Abstract
Conventionally, peripheral nerve stimulation (PNS) for treatment of chronic pain has involved a two-stage process: a short-term (e.g., 7 days) trial and, if significant pain relief is achieved, a permanent PNS system is implanted. A percutaneous PNS treatment is now available where a coiled lead may be implanted for up to 60 days with the goal of producing sustained relief. In the present review, published prospective trials using percutaneous PNS treatment were identified and synthesized. The collected evidence indicates that percutaneous PNS treatment for up to 60 days provides durable clinically significant improvements in pain and pain interference. Similar efficacy across diverse targets and etiologies supports the broad applicability for use within the chronic pain population using this nonopioid technology.
Plain language summary
What is this review about?
This review looks at a drug-free way to treat chronic pain called percutaneous peripheral nerve stimulation (PNS). Percutaneous means it is placed through the skin. PNS applies small amounts of electricity to the nerves to reduce chronic pain. Most PNS systems involve a two-step process. A short trial is first performed to see if a patient has pain relief. A permanent system is then placed if the person had pain relief. Percutaneous PNS treatments are different. They use a thin wire called a lead placed in the body for up to 60 days. The lead is taken out at the end of the treatment period. Studies have shown that this type of PNS treatment can reduce chronic pain even after the treatment is over. No previous article has collected all these studies of percutaneous PNS in one place.
What evidence was gathered?
This review found evidence from studies on treatment of chronic pain. Pain types included shoulder pain, neuropathic pain and low back pain. It found that percutaneous PNS treatment for up to 60 days can reduce pain and how pain interferes with daily life.
How can these data lead to better care for patients?
These findings mean that percutaneous PNS treatments could be a useful, non-drug option for many types of chronic pain.
GRAPHICAL ABSTRACT
Chronic pain exacts a high toll on quality of life, function and productivity for millions of Americans with a rising incidence rate.
Percutaneous peripheral nerve stimulation is a US FDA-cleared treatment that can be implanted for up to 60 days.
Data from prospective clinical studies have repeatedly demonstrated durable pain relief across diverse pain conditions and a robust safety profile.
Researchers employed stimulation strategies targeting efferent motor fibers and/or afferent sensory fibers in peripheral nerves innervating the region of pain.
A participant-level data analysis revealed comparable relief from pain and pain interference across studies for up to 14 months, suggesting a convergent mechanism of action across pain etiologies.
The collected evidence supports the use of percutaneous peripheral nerve stimulation as a nonopioid, non-neurodestructive treatment option for chronic pain patients.
1. Background
Chronic pain (pain lasting longer than 3 months) exacts an enormous toll on individuals [Citation1] including reduced quality of life [Citation2] and productivity loss [Citation3]. On average, adults with chronic pain lose 16 productivity days/year [Citation4] and have increased healthcare costs associated with treatment compared with individuals without chronic pain [Citation5,Citation6]. Left untreated, chronic pain continues to negatively affect sleep, independence and function [Citation7]. The Center for Disease Control estimates over 50 million Americans will experience a substantial negative influence on their well-being and productivity from chronic pain annually [Citation8] and the rising incidence rate of chronic pain among Americans contributes to a growing economic burden with estimated annual direct and indirect costs totaling as high as $600 billion nationally [Citation6,Citation9].
Devices that interface with the nervous system have significant potential to relieve chronic pain. Application of electric current to neural tissue, or neurostimulation, can alter pain signal processes and has been used successfully to treat acute and chronic pain across multiple etiologies [Citation10]. Neurostimulation can be applied at several levels of sensory or motor processing including the brain, spinal cord and peripheral nerves. The latter is especially promising as peripheral nerve stimulation (PNS) devices may produce focal pain relief with higher precision compared with other neuromodulation modalities (e.g., spinal cord stimulation [SCS] and dorsal root ganglion [DRG] stimulation) and some approaches may offer enduring effects without a permanently implanted system [Citation11]. Additionally, PNS may be an appropriate treatment option in patients who are not optimal candidates for SCS or DRG stimulation, including patients with complex spinal anatomy or those on anticoagulant therapy [Citation12,Citation13]. With the evolution of neuromodulation technology, a paradigm shift has occurred in interventional pain management, and stimulation is frequently employed much earlier in the treatment algorithm for a range of conditions including back pain, shoulder pain, knee pain, post-amputation pain and more [Citation14].
Contemporary PNS device designs can be permanently or temporarily implanted. Several permanently implanted PNS systems are currently available that apply stimulation to a nerve (or group of nerves) via permanently implanted leads with an internal or external pulse generator or receiver. Before the implantation of a permanent system, physicians will typically temporarily implant leads to ascertain whether the patient experiences significant pain reduction before recommending implantation of a permanent system. Historically, percutaneous leads have been temporarily implanted to allow PNS to be experienced for approximately 7 days. In contrast, one US FDA-cleared system (SPRINT® PNS System, SPR Therapeutics; OH, USA) uses temporarily implanted leads designed to remain indwelling and delivering PNS for an extended treatment period of up to 60 days with intent to provide pain relief during the treatment period that may endure following lead removal. A proposed mechanism for this sustained effect involves ‘reconditioning’ of the centralized pain state [Citation15]. When pain relief is significant, but not durable, an extended temporary period of stimulation may also be helpful in identifying delayed responders who may benefit from the subsequent implantation of a PNS system US FDA cleared to remain indwelling permanently [Citation16].
While several reviews of clinical PNS studies have been published in recent years [Citation17–22], no review has provided a summary of evidence for percutaneous PNS treatment with temporarily implanted leads (i.e., SPRINT PNS and earlier related studies). Therefore, the present review synthesizes findings from prospective clinical trials using percutaneous PNS leads implanted for up to 60 days to treat chronic pain.
2. Methods
2.1. Literature review
The objective of this study was to review prospective clinical trials using percutaneously implanted PNS leads to treat chronic pain that remained indwelling and externalized for up to 60 days prior to their withdrawal. A literature search was conducted to verify relevant studies. Search criteria were defined to identify prospective clinical trials investigating the efficacy of percutaneous PNS treatments in managing chronic pain. Percutaneous PNS treatments for acute pain have been reviewed elsewhere [Citation23–25]. Specific search terms were developed for PubMed, Web of Science and Scopus [Citation26]. EMBASE was considered but not accessible, and other tools were combined to maximize search coverage and minimize the risk of search bias [Citation26,Citation27]. Key phrases included ‘peripheral nerve stimulation’, ‘neuromuscular stimulation’ and ‘pain’ (search terms defined in Supplementary Materials: Literature Review Search Terms). The review excluded studies investigating permanently implanted devices or devices intended for permanent implantation, studies investigating devices used only during clinic visits or surface stimulation approaches (e.g., transcutaneous electrical nerve stimulation [TENS]), retrospective studies, case reports and studies where the primary therapeutic outcome was unrelated to pain. Duplicates, reviews, non-peer-reviewed publications (e.g., conference abstracts) and studies on acute disease and pain states were also excluded.
Study characteristics such as design, participant numbers for PNS treatment groups and control groups (if applicable), treatment descriptions and outcomes (e.g., pain intensity, pain interference, disability, etc.) were extracted from eligible publications. Safety data and adverse events were also summarized.
2.2. Participant-level data
To synthesize participant-level outcomes, data were extracted from study publications as available and outcomes were requested from authors for studies where participant-level outcomes were not provided in the publication. Commonly reported outcomes, such as worst pain, average pain and pain interference as quantified using the Brief Pain Inventory (BPI) questionnaire, were synthesized. Because of differing study designs and outcome measures, participant-level data were considered here as-observed and missing data are reported descriptively. Data were pooled for pain intensity (average pain as quantified by BPI Short Form Question 5 [BPISF-5] score), and for pain interference (as quantified by the BPISF Question 9 [BPISF-9] score). In the event average pain scores were not available, worst pain scores (as quantified by the BPISF Question 3 [BPISF-3] score) were included in the participant-level pooled data due to the demonstrated similarities in assay sensitivity, reliability and validity to detect therapeutic effects and quantify effect size across pain conditions [Citation28–30]. Data are summarized from the participant-level data for each study cohort, timepoint and outcome (pain intensity and pain interference). Data points from publications with shared study cohorts (e.g., a first publication to report primary end points at end-of-treatment and a second publication to report long-term follow-up end points) were not duplicated.
As the duration of treatment and follow-up timepoints were not uniform across all studies, data timepoints are presented as follows: end of treatment (EOT) is defined as the time of lead withdrawal regardless of treatment duration, follow-up outcome timepoints are standardized to reference from the implantation date (time from start of treatment), the treatment periods are defined as the time between lead implant and withdrawal, follow-up outcome timepoints are grouped across studies into the following epochs: 3–5 months, 6–8 months and 12–14 months.
Because studies also differed in definition of treatment response, a standardized composite responder definition (≥50% reduction in pain intensity and/or pain interference compared with baseline, which is considered a substantial change according to consensus recommendations [Citation31]) was applied to the participant-level data to calculate the responder rate for the pooled study cohorts. By considering pain and pain interference outcomes, the composite responder definition accounts for direct treatment effects on pain while also capturing indirect effects that are more indicative of the real-world benefits of pain treatments [Citation32–34]. The responder rate was calculated as the percentage of the pooled participants at each timepoint.
2.3. Data reporting
Continuous variable data are presented as mean ± standard error (SEM) unless specified otherwise. Binomial data are presented as proportions with 95% confidence interval.
3. Results
3.1. Literature review
Literature searches for all databases were performed through 10 November 2023, and 968 resulting publications were evaluated for inclusion. 112 publications were duplicates and 840 publications did not meet the inclusion criteria and were removed. After the literature search, 16 publications were eligible for inclusion in the present review (). The most common reasons for study exclusion included non-prospective study design and use of permanently implanted devices.
Figure 1. Flow chart for literature search. Publications that met the inclusion criteria are summarized by the publication count in parentheses and the number of unique study cohorts.
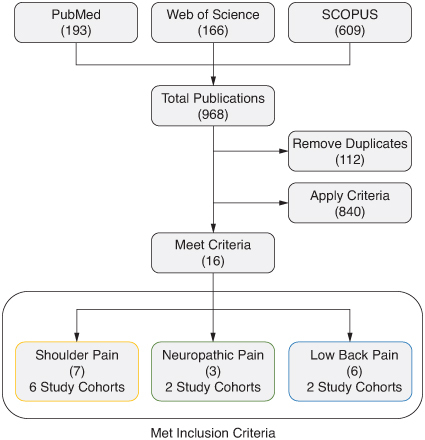
Eligible publications encompassed multiple prospective study designs (prospective case series and multi-center randomized controlled trials [RCT]) in three primary indications: shoulder pain, neuropathic pain and axial low back pain.
3.1.1. Shoulder pain
Six publications reported original data from prospective studies investigating percutaneous PNS treatment for shoulder pain [Citation35–40]. Five publications presented primary findings from five study cohorts (from EOT through a maximum of 7 months from the start of treatment), and one publication reported follow-up data from one cohort through 12 months [Citation37]. Studies varied by indication (e.g., hemiplegic shoulder pain and subacromial impingement syndrome) and design (prospective case series, single-center RCT and multicenter RCT).
A prospective clinical study was conducted to determine the feasibility of percutaneous PNS treatment to manage chronic hemiplegic shoulder pain in stroke patients [Citation41]. Yu and colleagues implanted percutaneous leads and stimulated the terminal branches of the axillary nerve and the suprascapular nerve to activate the posterior deltoid muscle and the supraspinatus muscles, respectively, to decrease shoulder pain and subluxation in participants with hemiplegic shoulder pain (n = 8, Study Cohort 1 in ). Two participants received an additional lead targeting the terminal branches of the spinal accessory nerve activating the upper trapezius muscle. Percutaneous PNS treatment was provided with constant frequency and amplitude and a 50% duty cycle (12 Hz, 20 mA, 10 s on-10 s off). Stimulation intensity was titrated by adjusting the pulse width (10–200 μs) to produce comfortable cycling stimulation which was used for 6 h/day. All participants received 6 weeks of percutaneous PNS treatment before the leads were removed. Shoulder biomechanics and mean pain (quantified using the BPI questionnaire) were measured before treatment, at EOT and at 3-month follow-up post-treatment. Participants reported reduced pain intensity (median 0/10) and pain interference (median 0/10) at EOT compared with pre-treatment baseline (5/10 median pain intensity and 1.15/10 median pain interference). The relief from pain intensity and pain interference was sustained at 3 months post-treatment (median score of 2/10 and 0.5/10, respectively).
Table 1. Review and synthesis of publications of prospective studies of percutaneous PNS treatment to manage chronic pain.
A single-arm case series study was performed by Renzenbrink and IJzerman to study the effectiveness of percutaneous PNS to treat hemiplegic shoulder pain [Citation42]. 15 participants with chronic hemiplegia and treatment-resistant shoulder pain (Study Cohort 2 in ) with baseline pain ≥4/10 received percutaneous PNS targeting the terminal branches of the axillary nerve, the suprascapular nerve and spinal accessory nerve terminals. The participants were instructed to apply stimulation (12 Hz, 20 mA, 10 s on-10 s off) for 6 h each day during the 6-week treatment period and reported pain intensity using the numeric rating scale (NRS; comparable to the BPI) between 0 (no pain) and 10 (worst pain imaginable). The cohort's mean pain intensity (± standard deviation) decreased significantly from baseline (7.2 ± 1.6) to EOT (1.5 ± 1.3) and participants reported sustained relief from shoulder pain at 18 weeks (1.3 ± 1.4) and 30 weeks (1.3 ± 1.4) following treatment onset.
These results encouraged Yu and colleagues to perform a multi-center, single-blinded, RCT using a four-lead system targeting the axillary nerve, suprascapular nerve and spinal nerve terminals (e.g., spinal accessory nerve and ventral rami of cervical spinal nerves) in the trapezius muscle [Citation36]. 32 participants with hemiplegic shoulder pain intensity ≥2/10 (Study Cohort 3 in ) received 6 weeks of percutaneous PNS treatment, and 29 participants received standard care (instructions to use a sling when affected shoulder was unsupported). Yu and colleagues recorded the worst pain (BPI Question 12 [BPI-12], equivalent to the BPISF-3) at baseline, EOT and follow-up timepoints of 3-months and 6-months post-treatment. Outcomes from the PNS treatment and control groups were compared using repeated-measure analysis of variance with post-hoc independent t-tests. The proportion of participants with a ≥3-point decrease in worst pain score in the PNS treatment group was significantly higher than the control group at EOT (66 and 24%, respectively; p < 0.01), 3 months post-treatment (59 and 21%, respectively; p < 0.01) and 6 months post-treatment (59 and 28%, respectively; p < 0.05). A 12-month follow-up intent-to-treat analysis [Citation37] reported pain relief was sustained for a year after lead removal for a higher proportion of participants who underwent percutaneous PNS treatment compared with the control group (mean ± standard deviation reduction in BPI-12 scores of 5.00 ± 3.30 and 2.31 ± 3.21, respectively; p < 0.001).
Chae and colleagues performed a prospective case series investigating the efficacy of a simplified approach with a single lead PNS treatment targeting the axillary nerve to treat hemiplegic shoulder pain [Citation38]. The lead was implanted for 3 weeks of active stimulation (12 Hz, 20 mA, pulse duration between 17–220 μs) following a 1-week post-implant stabilization period in participants experiencing hemiplegic shoulder pain ≥4/10 at baseline (n = 8, Study Cohort 4 in ). Worst pain (BPISF-3) and pain interference (BPISF-9) were recorded at baseline, EOT (4 weeks) and follow-up timepoints of 5, 8 and 16 weeks. Using an established definition of clinical significance [Citation43], all participants (100%, n = 8) reported a clinically significant (≥2-point) and statistically significant (p < 0.001) decrease in worst pain at EOT, 4 weeks post-treatment and 12-weeks post-treatment, reporting an average reduction of 70, 61 and 63% in worst pain intensity compared with baseline, respectively.
The case series was followed by a single center RCT [Citation39]. Wilson and colleagues compared pain reduction in participants with hemiplegic shoulder pain ≥4/10 at baseline who received 3 weeks of percutaneous PNS treatment following a 1-week stabilization period (n = 13, Study Cohort 5 in ) to participants who received usual care (4 weeks of physical therapy, n = 12). The study found greater decreases in reported worst pain (BPISF-3) at EOT when participants received percutaneous PNS treatment (mean worst pain score decreased 65% from baseline, from 7.5 to 2.6) than when participants received usual care (mean worst pain score decreased 34% from baseline, from 7.6 to 5.0). Sustained reduction in mean worst pain score was observed for participants in the PNS treatment group at 16 weeks post-treatment (decreased 60% from baseline, to 3.0), but not for the usual care group (p < 0.05).
Percutaneous PNS treatment has also been applied to participants with other types of chronic shoulder pain with musculoskeletal etiologies. In a prospective case series evaluating percutaneous PNS treatment for subacromial impingement syndrome, Wilson and colleagues enrolled ten participants (Study Cohort 6 in ) with pain intensity ≥4/10 at baseline who received 3 weeks of percutaneous PNS treatment following a 1-week post-implant stabilization period targeting the terminal branches of the axillary nerve [Citation40]. Key outcomes were pain intensity (BPISF-3) and pain interference (BPISF-9) measured at baseline, EOT (4 weeks) and follow-up timepoints at 5, 8 and 16 weeks. At EOT, participants reported statistically significant percent reductions in worst pain intensity (37% reduction compared with baseline; p < 0.01) and pain interference (52% reduction compared with baseline; p < 0.01). At the 16-week follow-up timepoint, participants reported sustained relief from worst pain intensity (49% reduction compared with baseline; p < 0.01) and in pain interference (58% reduction compared with baseline; p < 0.01).
3.1.2. Neuropathic pain
The literature review identified three publications reporting outcomes from prospective studies investigating percutaneous PNS treatment for neuropathic pain [Citation44,Citation51,Citation52], including long-term (12 months) follow-up data for one cohort [Citation52].
Following positive pain relief effects reported in a single-participant case report [Citation45], a prospective case series was performed by Rauck and colleagues treating chronic neuropathic pain in lower extremity amputees [Citation44]. Two percutaneous leads were placed to stimulate the femoral nerve and sciatic nerve in the residual lower limb in 16 participants with neuropathic pain that averaged ≥4/10 at baseline (Study Cohort 7 in ). 14 of 16 participants responded during the delivery of test stimulation and reported decreases in residual limb pain, phantom limb pain or both. Stimulation parameters were adjusted to produce continuously comfortable paresthesia coverage over the area of pain (frequency range of 50–100 Hz, pulse-width range of 10–40 μs and amplitude range of 1–20 mA). Of the nine participants who optionally completed a subsequent 2-week treatment period, a majority experienced clinically meaningful reductions in pain intensity (BPISF-3) compared with baseline (≥30%) at EOT (p < 0.005, n = 9) and at the 4-week follow-up (p < 0.005, n = 9). Compared with baseline, significant improvements in pain interference (BPISF-9) were also observed at the end of treatment (p < 0.05), including an 81% decrease in the six participants with baseline residual limb pain and an 83% decrease in the seven participants with baseline phantom limb pain.
A subsequent multicenter, double-blinded, randomized, placebo-controlled trial compared the efficacy of continuous (24 h per day) percutaneous PNS treatment to placebo (implanted leads that provided sham stimulation) for chronic neuropathic pain following amputation [Citation51]. Subjects had average pain ≥4/10 in at least one of the residual or phantom limb. The primary endpoint was defined as the proportion in each group experiencing highly clinically meaningful reductions (≥50%) in average pain (residual and/or phantom, BPISF-5) 4 weeks from start of treatment and outcomes were measured monthly up to 12 months from the start of treatment. Stimulation was applied at 100 Hz during treatment period, and after 4 weeks of treatment in the PNS treatment group and sham stimulation in the placebo group, 58%, (n = 12, Study Cohort 8 in ) of participants in the treatment group reported a ≥50% reduction in pain intensity (BPISF-5) compared with 14% (n = 14) in the placebo group (p = 0.037). The PNS treatment group continued with the percutaneous PNS treatment for a total of 60 days while the placebo group crossed over into treatment by applying therapeutic stimulation rather than sham stimulation. At 8 weeks, the proportion of participants in the PNS treatment group with ≥50% pain relief (67%, n = 12) continued to be significantly greater compared with the proportion in the placebo group at the end of the 4-week placebo period (14%, n = 14; p = 0.013). A follow-up analysis reported 67% of participants in the PNS treatment group (n = 9) continued to report ≥50% reduction in pain, and 56% (n = 9) also reported ≥50% reductions in pain interference through 12 months from start of treatment [Citation52].
3.1.3. Low back pain
Six publications on the use of percutaneous PNS treatment in managing chronic low back pain in two study cohorts met the inclusion criteria. The study cohorts consisted of a prospective single-center case series with outcomes through 13 months and a multicenter case series with follow-up through 14 months.
Following positive outcomes in a case report [Citation46], Gilmore and colleagues performed a prospective case series of percutaneous PNS treatment in the management of chronic axial low back pain [Citation47]. The investigators enrolled participants with chronic axial low back pain (≥4/10 average pain) in the lumbar region (n = 9, Study Cohort 9 in ), and leads were placed bilaterally to provide targeted stimulation of the medial branch of the dorsal ramus at the lumbar lamina in the anatomic center of the area of pain for 30 days. The intensity of stimulation was adjusted to produce comfortable cycling of the multifidus muscle (frequency of 12 Hz, pulse-width range of 15–200 μs and amplitude range of 5–20 mA). The authors recorded average pain (BPISF-5), worst pain (BPISF-3), pain interference (BPISF-9) and disability as measured by the Oswestry Disability Index (ODI). 67% (n = 9) experienced highly clinically meaningful (≥50%) reductions in reported pain intensity (BPISF-5) at EOT, and these responders reported an average of 80% reduction of pain intensity. Of the patients who completed the post-treatment follow-up 5 months from treatment onset, 80% reported sustained reductions (≥50%) in average pain and worst pain (n = 5). A follow-up publication of the same study cohort reported 60% of participants with available follow-up outcomes reported clinically meaningful (≥30%) reductions in pain intensity at 7 months from treatment onset (n = 5) [Citation48]. The same study cohort was followed out to 13 months [Citation49], and 67% of participants with follow-up available (n = 6; 3 participants were lost to follow-up) reported clinically meaningful (≥30%) reductions in pain intensity and/or decreases in disability (≥10-point reduction in ODI score). Further, 83% of participants reported improvements in quality of life during the long-term follow-up (≥1 point increase as quantified using the Patients' Global Impression of Change [PGIC] questionnaire) compared with baseline (n = 6).
Gilmore and colleagues performed a prospective multicenter study to investigate further the efficacy of percutaneous PNS treatment to manage chronic axial low back pain using a 60-day treatment duration in a larger cohort of 74 participants [Citation50]. Enrolled participants (Study Cohort 10 in ) had failed multiple conservative treatments (e.g., recalcitrant chronic pain), had average pain ≥4/10 at baseline and represented multiple low back pain etiologies including degenerative disc disease (32%) and lumbar spondylosis (37%). 73% of participants reported a clinically meaningful reduction in average pain (≥30%, BPISF-5) at EOT (n = 74). Among those responders, the mean percent reduction in average pain was 58%. Among all participants, the mean average pain score (± standard deviation) decreased from 6.1 ± 1.2 at baseline to 3.3 ± 1.9 at EOT. The authors observed sustained relief at 5-month (3.6 mean average pain score) and 8-month (3.9 mean average pain score) follow-ups. A follow-up publication reported pain outcomes at 14 months post-treatment [Citation53], in which 77% of participants (n = 62) experienced clinically meaningful reduction in pain intensity (≥30%), pain interference (≥30% reduction of BPISF-9 score) and/or disability (10-point reduction in ODI score) at the end of follow-up. There was no significant difference in response to PNS treatment by etiology. 75% of participants with baseline opioid use (n = 20) reduced or eliminated opioid consumption in the months following percutaneous PNS treatment. Participants who reported a reduction in opioid use also reported an 82% reduction in average opioid use from baseline (28.5 ± 6.5 mg morphine equivalent [MME]) to 14 months (5.1 ± 6.3 MME). Additionally, an analysis was performed on a subset of this study's participants who had previously undergone RFA of the medial branch of the spinal nerve [Citation54]. 93% of patients with a history of medial branch RFA (n = 15) experienced highly clinically meaningful reductions in pain intensity (≥50%, BPISF-5), pain interference (≥50%, BPISF-9), and/or disability (≥10 point reduction in ODI score) at EOT, with significant differences in average pain intensity from baseline to 2 and 5 months (6.3, 2.4 and 3.1 mean average pain intensity, respectively; p < 0.0001).
3.1.4. Safety outcomes
Cumulatively, there were no serious adverse events or unanticipated device-related complications reported across all studies that met the inclusion criteria for this review. The most commonly reported adverse event was redness or skin irritation at the bandage or lead exit site (all publications reported at least one incident of skin irritation). Other, less-common adverse events included new/worsening of pain from baseline and discomfort from the lead placement procedure. Two superficial skin infections were reported in one study cohort, one at a lead exit site and one under a bandage adhesive [Citation54], while the remaining study cohorts had no incidence of infection. In all cases, adverse events were reported as non-serious.
Device-related issues included lead dislodgement or migration during treatment, discomfort due to implantation or stimulation and lead fracture during treatment or explant. Reports of lead fracture varied in the reviewed studies. Overall, lead fracture with open-coil percutaneous leads in PNS systems for pain has historically been reported to occur in 6–8% of leads, predominately at the time of lead removal, though a markedly strengthened lead intended to reduce fracture rates was recently introduced [Citation55–57]. Lead fragments are magnetic resonance imaging conditional [Citation55], and studies reviewed here reported observing retained fragments in situ with no fragment-related sequelae or adverse effects during follow-up [Citation36,Citation39,Citation41,Citation51,Citation58].
3.2. Participant-level data
Follow-up timepoints were extracted from study data that were the closest available timepoint to 3, 6 and 12 months from percutaneous PNS treatment onset. Outcomes were reported at 3–5 months for nine study cohorts, while five studies continued to follow participants at 6–8 months and four studies at 12–14 months (). Participant-level data were made available for all studies. The summary of outcomes included multiple study cohorts at each timepoint (range: 4–10 pooled study cohorts, 108–187 participants, A–C). Across ten study cohorts at the end of treatment, 73% of participants (n = 187) had at least 50% reductions in pain intensity and/or pain interference at EOT, and 57% of participants with available data (n = 108) reported sustained improvements in pain and/or pain interference at the 12–14-month time point (57%, C).
Figure 2. Study outcomes timeline. Percutaneous PNS treatment period ranged from 14 to 60 days (green boxes). Reported outcome timepoints (triangles) were grouped as end-of-treatment, 3–5-month follow-up, 6–8-month follow-up and 12–14-month follow-up (months from therapy onset, orange boxes). Study cohort definitions and data sources are presented in .
EOT: End-of-treatment; PNS: Peripheral nerve stimulation.
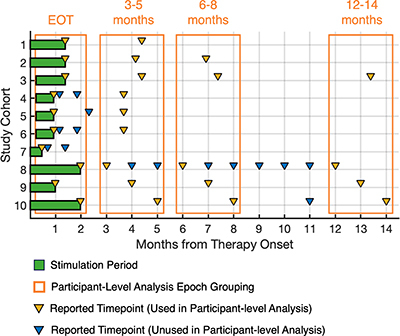
Figure 3. Summary data and composite responder analysis. (A) Mean pain intensity by study cohort. (B) Mean average pain interference scores by study cohort. Overall mean pain intensity and pain interference scores presented as dashed black lines in (A & B). Participant-level data presented as individual outcomes (points) and mean ± standard error (lines and error bars). (C) Summary of composite responder rate for each timepoint. Composite responder defined as a participant who experienced highly clinically meaningful reduction (≥50%) in pain intensity and/or pain interference. Responder rates presented as a percentage of total study participants with data available at each time point. (D) Composite responder rate for each study cohort at EOT, with the overall summary of responder rate at EOT (73%) represented by the black dotted line. Data presented as mean ± 95% confidence interval (bars and error bars). (E) Color legend for study cohorts. Data sources are presented in .
Avg: Average; BPI: Brief pain inventory; EOT: End-of-treatment; mo: Month; NA: Not applicable.
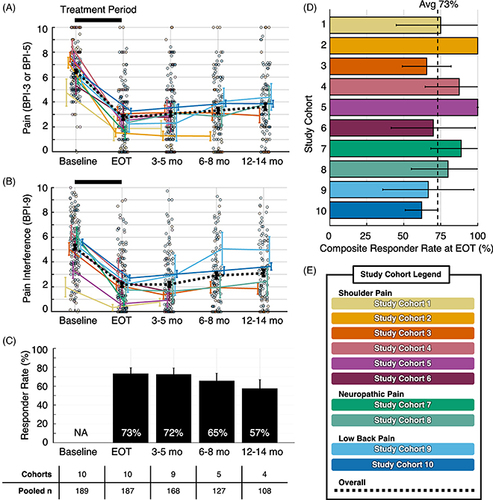
4. Discussion
The present review summarizes outcomes from prospective studies evaluating percutaneous PNS treatments for chronic pain, including pain relief and the interference of pain with function both during treatment (e.g., at EOT) and through follow-up ranging from 3 to 14 months after the start of treatment. The literature identified during the review ultimately included trials using a single FDA-cleared PNS System with temporarily implanted leads as well as prior related studies. Study designs included prospective case series and RCTs and studies included participants with various pain etiologies and indications including chronic shoulder pain, neuropathic pain and axial low back pain. While individual studies ranged in size and used different definitions of treatment success, pooled participant-level data showed that a majority of participants (73%) reported highly clinically meaningful (≥50%) improvements in pain and/or pain interference at EOT, and all studies reported sustained relief for a majority of patients throughout long-term follow-up ranging up to 14 months from the start of treatment. Treatment safety was assessed qualitatively from the publications, and studies reported no serious or unanticipated device-related adverse events.
The studies reviewed here demonstrate the use of a percutaneous PNS treatment to manage effectively chronic pain without requiring a permanently implanted system. Traditionally, brief (e.g., 7–10 days) trials have been employed to assess patient response to PNS prior to permanent PNS system implantation [Citation59,Citation60]. Conventional trial periods are limited in duration in large part due to the risk of infection when implanting conventional, noncoiled, cylindrical leads [Citation61,Citation62]. The studies reviewed here utilized variations of open-coil leads of significantly smaller diameter compared with conventional cylindrical leads. The open-coil, helical lead structure is thought to contribute to the reduced infection risk during implantation periods [Citation50,Citation51,Citation63]. Reduced infection risks for open-coil leads may further be attributed to the encouragement of fibrotic tissue ingrowth into the coiled lead structure which could decrease the ingress of bacterial contamination of the lead exit site [Citation63,Citation64]. Open-coil leads are less susceptible to ‘pistoning’ whereas longitudinal movement of cylindrical leads can allow passage of pathogens through the skin barrier while the lead is externalized during a trial [Citation63,Citation65,Citation66]. The development of open-coil percutaneous leads with a lower infection risk profile compared with conventional cylindrical leads has enabled PNS treatments with lead indwelling periods of up to 60 days as demonstrated in multiple studies in the present review.
The reviewed studies applied percutaneous PNS treatment in the management of an etiologically diverse set of pain conditions and reported consistent rates of treatment response and pain relief over time (). The pain conditions included in these studies have unique and complex pathways, and despite the apparent differences in composition of pain types (e.g., neuropathic and nociplastic), the study outcomes indicate that percutaneous PNS treatment was roughly equivalently effective across these varying pain etiologies. A recent publication of retrospective real-world outcomes following 60-day percutaneous PNS treatment in 6,160 patients found that 71% of patients reported ≥50% patient-reported pain relief and/or clinically significant improvement in quality of life across myriad peripheral nerve targets, which is highly similar to the aggregate responder rate in the present review (73% of patients reporting ≥50% reductions in pain relief and/or pain interference at EOT) [Citation55]. Additionally, a cross-sectional survey of patients who previously underwent 60-day percutaneous PNS treatment indicated that a majority of responders reported sustained relief ranging from 3 to 30 months from the start of treatment [Citation67]. These real-world studies included participants independent of pain condition or diagnosis with nerve targets throughout the body (e.g., shoulder, back, extremities, head and neck), complementing the prospective studies that have demonstrated consistency of treatment response across body regions and pain conditions.
The preponderance of clinical data demonstrates the therapeutic benefits of percutaneous PNS treatment through follow-up periods up to 14 months from lead placement (). In addition to the observed potential for long-term relief following percutaneous PNS treatments of up to 60 days, Deer and colleagues have summarized the proposed mechanistic factors that may explain how relief is sustained following lead withdrawal [Citation52,Citation53,Citation68]. Briefly, the central nervous system (CNS) responds to persistent nociceptive signaling from the periphery through neural plasticity-mediated reorganization and, in some cases, central sensitization to pain [Citation69]. This process may produce a chronic pain state whereby the individual experiences pain following an injury long after the expected time course of tissue healing. Conventional approaches to PNS are traditionally thought to rely on spinal segmental ‘gating’ of pain signals to provide relief [Citation70], thereby transiently reducing pain but requiring permanent implantation and/or continuous stimulation for long-term ongoing relief. In addition to reducing pain during the treatment period by transiently attenuating nociceptive signaling, a percutaneous PNS system designed to provide more targeted and robust activation of target fibers (e.g., the lead is placed remote to the nerve [0.5–3 cm]) may drive beneficial reconditioning in the CNS, supporting long-term pain relief by addressing underlying features of central sensitization [Citation15,Citation44,Citation71]. The demonstrated efficacy of percutaneous PNS treatment to manage chronic pain conditions such as post-amputation pain, shoulder pain and low back pain that have been refractory to front-line therapies, likely due to the central pain component [Citation72,Citation73], further supports a central role in PNS-mediated analgesic mechanisms.
Due to the central maintenance of pain, those with chronic pain often experience the effects of sensitization (e.g., primary and secondary hyperalgesia, sensory cortical remapping and allodynia) regardless of the original peripheral etiology or primary region of pain [Citation74,Citation75]. The efficacy of percutaneous PNS treatments across diverse applications (e.g., shoulder pain, neuropathic pain, axial low back pain, see and ) may imply convergent mechanisms of pain and pain relief in various pain indications [Citation55]. In other words, if chronic pain is centrally maintained, those with chronic pain may experience benefit from percutaneous PNS treatment targeting the nerve(s) innervating their region of pain whether in the shoulder, back, extremities or other regions. The consistency of demonstrated effects of percutaneous PNS treatment to reduce pain of both neuropathic and non-neuropathic origin, in addition to the sustained relief in pain and pain interference, supports the proposal that percutaneous PNS treatments act via central mechanisms [Citation36,Citation76,Citation77].
The reviewed studies included two PNS treatment strategies: a sensory stimulation approach and a motor activation approach. Stimulation at higher frequencies (e.g., 100 Hz stimulation of the femoral or sciatic nerves as in studies of post-amputation pain) is intended to target large diameter afferent fibers to produce comfortable paresthesia in the painful region. Stimulation of efferent motor fibers at lower frequencies (e.g., 12 Hz stimulation of terminal branches of motor nerves as in studies of shoulder or low back pain) is intended to produce comfortable muscle contractions and indirectly create physiological large-diameter afferent input (e.g., generated by golgi tendon organs, muscle spindles, etc.). A multi-modal approach utilizing combined sensory and motor stimulation has also been proposed as a complementary approach for certain body regions like the shoulder, which may leverage convergent mechanisms from direct sensory activation in conjunction with the indirect sensory feedback provided by motor activation [Citation15]. The outcomes of the studies highlight that both sensory and motor PNS treatment approaches produced significant reductions in pain and pain interference during treatment and after the EOT period (), supporting their convergence on a central mechanism as proposed by Deer and colleagues [Citation15].
The studies had treatment durations that ranged from 14 to 60 days. The FDA-cleared percutaneous PNS system referenced in several studies is indicated for up to 60 days of use and the most recent studies used 60-day treatment periods, though earlier studies also used varying stimulation periods (). While the outcomes were largely consistent across the reviewed studies, the heterogeneity of study designs does not permit direct comparison of treatment duration in this review. Of note, a recent real-world study found that longer treatment periods up to 60 days may improve identification of delayed responders and non-responders to stimulation with potential implications for stepwise treatment strategies prior to permanent implantation of neurostimulation systems [Citation16].
4.1. Limitations
The goal of the present review was to summarize the evidence from prospective studies of percutaneous PNS treatment in the management of chronic pain. Other recent publications have performed systematic reviews and produced consensus guidance for PNS more broadly [Citation11,Citation68]. In the current review, the authors constructed specific search terms for common literature indexing sites. Eligible publications varied in nerve target, study structure and follow-up duration and outcome measures which required consideration for pooled analyses. Seven studies were prospective case series, and three were RCTs (one placebo controlled and two standard of care controlled), and four studies had follow-up through at least 12 months. Additionally, the screening methodology varied among studies. Eight of the ten study cohorts used a baseline average or worst pain intensity of ≥4 as an eligibility criterion. In the other two studies, most patients had a baseline pain score ≥4 as well, even though one study used a threshold of ≥2/10 and one study did not report a pain threshold for eligibility [Citation36,Citation41]. Due to the heterogeneity in study designs and patient populations, no corrections were performed to account for missing data and the present review summarized outcomes ‘as-observed’. Regarding the potential for attrition bias, five of the ten study cohorts reported some level of attrition from baseline to the end of follow-up ranging from 16% (12/74) to 33% (3/9), and the other five cohorts reported no attrition. Across all ten cohorts, just 23/189 participants (12%) failed to complete follow-up (). Based on the number of total potential study visits, only 8% were found to be incomplete across all studies in the pooled patient-level data, which suggests the potential for attrition bias is relatively low ().
Pain was typically reported in individual studies as either the worst pain experienced by the participant in the last week (BPISF-3) or the average pain experienced by the participant in the last week (BPISF-5); both use a scale of 0 to 10 where 0 is no pain and 10 is the worst pain imaginable. Participant-level data analysis and the composite responder definition utilized BPISF-5 scores or, when average pain score was not reported in the study, BPISF-3 scores. While the questions are distinct, both have demonstrated strong correlation with each other, high test-retest reliability and similar sensitivity as valid primary outcome measures in clinical trials for chronic pain [Citation78,Citation79]. Study structure also varied in treatment duration, follow-up timepoints and sample size, with the largest enrolling up to 74 participants and the smallest with fewer than ten participants. To overcome differences in follow-up timelines and enable aggregation of data across study cohorts, follow-up data were combined into epochs defined from the time of lead implantation. Consistent with guidelines for meta-analysis in medical research, no additional meta-analysis was performed due to heterogeneity of study designs, study group sizes and pain indications [Citation80]; nonetheless, the aggregate data suggest that treatment using percutaneous PNS treatment for up to 60 days effectively reduces pain intensity and pain interference, and relief endures post-treatment in many patients.
5. Conclusion
This review of percutaneous PNS treatments for chronic pain summarizes the evidence across etiologies and nerve targets, including multiple randomized controlled trials, demonstrating the ability of this neurostimulation treatment to provide sustained relief of pain and the interference of pain with function without the need for implantation of a permanent PNS system. The evidence for efficacy alongside its strong safety profile indicates that percutaneous PNS can be a well-considered option in the nonopioid treatment of chronic pain.
6. Future perspective
This review of prospective clinical trials complements emerging real-world evidence supporting the use of percutaneous peripheral nerve stimulation to treat chronic pain. This evidence may be used to support the utilization of percutaneous PNS earlier in the treatment algorithm (e.g., ahead of opioid or neurodestructive options) to provide durable pain relief for patients.
Author contributions
SG Pritzlaff, J Chae, RD Wilson, WJ Huffman, ND Crosby and JW Boggs contributed to the design of the review. WJ Huffman and ND Crosby performed the literature review and data synthesis. SG Pritzlaff, U Latif, JM Rosenow, J Chae, RD Wilson, WJ Huffman, ND Crosby and JW Boggs contributed to drafting and revision of the manuscript.
Financial disclosure
The study was supported by SPR Therapeutics. The author has no other relevant affiliations or financial involvement with any organization or entity with a financial interest in or financial conflict with the subject matter or materials discussed in the manuscript apart from those disclosed.
Supplementary Materials
Download MS Word (60.3 KB)Acknowledgments
The authors wish to acknowledge the additional study investigators and study sponsors who provided access to patient-level data for this review, including M IJzerman and SPR Therapeutics.
Supplementary material
Supplementary data for this article can be accessed at https://doi.org/10.1080/17581869.2024.2352398
Competing interests disclosure
SG Pritzlaff has consulting agreements with Bioness, SPR Therapeutics, Nalu Medical, EBT Medical and receives royalties from Oxford University Press, Wolters Kluwer and has received educational grants (institutional) from Medtronic, Nevro, Biotronik and Abbott. U Latif has consulting agreements with SPR Therapeutics and Hydrocision, and has consulting agreements and is on the advisory board for Nevro, Abbott, Nalu, Spinal Simplicity, Vertos Medical, Omnia Medical and informed Consent and has received research funding from Mainstay Medical. JM Rosenow has consulting agreements with Boston Scientific, Monteris, Stryker and AIM Medical Robotics. J Chae has consulting agreements with and owns stock options in SPR Therapeutics, and is a coinventor on patents related to technologies reviewed herein. WJ Huffman is an employee of SPR Therapeutics. ND Crosby and JW Boggs are employees and hold stock options in SPR Therapeutics, and are coinventors on patents related to technologies reviewed herein. The author has no other competing interests or relevant affiliations with any organization or entity with the subject matter or materials discussed in the manuscript apart from those disclosed.
Writing disclosure
No writing assistance was utilized in the production of this manuscript.
References
- Treede RD, Rief W, Barke A, et al. Chronic pain as a symptom or a disease: the IASP Classification of Chronic Pain for the International Classification of Diseases (ICD-11). Pain. 2019;160(1):19–27. doi:10.1097/j.pain.0000000000001384
- Gureje O, Von Korff M, Simon GE, et al. Persistent pain and well-being. JAMA. 1998;280(2):147. doi:10.1001/jama.280.2.147
- Kawai K, Kawai AT, Wollan P, et al. Adverse impacts of chronic pain on health-related quality of life, work productivity, depression and anxiety in a community-based study. Fam Pract. 2017;34(6):656–661. doi:10.1093/fampra/cmx034
- Henschke N, Kamper SJ, Maher CG. The epidemiology and economic consequences of pain. Mayo Clin Proc. 2015;90(1):139–147. doi:10.1016/j.mayocp.2014.09.010
- Schaefer C, Sadosky A, Mann R, et al. Pain severity and the economic burden of neuropathic pain in the United States: BEAT Neuropathic Pain Observational Study. Clinicoecon Outcomes Res. 2014;6:483–496. doi:10.2147/CEOR.S63323
- Smith TJ, Hillner BE. The cost of pain. JAMA Network Open. 2019;2(4):e191532. doi:10.1001/jamanetworkopen.2019.1532
- King NB, Fraser V. Untreated Pain, Narcotics Regulation, and Global Health Ideologies. PLoS Med. 2013;10(4):e1001411. doi:10.1371/journal.pmed.1001411
- Rikard SM, Strahan AE, Schmidt KM, et al. Chronic pain among adults - United States, 2019–2021. MMWR. 2023;72(15):379–385. doi:10.15585/mmwr.mm7215a1
- Gaskin DJ, Richard P. The economic costs of pain in the United States. J Pain. 2012;13(8):715–724. doi:10.1016/j.jpain.2012.03.009
- Knotkova H, Hamani C, Sivanesan E, et al. Neuromodulation for chronic pain. Lancet. 2021;397(10289):2111–2124. doi:10.1016/S0140-6736(21)00794-7
- Deer TR, Naidu R, Strand N, et al. A review of the bioelectronic implications of stimulation of the peripheral nervous system for chronic pain conditions. Bioelectron Med. 2020;6:9. doi:10.1186/s42234-020-00045-5
- Deer T, Masone RJ. Selection of spinal cord stimulation candidates for the treatment of chronic pain. Pain Medicine. 2008;9(Suppl. 1):S82–S92. doi:10.1111/j.1526-4637.2008.00443.x
- Horlocker TT, Wedel DJ, Benzon H, et al. Regional anesthesia in the anticoagulated patient: defining the risks (the second ASRA Consensus Conference on Neuraxial Anesthesia and Anticoagulation). Reg Anesth Pain Med. 2003;28(3):172–197. doi:10.1053/rapm.2003.50046
- Lin CP, Chang KV, Wu WT, et al. Ultrasound-guided peripheral nerve stimulation for knee pain: a mini-review of the neuroanatomy and the evidence from clinical studies. Pain Med. 2020;21(Suppl. 1):S56–S63. doi:10.1093/pm/pnz318
- Deer TR, Eldabe S, Falowski SM, et al. Peripherally induced reconditioning of the central nervous system: a proposed mechanistic theory for sustained relief of chronic pain with percutaneous peripheral nerve stimulation. J Pain Res. 2021;14:721–736. doi:10.2147/JPR.S297091
- Naidu R, Li S, Desai MJ, et al. 60-day PNS treatment may improve identification of delayed responders and delayed non-responders to neurostimulation for pain relief. J Pain Res. 2022;15:733–743. doi:10.2147/JPR.S349101
- Zhou S, Hussain N, Abd-Elsayed A, et al. Peripheral nerve stimulation for treatment of headaches: an evidence-based review. Biomedicines. 2021;9(11):1–16. doi:10.3390/biomedicines9111588
- Xu J, Sun Z, Wu J, et al. Peripheral nerve stimulation in pain management: a systematic review. Pain Physician. 2021;24(2):E131–E152. doi:10.36076/ppj.2021.24.E131-E152
- Wilson RD, Kim CH. Percutaneous and implanted peripheral nerve stimulation for the management of pain: current evidence and future directions. Curr Phys Med Rehabilit Rep. 2020;8(1):1–7. doi:10.1007/s40141-019-00256-1
- Smith BJ, Twohey EE, Dean KP, et al. Peripheral nerve stimulation for the treatment of postamputation pain: a systematic review. Am J Phys Med Rehabil. 2023;102(9):846–854. doi:10.1097/PHM.0000000000002237
- D'Souza RS, Jin MY, Abd-Elsayed A. Peripheral nerve stimulation for low back pain: a systematic review. Curr Pain Headache Rep. 2023;27(5):117–128. doi:10.1007/s11916-023-01109-2
- Char S, Jin MY, Francio VT, et al. Implantable peripheral nerve stimulation for peripheral neuropathic pain: a systematic review of prospective studies. Biomedicines. 2022;10(10):2606. doi:10.3390/biomedicines10102606
- Gabriel RA, Ilfeld BM. Peripheral nerve blocks for postoperative analgesia: from traditional unencapsulated local anesthetic to liposomes, cryoneurolysis and peripheral nerve stimulation. Best Pract Res Clin Anaesthesiol. 2019;33(3):293–302. doi:10.1016/j.bpa.2019.06.002
- Gabriel RA, Swisher MW, Ilfeld BM. Percutaneous peripheral nerve stimulation for acute postoperative pain. Pain Manag. 2019;9(4):347–354. doi:10.2217/pmt-2018-0094
- Gabriel RA, Ilfeld BM. Percutaneous peripheral nerve stimulation and other alternatives for perineural catheters for postoperative analgesia. Best Pract Res Clin Anaesthesiol. 2019;33(1):37–46. doi:10.1016/j.bpa.2019.02.002
- Bramer WM, De Jonge GB, Rethlefsen ML, et al. A systematic approach to searching: an efficient and complete method to develop literature searches. JMed Lib Assoc. 2018;106(4):531–541. doi:10.5195/jmla.2018.283
- Sampson M, Barrowman NJ, Moher D, et al. Should meta-analysts search Embase in addition to Medline? J Clin Epidemiol. 2003;56(10):943–955. doi:10.1016/S0895-4356(03)00110-0
- Smith SM, Jensen MP, He H, et al. A comparison of the assay sensitivity of average and worst pain intensity in pharmacologic trials: an ACTTION systematic review and meta-analysis. J Pain. 2018;19(9):953–960. doi:10.1016/j.jpain.2018.02.016
- Schneider S, Junghaenel DU, Ono M, et al. III. Detecting treatment effects in clinical trials with different indices of pain intensity derived from ecological momentary assessment. J Pain. 2021;22(4):386–399. doi:10.1016/j.jpain.2020.10.003
- Jensen MP, Turner JA, Romano JM, et al. Comparative reliability and validity of chronic pain intensity measures. Pain. 1999;83(2):157–162. doi:10.1016/S0304-3959(99)00101-3
- Dworkin RH, Turk DC, Wyrwich KW, et al. Interpreting the clinical importance of treatment outcomes in chronic pain clinical trials: IMMPACT recommendations. J Pain. 2008;9(2):105–121. doi:10.1016/j.jpain.2007.09.005
- Pilitsis JG, Fahey M, Custozzo A, et al. Composite score is a better reflection of patient response to chronic pain therapy compared with pain intensity alone. Neuromodulation. 2021;24(1):68–75. doi:10.1111/ner.13212
- Goudman L, Billot M, Duarte RV, et al. Gradation of clinical holistic response as new composite outcome to evaluate success in spinal cord stimulation studies for pain. Neuromodulation. 2022;26(1):1–20. doi:10.1016/j.neurom.2022.08.113
- Turk DC, Dworkin RH, McDermott MP, et al. Analyzing multiple endpoints in clinical trials of pain treatments: IMMPACT recommendations. Initiative on methods, measurement, and pain assessment in clinical trials. Pain. 2008;139(3):485–493. doi:10.1016/j.pain.2008.06.025
- Chae J, Yu D, Walker M. Percutaneous, intramuscular neuromuscular electrical stimulation for the treatment of shoulder subluxation and pain in chronic hemiplegia: a case report. Am J Phys Med Rehabil. 2001;80(4):296–301. doi:10.1097/00002060-200104000-00014
- Yu DT, Chae J, Walker ME, et al. Intramuscular neuromuscular electric stimulation for poststroke shoulder pain: a multicenter randomized clinical trial. Arch Phys Med Rehabil. 2004;85(5):695–704. doi:10.1016/j.apmr.2003.07.015
- Chae J, Yu DT, Walker ME, et al. Intramuscular electrical stimulation for hemiplegic shoulder pain: a 12-month follow-up of a multiple-center, randomized clinical trial. Am J Phys Med Rehabil. 2005;84(11):832–842. doi:10.1097/01.phm.0000184154.01880.72
- Chae J, Wilson RD, Bennett ME, et al. Single-lead percutaneous peripheral nerve stimulation for the treatment of hemiplegic shoulder pain: a case series. Pain Pract. 2013;13(1):59–67. doi:10.1111/j.1533-2500.2012.00541.x
- Wilson RD, Gunzler DD, Bennett ME, et al. Peripheral nerve stimulation compared with usual care for pain relief of hemiplegic shoulder pain: a randomized controlled trial. Am J Phys Med Rehabil. 2014;93(1):17–28. doi:10.1097/PHM.0000000000000011
- Wilson RD, Harris MA, Gunzler DD, et al. Percutaneous peripheral nerve stimulation for chronic pain in subacromial impingement syndrome: a case series [Journal article]. Neuromodulation. 2014;17(8):771–776; discussion 776. doi:10.1111/ner.12152
- Yu DT, Chae J, Walker ME, et al. Percutaneous intramuscular neuromuscular electric stimulation for the treatment of shoulder subluxation and pain in patients with chronic hemiplegia: a pilot study. Arch Phys Med Rehabil. 2001;82(1):20–25. doi:10.1053/apmr.2001.18666
- Renzenbrink GJ, Ijzerman MJ. Percutaneous neuromuscular electrical stimulation (P-NMES) for treating shoulder pain in chronic hemiplegia. Effects on shoulder pain and quality of life. Clin Rehabil. 2004;18(4):359–365. doi:10.1191/0269215504cr759oa
- Farrar JT, Young JP Jr, LaMoreaux L, et al. Clinical importance of changes in chronic pain intensity measured on an 11-point numerical pain rating scale. Pain. 2001;94(2):149–158. doi:10.1016/S0304-3959(01)00349-9
- Rauck RL, Cohen SP, Gilmore CA, et al. Treatment of post-amputation pain with peripheral nerve stimulation. Neuromodulation. 2014;17(2):188–197. doi:10.1111/ner.12102
- Rauck RL, Kapural L, Cohen SP, et al. Peripheral nerve stimulation for the treatment of postamputation pain–a case report. Pain Pract. 2012;12(8):649–655. doi:10.1111/j.1533-2500.2012.00552.x
- Kapural L, Gilmore CA, Chae J, et al. Percutaneous peripheral nerve stimulation for the treatment of chronic low back pain: two clinical case reports of sustained pain relief. Pain Pract. 2018;18(1):94–103. doi:10.1111/papr.12571
- Gilmore CA, Kapural L, McGee MJ, et al. Percutaneous peripheral nerve stimulation (PNS) for the treatment of chronic low back pain provides sustained relief. Neuromodulation. 2019;22(5):615–620. doi:10.1111/ner.12854
- Cohen S, Gilmore C, Kapural L, et al. Percutaneous peripheral nerve stimulation for pain reduction and improvements in functional outcomes in chronic low back pain. Mil Med. 2019;184(Suppl. 1):537–541. doi:10.1093/milmed/usy310
- Gilmore CA, Kapural L, McGee MJ, et al. Percutaneous peripheral nerve stimulation for chronic low back pain: prospective case series with 1 year of sustained relief following short-term implant. Pain Pract. 2020;20(3):310–320. doi:10.1111/papr.12856
- Gilmore CA, Desai MJ, Hopkins TJ, et al. Treatment of chronic axial back pain with 60-day percutaneous medial branch PNS: primary end point results from a prospective, multicenter study. Pain Practice. 2021;21:877–889. doi:10.1111/papr.13055
- Gilmore C, Ilfeld B, Rosenow J, et al. Percutaneous peripheral nerve stimulation for the treatment of chronic neuropathic postamputation pain: a multicenter, randomized, placebo-controlled trial. Reg Anesth Pain Med. 2019;44(6):637–645. doi:10.1136/rapm-2018-100109
- Gilmore CA, Ilfeld BM, Rosenow JM, et al. Percutaneous 60-day peripheral nerve stimulation implant provides sustained relief of chronic pain following amputation: 12-month follow-up of a randomized, double-blind, placebo-controlled trial. Region Anesth Pain Med. 2020;45(1):44–51. doi:10.1136/rapm-2019-100937
- Gilmore C, Deer T, Desai M, et al. Durable patient-reported outcomes following 60-day percutaneous peripheral nerve stimulation (PNS) of the medial branch nerves. Intervent Pain Med. 2023;2:100243. doi:10.1016/j.inpm.2023.100243
- Deer TR, Gilmore CA, Desai MJ, et al. Percutaneous peripheral nerve stimulation of the medial branch nerves for the treatment of chronic axial back pain in patients after radiofrequency ablation. Pain Med. 2021;22(3):548–560. doi:10.1093/pm/pnaa432
- Huntoon MA, Slavin KV, Hagedorn JM, et al. A retrospective review of real-world outcomes following 60-day peripheral nerve stimulation for the treatment of chronic pain. Pain Physician. 2023;26(3):273–281. doi:10.1016/j.neurom.2023.04.305
- Boggs JW, Chae J, Bennett M. Peripheral nerve stimulation for pain suppression. In: Krames E, Peckham PH, Rezai AR, editors. Neuromodulation. Vol. 2. Cambridge, MA: Academic Press; 2018. p. 729–740. doi:10.1016/B978-0-12-805353-9.00057-7
- Hoffmann CM, Coy DS, Moeschler SM, et al. Incidence of temporary peripheral nerve stimulator lead tip retention: a retrospective review of 80 lead placements. Neuromodulation. 2023;26(3):690–693. doi:10.1016/j.neurom.2022.01.018
- Wilson R, Harris M, Bennett M, et al., , et al. editors. Intramuscular Peripheral Nerve Stimulation for Chronic Subacromial Impingement Syndrome. Berlin, Germany: International Neuromodulation Society 11th World Congress; 2013.
- Pope JE, Deer TR, Falowski S, et al. Multicenter retrospective study of neurostimulation with exit of therapy by explant. Neuromodulation. 2017;20(6):543–552. doi:10.1111/ner.12634
- Murphy KR, Han JL, Hussaini SM, et al. The volume-outcome effect: impact on trial-to-permanent conversion rates in spinal cord stimulation. Neuromodulation. 2017;20(3):256–262. doi:10.1111/ner.12526
- Eldabe S, Duarte RV, Gulve A, et al. Does a screening trial for spinal cord stimulation in patients with chronic pain of neuropathic origin have clinical utility and cost-effectiveness (TRIAL-STIM)? A randomised controlled trial. Pain. 2020;161(12):2820. doi:10.1097/j.pain.0000000000001977
- North R, Desai MJ, Vangeneugden J, et al. Postoperative infections associated with prolonged spinal cord stimulation trial duration (PROMISE RCT). Neuromodulation. 2020;23(5):620–625. doi:10.1111/ner.13141
- Ilfeld BM, Gabriel RA, Saulino MF, et al. Infection rates of electrical leads used for percutaneous neurostimulation of the peripheral nervous system. Pain Pract. 2017;17(6):753–762. doi:10.1111/papr.12523
- Corey JM. Tissue response and corrosion study of the Peterson type intramuscular electrode. MS Thesis. Case Western Reserve University; 1990;1-234.
- Marsolais EB, Kobetic R. Implantation techniques and experience with percutaneous intramuscular electrodes in the lower extremities. J. Rehabil. Res. Dev. 1986;23(3):1–8.
- Mortimer JT, Bhadra N. Peripheral nerve and muscle stimulation. Singapore: Neuroprosthetics: theory and practice: World Scientific; 2004. p. 638–682. doi:10.1142/9789812561763_0020
- Pingree MJ, Hurdle MF, Spinner DA, et al. Real-world evidence of sustained improvement following 60-day peripheral nerve stimulation treatment for pain: a cross-sectional follow-up survey. Pain Manag. 2022;12(5):611–621. doi:10.2217/pmt-2022-0005
- Deer TR, Esposito MF, McRoberts WP, et al. A systematic literature review of peripheral nerve stimulation therapies for the treatment of pain. Pain Med. 2020;21(8):1590–1603. doi:10.1093/pm/pnaa030
- Latremoliere A, Woolf CJ. Central sensitization: a generator of pain hypersensitivity by central neural plasticity. J Pain. 2009;10(9):895–926. doi:10.1016/j.jpain.2009.06.012
- Melzack R, Wall PD. Pain mechanisms: a new theory. Science (New York, NY). 1965;150(3699):971–979. doi:10.1126/science.150.3699.971
- Brocker DT, Grill WM. Principles of electrical stimulation of neural tissue. Handb Clin Neurol. 2013;116:3–18. doi:10.1016/B978-0-444-53497-2.00001-2
- Cohen S, Hsu. Postamputation pain: epidemiology, mechanisms, and treatment. J Pain Res. 2013;2013(6):121. doi:10.2147/JPR.S32299
- Treister AK, Hatch MN, Cramer SC, et al. Demystifying poststroke pain: from etiology to treatment. PM&R. 2017;9(1):63–75. doi:10.1016/j.pmrj.2016.05.015
- Bartsch T, Goadsby PJ. Central mechanisms of peripheral nerve stimulation in headache disorders. Prog Neurol Surg. 2011;24:16–26. doi:10.1159/000323008
- Strand NH, D'Souza R, Wie C, et al. Mechanism of action of peripheral nerve stimulation. Curr Pain Headache Rep. 2021;25(7):47. doi:10.1007/s11916-021-00962-3
- Chae J, Wilson RD, Bennett ME, et al. Percutaneous peripheral nerve stimulation for the treatment of acute and chronic pain. In: Comprehensive Pain Management in the Rehabilitation Patient, a Reference Guide: New York City, NY, USA: Springer; 2017. p. 899–910. doi:10.1007/978-3-319-16784-8_70
- Wilson RD, Knutson JS, Bennett ME, et al. The effect of peripheral nerve stimulation on shoulder biomechanics. Am J Phys Med Rehabilit. 2017;96(3):191–198. doi:10.1097/PHM.0000000000000677
- Radbruch L, Loick G, Kiencke P, et al. Validation of the German version of the Brief Pain Inventory. J Pain Symptom Manage. 1999;18(3):180–187. doi:10.1016/S0885-3924(99)00064-0
- Erdemoglu AK, Koc R. Brief Pain Inventory score identifying and discriminating neuropathic and nociceptive pain. Acta Neurol Scand. 2013;128(5):351–358. doi:10.1111/ane.12131
- Haidich AB. Meta-analysis in medical research. Hippokratia. 2010;14(Suppl. 1):29–37.