mean = 9.4) Sphagnum specimens were randomly sampled from each treatment on four sampling dates: 23 May 2014, 9 June 2014, 8 December 2015 and 10 October 2016. As fires were burnt sequentially over the duration of the experiment, two, four, nine and nine experimental fires at each date, respectively, were available for sampling. The Sphagnum samples were identified to species level and dark-adapted in air-tight containers for 2–4 h before measuring chlorophyll fluorescence parameters of their capitula using a Handy PEA chlorophyll fluorimeter (Hansatech Instruments Ltd.). We used the maximum quantum yield of the photosystem II (Fv/Fm), an indicator of photosynthetic capacity and plant stress (Krause and Weis Citation1991), to assess post-fire recovery. The Fv/Fm ratio is a good indicator of the physiological state of the leaf photosynthetic system (Maxwell and Johnson Citation2000) and has been successfully used in Sphagnum (Hájek and Beckett Citation2008; Manninen et al. Citation2011; Taylor et al. Citation2017).
We used R 3.3.2 for all plotting and statistical analysis (R Core Team Citation2016). The effect of treatment (unburnt, no-drought and drought) on Sphagnum cover was analysed using a linear mixed-effects model with treatment as a fixed effect and fire as a random effect (function “lme” in package nlme; Pinheiro et al. Citation2015). A constant variance function accounted for the heterogeneity of variance associated with the treatment factor. S. capillifolium was the most abundant and widely distributed species and the only one where statistical analysis of photosynthetic capacity (as Fv/Fm) was made. The small sample size of other Sphagnum species meant that comparisons were made graphically. Changes in photosynthetic capacity of S. capillifolium in relation to fire severity treatment and time since fire were investigated using a linear mixed-effects model with an interaction between treatment and sampling date as fixed effects and fire as a random effect. Sampling date was used as a proxy for recovery time as it also integrated information on seasonal and environmental effects that can influence Fv/Fm (Granath et al. Citation2009; Taylor et al. Citation2017), and dependence between time since fire and sampling date meant both variables could not be modelled together. A constant variance function for sampling date was used. Comparisons between treatment levels within each sampling date were analysed using multiple comparisons procedures (function “glht” in package multcomp; Hothorn et al. Citation2008).
Results
Pre-fire surface moisture content of Sphagnum mosses was similar in no-drought plots (709 ± 310%) and drought plots (610 ± 174%). No significant consumption of Sphagnum was observed in either burn treatment. Sphagnum cover, dominated by S. capillifolium (average cover across all treatments was 5.2%, range 0–34%), was similar in unburnt and burnt plots assessed 10–24 months after the fire ().
Figure 1. Sphagnum cover on September 2015, when time since fire ranged 306–705 days (mean = 514) for the different burns. Values are shown for all species identified as well as total Sphagnum cover (‘total’) in each treatment: unburnt, low severity burnt (‘no-drought’) and high severity burnt (‘drought’). Average Sphagnum cover was 6.3% (SD = 8.7%) across all plots. Height of the box is the interquartile range, the horizontal bar is the median, whiskers extend to 1.5 times the first or third quartile, circles are outliers. The same letters above the boxplots of total Sphagnum cover indicate that differences between treatments were not statistically significant (α = 0.05). Statistical testing details are provided in Table S1.
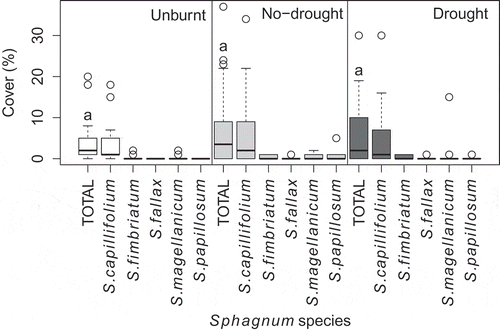
Burning, and particularly higher severity burning, lowered the photosynthetic capacity of S. capillifolium shortly after the fire (). Fv/Fm of S. capillifolium 1–8 months post-fire was significantly higher in unburnt plots (0.65 ± 0.09 in May 2014 and 0.68 ± 0.11 in June 2014) than in no-drought (0.37 ± 0.23 and 0.50 ± 0.16, respectively) and drought plots (0.09 ± 0.08 and 0.38 ± 0.17, respectively).
Figure 2. Boxplots of the chlorophyll fluorescence Fv/Fm ratio of S. capillifolium capitula, for the different plot treatments and sampling dates. Width of the box is proportional to the number of observations, provided below each boxplot. Different letters above boxplots within sampling date indicate significant differences (α = 0.05). Generally, wet weather before sampling meant Sphagnum was probably not stressed due to desiccation. See Supplementary material for statistical testing details (Tables S2 and S3), weather information (Table S4) and Fv/Fm vs. time since fire for individual samples (Figure S1).
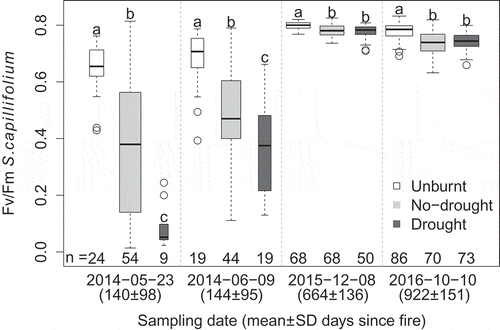
Post-fire photosynthetic capacity in S. capillifolium had recovered to healthy levels (Fv/Fm > 0.75), after less than 2 years. Although Fv/Fm in burnt plots remained significantly lower than in unburnt plots during the last two sampling dates, ca. 22 and 31 months after the fires, differences were small: unburnt plots averaged 0.79 ± 0.02 and burnt 0.76 ± 0.03. In contrast to the two earlier sampling dates, differences in photosynthetic capacity between higher and lower severity burnt plots were not statistically significant in the last two sampling dates. Graphical analysis of Fv/Fm in Sphagnum species belonging to section Sphagnum showed similar patterns to that of S. capillifolium, with low values in burnt plots ca. 7 months after fire and small differences between treatments ca. 20 months post-fire ().
Figure 3. Boxplots of the chlorophyll fluorescence Fv/Fm ratio of S. magellanicum (n = 23), S. papillosum (n = 13) and S. palustre (n = 8) capitula, for the different plot treatments and sampling dates. Width of the box is proportional to the number of observations, provided below each boxplot. See Figure S2 for Fv/Fm against days since fire of individual samples.
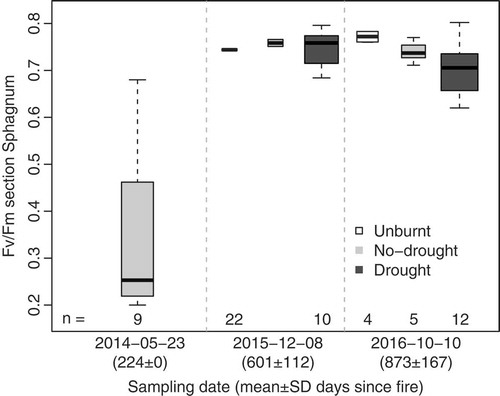
Discussion
Burning did not lead to sustained reductions in short-term (10–24 months post-fire) Sphagnum cover (). This was likely due to the negligible Sphagnum combustion and lack of extensive heating below the Sphagnum surface (Grau-Andrés et al. CitationIn Press). This resilience to burning and heating is driven by the large water-holding capacity of Sphagnum (Hayward and Clymo Citation1982). Pre-fire surface FMC was >600%, above the moisture content threshold for sustained combustion of the bryophyte layer in moorlands, estimated at ca. 70% (Davies and Legg Citation2011; Santana and Marrs Citation2014). Although differences in Sphagnum cover between burnt and unburnt plots were not significant, the slight but consistently higher cover values following burning suggest that Sphagnum abundance could increase in the longer term as a result of the removal of the Calluna canopy and increases in competitive ability relative to pleurocarpous mosses (Benscoter and Vitt Citation2008; Lee et al. Citation2013). This hypothesis is supported by studies on a blanket bog in northern England which have previously found higher Sphagnum abundance at smaller times since fire (Burch Citation2009), and at short (10-year) vs. longer (20-year) burning rotations or unburnt (47 years) conditions (Lee et al. Citation2013).
Fire-induced heating greatly reduced S. capillifolium photosynthetic capacity as measured at ca. 5 months after burning (). While the higher fire severity measured in drought plots did not cause increased Sphagnum consumption, fire-induced heating at the soil surface, and presumably at the moss surface too, was greater (Grau-Andrés et al. CitationIn Press). The lower photosynthetic capacity in drought plots may have resulted from the higher magnitude of the fire-induced thermal shock causing greater damage to chlorophyllous cells (e.g. via protein denaturation, lipid mobility) and possibly to hyaline cells thus lowering water retention capacity (Levitt Citation1980; Rydin and Jeglum Citation2013). Previous work in laboratory conditions showed similar patterns in S. capillifolium Fv/Fm response to temperatures consistent with managed fires: a substantial decrease from ca. 0.59 to ca. 0.1 shortly after burning and rapid subsequent recovery to ca. 0.37 after 100 days (Taylor et al. Citation2017). Taylor et al. (Citation2017) also reported slower recovery in the capacity of Sphagnum to retain moisture in samples subjected to higher temperatures, an important control on photosynthetic capacity (Van Gaalen et al. Citation2007). Recovery of structural and photosynthetic tissues could thus occur at different rates and stages; further work on physiological responses to fire in Sphagnum may clarify mechanisms and rates of recovery. Other disturbances such as desiccation, nitrate deposition and salinity have been found to lead to Fv/Fm decreases of similar magnitude in Sphagnum species (0.75–0.85 to 0.05–0.5; Hájek and Beckett Citation2008; Bates et al. Citation2009; Manninen et al. Citation2011).
Approximately 2 years following fire, S. capillifolium Fv/Fm in burnt plots was lower than in unburnt but within the range of healthy plants, considered to be around 0.75 (range: 0.55–0.85) in bryophytes (Van Gaalen et al. Citation2007; Hájek and Beckett Citation2008; Bates et al. Citation2009; Manninen et al. Citation2011; Taylor et al. Citation2017) and so differences in physiological functioning were likely negligible. Given the relatively rapid recovery of S. capillifolium Fv/Fm following fire-induced heating, at least during the first 100 days (Taylor et al. Citation2017), and its resilience to other disturbances such as drought (Hájek and Vicherová Citation2014) and high exposure to light (Maraschall and Proctor Citation2004), we hypothesise that sustained differences between burnt and unburnt plants are a result of microclimatic changes brought about by fire. These may have included increased water stress due to increased evaporative demand following the removal of the Calluna canopy and increased solar irradiation (Hájek et al. Citation2009; Laing et al. Citation2014; Leonard et al. Citation2017). The higher variability in photosynthetic capacity in the burnt plots in the immediate post-fire period is likely to be due to the substantial heterogeneity in fire severity at small spatial scales (Fernandes et al. Citation2000; Bova and Dickinson Citation2008). Despite having little data for Sphagnum species belonging to section Sphagnum, the low photosynthetic capacity in burnt plots ca. 7 months after fire, but values comparable to unburnt plots ca. 20 months later, suggest response to burning was similar to that seen for S. capillifolium. The Sphagnum species examined here from section Sphagna, particularly the dominant species S. magellanicum, tend to grow closer to the water table than S. capillifolium, a hummock species, which may have important implications for controlling disturbance severity (Benscoter and Wieder Citation2003) and for recovery of photosynthesis after disturbance (Hájek and Beckett Citation2008; Hájek and Vicherová Citation2014; Bengtsson et al. Citation2016). Therefore, the next step would be to research the variation in responses to fire along gradients of severity, examining different functional traits and microhabitats of Sphagnum species.
Management implications
Our results show a limited impact of managed burning on S. capillifolium abundance and functioning, particularly when pre-fire moisture content of the ground fuels is high and thus fire severity is low. Managed burning has potential benefits for maintaining Sphagnum abundance by improving light conditions through removal of dense Calluna canopies (although the associated greater evaporative loss may be detrimental) and reducing both the abundance and competitive advantage of pleurocaropous mosses (Heijmans et al. Citation2002; Benscoter and Vitt Citation2008; Lee et al. Citation2013). In bogs with high Sphagnum cover, low severity fires, where surface moisture content (top 2 cm) of the moss and litter layer is >200–300% (Grau-Andrés et al. CitationIn Press), could help maintain high Sphagnum abundance. At low fire severities, consumption of the bryophyte layer is minimal, and Calluna regeneration, especially in old stands where resprouting capacity is low (Hobbs and Gimingham Citation1984), may be slow given the limited establishment of Calluna seedlings on moss substrates (Davies et al. Citation2010). Furthermore, burning in spring rather than in autumn could result in faster post-fire Sphagnum recovery due to warmer temperatures and improved light quality (Taylor et al. Citation2017). In degraded, drying bogs where Sphagnum is displaced by pleurocarpous mosses, higher severity fires could be trialled to increase consumption of pleurocarpous mosses while impact on Sphagnum hummocks remains low due to their high water-holding capacity, thus facilitating Sphagnum expansion. Such conditions can be achieved by burning when the surface moisture content of the pleurocarpous moss layer is <150% (Grau-Andrés et al. CitationIn Press).
Conclusions
There has been significant controversy over the use of managed burning on peatlands (Davies et al. Citation2016a) due to perceived negative impacts on ecological functioning, particularly with regard to Sphagnum. Our results contradict this perception and show a limited and short-lived effect of managed fire on S. capillifolium abundance and photosynthetic capacity. Moreover, our limited data on S. magellanicum, S. papillosum and S. palustre indicate that other Sphagnum species can also achieve rapid post-fire recovery. These findings, together with previous work on British and boreal peatlands, show that some Sphagnum species are resilient to disturbance by fire, particularly at low–moderate fire severities. Our results suggest that carefully applied managed burning is not at odds with the restoration and conservation of peatland ecosystem function. This does not preclude the fact that some Sphagnum species may be sensitive to fire and that regular managed burning could drive changes in composition.
TPED_A_1394394_Supplementary_material_RGA_edit.docx
Download MS Word (83.7 KB)Acknowledgements
Roger Grau-Andrés was funded by the Lord Kelvin and Adam Smith scholarship (University of Glasgow). E. Marian Scott advised on statistical analysis. Susan Waldron provided logistical support. Scottish Natural Heritage provided site access and support. Laszlo Nagy gave useful advice on the manuscript.
Disclosure statement
No potential conflict of interest was reported by the authors.
Supplemental data
Supplemental data for this article can be accessed here.
Additional information
Funding
Notes on contributors
Roger Grau-Andrés
Roger Grau-Andrés is an environmental scientist with a background in fire behaviour and peatland ecology research.
Alan Gray
Alan Gray is a plant ecologist researching peatland and island ecosystems.
G. Matt Davies
G. Matt Davies is an ecologist with interests in wildland fire management and plant community restoration.
References
- Allen KA, Denelle P, Sánchez-Ruiz FM, Santana VM, Marrs RH. 2016. Prescribed moorland burning meets good practice guidelines: a monitoring case study using aerial photography in the Peak District, UK. Ecological Indicators 62:76–85.
- Bates JW, Wibbelmann MH, Proctor MCF. 2009. Salinity responses of halophytic and non-halophytic bryophytes determined by chlorophyll fluorometry. Journal of Bryology 31:11–19.
- Bengtsson F, Granath G, Rydin H. 2016. Photosynthesis, growth, and decay traits in Sphagnum –a multispecies comparison. Ecology and Evolution 6:3325–3341.
- Benscoter BW, Vitt DH. 2008. Spatial patterns and temporal trajectories of the bog ground layer along a post-fire chronosequence. Ecosystems 11:1054–1064.
- Benscoter BW, Wieder RK. 2003. Variability in organic matter lost by combustion in a boreal bog during the 2001 Chisholm fire. Canadian Journal of Forest Research 33:2509–2513.
- Bova AS, Dickinson MB. 2008. Beyond ‘fire temperatures’: calibrating thermocouple probes and modeling their response to surface fires in hardwood fuels. Canadian Journal of Forest Research 38:1008–1020.
- Burch J. 2009. The regeneration of bryophytes after the burning of dry heath and wet heath moorland on the North York Moors.Sheffield: Natural England.
- Davies GM, Kettridge N, Stoof CR, Gray A, Ascoli D, Fernandes PM, Marrs R, Allen KA, Doerr SH, Clay GD, et al. 2016a. The role of fire in UK peatland and moorland management: the need for informed, unbiased debate. Philosophical Transactions of the Royal Society of London B: Biological Sciences 371.
- Davies GM, Kettridge N, Stoof CR, Gray A, Marrs R, Ascoli D, Fernandes PM, Allen KA, Doerr SH, Clay GD, et al. 2016b. Informed debate on the use of fire for peatland management means acknowledging the complexity of socio-ecological systems. Nature Conservation 16:59–77.
- Davies GM, Legg CJ. 2011. Fuel moisture thresholds in the flammability of Calluna vulgaris. Fire Technology 47:421–436.
- Davies GM, Smith AA, MacDonald AJ, Bakker JD, Legg CJ. 2010. Fire intensity, fire severity and ecosystem response in heathlands: factors affecting the regeneration of Calluna vulgaris. Journal of Applied Ecology 47:356–365.
- De Groot WJ, Flannigan MD, Cantin AS. 2013. Climate change impacts on future boreal fire regimes. Forest Ecology and Management. 294:35–44.
- Dorrepaal E, Toet S, Van Logtestijn RSP, Swart E, Van De Weg MJ, Callaghan TV, Aerts R. 2009. Carbon respiration from subsurface peat accelerated by climate warming in the subarctic. Nature 460:616–619.
- European Commission. 1992. Council Directive 92/43/EEC of 21 May 1992 on the conservation of natural habitats and of wild fauna and flora. AQ30:Official Journal of the European Communities. Series L 206: 7-49.
- Fernandes PM, Botelho HS. 2003. A review of prescribed burning effectiveness in fire hazard reduction. International Journal of Wildland Fire 12:117–128.
- Fernandes PM, Catchpole WR, Rego FC. 2000. Shrubland fire behaviour modelling with microplot data. Canadian Journal of Forest Research 30:889–899.
- Granath G, Strengbom J, Breeuwer A, Heijmans MMPD, Berendse F, Rydin H. 2009. Photosynthetic performance in Sphagnum transplanted along a latitudinal nitrogen deposition gradient. Oecologia 159:705–715.
- Grau-Andrés R, Davies GM, Gray A, Scott EM, Waldron S. 2017. Fire severity is more sensitive to low fuel moisture content on Calluna heathlands than on peat bogs. Science of the Total Environment. http://www.sciencedirect.com/science/article/pii/S0048969717328991 .
- Hájek T, Beckett RP. 2008. Effect of water content components on desiccation and recovery in Sphagnum Mosses. Annals of Botany 101:165–173.
- Hájek T, Tuittila E-S, Ilomets M, Laiho R. 2009. Light responses of mire mosses -a key to survival after water-level drawdown? Oikos 118:240–250.
- Hájek T, Vicherová E. 2014. Desiccation tolerance of Sphagnum revisited: a puzzle resolved. Plant Biology 16:765–773.
- Harris MPK, Allen KA, McAllister HA, Eyre G, Le Duc MG, Marrs RH. 2011. Factors affecting moorland plant communities and component species in relation to prescribed burning. Journal of Applied Ecology 48:1411–1421.
- Hayward PM, Clymo RS. 1982. Profiles of water content and pore size in sphagnum and peat, and their relation to peat bog ecology. Proceedings of the Royal Society of London B: Biological Sciences 215:299–325.
- Heijmans MMPD, Klees H, Berendse F. 2002. Competition between Sphagnum magellanicum and Eriophorum angustifolium as affected by raised CO2 and increased N deposition. Oikos 97:415–425.
- Heimann M, Reichstein M. 2008. Terrestrial ecosystem carbon dynamics and climate feedbacks. Nature 451:289–292.
- Hobbs RJ, Gimingham CH. 1984. Studies on fire in Scottish heathland communities II. Post-fire vegetation development. Journal of Ecology 72:585–610.
- Hothorn T, Bretz F, Westfall P. 2008. Simultaneous inference in general parametric models. Biometrical Journal 50:346–363.
- Joosten H, Clarke D. 2002. Wise use of mires and peatlands. International Mire Conservation Group and International Peat Society, Saarijärvi, Finland.
- Krause GH, Weis E. 1991. Chlorophyll fluorescence and photosynthesis: the basics. Annual Review of Plant Physiology and Plant Molecular Biology 42:313–349.
- Krawchuk MA, Moritz MA, Parisien M-A, Van Dorn J, Hayhoe K. 2009. Global pyrogeography: the current and future distribution of wildfire. PLoS ONE 4:1–12.
- Laing CG, Granath G, Belyea LR, Allton KE, Rydin H. 2014. Tradeoffs and scaling of functional traits in Sphagnum as drivers of carbon cycling in peatlands. Oikos 123:817–828.
- Lee H, Alday JG, Rose RJ, O’Reilly J, Marrs RH. 2013. Long-term effects of rotational prescribed burning and low-intensity sheep grazing on blanket-bog plant communities. Journal of Applied Ecology 50:625–635.
- Leonard R, Kettridge N, Krause S, Devito KJ, Granath G, Petrone R, Mendoza C, Waddington JM. 2017. Peatland bryophyte responses to increased light from black spruce removal. Ecohydrology 10: e1804.
- Levitt J. 1980. Responses of plants to environmental stress. In: Chilling, freezing, and high temperature stresses. 2nd ed. Vol. 1. New York, NY: Academic Press.
- Manninen S, Woods C, Leith ID, Sheppard LJ. 2011. Physiological and morphological effects of long-term ammonium or nitrate deposition on the green and red (shade and open grown) Sphagnum capillifolium. Environmental and Experimental Botany 72:140–148.
- Maraschall M, Proctor MCF. 2004. Are bryophytes shade plants? Photosynthetic light responses and proportions of chlorophyll A, chlorophyll B and total carotenoids. Annals of Botany 94:593–593.
- Maxwell K, Johnson GN. 2000. Chlorophyll fluorescence -a practical guide. Journal of Experimental Botany 51:659–668.
- Met Office. 2012. Met Office Integrated Data Archive System (MIDAS) land and marine surface stations data (1853-current). Harwell Oxford: NCAS British Atmospheric Data Centre, 2016-03-25.
- Millennium Ecosystem Assessment. 2005. Ecosystems and human well-being: synthesis. Washington, DC: Island Press.
- Page SE, Rieley JO, Banks CJ. 2011. Global and regional importance of the tropical peatland carbon pool. Global Change Biology 17:798–818.
- Pinheiro J, Bates D, DebRoy S, Sarkar D, R Core Team. 2015. nlme: linear and nonlinear mixed effects models.
- R Core Team. 2016. R: A language and environment for statistical computing.Vienna: R Foundation for Statistical Computing.
- Rydin H, Jeglum JK. 2013. The biology of peatlands. New york, NY: Oxford University Press.
- Santana VM, Marrs RH. 2014. Flammability properties of British heathland and moorland vegetation: models for predicting fire ignition. Journal of Environmental Management 139:88–96.
- Scharlemann JPW, Tanner EVJ, Hiederer R, Kapos V. 2014. Global soil carbon: understanding and managing the largest terrestrial carbon pool. Carbon Management 5:81–91.
- Scottish Natural Heritage. 2012. Braehead moss site management statement. Inverness: Scottish Natural Heritage.
- Stockmann U, Adams MA, Crawford JW, Field DJ, Henakaarchchi N, Jenkins M, Minasny B, McBratney AB, de Remy de Courcelles V, Singh K, et al. 2013. The knowns, known unknowns and unknowns of sequestration of soil organic carbon. Agriculture, Ecosystems and Environment 164:80–99.
- Taylor ES, Levy PE, Gray A. 2017. The recovery of Sphagnum capillifolium following exposure to temperatures of simulated moorland fires: a glasshouse experiment. Plant Ecology and Diversity 10:77–88.
- Turetsky MR. 2003. The role of bryophytes in carbon and nitrogen cycling. The Bryologist 106:395–409.
- Turetsky MR, Amiro BD, Bosch E, Bhatti JS. 2004. Historical burn area in western Canadian peatlands and its relationship to fire weather indices. Global Biogeochemical Cycles 18: GB4014.
- Van Breemen N. 1995. How Sphagnum bogs down other plants. Trends in Ecology and Evolution 10:270–275.
- Van Gaalen KE, Flanagan LB, Peddle DR. 2007. Photosynthesis, chlorophyll fluorescence and spectral reflectance in Sphagnum moss at varying water contents. Oecologia 153:19–28.
- Verhoeven JTA, Liefveld WM. 1997. The ecological significance of organochemical compounds in Sphagnum. Acta Botanica Neerlandica 46:117–130.
- Yahdjian L, Sala OE. 2002. A rainout shelter design for intercepting different amounts of rainfall. Oecologia 133:95–101.
- Yu Z, Loisel J, Brosseau DP, Beilman DW, Hunt SJ. 2010. Global peatland dynamics since the Last Glacial Maximum. Geophysical Research Letters 37: L13402.
- Yu ZC. 2012. Northern peatland carbon stocks and dynamics: a review. Biogeosciences 9:4071–4085.