ABSTRACT
Background
As agriculture has intensified, many once-common wildflowers have declined in arable landscapes, which has widespread implications for associated ecosystem services. The incorporation of sustainable practices, for example, growing living mulches (in-field, non-crop plant ground cover, maintained during the target crop growing season), can boost arable biodiversity, but few wildflower species have been utilised in this context.
Aims
Our aim was to determine the suitability of arable wildflower species, once considered weeds, for use as living mulches.
Methods
We first screened a number of arable wildflower species for germination when growing with a common cereal, barley (Hordeum vulgare). We then grew two (Centaurea cyanus and Scandix pecten-veneris) in pots in a glasshouse with and without barley, and grew barley alone to test the impact of the wildflowers on barley growth and biomass.
Results
Neither of the wildflowers significantly negatively impacted barley biomass. Barley initially facilitated germination in S. pecten-veneris, but ultimately suppressed the above-ground biomass of both wildflowers. However, both wildflower species were able to coexist alongside barley.
Conclusions
Our experiment provides evidence that wildflowers that were considered weeds in traditional agriculture have the potential to be grown alongside barley and could be incorporated as part of a living mulch.
Introduction
One of the biggest recent international concerns has been the dramatic decline in global biodiversity over the past century across multiple taxonomic groups (RBG Kew Citation2016; Ceballos et al. Citation2017; Hallmann et al. Citation2017; Willis Citation2017). The general consensus is that this has been caused by the action of humans, and that a major contributing factor is land use change (Potts et al. Citation2010; Willis Citation2017). Modern agricultural practices with a high-input approach are often incompatible with conservation, and the successful increase of agricultural yields has come at a cost to biodiversity (Matson et al. Citation1997). In particular, this had led to a major decline in arable wildflowers (Wilson and King Citation2003), with knock-on effects on birds (DEFRA Citation2018) and pollinators (Potts et al. Citation2010).
The removal of potential competitor plant species is a key management goal of many agricultural cropping systems to maximise the yield of the target species (Brooker et al. Citation2016). However, ecological studies have demonstrated that plants do not only compete for resources with each other but that they can also facilitate each other’s growth and survival, or have no discernible negative effects whilst co-existing (Brooker et al. Citation2008). Academic interest in facilitation (positive, non-trophic interactions between physiologically independent plants) has increased over the past decades and there is good evidence that facilitative interactions are more common than was previously thought (Brooker et al. Citation2008). Importantly, recent research has highlighted that such beneficial interactions can also be found in agricultural systems and could be central to the development of sustainable food production (Brooker et al. Citation2015; Vincent et al. Citation2017; Izumi et al. Citation2018; Yin et al. Citation2020).
Facilitative and competitive interactions, however, appear to be a continuum, and the balance of interactions within a system can switch depending on the influence of the environment (Brooker et al. Citation2008) and the traits of the interacting species (Schöb et al. Citation2013). To increase the diversity of plants in agricultural settings, we need to develop our understanding of when plant–plant interactions are likely to be positive or negative for key ecosystem functions (i.e., productivity, pest and disease resistance, soil health), and how non-crop diversity can support some of these functions.
One way to increase biodiversity in arable fields, whilst also providing potential benefits to crop plants, is by the use of living mulches (Hartwig and Ammon Citation2002). Living mulches are single species or simple plant communities sown as an understorey and maintained as a living ground cover during the growing season of the target crop. They provide ground cover that prevent soil erosion (Hartwig and Ammon Citation2002), and can also enhance the abundance of invertebrate species (i.e., pollinators, predators, parasitoids) beneficial to the crop (Rjg et al. Citation2012; George et al. Citation2015). The species composition of living mulches tends to be dominated by legumes, such as clover (Trifolium spp.) (Hiltbrunner et al. Citation2007; Baresel et al. Citation2018). Incorporating legumes into arable fields can improve soil nitrogen and phosphorus status through biological nitrogen fixation (BNF) (Iannetta et al. Citation2016) and formation of arbuscular mycorrhizal (AMF) relationships (Scheublin et al. Citation2004) and can also attract pollinating insects (Sands and Rowntree Citation2016). However, some legume species can be highly competitive (Den Hollander et al. Citation2007), and competition with crops can lead to a reduction in yield (Thorsted et al. Citation2006), a loss of economic benefit to the farmer, and declining enthusiasm for sustainable agricultural approaches.
There are, however, opportunities to broaden the types of plants used in living mulches and to include some rare species in mixtures (Germeier Citation2000). Central to this idea is recent research that has shown that the beneficial effects on ecosystem functions resulting from enhanced diversity in species-rich plant communities (Cardinale et al. Citation2012) can also be delivered without the inclusion of legumes (Schöb et al. Citation2018). Although historically viewed as potential weeds, many arable wildflowers have declined over the past century because they are poorly adapted to intensive farming and have not developed the more aggressive traits of now common and potentially pernicious arable weeds (Wilson and King Citation2003). This may make it possible to reintroduce key components of native farmland plant diversity without introducing highly competitive species that depreciate crop yield, and at the same time, enhance ecosystem functions to support the development of more sustainable farming systems.
Here, we used an experimental approach, first to screen a number of arable wildflowers for germination responses when grown with and without a common arable crop, barley (Hordeum vulgare L.) in a greenhouse. We then selected two species with alternate responses (enhanced and supressed germination with barley) and investigated interactions with the crop in a greenhouse until production of seed. Our key objectives were (1) to determine whether there were negative or positive effects on barley biomass when grown in combination with each arable wildflower and (2) to determine whether the arable wildflowers would grow alongside the barley and might therefore be suitable for inclusion in living mulch mixtures and further testing in the field.
Materials and methods
Study species and experimental set-up
Arable plant species were selected from those outlined by Byfield and Wilson (Citation2005) and then chosen by their availability from UK wildflower seed suppliers. A full species list with supplier information can be found in . Barley (Hordeum vulgare), cultivar Odyssey was used for the experiments and supplied by Openfield Agriculture (Grantham, UK). Two greenhouse experiments were established from September 2017 to May 2018 at Manchester Metropolitan University in Manchester, England (53°28ʹ16.83” N, 2°14ʹ28.09 W).
Table 1. Full list of rare arable species and supplier information used in Experiments 1 and 2
Experiment 1 – Wildflower species screening
Sixteen rare arable wildflower species () were sown either in monoculture or alongside barley into a sandy clay loam substrate (Boughton Kettering loam, Amenity Land Solutions, Telford, UK) in individual trays (15 x 21 × 5 cm). Nutrient analysis of the soil was not undertaken, but typical values for Broughton Kettering loam are 5% total organic matter, 4.5 μg g−1 available phosphorus, 135 μg g−1 available potassium and a pH (1:1 soil:water) of 7.1 (R.Chinn, Boughton pers. comm.). A barley monoculture treatment was also included and there were five replicates per species per treatment giving a total of 165 replicate trays. Barley and wildflower seeds were sown simultaneously, and sowing density was designed to correspond to typical sowing densities used in larger field trials (Brooker et al. Citation2018), with arable wildflowers sown at a density of nine seeds per tray (corresponding to a field density of ca. 300 seed m−2), and barley sown at six seeds per tray (ca. 220 seed m−2). An additive approach was used for sowing, so that an individual arable wildflower + barley replicate contained nine arable plant seeds plus six barley seeds, whereas monocultures contained either nine wildflower or six barley seeds. This approach was taken to best mimic field conditions where living mulches are used. The barley was sown at a depth of 3 cm and the arable wildflower species were sown, either on or just below the soil surface, according to the instructions from the supplier. The seeds were spread evenly across the trays and were not sown in any pattern. The replicates were placed in a randomised block design and grown in a single glasshouse for the duration of the experiment. Pots were not re-randomised during the experiment. Additional artificial lighting, heating and ventilation was utilised to mimic spring and early summer conditions. This was provided by Osram Plantastar HPS 400 W lamps, hung 140 cm above the bench, which had a rated and nominal luminous flux value of 54,800 lm. Each tray was uniformly drenched with tap water at the point of sowing, and subsequently watered to maintain moist soil conditions. No fertiliser was added over the duration of the experiment.
The trays were monitored daily from the day after sowing for 30 days. Each day, the count of living, germinated arable wildflower and barley seedlings was recorded for each tray. Most germination occurred within the first 14 days of the experiment; however, the trays were monitored for a further 16 days by which time germination rate had reached a plateau in all treatments.
Experiment 2 – Interactions between selected wildflower species and barley
The arable wildflowers Centaurea cyanus and Scandix pecten-veneris were selected for further investigation as they displayed divergent germination responses to the presence of barley in Experiment 1. The same treatments were used as in Experiment 1 (monocultures and mixtures of each wildflower species with barley), but seeds were sown into deeper pots (15 × 13 × 12 cm) to facilitate long-term growth of the plants. For each treatment, a total of 33 replicates were sown, 11 of which were randomly assigned to one of three harvests. Seeds were sown into a 1:1 mix of sandy clay loam (Boughton Kettering loam, Amenity Land Solutions, Telford, UK) and sharp sand (Kelkay, Pollington, UK), with the addition of sand intended to facilitate extraction of the roots at harvest. Barley and wildflower seeds were sown simultaneously, as previously, at a density of 300 seed m−2 (arable wildflowers) and 220 seed m−2 (barley) (Brooker et al. Citation2018), which corresponded to six arable wildflower seeds per pot and four barley seeds. Barley seeds were sown at a depth of around 3 cm and the wildflower seeds on or just below the surface of the soil. Seeds were spread evenly across the pots and not planted in a particular pattern. The replicates were placed in a randomised block design and grown in the same glasshouse, under the same regime as for Experiment 1 and without the addition of fertiliser. Pots were monitored daily for emergence up until the first harvest.
The first harvest was conducted at 42 days after sowing once all plants that germinated had begun to grow, the second at 92 days prior to production of any flower or fruiting structures in any of the plant species, and the third at 162 days, when flowers and fruits had formed in all of the plant species. In harvests one and two, both the above-ground and below-ground biomass were harvested, shoots and roots were separated, and roots washed to remove adhering soil. At these harvests, no fruiting structures had formed on any of the plants. At harvest three, only the above-ground biomass was harvested, but shoots were separated from fruiting material (comprised of the entire inflorescence). We were unable to collect root biomass data with confidence at the final harvest, as the roots had grown to fill the experimental pots and separation of species was not possible. All harvested biomass was oven dried at 70°C for 72 h. Dry material was weighed to the nearest 0.01 mg to obtain per replicate biomass for shoots (above-ground; all harvests), roots (below-ground; harvests one and two) and fruits (flower/seed head; harvest three). The shoot:root ratio was calculated for each species at harvests one and two.
Statistical analyses
All statistical analyses were conducted in the R environment (version 3.6.1) (R Core Team Citation2019). Germination data were visually inspected and the mean difference in germination success of each wildflower species with and without barley 30 days after planting was analysed using randomisation tests with 5000 resamplings. For experiment two, all data except for barley fruit biomass were log-transformed prior to analyses to improve normality of residuals. Barley shoot and root biomass and shoot:root ratio data at harvests one and two were analysed with a two-way fully factorial linear model with arable wildflower presence and absence (3 treatment levels: C. cyanus present, S. pecten-veneris present and no wildflowers) and harvest included as factors. Where appropriate, post hoc one-way ANOVAs were then undertaken at each harvest to determine effects of the presence of the arable plants at the different time points. Barley fruit data from harvest three was analysed using a one-way linear model where arable wildflower presence (3 treatment levels: C. cyanus present, S. pecten-veneris present and no wildflowers) was the only factor. Arable wildflower data were analysed as above, but with barley presence or absence (2 treatment levels) and harvest (where appropriate) as the factors. The per cent mean biomass change from control treatments was estimated for all fruit data at harvest three and Hedges d effect sizes calculated (Rosenberg et al. Citation2013).
Results
Experiment 1
Of the arable wildflowers tested, no seeds germinated for three species (Bupleurum rotundifolium L., Chrysanthemum segetum L., Lithospermum arvense L.) and all seeds germinated for one species (Silene noctiflora L.) across all treatments. These were removed from further analyses. Germination rates varied across replicates for all other species. Germination for C. cyanus was marginally significantly lower in the presence of barley (P = 0.07) and occurred at about the same time as the barley. Germination for S. pecten-veneris was marginally significantly higher in the presence of barley (P = 0.06) and occurred after the barley had germinated. These species were chosen for further investigation in experiment two. There were no significant differences for any other species (, see for full results).
Table 2. Significance values comparing germination rates of the arable wildflowers grown with and without barley in a glasshouse experiment. Values were generated from randomisation tests with 5000 re-samplings. NA denotes species where either no seeds germinated† or all seeds planted germinated‡
Experiment 2
Barley
The presence of the two arable wildflowers did not significantly reduce final barley shoot or fruit biomass, with the largest effects on barley characteristics resulting from harvest date. For shoot biomass, the full model was highly significant, explaining the majority of variation in the data (F8,89 = 397.6, R2adj = 0.97, P < 0.0001). There was no significant effect of arable wildflower (F2,89 = 1.62, P = 0.20), but a significant effect of harvest (F2,89 = 1585.73, P < 0.0001) and no interaction between harvest and arable wildflower (F4,89 = 1.60, P = 0.18; ). For root biomass, the full model was highly significant, explaining a large proportion of the variation in the data (F5,59 = 74.22, R2adj = 0.85, P < 0.0001). There was a marginally significant effect of arable wildflower (F2,59 = 1.28, P = 0.09) with a tendency for increased root biomass at harvest two when no arable wildflowers were present, a significant effect of harvest (F1,59 = 361.80, P < 0.0001) and no significant interaction between the two (F2,59 = 1.07, P = 0.13; ). For shoot:root ratio, although significant, the model explained much less variation in the data (F5,59 = 4.90, R2adj = 0.23, P < 0.0008). For these data there was a significant effect of arable wildflower (F2,59 = 5.85, P = 0.005) as well as harvest (F1,59 = 9.35, P = 0.003) but no interaction between the two (F2,59 = 1.71, P = 0.19; ). The wildflower effects were caused by a decreased shoot:root ratio at harvest two in the absence of arable wildflowers (P = 0.005). For fruit biomass, the full model was not significant (F2,30 = 0.37, R2adj = −0.04, P = 0.69), although the presence of either wildflower species reduced mean biomass by 9%, equating to a Hedges d effect size of 0.3 for each species ().
Figure 2. Shoot biomass (a), root biomass (b), shoot:root ratio (c) and fruit (inflorescence) biomass (d) of barley at harvest 1 (42 days after sowing – square), harvest 2 (92 days after sowing – circle) and harvest 3 (162 days after sowing – triangle) when grown in the presence of either Centaurea cyanus or Scandix pecten-veneris or without any other plants in a glasshouse experiment. There were no significant negative effects of the arable wildflowers on the barley, but a significant increase in barley shoot:root ratio (relative shoot investment) when arable wildflowers were present (P = 0.005) at harvest two. Means of untransformed data (n = 11*) are presented and error bars are 95% confidence intervals. *n = 10 for barley grown alone at harvest 2
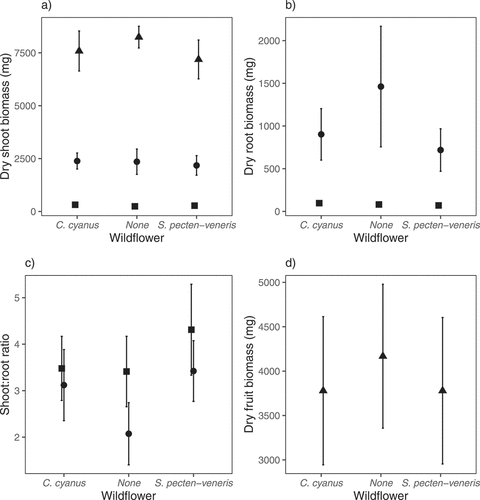
Centaurea cyanus
Presence of barley decreased shoot biomass, fruit biomass and shoot:root ratio but increased root biomass between the first and second harvests. For shoot biomass, the full model was highly significant, explaining around 90% of the variation in the data (F5,60 = 121.8, R2adj = 0.90, P < 0.0001). There was a highly significant effect of the presence of barley (F1,60 = 35.3, p < 0.0001) and harvest (F2,60 = 274.9, p < 0.0001) and a significant interaction between the two (F2,60 = 12.1, p < 0.0001; ). There was no effect of barley at harvest one (P = 0.79), but a significant effect at harvests two (P = 0.002) and three (P < 0.0001). For root biomass, the full model was highly significant, explaining around three quarters of the variation in the data (F3,40 = 38.8, R2adj = 0.73, P < 0.0001). There was a significant effect of the presence of barley (F1,40 = 11.1, P = 0.002), a highly significant effect of harvest (F1,40 = 101.0, P < 0.0001) and a marginally significant interaction between the two (F1,40 = 4.2, P = 0.05; ). There was no effect of barley at harvest one (P = 0.35), but a significant effect at harvest two (P = 0.002). For shoot:root ratio, the full model was highly significant, explaining over half of the variation in the data (F3,40 = 21.13, R2adj = 0.58, P < 0.0001). There was a significant effect of the presence of barley (F1,40 = 38.3, P < 0.001), no effect of harvest (F1,40 = 0.10, P = 0.75) but a significant interaction between the two (F1,40 = 24.9, P < 0.0001; ). There was no effect of barley at harvest one (P = 0.46), but a highly significant effect at harvest two (P < 0.0001). For fruit biomass, the full model was highly significant, explaining over 60% of the variation in the data (F1,20 = 40.0, R2adj = 0.65, P < 0.0001; ). The presence of barley reduced the mean fruit biomass of C. cyanus by 81% equating to a Hedges d effect size of 2.8.
Figure 3. Shoot biomass (a), root biomass (b), shoot:root ratio (c) and fruit (inflorescence) biomass (d) of Centaurea cyanus at harvest 1 (42 days after sowing – square), harvest 2 (92 days after sowing – circle) and harvest 3 (162 days after sowing – triangle) when grown in the presence or absence of barley (cultivar Odyssey) in a glasshouse experiment. Barley had a significant negative effect on shoot biomass, fruit (inflorescence) biomass and shoot:root ratio as the growing season progressed and a significant positive effect on root biomass. Means of untransformed data (n = 11) are presented and error bars are 95% confidence intervals
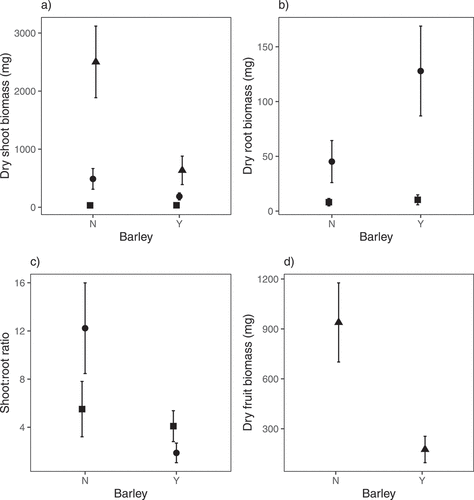
Scandix pecten-veneris
Presence of barley decreased shoot biomass and fruit biomass but increased root biomass between the first and second harvests. For shoot biomass, the full model was highly significant, explaining over 90% of the variation in the data (F5,60 = 146.6, R2adj = 0.92, P < 0.0001). There was a significant effect of the presence of barley (F1,60 = 8.1, P = 0.006), a highly significant effect of harvest (F2,60 = 347.2, P < 0.0001) and a highly significant interaction between the two (F2,60 = 15.1, P < 0.0001; ). There was a marginally significant effect of barley at harvest one (P = 0.03), with the presence of barley decreasing shoot biomass, and at harvest two (P = 0.03), where the barley led to an increase in S. pecten-veneris shoot biomass. At harvest three, barley presence had a significant negative effect on shoot biomass (P = 0.0001). For root biomass, the full model was highly significant, explaining over 80% of the variation in the data (F3,40 = 80.4, R2adj = 0.85, P < 0.0001). There was a marginally significant effect of the presence of barley (F1,40 = 3.4, P = 0.07), a highly significant effect of harvest (F1,40 = 230.6, P < 0.0001) and a significant interaction between the two (F1,40 = 7.1, P = 0.01; ). There was no effect of barley at harvest one (P = 0.35), but a significant effect at harvest two (P = 0.003). For shoot:root ratio, the full model was highly significant, but only explained around 40% of the variation in the data (F3,40 = 10.13, R2adj = 0.39, P < 0.0001). There was no significant effect of the presence of barley (F1,40 = 2.7, P = 0.1), a highly significant effect of harvest (F1,40 = 6.9, P < 0.0001) and no significant interaction between the two (F1,40 = 0.7, P = 0.42; ). For fruit biomass, the full model was highly significant, explaining around 70% of the variation in the data (F1,20 = 49.8, R2adj = 0.70, P < 0.0001; ). The presence of barley reduced mean fruit biomass of S. pecten-veneris by 66%, equating to a Hedges d effect size of 3.0.
Figure 4. Shoot biomass (a), root biomass (b), shoot:root ratio (c) and fruit (inflorescence) biomass (d) of Scandix pecten-veneris at harvest 1 (42 days after sowing – square), harvest 2 (92 days after sowing – circle) and harvest 3 (162 days after sowing – triangle) when grown in the presence or absence of barley (cultivar Odyssey) in a glasshouse experiment. Barley had a significant negative effect on shoot biomass and fruit (inflorescence) biomass as the growing season progressed and a significant positive effect on root biomass. Means of untransformed data (n = 11) are presented and error bars are 95% confidence intervals
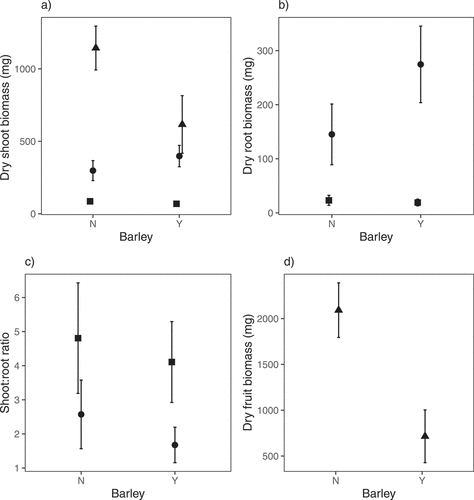
Discussion
Germination effects
Of the 16 arable wildflowers screened, three did not germinate at all, all seeds germinated for one species and the presence of barley only had marginally significant effects on the germination of two species. These two showed divergent responses with germination decreasing for C. cyanus and increasing for S. pecten-veneris in the presence of barley, which could be related to the relative timing of germination of each species. Centaurea cyanus germinated around the same time as the barley, while the majority of S. pecten-veneris seeds germinated once barley was already growing. The positive effects of barley on S. pecten-veneris germination could be due to favourable changes in microclimate caused by barley growth. In a previous study, Brooker et al. (Citation2018) have found positive effects of barley on the number of seeds germinated for the rare arable plant Valerianella rimosa Bastard and that this co-occurred with a decrease in soil moisture levels.
Effects of wildflowers on barley
We found no evidence for a facilitative effect of either C. cyanus or S. pecten-veneris on barley as above-ground biomass and fruit production were not enhanced. However, we also did not find evidence that either wildflower species were strong competitors, as there was no significant negative impact of their presence on barley above-ground biomass or fruit production. It is worth noting, however, that mean fruit biomass was reduced by 9% when barley was grown with either wildflower species and that variation in the data set was large. This level of biomass reduction falls within the levels of annual yield fluctuation for barley grown in the UK 2016–2020 (DEFRA Citation2020), but the experiment would need repeating under a variety of conditions and in the field to confirm the lack of significant effect as a universal response. Previous field-based experiments have shown C. cyanus to have a non-significant impact on spring wheat (Triticum aestivum) yield, but a significant negative impact on winter wheat yield when grown as a companion plant (Germeier Citation2000). There were some subtle, potentially positive effects of the wildflowers as barley shoot:root ratio increased at the second harvest compared to the first. This response suggests the promotion of investment in shoot material, and a lack of nutrient limitation at this time (Poorter and Nagel Citation2000), although it could also indicate that the barley experienced stronger below-ground competition than above-ground competition. The effect on shoot:root ratio was greatest with S. pecten-veneris.
Effects of barley on wildflowers
The initial positive effects of barley on S. pecten-veneris were lost as the plants completed their lifecycle, and by the end of the experiment the presence of barley had significant negative impacts on above-ground vegetative and fruiting biomass for both wildflower species. This finding is reflected in the responses previously documented for V. rimosa, where early increases in the abundance of individuals did not translate to increases in final productivity (Brooker et al. Citation2018). It also mirrors responses of Sinapis arvensis L. to barley root exudates, which although having no effect on germination, did inhibit later plant growth (Baghestani et al. Citation1999)
For the arable wildflowers, root biomass generally increased when grown with barley, suggesting that these species were experiencing some nutrient stress (Poorter and Nagel Citation2000) and that they were not growing under optimal conditions. However, both wildflowers we tested did go on to produce some seed, and in the context of conserving rare arable wildflowers, even if only a few individuals are able to produce seed, there is potential for them to continue surviving in arable landscapes.
Experimental considerations
We used above- and below-ground biomass and biomass of fruiting parts as an indicator of competitive or facilitative interactions between the arable wildflowers and barley. There are limitations to the usefulness of snapshot biomass measures as a way of assessing competition because the dynamics of interactions among plants change with time (Schofield et al. Citation2018, Citation2019). However, we were able to obtain some information on the complexity of coexistence dynamics by undertaking three harvests throughout the growing season. This enabled us to see changes in interaction strength and suggested that there may be some interesting temporal dynamics in the relationship between barley and S. pecten-veneris with the strength and direction of interactions changing over time.
We used a single cultivar of barley (Odyssey) for this study. As cultivar effects for barley and other crop species are well documented (Zytynska et al. Citation2010; Rowntree et al. Citation2011), this does not allow us to generalise our findings to barley or other cereal crops more broadly. However, Odyssey is a spring malting variety that has been widely used throughout the country, and was a recommended variety for many years until 2018. It remains a useful variety in pedigree development (AHDB (Agriculture and Horticulture Development Board) Citation2018) making it a good general cultivar for this experiment.
Inclusion of wildflowers in living mulches
This study was conducted a single time and in a greenhouse. Both the lack of replication and the context on which the experiment was undertaken, make it difficult to generalise findings more widely and specifically into the field (Limpens et al. Citation2012). Although more studies are needed to verify the findings here, the low impact on barley and promotion of investment in barley above-ground biomass suggest that both wildflower species tested could potentially be used in living mulches with this crop. In addition, the greatly reduced fruiting biomass for the wildflowers reduces the likelihood that these species will present a future problem as weeds.
Previous work on C. cyanus, provides evidence of additional benefits that this species might bring to arable fields, as it is attractive to pollinators (particularly hoverflies) and decomposing fly species (Rjg et al. Citation2012) and has been shown to increase parasitism rates of cabbage moths (Mamestra brassicae) in cabbage fields (Géneau et al. Citation2012; Balmer et al. Citation2013). However, in contrast to this study, it has also been shown to compete for nutrients and reduce crop biomass when planted as a living mulch alongside winter wheat crops in small field plots (Germeier Citation2000, Citation2006) and increase the occurrence of Thysanoptera caught in the field, many of which are plant pests (Rjg et al. Citation2012). Previous work on S. pecten-veneris illustrates its potential as a high-value intercrop, as it is edible (Psaroudaki et al. Citation2015) with potential health benefits (Sharifi-Rad et al. Citation2016). Extracts of the plant have also been shown to have antimicrobial properties (Sharifi-Rad et al. Citation2016; Wahab et al. Citation2018) which, as well as being useful in medicinal products, could have beneficial effects on the soil, in terms of controlling pathogenic bacteria, although they might also impact the growth of beneficial bacteria.
Conclusions
Interest in living mulches is increasing and although some mixtures are commercially available, very few, if any, focus on utilising arable wildflowers. There is real potential to expand the diversity of species used as living mulches and focusing on declining arable wildflower species could provide a counterbalance to the historical negative impact of many modern agricultural practices on some previously common species that have become rare (Byfield and Wilson Citation2005). Previous research has shown that plants can adapt to growing in mixtures and that mixtures of species have the potential to outperform monocultures (Schöb et al. Citation2018). By better understanding species interactions among plants and incorporating ecological knowledge into agronomy, it may be possible to preserve or boost yields, whilst increasing levels of plant diversity in the field (Brooker et al. Citation2015). Increases in plant diversity also have the potential to bring increases in the diversity of associated communities (Schuldt et al. Citation2019). With careful management, these wins for biodiversity need not necessarily be traded off against yield or grain nutrient value (Germeier Citation2000) and could bring additional benefits (Brooker Citation2017). Here we show in a greenhouse system that two increasingly rare arable wildflower species do not significantly negatively impact on barley fruit production and have the potential for inclusion in living mulch mixtures. We suggest that there is scope for a far wider screening of plants for use in living mulches and for testing their efficacy in large-scale field settings.
Boughton_Kettering_Loam.pdf
Download PDF (25.4 KB)Acknowledgements
RWB’s contribution was funded by the Scottish Government’s Rural and Environmental Science and Analytical Services Division through the Strategic Research Programme. Experimental work formed part of FM’s BSc research project. Thanks to Openfield Agriculture, Grantham, UK for donation of barley (cul. Odyssey) seeds.
Disclosure Statement
No potential conflict of interest was reported by the author(s).
Data availability statement
Data and scripts for analysis are available through Dryad digital repository DOI https://doi.org/10.5061/dryad.6t1g1jwvk
Supplementary material
Supplemental data for this article can be accessed here
Additional information
Funding
Notes on contributors
Jennifer K. Rowntree
Jennifer K. Rowntree (https://orcid.org/0000-0001-8249-8057) is a plant ecologist with a long-standing interest in species interactions and how we can use our understanding of this to enhance biodiversity conservation in agricultural settings.
Clare Dean
Clare Dean (https://orcid.org/0000-0003-0493-1796) studied invasive plant ecology during her Ph.D. during which she investigated the ecological mechanisms underpinning the ability of Crassula helmsii to dominate the vegetation in some drawdown zone communities.
Freddie Morrison
Freddie Morrison is an ecology student interested in plant – soil and weed interactions. He is keen to aid the development of improved practices for the future of biodiversity in agriculture.
Rob W. Brooker
Rob W. Brooker (https://orcid.org/0000-0002-7014-0071) is a plant ecologist with a particular interest in plant-plant interactions and their role in regulating community composition and ecosystem function.
Elizabeth A. C. Price
Elizabeth A.C. Price (https://orcid.org/0000-0002-5723-7856) is a plant ecologist with an interest in mechanisms to explain plant responses to spatial and temporal change and the implications for community composition, with applications in vegetation responses to pollution, and to the management, creation and restoration of habitats.
References
- AHDB (Agriculture and Horticulture Development Board). 2018. AHDB recommended list.
- Baghestani A, Lemieux C, Leroux GD, Baziramakenga R, Simard RR. 1999. Determination of allelochemicals in spring cereal cultivars of different competitiveness. Weed Sci. 47:498–504. doi:https://doi.org/10.1017/S0043174500092171.
- Balmer O, Pfiffner L, Schied J, Willareth M, Leimgruber A, Luka H, Traugott M. 2013. Noncrop flowering plants restore top-down herbivore control in agricultural fields. Ecol Evol. 3:2634–2646. doi:https://doi.org/10.1002/ece3.658.
- Baresel JP, Nichols P, Charrois A, Schmidhalter U. 2018. Adaptation of ecotypes and cultivars of subterranean clover (Trifolium subterraneum L.) to German environmental conditions and its suitability as living mulch. Genet Resour Crop Evol. 65:2057–2068. doi:https://doi.org/10.1007/s10722-018-0672-z.
- Brooker RW. 2017. Nature’s role in feeding the 10 billion: how biodiversity can benefit agriculture. In: Gordon I, Prins H, Squire G, editors. Food prod nat conserv conflicts solut. Abingdon and New York: Routlegde - Taylor and Francis; p. 238–258.
- Brooker RW, Bennett AE, Cong W, Daniell TJ, George TS, Hallett PD, Hawes C, Iannetta PPM, Jones HG, Karley AJ, et al. 2015. Improving intercropping : a synthesis of research in agronomy, plant physiology and ecology. New Phytol. 206(1):107–117. doi:https://doi.org/10.1111/nph.13132
- Brooker RW, Karley AJ, Morcillo L, Newton AC, Pakeman RJ, Schöb C. 2018. Crop presence, but not genetic diversity, impacts on the rare arable plant Valerianella rimosa. Plant Ecol Divers. 10:495–507. doi:https://doi.org/10.1080/17550874.2018.1437646.
- Brooker RW, Karley AJ, Newton AC, Pakeman RJ, Schöb C. 2016. Facilitation and sustainable agriculture : a mechanistic approach to reconciling crop production and conservation. Funct Ecol. 30:98–107. doi:https://doi.org/10.1111/1365-2435.12496.
- Brooker RW, Maestre FT, Callaway RM, Lortie CL, Cavieres LA, Kunstler G, Liancourt P, Tielbörger K, Travis JMJ, Anthelme F, et al. 2008. Facilitation in plant communities : the past, the present, and the future. J Ecol. 96:18–34.
- Byfield A, Wilson PJ. 2005. Important Arable plant areas: identifying priority sites for arable plant conservation in the United Kingdom. Salisbury (UK): Plantlife International.
- Cardinale BJ, Duffy JE, Gonzalez A, Hooper DU, Perrings C, Venail P, Narwani A, MacE GM, Tilman D, Wardle DA, et al. 2012. Biodiversity loss and its impact on humanity. Nature. 486(7401):59–67. doi:https://doi.org/10.1038/nature11148
- Ceballos G, Ehrlich PR, Dirzo R. 2017. Biological annihilation via the ongoing sixth mass extinction signaled by vertebrate population losses and declines. Proc Natl Acad Sci. 114(30):E6089–E6096. doi:https://doi.org/10.1073/pnas.1704949114.
- Core Team R. 2019. R: a language and environment for statistical computing. Available from: https://www.r-project.org/
- DEFRA. 2018. Wild bird populations in the UK, 1970-2017. Department for Environment, Food and Rural Affairs, UK Government, London, UK.
- DEFRA. 2020. Farming statistics – provisional arable crop areas, yields and livestock populations at June 2020 United Kingdom. Department for Environment, Food and Rural Affairs, UK Government, London, UK.
- Den Hollander NG, Bastiaans L, Kropff MJ. 2007. Clover as a cover crop for weed suppression in an intercropping design. II. Competitive ability of several clover species. Eur J Agron. 26:104–112. doi:https://doi.org/10.1016/j.eja.2006.08.005.
- Géneau CE, Wäckers FL, Luka H, Daniel C, Balmer O. 2012. Selective flowers to enhance biological control of cabbage pests by parasitoids. Basic Appl Ecol. 13:85–93. doi:https://doi.org/10.1016/j.baae.2011.10.005.
- George D, Banfield-Zanin J, Wäckers F 2015. Flower power : making the most of flowering seed mixes - Factsheet 08/15.
- Germeier CU. 2000. Wide row spacing and living mulch: new strategies for producing high protein grains in organic cereal production. Biol Agric Hortic. 18:127–139. doi:https://doi.org/10.1080/01448765.2000.9754875.
- Germeier CU. 2006. Competitive and soil fertility effects of forbs and legumes as companion plants or living mulch in wide spaced organically grown cereals. Biol Agric Hortic. 23:325–350. doi:https://doi.org/10.1080/01448765.2006.9755335.
- Hallmann CA, Sorg M, Jongejans E, Siepel H, Hofland N, Schwan H, Stenmans W, Müller A, Sumser H, Hörren T, et al. 2017. More than 75 percent decline over 27 years in total flying insect biomass in protected areas. PLoS One. 12:e0185809. doi:https://doi.org/10.1371/journal.pone.0185809.
- Hartwig NL, Ammon HU. 2002. Cover crops and living mulches. Weed Sci. 50:688–699. doi:https://doi.org/10.1614/0043-1745(2002)050[0688:AIACCA]2.0.CO;2.
- Hiltbrunner J, Liedgens M, Bloch L, Stamp P, Streit B. 2007. Legume cover crops as living mulches for winter wheat: components of biomass and the control of weeds. Eur J Agron. 26:21–29. doi:https://doi.org/10.1016/j.eja.2006.08.002.
- Iannetta PPM, Young M, Bachinger J, Bergkvist G, Doltra J, Lopez-Bellido RJ, Monti M, Pappa VA, Reckling M, Topp CFE, et al. 2016. A comparative nitrogen balance and productivity analysis of legume and non-legume supported cropping systems: the potential role of biological nitrogen fixation. Front Plant Sci. 7:1700. doi:https://doi.org/10.3389/fpls.2016.01700.
- Izumi Y, Okaichi S, Awala SK, Kawato Y, Watanabe Y, Yamane K, Iijima M. 2018. Water supply from pearl millet by hydraulic lift can mitigate drought stress and improve productivity of rice by the close mixed planting. Plant Prod Sci. 21:8–15. doi:https://doi.org/10.1080/1343943X.2018.1428494.
- Kew RBG. 2016. State of the world’s plants report - 2016. Kew: Royal Botanic Gardens.
- Limpens J, Granath G, Aerts R, Heijmans MMPD, Sheppard LJ, Bragazza L, Williams BL, Rydin H, Bubier J, Moore T, et al. 2012. Glasshouse vs field experiments: do they yield ecologically similar results for assessing N impacts on peat mosses? New Phytol. 195:408–418. doi:https://doi.org/10.1111/j.1469-8137.2012.04157.x.
- Matson PA, Parton WJ, Power AG, Swift MJ. 1997. Agricultural intensification and ecosystem properties. Science. 277:504–509. doi:https://doi.org/10.1126/science.277.5325.504.
- Poorter H, Nagel O. 2000. The role of biomass allocation in the growth response of plants to different levels of light, CO2, nutrients and water: a quantitative review. Aust J Plant Physiol. 27:595–607.
- Potts SG, Biesmeijer JC, Kremen C, Neumann P, Schweiger O, Kunin WE. 2010. Global pollinator declines: trends, impacts and drivers. Trends Ecol Evol. 25:345–353. doi:https://doi.org/10.1016/j.tree.2010.01.007.
- Psaroudaki A, Nikoloudakis N, Skaracis G, Katsiotis A. 2015. Genetic structure and population diversity of eleven edible herbs of Eastern Crete. J Biol Res. 22:7.
- Rjg C, George DR, Wäckers FL. 2012. Selection of floral resources to optimise conservation of agriculturally-functional insect groups. J Insect Conserv. 16:635–640. doi:https://doi.org/10.1007/s10841-012-9508-x.
- Rosenberg MS, Rothstein HR, Gurevitch J. 2013. Effect sizes: conventional choices and calculations. In: Koricheva J, Gurevitch J, Mengersen K, editors. Handbook of meta-analyses in ecology and evolution. Princeton and Oxford: Princeton University Press; p. 61–71.
- Rowntree JK, Cameron DD, Preziosi RF. 2011. Genetic variation changes the interactions between the parasitic plant-ecosystem engineer Rhinanthus and its hosts. Philos Trans R Soc B Biol Sci. 366:1380–1388. doi:https://doi.org/10.1098/rstb.2010.0320.
- Sands RRJ, Rowntree JKJK. 2016. Interactions between the bumblebee Bombus pascuorum and red clover (Trifolium pratense) are mediated by plant genetic background. PLoS One. 11:e0161327. doi:https://doi.org/10.1371/journal.pone.0161327.
- Scheublin TR, Ridgway KP, Young JPW, Van Der Heijden MGA. 2004. Nonlegumes, legumes, and root nodules harbor different arbuscular mycorrhizal fungal communities. Appl Environ Microbiol. 70:6240–6246. doi:https://doi.org/10.1128/AEM.70.10.6240-6246.2004.
- Schöb C, Armas C, Guler M, Prieto I, Pugnaire FI. 2013. Variability in functional traits mediates plant interactions along stress gradients. J Ecol. 101:753–762. doi:https://doi.org/10.1111/1365-2745.12062.
- Schöb C, Brooker RW, Zuppinger-Dingley D. 2018. Evolution of facilitation requires diverse communities. Nat Ecol Evol. 2:1381–1385. doi:https://doi.org/10.1038/s41559-018-0623-2.
- Schofield EJ, Rowntree JK, Paterson E, Brewer MJ, Price EAC, Brearley FQ, Brooker RW. 2019. Cultivar differences and impact of plant-plant competition on temporal patterns of nitrogen and biomass accumulation. Front Plant Sci. 10:215. doi:https://doi.org/10.3389/fpls.2019.00215.
- Schofield EJ, Rowntree JK, Paterson E, Brooker RW, Scho EJ, Rowntree JK, Paterson E, Brooker RW. 2018. Temporal dynamism of resource capture: a missing factor in ecology? Trends Ecol Evol. 33:277–286. doi:https://doi.org/10.1016/j.tree.2018.01.009.
- Schuldt A, Ebeling A, Kunz M, Staab M, Guimarães-Steinicke C, Bachmann D, Buchmann N, Durka W, Fichtner A, Fornoff F, et al. 2019. Multiple plant diversity components drive consumer communities across ecosystems. Nat Commun. 10(1):10. doi:https://doi.org/10.1038/s41467-019-09448-8
- Sharifi-Rad M, Tayeboon GS, Miri A, Sharifi-Rad M, Setzer WN, Fallah F, Kuhestani K, Tahanzadeh N, Sharifi-Rad J. 2016. Mutagenic, antimutagenic, antioxidant, anti-lipoxygenase and antimicrobial activities of Scandix pecten-veneris L. Cell Mol Biol. 62:8–16.
- Thorsted MD, Olesen JE, Weiner J. 2006. Width of clover strips and wheat rows influence grain yield in winter wheat/white clover intercropping. F Crop Res. 95:280–290. doi:https://doi.org/10.1016/j.fcr.2005.04.001.
- Vincent C, Schaffer B, Rowland DL, Migliaccio KW, Crane J, Li Y. 2017. Sunn hemp intercrop and mulch increases papaya growth and reduces wind speed and virus damage. Sci Hort. 218:304–315. doi:https://doi.org/10.1016/j.scienta.2017.02.042.
- Wahab A, Jan SA, Rauf A, Rehman Z, Khan Z, Ahmed A, Syed F, Safi SZ, Khan H, Imran M. 2018. Phytochemical composition, biological potential and enzyme inhibition activity of Scandix pecten-veneris L. J Zhejiang Univ B (Biomedicine Biotechnol). 19:120–129. doi:https://doi.org/10.1631/jzus.B1600443.
- Willis KJ, editor. 2017. State of the world’s plants 2017. Report. Royal Botanic Gardens, Kew.
- Wilson P, King M. 2003. Arable plants - a field guide. Old basing. Hampshire (UK): English Nature and WildGuildes.
- Yin W, Chai Q, Zhao C, Yu A, Fan Z, Hu F, Fan H, Guo Y, Coulter JA. 2020. Water utilization in intercropping: a review. Agricult Water Manag. 241:106335. doi:https://doi.org/10.1016/j.agwat.2020.106335.
- Zytynska SE, Fleming S, Tetard-Jones C, Kertesz MA, Preziosi RF. 2010. Community genetic interactions mediate indirect ecological effects between a parasitoid wasp and rhizobacteria. Ecology. 91:1563–1568. doi:https://doi.org/10.1890/09-2070.1.