ABSTRACT
Background: The effects of trees on understorey communities is a major driver of vegetation composition. However, we have little understanding on how isolated forest patches of the forest-steppe transition affect their herb layer as compared to adjacent grasslands.
Aims: Our aim was to test whether trees had a protective effect on understorey herbaceous communities in the most arid regions of the forest-steppe transition, where the stress gradient hypothesis predicts positive net effects.
Methods: We surveyed herbaceous cover and species composition in 135 forest-steppe sites and recorded soil moisture, microclimate and canopy cover in northern Kazakhstan.
Results: Total cover and species richness were lower in the herb layer of groves than in the steppe stands and the soil of the groves was not moister than that of the steppes. Groves were dominated by grassland specialist plants, while forest specialist species remained scarce. However, these grassland species were different from the ones inhabiting the steppes, leading to little nestedness between groves and steppes.
Conclusions: Although the presence of groves greatly increase the landscape level diversity of Middle-Asian forest-steppes, trees in the southern edge of the zone seem to have net negative effect on the understorey vegetation, seemingly contradicting the stress gradient hypothesis.
Introduction
Mosaic habitats consisting of grassland and forest components often harbour high biodiversity (Scholes and Archer Citation1997; Manning et al. Citation2006). Their dynamics and species composition largely depend on the interactions between the trees and their herb layer (Staal and Flores Citation2015). Tree-herb interactions occur over a wide array of spatial scales (Laurence et al. Citation2001; Cadenasso et al. Citation2003) from infra-patch scales (e.g. between solitary trees of a wood-pasture and the surrounding herbaceous plants; López-Sanchez et al. Citation2016) to biome-wide regions (e.g. along the boreal forest-tundra transition; D’Odorico et al. Citation2013).
The occurrence of trees at the global scale is related to macroclimatic conditions (precipitation and temperature). D’Odorico et al. (Citation2013) have emphasised the importance of temperature in determining the position of treelines at high elevations and latitudes as trees are mostly more sensitive to cold stress than their herbaceous counterparts. At forest-grassland transitions where low temperature is not limiting, climatic water deficit constrains tree recruitment and survival (Chhin and Wang Citation2002; Dulamsuren et al. Citation2005). Examples include the transitions between tropical forests and grasslands (e.g. Vetaas Citation1992) and between temperate forests and steppes (e.g. Magyari et al. Citation2010). In all these cases, trees do not occur beyond the transitional zones because external conditions are beyond their limit of tolerance. Conversely, grassland communities would be able to thrive on the forest-ward side of the treelines without any constraining effect of macroclimatic filters, but their composition is altered by biotic constraints (i.e. the presence and shading effect of closed canopy forming trees). This deterministic relationship is affected to some extent by local to regional disturbance factors such as fire or herbivory, frequently making forested and herbaceous patches alternative stable states within the transitional zones (Staver et al. Citation2011; Hoffmann et al. Citation2012). Nevertheless, the resulting biodiversity patterns in a temporal snapshot are determined by the presence or absence of trees, owing to their ecosystem engineering effect.
Trees can modify the physical properties of their environment (e.g. light availability, microclimate, nutrient content, soil moisture) and, through this, change the conditions for plant assemblages in the herb layer as compared with adjacent treeless grasslands (Manning et al. Citation2006; Tinya et al. Citation2009). The extent and even the direction of the effects can differ according to tree species, tree stand density and climatic conditions, highlighting the context dependence of the net effects of trees in forest-grassland transitions (Scholes and Archer Citation1997; Armas et al. Citation2011). A voluminous literature is available regarding such effects in tropical (e.g. Scholes and Archer Citation1997; Dohn et al. Citation2013) and Mediterranean (e.g. Gea-Izquierdo et al. Citation2009; Fernández-Moya et al. Citation2011; Rivest et al. Citation2011) forest-grassland transitions while information on tree-herb interactions in the continental forest-steppe biome is scarce (but see, e.g., Erdős et al. Citation2014).
The forest-steppe biome, i.e. the transition between temperate forests and continental dry grasslands, stretches across Eurasia from central Europe to eastern Siberia, covering a total of 2.9 M km2 (Wesche et al. Citation2016; Erdős et al. Citation2018). Smaller forest-steppe transition zones with similar physiognomy can also be found along the boundaries of North American prairies (Williams et al. Citation2009) and South American pampas (Mancini Citation2009). While trees in most tropical and subtropical forest-grassland transitions form an intermediate, sparsely wooded transition, the savanna (Hirota et al. Citation2011; Staver et al. Citation2011), the forest-steppe transition is mostly a macro-mosaic of compact forest and grassland patches (Walter and Breckle Citation1989; Erdős et al. Citation2014, Citation2015a). This difference in physiognomy along with the differences in climatic regimes indicates that theories developed for forest-savanna transitions may not be directly transferable to the forest-steppe biome.
Understanding how tree-herb interactions drive biodiversity patterns in forest-steppes is an especially urgent task for two main reasons. First, forest-steppe transitions concentrate the biota of both the adjacent steppes and temperate forests, leading to inherently high landscape-scale biodiversity (Fekete et al. Citation2010; Habel et al. Citation2013). Second, both biomes are among the most threatened ones in the world, as they provide favourable climatic and soil conditions for agriculture (Deák et al. Citation2016). Large areas have been turned into croplands as a result of large-scale deforestation (Molnár et al. Citation2012; Kämpf et al. Citation2016). In addition, exotic tree plantations (Biró et al. Citation2008) and invasive species (Kelemen et al. Citation2016) have also been affecting biodiversity. Due to the long history of humans in these areas, it is frequently debated whether landscape patterns are ancient features or results of human activities (Dulamsuren et al. Citation2005; Fekete et al. Citation2010; Feurdean et al. Citation2015), making the definition of appropriate target states for conservation difficult (Novenko et al. Citation2016). In addition, the forest-steppe transition is heavily affected by climate change (Erdős et al. Citation2018). Most scenarios forecast a warming climate, a decrease in precipitation and an increase in the frequency of droughts, favouring the expansion of steppe and reduction of forest, eventually leading to the northward displacement of the transitional zone (Tchebakova et al. Citation2009; Kamp et al. Citation2016). As part of this process, it may be expected that tree-herb interactions will also change, leading to profound changes in the overall structure and species composition of the vegetation as simulated by Daly et al. (Citation2000). Given the forecast changes and uncertainties shown above (i.e. which are natural properties of the biome and which are related to anthropogenic effects), the principles of tree-herb interactions in the forest-steppe biome should be clarified to avoid misinterpreting patterns and processes or creating artefacts during conservation activities.
We aimed to contribute to filling this gap of knowledge by investigating tree-herb interactions in the southern edge of Middle Asian forest steppes, where woody vegetation first appears along the aridity gradient. These forest-steppes are also among the most pristine stands of this vegetation type and hence are expected to exhibit unbiased natural patterns and mechanisms. Specifically, we addressed the following questions: (1) Competition or facilitation - is the net effect of trees on the productivity and diversity of the herb layer negative or positive relative to the adjacent grasslands and what ecosystem engineering mechanisms of the trees may contribute to this effect? (2) Community assembly - do forest specialist species comprise the herb layer of the groves or it contains only a filtered subset of the species pool of the surrounding grassland matrix?
Based on the stress gradient hypothesis, which postulates that the net effect between coexisting partners can change from competitive to facilitative at higher stress levels (Bertness and Callaway Citation1994; Blaser et al. Citation2013), we hypothesised that the net effect of trees at the southern edge of the forest-steppe transition should be positive, meaning that the overstorey should create sheltered microenvironments for herbaceous plants, while the plants of open grasslands would not benefit from this effect. Furthermore, we expected that the sheltered micro-environments under the canopy of trees would be suitable for forest specialists; therefore, they would significantly contribute to the formation of the herb layer of forested patches.
Materials and methods
Study areas
We carried out our study in extrazonal forest-steppes embedded in the northern edge of the steppe zone of Kazakhstan, i.e. in the southernmost patches of forest-steppes in the region. There were two main types of these forest-steppes: one on pure sand and one on loamy soil (). The sandy study sites were located in the Naurzum Nature Reserve (N51°27ʹ–51°34ʹ E64°08ʹ–64°29ʹ), 200 km south of Kostanay city, while the loamy sites were 20–30 km east of Kostanay in a non-protected area (N53°07ʹ–53°10ʹ E63°58ʹ–64°04ʹ). The climate was strongly continental in both areas. According to the WorldClim database (Hijmans et al. Citation2005), annual precipitation is 261 mm in the sandy area and 324 mm in the loamy area. Mean annual temperature is 5.0°C in the sandy area and 3.0°C in the loamy one (January: −14.3°C and −16.3°C; July: 23.3°C and 21.1°C, respectively).
Figure 1. Location of the sandy (open circle) and loamy (filled circle) forest-steppe sites studied in northern Kazakhstan (A). The studied habitat types included sandy pine and birch groves embedded in sandy steppes (B-C) and a loamy birch grove surrounded by meadow-steppes (D).
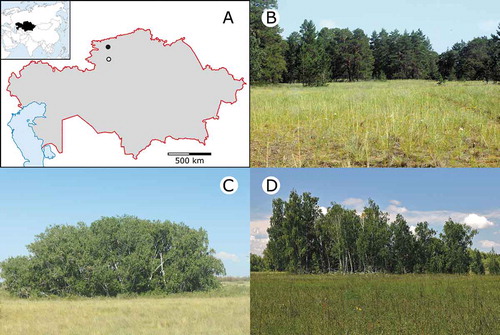
The soil of the sandy area is coarse-grained, calcareous sand with low organic matter content and poor water-holding capacity (Bragina Citation2004). The surface is slightly undulating with an average difference of 2–3 m between dune tops and dune slacks. Groundwater is 2–3 m below the surface of the dune slacks, enabling the occurrence of birch-aspen groves with Betula pendula and Populus tremula as the dominant tree species (hereafter sandy birch groves). In slightly higher elevations, there is a random-looking mosaic of pine groves and xeric grassland patches (hereafter sandy pine groves and sandy steppes, respectively). Sandy pine groves are mono-dominant stands of Pinus sylvestris with sparse undergrowth. Sandy grasslands are dominated by psammophytic bunch-grasses (Stipa pennata ssp. sabulosa and Festuca beckeri). Since Naurzum is a remote, strictly protected area, no grazing or any other type of land use occurs (Bragina et al. Citation2007).
The soil of the loamy forest-steppe is dark chestnut and chestnut soil. Micro-topographic differences are negligible; land cover is characterised by compact stands of B. pendula (hereafter loamy birch groves) embedded in a matrix of grasslands (hereafter meadow-steppes). Meadow-steppes are tall grasslands with a high proportion of dicots. Anthropogenic disturbance is low in this region as well, although there were signs of coppicing in some loamy birch groves. There is no grazing in the meadow-steppes; some patches are mown but probably not every year. Only patches with no apparent signs of recent land use were used for the study.
Data collection and analysis
We selected three localities spaced 2–3 km apart in both study areas. In the sandy forest-steppe, we chose nine sandy birch groves, nine sandy pine groves and nine sandy steppe patches for vegetation surveys in each locality. In the loamy forest-steppes, there were only two habitat types, loamy birch groves and meadow-steppes, of which we also selected nine replicates in each locality, making a total of 135 sampling sites (5 habitat types × 3 localities × 9 habitat replicates). Sampling sites within localities were spaced 50–500 m apart, in a random pattern. In July 2016, we recorded all vascular plant species in one 25-m2 plot in the herb layer of every sampling site and estimated their cover in percent. Trees and shrubs up to a height of 50 cm were included in the herb layer.
The total cover and species richness of the herb layer were compared across habitat types using linear mixed-effects models (LMMs) with locality as the random factor. To better approximate biomass, we used the summed percentage cover scores of individual species instead of using real cover values of the herb layer. Thus, in some cases, the total vegetation cover exceeded 100%. We used Gaussian error term for the vegetation cover and Poisson term for species richness. The compositional distinctness of the habitat types was assessed with principal coordinate analysis (PCoA) using Bray–Curtis dissimilarity on square-root transformed percentage cover scores. Since the separation between sandy birch and sandy pine groves was not complete, we also carried out a permutational multivariate analysis of variance (MANOVA) with 1000 permutations to test their compositional distinctness.
Compositional pattern was further analysed by partitioning dissimilarities into components resulting from nestedness and turnover. Nestedness is high if the composition of a habitat type is a subset of the other one, while the turnover component reflects species replacement rather than species loss between the compared habitat types (Baselga Citation2010). The nine replicates of each habitat type in each locality were averaged for this analysis, leading to three large datasets for every habitat type. We calculated Jaccard dissimilarity index between the habitat types, resulting in nine pair-wise dissimilarities for each habitat pair. These were then partitioned and the nestedness and turnover components were compared.
Finally, we sorted the species into four main groups according to habitat preference: species of psammophytic grasslands (including species of sandy semi-deserts), steppes (non-psammophytic dry grassland species and meadow-steppe species), moist grasslands and forests (including species of shrublands and forest edges), following the classifications of Komarov (Citation1968-2002) and Brinkert et al. (Citation2016) (Table S1). The cover values of species with a more generalist character (e.g. those occurring frequently in more than one habitat type according to the literature) were equally divided among the relevant groups. The cumulative absolute cover of each species group was square-root transformed and compared within habitat types using LMMs with Gaussian error term and quadrat as the random factor. Models were built only for the three grove types and only the comparisons with the cover of forest species were considered.
We measured soil moisture in three of the sampling sites of each habitat type in each locality, making a total of 45 sampling sites. We measured the volumetric moisture content of the upper 20 cm of the soil in five random locations in each sampling site using a FieldScout TDR 300 soil moisture metre. Thus, in total, we had 225 soil moisture data values (5 habitat types × 3 localities × 3 habitat replicates × 5 measurements per replicate). Measurements were made in July 2016 in late morning hours, with no rainfall in the preceding five days to confound typical soil-moisture heterogeneity. We averaged the five data values of each sampling site and the resulting moisture records were analysed with LMMs.
We also measured the microclimate of the five habitat types in two replicates (10 sampling sites in total) for a 24-hour period. Measurements were made with Voltcraft DL-121TH data loggers, capable of recording air temperature and humidity. Data loggers were installed 5 cm above ground level and white plastic plates were placed 10 cm above them to protect them from direct sunlight. Data were recorded once a minute and then were averaged for 1-hour periods. Data of the two replicates of each habitat type were averaged, leading to one dataset per habitat type. The datasets were divided into daytime (7 a.m.–7 p.m.) and night-time ones (7 p.m.–7 a.m.) and were compared across habitat types within each forest-steppe type with paired t-tests.
We used tree canopy cover of the three woody habitat types as a proxy for light availability in the herb layer. We took digital photographs of the canopy in the centre of 17 sandy birch groves, 17 sandy pine groves and 24 loamy birch groves. The spatial arrangement of these sampling sites was independent of the other measurements and had no nested design. The camera was positioned 50 cm above ground level, so the shading effect of all woody species (trees and tall shrubs) that were excluded from the vegetation data of the plots was taken into consideration. Photographs were converted to black and white with manual thresholding in GIMP 2.8 software and canopy cover was defined as the percentage of black pixels in the images. These canopy cover data were compared across grove types with analysis of variance (ANOVA).
All statistical analyses were made in the R environment. For building the LMMs, we used the ‘lme’ (for vegetation cover, functional groups and soil moisture) and ‘glmer’ (for species richness) functions of the nlme and lme4 packages, respectively. The significance of the models was tested with the 'anova' (stat package, ‘lme’ models) and the 'Anova' (car package, ‘glmer’ model) functions. Pair-wise comparisons of factor levels were enabled by the ‘relevel’ function and the false discovery rate (FDR) method was applied to correct P-values. Pair-wise comparisons across sandy and loamy habitats were mostly ignored because the effect of substrate might be confounded by the climatic differences. We used the ‘cmdscale’ and ‘adonis’ functions of the vegan package for the PCoA and the permutational MANOVA, respectively. Dissimilarity partitioning was made with the ‘beta.pair’ function of the betapart package.
Nomenclature of plant species follows The Plant List (www.theplantlist.org; accessed in 2017).
Results
On sandy soil, birch groves had the highest total cover in the herb layer; steppes had significantly lower values and pine groves the lowest. In contrast, on loamy soil, meadow-steppes had higher herb layer cover than birch groves (, ). The species richness of sandy steppes and sandy birch groves were similar and both had higher values than those in the pine groves (, ). Species richness was higher in the meadow-steppes (often exceeding 30 species/25 m2) than in the loamy birch groves.
Table 1. Pair-wise results of the linear mixed-effects models developed for the herb layer cover, species richness and the soil moisture of the studied forest-steppe habitats (*, P < 0.05)..
Figure 2. Total vegetation cover values of the herb layer of the five forest-steppe habitat types (A) and their species richness (B), soil moisture content in the upper 20 cm of the soil (C) and the canopy cover of the woody habitat types (D). Different lowercase letters indicate significant differences (P < 0.05). SS: sandy steppe, SP: sandy pine grove, SB: sandy birch grove, MS: meadow-steppe, LB: loamy birch grove. Solitary dots are outliers.
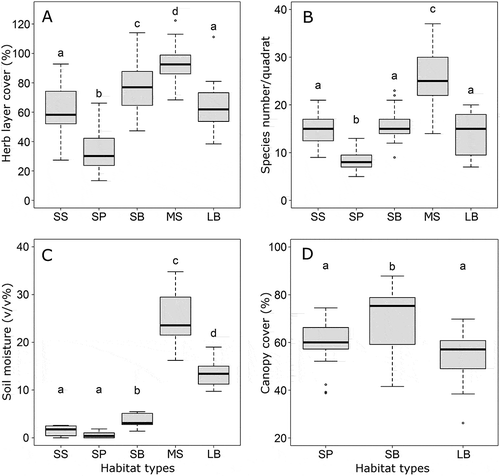
Sandy steppes, meadow-steppes and loamy birch groves occupied distinct regions in the ordination space with no overlap with any other habitat type according to the PCoA (). Sandy pine groves and sandy birch groves showed some overlapping but the permutational MANOVA confirmed significant difference between their composition (F = 11.93, R2 = 0.19, P = 0.001).
Figure 3. Scatterplot of the principal coordinate analysis of the studied forest-steppe habitat types. Empty circle: sandy steppe, filled square: sandy pine grove, empty triangle: sandy birch grove, filled circle: loamy birch grove, filled triangle: meadow-steppe. Eigenvalues of the first and second axes were 10.51 and 6.76, respectively.
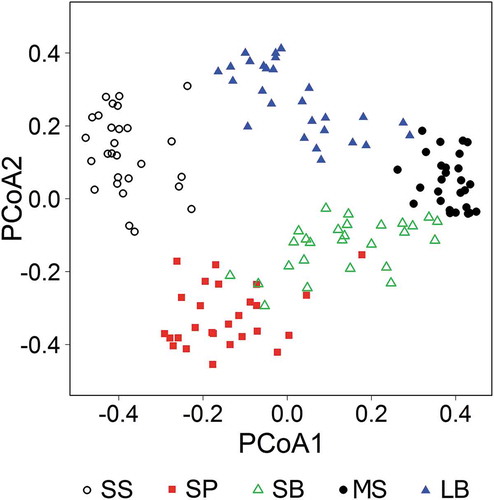
The pair-wise dissimilarity partitioning showed that nestedness was negligible compared with the turnover component in any habitat pairs ().
Table 2. Microclimatic data of the studied habitat types (mean ±SD) and the test results of the relevant comparisons (paired t-tests, *, P < 0.05).
Table 3. Nestedness and turnover resultant components of the Jaccard dissimilarity between habitat pairs (mean ± SD) expressed in per cents.
The comparisons of the species groups () showed that sandy steppes were dominated by species of psammophytic grasslands (e.g. Achillea micrantha, Festuca beckeri and Potentilla incana); species of the other groups, apart from a low cover of steppe species, were scarce. Steppe species comprised the majority of meadow-steppes (e.g. Festuca valesiaca, Filipendula vulgaris and Prunus fruticosa), supplemented with low amounts of psammophytic grassland (e.g. Carex supina) and moist grassland species (e.g. Glycirrhiza uralensis). The composition of the groves was more mixed, with no dominance of forest species in any of them. Psammophytic grassland species such as Agropyron fragile were the most common in sandy pine groves, with a significantly higher cover than forest species (t = 4.66, P < 0.001) like Elymus caninus. The cover of moist grassland (e.g. Galium verum) and steppe species (e.g. Bromus inermis) did not differ from that of the forest species (t = 1.25, P = 0.214 and t = 1.79, P = 0.077, respectively). Psammophytic grassland species of sandy birch groves formed a minor group and it was the steppe species (e.g. Bromus inermis, Spiraea hypericifolia and Thalictrum minus) that took over their place as the dominant group; their cover was higher than that of forest species (t = –3.16, P = 0.002). The cover of moist grassland species (e.g. Glycyrrhiza uralensis) did not differ significantly from that of forest species (t = 1.681, P = 0.097), while psammophytic grassland species were scarcer than forest species (t = 3.66, P < 0.001). In the loamy birch groves, steppe species such as Artemisia pontica and Festuca valesiaca were the most common, with a significantly higher cover than that of forest species (t = –4.23, P < 0.001) such as Rubus saxatilis. There was no difference between moist grassland (e.g. Lathyrus pratensis and Sanguisorba officinalis) and forest species (t = 1.36, P = 0.179). Sandy steppe species were virtually absent from this habitat type.
Figure 4. Average cover values of the distinguished species groups in the five forest-steppe habitat types. White: psammophytic grassland species, light grey: steppe species, dark grey: moist grassland species, black: forest species. SS: sandy steppe, SP: sandy pine grove, SB: sandy birch grove, MS: meadow-steppe, LB: loamy birch grove.
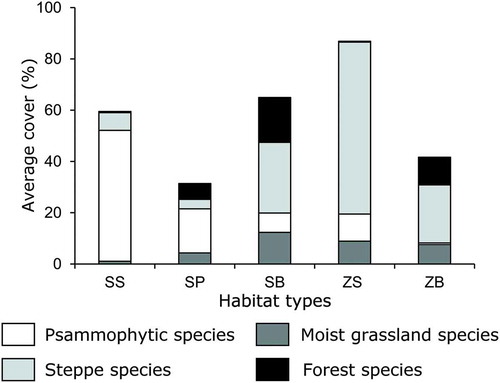
Soil moisture was very low (ca. 5%) in all three habitat types of the sandy forest-steppes. The highest values were found in the birch groves, while pine groves and steppes had significantly lower ones. Sandy pine groves tended to be even drier than sandy steppes, the difference being only marginally significant. Regarding the loamy forest-steppe, moisture values were significantly lower in the birch groves than in the meadow-steppes (, ).
Tree canopy cover differed significantly among the wooded habitat types (F = 7.89, P = 0.001). It was higher in the sandy birch groves than in the sandy pine and loamy birch groves (Tukey’s post hoc test, P = 0.033 and P < 0.001, respectively). The difference between the latter two habitats was not significant (P = 0.495) ().
Microclimatic data generally corresponded to a typical, cloudless mid-summer day (). Average daytime air temperatures were high, exceeding 30°C in most habitat types. In the sandy forest steppe, pine and birch groves did not differ from each other, while sandy steppes were warmer. The pattern was opposite at night, with lower values in the sandy steppe and higher ones in the sandy groves. Meadow-steppes had a higher daytime air temperature than birch groves in the loamy forest-steppe and the relationship was opposite at night. Daytime air humidity in the sandy habitats was highest in the birch groves, while steppes and pine groves had lower values. No significant difference could be detected between the latter two habitats. At night, pine groves had the driest air, followed by the birch groves and the steppes were the moistest. Air humidity in the loamy forest-steppe was higher in the birch groves than in the steppes during daytime but no difference was found at night.
Discussion
Competition or facilitation?
Regarding total herb layer cover, which we used as a proxy for herb layer biomass (Muukkonen et al. Citation2006), sandy steppes and meadow-steppes have higher values than the herb layer of adjacent sandy pine groves and loamy birch groves, respectively (for sandy birch groves see later). Species richness had an identical pattern, with lower values in the sandy pine and loamy birch groves than in the adjacent steppes. These patterns are consistent with a system in which tree cover suppresses plant life in the undergrowth, meaning that the net effect of trees is negative (i.e. competition).
A meta-analysis by Dohn et al. (Citation2013) has suggested that net facilitation along aridity-driven woodland-grassland transitions is more typical at the arid end of the spectrum, while competition prevails when water supply is more adequate, corresponding to the stress gradient hypothesis (Bertness and Callaway Citation1994; Blaser et al. Citation2013). Our forest-steppe system, representing the southernmost occurrences of forest patches in the region, does not seem to be in line with this as we did not detect the expected facilitative effect. The clue may lie in the fact that these areas are not only arid, but winters are also very harsh. Thus, trees need to tolerate an intense cold stress in addition to aridity. And indeed, forest-steppes north of and embedded in the northern edge of the steppe biome are dominated by cold-tolerant trees (Erdős et al. Citation2018), while tree species with adaptations for drought tolerance (e.g. with pubescent or reduced leaves) or with centres of distribution in warmer climatic belts are absent. Deciding whether this is due to a trade-off between the adaptations of woody species to these different stressors or to paleo-biogeographic legacies (cf. Magyari et al. Citation2010) is beyond the scope of this study. However, the resulting southern edge of the occurrences of woody patches is likely to be in a more northern position than what would be the case in the presence of drought-adapted trees. So, extant tree species of forest-steppes may not be able to reach those dry zones in Middle Asia where their net effects on the herb layer could shift to facilitation relative to the adjacent open grasslands.
The direct mechanism of the net negative effect may be traced back to some tree-induced micro-environmental differences between groves and open steppes. Since the herb layer vegetation persists due to its abilities to endure and take advantage of microsite conditions during the full growing season, our measurements, which were made in mid-summer, may not be fully representative. Nevertheless, mid-summer is the peak of the growing season and the interaction of trees and the herb layer can be expected to be the most intensive at this time. Thus, it is not surprising that the microsite conditions we detected are in good agreement with the vegetation pattern. Sandy pine and loamy birch groves were mostly drier than the adjacent steppes and, due to canopy shading, light availability was also lower in their undergrowth than in the steppes. These conditions can be responsible for controlling plant growth in the herb layer of groves. Although the well-known temperature ameliorating effect of trees (Vetaas Citation1992), which we also detected, may reduce heat stress and evaporation in the understorey during the day, it can also prevent dew formation at dawn. Dew has been shown to be an important source of moisture in arid ecosystems (Agam and Berliner Citation2006), and it is more likely to occur in the steppes than at ground level in the groves. Besides the physical microsite conditions, plant growth may be further suppressed in the sandy pine groves by the slowly decaying litter layer and by various allelopathic compounds released from pine needles (Hänninen et al. Citation2011).
The absence of more humid conditions in the sandy pine and loamy birch groves compared with the adjacent steppes contradicts several other studies that discuss humidity relations in forest-steppes. For instance, Dulamsuren et al. (Citation2005) have considered steppes in a diverse forest-steppe landscape of northern Mongolia drier than the driest forest patches (also Pinus sylvestris groves), Erdős et al. (Citation2013) have reported moister soils in the Populus alba groves of a Hungarian forest-steppe than in adjacent grasslands based on Ellenberg-type indicators, and Wilson and Kleb (Citation1996) have measured higher soil moisture in P. tremuloides groves than in adjacent prairies. The preconception of these authors may have been based on several general phenomena. Trees reduce evapotranspiration under their canopy by shading (Amundson et al. Citation1995) and can improve the water economy of the soil by improving soil structure (Vetaas Citation1992). However, trees themselves also evaporate considerable quantities of water and reduce the amount of water reaching the ground by intercepting precipitation with their canopy (Gea-Izquierdo et al. Citation2009; Barbeta and Penuelas Citation2016). Our findings suggest that the negative effects of trees on water balance can override the positive ones in the southernmost forest patches of the region, where precipitation is lower and/or summer temperature is higher than that reported in other studies (e.g. Dulamsuren et al. Citation2005; Erdős et al. Citation2013; Wilson and Kleb Citation1996). In this sense, these forest patches are not necessarily moist micro-habitats surrounded by a dry matrix but may be drier enclaves during rain-free periods in mid-summer. In spring, a reverse situation could potentially allow the growth of annuals (or early spring geophytes); however, we did not detect dry remains of such species (with the exception of some dry Tulipa schrenkii stems) and the published species lists of the areas include mostly hemicryptophytic perennial species (Bragina et al. Citation2007).
Sandy birch groves were different from the patterns outlined above. Their total herb layer cover was higher than that of sandy steppes and species richness was not different between sandy birch groves and sandy steppes. We explain this anomaly compared with the other grove-steppe pairs by the low-lying topographic position of sandy birch groves. Lying in dune-slacks, sandy birch groves can have more humid and more nutrient-rich conditions as a result of surface runoff and the close vicinity of the water table, a pattern well-described in other inland sand dune ecosystems (e.g. Molnár et al. Citation2003; Tölgyesi et al. Citation2016). Our environmental measurements confirmed these, as the highest soil moisture within the sandy forest-steppe was detected in the sandy birch groves and the microclimatic parameters were also mostly more favourable in these groves than in the other sandy habitat types. The benefits of growing in the dune-slacks appear to override the negative effect of the low light availability (which was lower than in the sandy pine groves). Thus, if excess water supports the undergrowth of groves compared with the surrounding steppe habitats, the herb layer can outperform the steppe, not as a result of the protective effect of the trees but probably despite their negative effects.
Another interesting finding regarding sandy birch groves is that their herb layer cover was greater than that of loamy birch groves while having similar species richness. These findings are unexpected as all microsite properties (and that of the annual precipitation) seem to be more favourable in the loamy birch groves than in the sandy ones. Light availability is higher in the loamy birch groves, and the chestnut soil (good water-holding capacity and high humus content) is also better for plant growth than the sandy soil (poor water-holding capacity and low humus content) of the sandy birch groves. Although we have no information on the depth of the water table in the loamy forest-steppe, it is evident that a high proportion of the precipitation is retained in the topsoil. Thus, the roots of trees and herbaceous species are forced to directly compete for water in the upper layer, which has been shown to mutually hinder the growth of trees and herb layer species (Dawson Citation1993; Scholes and Archer Citation1997). Conversely, precipitation seeps down to deeper layers in sandy birch groves, precluding direct competitive interaction between the roots of trees and herb layer species. This mechanism, known as spatial resource partitioning, has been shown to support the coexistence of trees and herb layer species in various ecosystems (Weltzin and McPherson Citation1997).
Community assembly
According to the compositional analysis, each habitat type had its distinct assemblage. Since the herb layer of the groves that received no extra water supply compared with the steppes had lower cover, fewer species and generally more demanding environmental conditions than steppes, a plausible assembly rule would be a simple filtering of the ‘toughest’ from the species pool of the surrounding steppes. This would, however, lead to a high nestedness, which we could not confirm. On the contrary, the high turnover indicated that groves had their own species pool. This was not caused exclusively by the appearance of forest species, which could be expected in forested patches. The herb layer of groves was dominated by grassland species, while forest specialists were scarce. The lack of propagules may have a role in this pattern; however, these groves are remains of prehistoric continuous forests that have broken up owing to climatic shifts during the Holocene (Hoffmann and Usoltsev Citation2001) and there have always been some groves in the region ever since. Thus, the lack of propagules cannot be the primary cause for the low abundance of forest species.
Low species richness and the scarcity of forest species are also typical features of forested patches in European forest-steppes, such as the ones in the Carpathian Basin. However, these are explained by the secondary character of those groves as trees were almost completely cleared from most places in the Middle Ages and regenerated in the twentieth century (Biró et al. Citation2008; Erdős et al. Citation2015a). As a result, lower conservation values are assigned to groves with poor undergrowth and a lack of forest species (Molnár et al. Citation2000; Erdős et al. Citation2017). However, our findings indicate that natural groves of forest-steppes are inherently suboptimal for forest specialist species. Therefore, caution should be applied when using herb layer composition to prioritise forest-steppe fragments.
Regarding grassland species found in the groves, it seems that groups preferring more stable conditions than what is offered by the steppes occur in higher abundance. Loamy birch groves host several moist grassland species besides the steppe ones and sandy birch groves are inhabited by steppe species and moist grassland species. Thus, for grassland species (and also for those few forest specialists we detected), probably the reduced heat stress under the canopy is compensated for by the reduced moisture availability. Although sandy pine groves are dominated by psammophytic grassland species, the low nestedness with sandy steppes indicates that pine groves have their own psammophytic grassland species in the area (e.g. Agropyron fragile), probably those that tolerate drier and shadier conditions better than the heat stress of open sandy sites.
Similarly, high occurrences of grassland species have been reported from the groves of other forest-steppe regions such as the Carpathian Basin (Erdős et al. Citation2015b) or Siberia (Chytry et al. Citation2007, Citation2012) and have mostly been explained by the high light availability in the herb layer due to the low canopy cover of trees. However, we think that the reason lies in a more complex ecosystem engineering effect of the trees and in the resulting community assembly rules.
Conclusions
As forested patches appear at the northern edge of Middle-Asian steppes, they create new micro-habitats in the landscape. Interestingly, these islands of trees appear to offer little protection for the herb layer against the harsh environmental conditions of the region and seem to have a net negative effect, probably by making the environmental conditions even harsher. Herb layer communities of these groves are not simply nested subsets of the surrounding steppes but have their own species pool, greatly increasing the overall plant diversity and conservation value of the landscapes. Most species found in the groves are not forest specialists; the conditions are presumably inappropriate for most of them, so forested enclaves cannot function as refuges for sensitive forest specialists during the northward migration of the forest-steppe zone either. Taking these into consideration, climate change may have profound effects on the herb layer of more northern forested habitats (e.g. the presently species-rich hemiboreal forests; see, e.g., Chytry et al. Citation2012) and it might be the trees that directly drive this effect. The negative effect of trees may lead to the decrease of forest specialists and the spreading of various grassland species in the herb layer of these forest groves, along with a general impoverishment of the communities, maybe even before the overall physiognomy of the landscapes would turn to more open.
Supplemental Material
Download Zip (39.7 KB)Acknowledgements
We express our gratitude to the Kostanay State Pedagogical Institute and the Science-Research Centre of the Problems of Ecology and Biology of KSPI for their guidance during the research in Kazakhstan.
Disclosure statement
No potential conflict of interest was reported by the authors.
Supplementary material
Supplementary data for this article can be accessed here.
Additional information
Funding
Notes on contributors
Csaba Tölgyesi
Csaba Tölgyesi is an assistant professor. His main interest is the ecology of forest-grassland mosaics like the forest-steppe transition or European wood-pastures.
Orsolya Valkó
Orsolya Valkó is an associate professor. Her field of interest covers the restoration, management and conservation of steppe ecosystems.
Balázs Deák
Balázs Deák is a research fellow. His research covers the restoration and management of steppe vegetation, with a special focus on kurgans.
András Kelemen
András Kelemen is a research fellow. His research focuses on facilitative plan-plant interactions and various fine-scale patterns and mechanisms in vegetation ecology.
Tatyana M. Bragina
Tatyana M. Bragina is a professor. She is committed to the conservation of steppe ecosystems and has contributed to the establishment and ecological exploration of several nature reserves in the country.
Róbert Gallé
Róbert Gallé is an associate professor. His main interest is the ecology of heterogeneous landscapes, including both natural and man-made types.
László Erdős
László Erdős is a research fellow. His main research interest is the vegetation ecology of forest-steppes.
Zoltán Bátori
Zoltán Bátori is an associate professor. He is interested in climatic refuges and their role in preserving biodiversity under climate change.
References
- Agam N, Berliner PR. 2006. Dew formation and water vapour adsorption in semi-arid environments - A review. J Arid Environ. 65:572–590.
- Amundson RG, Ali AR, Belsky AJ. 1995. Stomatal responsiveness to changing light intensity increases rain-use efficiency of below-crown vegetation in tropical savannas. J Arid Environ. 29:139–153.
- Armas C, Rodríguez-Echeverría S, Pugnaire FI. 2011. A field test of stress-gradient hypothesis along an aridity gradient. J Vegetation Sci. 22:818–827.
- Barbeta A, Penuelas J. 2016. Sequence of plant responses to droughts of different timescalses: lessons from holm oak (Quercus ilex) forests. Plant Ecol Divers. 9:321–338.
- Baselga A. 2010. Partitioning the turnover and nestedness components of beta diversity. Glob Ecol Biogeogr. 19:134–143.
- Bertness M, Callaway RM. 1994. Positive interactions in communities. Trends Ecol Evol. 9:191–193.
- Biró M, Révész A, Molnár Z, Horváth F, Czúcz B. 2008. Regional habitat pattern of the Danube-Tisza Interfluve in Hungary II. Acta Biol Hung. 50:19–60.
- Blaser WJ, Sitters J, Hart SP, Edwards PJ, Venterink HO. 2013. Facilitative or competitive effects of woody plants on understorey vegetation depend on N-fixation, canopy shape and rainfall. J Ecol. 101:1598–1603.
- Bragina TM 2004. Regularities of changes in the soil fauna communities under desertification [dissertation]. Moscow: A.N. Severtsov Institute for Ecology and Evolution of the Russian Academy of Sciences.
- Bragina TM, Bragin EA, Tairbergenov Y, Sidorova TV, Rachkovskaya EI, Geldieva G, Shestakov AS, Pereladova OB, Dietrich T, Koshkin M, et al. 2007. Saryarka – steppe and lakes of Northern Kazakhstan. Nomination dossier for inscription on the list of cultural and natural world heritage of UNESCO; [accessed 2017]. http://whc.unesco.org/uploads/nominations/1102rev.pdf
- Brinkert A, Hölzel N, Sidorova TV, Kamp J. 2016. Spontaneous steppe restoration on abandoned cropland in Kazakhstan: grazing determines successional pathways. Biodivers Conserv. 12:2543–2561.
- Cadenasso ML, Pickett STA, Weathers KC, Bell SS, Benning TL, Carreiro MM, Dawson TE. 2003. An interdisciplinary and synthetic approach to ecological boundaries. Bioscience. 53:717–722.
- Chhin S, Wang GG. 2002. Spatial and temporal pattern of white spruce regeneration within mixed-grass prairie in the spruce woods provincial park of manitoba. J Biogeogr. 29:903–912.
- Chytry M, Danihelka J, Ermakov N, Hájek M, Hájková P, Koci M, Kubesová S, Lustyk P, Otypková Z, Popov D, et al. 2007. Plant species richness in continental southern Siberia: effects of pH and climate in the context of the species pool hypothesis. Glob Ecol Biogeogr. 16:668–678.
- Chytry M, Ermakov N, Danihelka J, Hajek M, Hajkova P, Horsak M, Koci M, Kubesova S, Lustyk P, Otypkova Z, et al. 2012. High species richness in hemiboreal forests of the northern Russian Altai, southern Siberia. J Vegetation Sci. 23:605–616.
- D’Odorico P, He Y, Collins S, De Wekker SFJ, Engel V, Fuentes JD. 2013. Vegetation-microclimate feedbacks in woodland-grassland ecotones. Glob Ecol Biogeogr. 22:364–379.
- Daly C, Bachelet D, Lenihan JM, Neilson RP, Parton W, Ojima D. 2000. Dynamic simulation of tree-grass interactions for global change studies. Ecological Appl. 10:449–469.
- Dawson TE. 1993. Hydraulic lift and water use by plants-implications for water balance, performance and plant-plant interactions. Oecologia. 95:565–574.
- Deák B, Valkó O, Török P, Tóthmérész B. 2016. Factors threatening grassland specialist plants – A multi-proxy study on the vegetation of isolated grasslands. Biol Conserv. 204:255–262.
- Dohn J, Dembele F, Karembe M, Moustakas A, Amevor KA, Hanan NP. 2013. Tree effects on grass growth in savannas: competition, facilitation and the stress-gradient hypothesis. J Ecol. 101:202–209.
- Dulamsuren C, Hauck M, Mühlenberg M. 2005. Ground vegetation in the Mongolian taiga forest-steppe ecotone does not offer evidence for the human origin of grasslands. Appl Vegetation Sci. 8:149–154.
- Erdős L, Ambarli D, Anenkhonov OA, Bátori Z, Cserhalmi D, Kiss M, Kröel-Dulay G, Liu H, Magnes M, Molnár Z, et al. 2018. The edge of two worlds: A new synthesis on Eurasian forest-steppes. Appl Vegetation Sci. 21:345–362.
- Erdős L, Bátori Z, Penksza K, Dénes A, Kevey B, Kevey D, Magnes M, Sengl P, Tölgyesi C. 2017. Can naturalness indicator values reveal habitat degradation? A test of four methodological approaches. Polish J Ecol. 65:1–13.
- Erdős L, Gallé R, Körmöczi L, Bátori Z. 2013. Species composition and diversity of natural forest edges: edge responses and local edge species. Community Ecol. 14:48–58.
- Erdős L, Tölgyesi C, Cseh V, Tolnay D, Cserhalmi D, Körmöczi L, Gellény K, Bátori Z. 2015a. Vegetation history, recent dynamics and future prospects of a Hungarian sandy forest-steppe reserve: forest-grassland relations, tree species composition and size-class distribution. Community Ecol. 16:95–105.
- Erdős L, Tölgyesi C, Horzse M, Tolnay D, Hurton Á, Schulz N, Körmöczi L, Lengyel A, Bátori Z. 2014. Habitat complexity of the Pannonian forest-steppe zone and its nature conservation implications. Ecological Complexity. 17:107–118.
- Erdős L, Tölgyesi C, Körmöczi L, Bátori Z. 2015b. The importance of forest patches in supporting steppe-species: a case study from the Carpathian Basin. Polish J Ecol. 63:213–222.
- Fekete G, Somodi I, Molnár Z. 2010. Is chorological symmetry observable within the forest steppe biome in Hungary? - A demonstrative analysis of floristic data. Community Ecol. 11:140–147.
- Fernández-Moya J, San Miguel-Ayanz A, Ca Nellas I, Gea-Izquierdo G. 2011. Variability in Mediterranean annual grassland diversity driven by small-scale changes in fertility and radiation. Plant Ecol. 212:865–877.
- Feurdean A, Marinova E, Nielsen AB, Liakka J, Veres D, Hutchinson SM, Braun M, Timar-Gabor A, Astalos C, Mosburgger V, et al. 2015. Origin of the forest steppe and exceptional grassland diversity in Transylvania (central-eastern Europe). J Biogeogr. 42:951–963.
- Gea-Izquierdo G, Montero G, Canellas I. 2009. Changes in limiting resources determine spatio-temporal variability in tree-grass interactions. Agroforestry Syst. 76:375–387.
- Habel JC, Dengler J, Janisová M, Török P, Wellstein C, Wiezik M. 2013. European grassland ecosystems: threatened hotspots of biodiversity. Biodivers Conserv. 22:2131–2138.
- Hänninen T, Kontturi E, Vuorinen T. 2011. Distribution of lignin and its coniferyl alcohol and coniferyl aldehyde groups in Picea abies and Pinus sylvestris as observed by Raman imaging. Phytochemistry. 72:1889–1895.
- Hijmans RJ, Cameron SE, Parra JL, Jones PG, Jarvis A. 2005. Very high resolution interpolated climate surfaces for global land areas. Int J Climatol. 25:1965–1978.
- Hirota M, Holmgren M, Van Nes EH, Scheffer M. 2011. Global resilience of tropical forest and savanna to critical transitions. Science (80-). 334:232–235.
- Hoffmann CW, Usoltsev VA. 2001. Modelling root biomass distribution in Pinus sylvestris forests of the Turgai Depression of Kazakhstan. For Ecol Manage. 149:103–114.
- Hoffmann WA, Geiger EL, Gotsch SG, Rossatto DR, Silva LCR, Lau OL, Haridasan M, Franco AC. 2012. Ecological thresholds at the savanna-forest boundary: how plant traits, resources and fire govern the distribution of tropical biomes. Ecol Lett. 15:759–768.
- Kamp J, Koshkin MA, Bragina TM, Katzner TE, Milner-Gulland EJ, Schreiber D, Sheldon R, Shmalenko A, Smelansky I, Terraube J, et al. 2016. Persistent and novel threats to the biodiversity of Kazakhstan’s steppes and semi-deserts. Biodivers Conserv. 25:2521–2541.
- Kämpf I, Mathar W, Kuzmin I, Hölzel N, Kiehl K. 2016. Post-Soviet recovery of grassland vegetation on abandoned fields in the forest steppe zone of Western Siberia. Biodivers Conserv. 25:2563–2580.
- Kelemen A, Valkó O, Kröel-Dulay G, Deák B, Török P, Tóth K, Miglécz T, Tóthmérész B. 2016. The invasion of common milkweed (Asclepias syriaca) in sandy old-fields - is it a threat to the native flora? Appl Vegetation Sci. 19:218–224.
- Komarov VL. 1968-2002. Flora of the U.S.S.R. Washington: Smithsonian Institution Libraries.
- Laurance WF, Didham RK, Power ME. 2001. Ecological boundaries: a search for synthesis. Trends Ecol Evol. 16:70–71.
- López-Sánchez A, Miguel AS, López-Carrasco C, Huntsinger L, Roig S. 2016. The important role of scattered trees on the herbaceous diversity of grazed Mediterranean dehesa. Acta Oecologica. 76:31–38.
- Magyari EK, Chapman JC, Passmore DG, Allen JRM, Huntley JP, Huntley B. 2010. Holocene persistence of wooded steppe in the Great Hungarian Plain. J Biogeogr. 37:915–935.
- Mancini MV. 2009. Holocene vegetation and climate changes from a peat pollen record of the forest – steppe ecotone, Southwest of Patagonia (Argentina). Quat Sci Rev. 28:1490–1497.
- Manning AD, Fischer J, Lindenmayer DB. 2006. Scattered trees are keystone structures – implications for conservation. Biol Conserv. 132:311–321.
- Molnár Z, Papp L, Molnár A, Horváth A, Kevey B, Schmotzer A, Vidra T, Király G, Bölöni J, Virágh K. 2000. Az alföldi erdőssztyeppek mai helyzete Magyarországon: A fennmaradt állományok adatbázisa. In: Molnár Z, Kun A, editors. Alföldi erdőssztyepp-maradványok Magyarországon. WWF füzetek. Vol. 15. Budapest: WWF Magyarország; p. 42–48.
- Molnár Z, Biró M, Bartha S, Fekete G. 2012. Past trends, present state and future prospects of Hungarian forest-steppes. In: Werger MJA, van Staalduinen MA, editors. European Steppes. Berlin: Springer; p. 209–252.
- Molnár Z, Sipos F, Vidéki R, Biró M, Iványosi-Szabó A. 2003. Dry sand vegetation of the Kiskunság. Budapest: TermészetBÚVÁR Alapítvány Kiadó.
- Muukkonen P, Mäkipää R, Laiho R, Minkkinen K, Vasander H, Finér L. 2006. Relationship between biomass and percentage cover in understorey vegetation of boreal coniferous forests. Silva Fenn. 40:231–245.
- Novenko EY, Tsyganov AN, Rudenko OV, Volkova EV, Zuyganova IS, Babeshko KV, Olchev AV, Losbenev NI, Payne RJ, Mazei YA. 2016. Mid- and late-Holocene vegetation history, climate and human impact in the forest-steppe ecotone of European Russia: new data and a regional synthesis. Biodivers Conserv. 25:2453–2472.
- Rivest D, Rolo V, López-Díaz L, Moreno G. 2011. Shrub encroachment in Mediterranean silvopastoral systems: retama sphaerocarpa and Cistus ladanifer induce contrasting effects on pasture and Quercus ilex production. Agric Ecosyst Environ. 141:447–454.
- Scholes RJ, Archer SR. 1997. Tree-grass interactions in savannas. Annu Rev Ecol Syst. 28:517–544.
- Staal A, Flores BM. 2015. Sharp ecotones spark sharp ideas: comment on “Structural, physiognomic and above-ground biomass variation in savanna–forest transition zones on three continents – how different are co-occurring savanna and forest formations?” by Veenendaal et al. (2015). Biogeosciences. 12:5563–5566.
- Staver AC, Archibald S, Levin SA. 2011. The global extent and determinants of savanna and forest as alternative biome states. Science. 334:230–232.
- Tchebakova N, Parfenova E, Soja A. 2009. The effects of climate, permafrost and fire on vegetation change in Siberia in a changing climate. Environ Res Lett. 4:045013.
- Tinya F, Márialiget S, Király I, Németh B, Ódor P. 2009. The effect of light conditions on herbs, bryophytes and seedlings of temperate mixed forests in Őrség, Western Hungary. Plant Ecol. 204:69–81.
- Tölgyesi C, Zalatnai M, Erdős L, Bátori Z, Hupp N, Körmöczi L. 2016. Unexpected ecotone dynamics of a sand dune vegetation complex following water table decline. J Plant Ecol. 9:40–50.
- Vetaas OR. 1992. Micro-site effects of trees and shrubs in dry savannas. J Vegetation Sci. 3:337–344.
- Walter H, Breckle S-W. 1989. Ecological systems of the geobiosphere 3 – temperate and polar zonobiomes of northern Eurasia. Berlin: Springer.
- Weltzin JF, McPherson GR. 1997. Spatial and temporal soil moisture resource partitioning by trees and grasses in a temperate savanna, Arizona, USA. Oecologia. 112:156–164.
- Wesche K, Ambarli D, Kamp J, Török P, Treiber J, Dengler J. 2016. The Palearctic steppe biome: A new synthesis. Biodivers Conserv. 25:2197–2231.
- Williams JW, Shuman B, Bartlein PJ. 2009. Rapid responses of the prairie-forest ecotone to early Holocene aridity in mid-continental North America. Glob Planet Change. 66:195–207.
- Wilson SD, Kleb HR. 1996. The influence of prairie and forest vegetation on soil moisture and available nitrogen. Am Midl Nat. 136:22–231.