Abstract
Renewable energies are advancing across many fronts – and now a new front has opened up in the form of dispatchable concentrating solar power (CSP). It is in emerging markets such as China, India and countries in North Africa and the Middle East as well as South America and southern Africa that CSP power plants with 24/7 dispatchable power are starting to be viewed as a viable way forward. The emerging CSP industrial value chain in China, India, southern Africa and elsewhere is expected to exert its consequent dramatic impact on cost reductions, which will in turn drive the diffusion of CSP around the world as a key challenger and alternative to nuclear and fossil fuel power. In this paper, we review these developments and discuss the effects attributable to the emerging learning curve, combined with technology progress involving molten salt heat transfer and reductions in water requirements, as well as patenting trends in the global CSP industry.
Introduction
The prime solution to the problem of mitigating climate change, and managing carbon, is to move the world as fast as possible to renewables. The technologies that permit the generation of electric energy from renewable sources are advancing along many fronts, as wind power, solar photovoltaics and bioenergy (e.g., waste-to-energy) move ahead, driven by dramatically falling costs and improving technologies. But the goal of dispatchable power achieved through MW-scale and even GW-scale solar power stations has remained elusive. Now, that is about to change as concentrating solar power (CSP) moves to a new phase, driven this time by developments in the emerging markets of China, India, southern Africa and Middle East and North Africa (MENA) countries as well as South America. This is, finally, where the real cost advantages of CSP will be able to prove themselves, as large-scale adoption becomes the norm and costs are driven down accordingly.
In this paper, we survey developments in CSP (as well as current patenting trends) to gain insight into the direction of its future technology and business evolution.Footnote1 We review the evidence and, based on this, develop two main arguments. The first is to demonstrate that CSP with molten salts technology provides the promise and reality of dependable, dispatchable power on a 24/7 basis – thereby providing a realistic alternative to thermal fossil fuel and nuclear power. The second argument concerns emerging industrial powers, China in particular, and how they are rapidly moving to a world leadership position in CSP. China is scaling up its goals under the 12th Five Year Plan to reach 30% of non-fossil energy sources by 2015; CSP is likely to play a major role in achieving this policy goal. China is also emerging as a leader in CSP technology patenting, accounting for no less than a third of all patents taken out so far. Our argument is that this serious entry by China as well as other players is about to have a dramatic impact in lowering costs, both for China and for other countries.
Keywords | ||
Concentrating solar power (CSP): | = | Solar energy can be concentrated via fields of lenses and mirrors on a heat pipe, or tower, which produces temperatures sufficient to vaporize fluid in order to drive a turbine - just as in a regular thermal power station. |
Renewable energy: | = | Renewable energy is defined as energy that comes from natural resources such as sunlight, wind, hydro, tides, waves and geothermal. |
Molten salts: | = | Molten salts (e.g., nitrate salts of sodium or potassium) were utilized as a means of heat absorption in nuclear reactors. It was the Italian nuclear physicist Carlo Rubbia who came up with the original conception of transferring this energy absorption technology from the nuclear realm to that of solar power, where the molten salts would again absorb energy but, in this case, with a view to extracting it later in a power-generating device. |
Dispatchable power: | = | Dispatchable power refers to sources of electricity that can be dispatched at the request of power grid operators; that is, power plants can adjust their power output on demand. |
CSP versus photovoltaic
Electric power can be generated from the sun in two quite different ways, as elaborated in . Solar energy can be converted directly via the photoelectric effect, as in photovoltaic (PV) cells. Or the sun's energy can be concentrated via fields of lenses and mirrors on a heat pipe, or tower, which produces temperatures sufficient to drive a turbine – just as in a thermal power station.Footnote2 The contending technologies involved in CSP are fourfold; they involve fields of parabolic troughs, or mirrors, which can be rotated to follow the sun; fields of flat Fresnel mirrors, which likewise follow the sun (lower efficiency but cheaper cost); power towers, where the sun's energy is focused on a single elevated point at the centre of the field; or parabolic dish systems, each of which carries its own point of collection. There is as yet no clear leader amongst these competing CSP technologies. Note that PV cells can also produce power from concentrated sunlight, in which case the systems are known as concentrated photovoltaic (CPV).
Table 1. Major difference between concentrating solar power and photovoltaic.
While CSP costs were coming down and investment was starting to pick up, in the mid-2000s, it looked as though CSP might outdo solar PV as the next solar wave. But then the dramatic cost reductions driven by China's expansion of PV cell production, combined with the proven bankability of PV power, reversed that situation, and investments in CSP slowed. Indeed, some plants scheduled as CSP operations were partially switched to PV – such as the much-discussed 1-GW facility at Blythe (California), destined to be the largest in the world, which has been scaled back.Footnote3 Investments in Spain which had dominated the 2000s have been declining with the announcement by the new Popular Unity government elected in 2011 that financial support for CSP would be wound back, while planned installations in the US have met with (perhaps predictable) delays.
Now, new players are weighing in and transforming CSP prospects once again. China is one of the leaders, with its announcement that it intends to build 1 GW of CSP installations by 2015 and further ramp up to 3 GW of commercial-scale activity by 2020. Such commitments promise to drastically enlarge the scale of activities and thereby impact on the learning curve, driving down costs of components and systems, just as China's large-scale entry into solar PV had a dramatic impact on costs in that sector. But China is not alone. Both India and South Africa are scaling up their interest, and have opened up reverse auctions for new CSP licenses that are also helping to drive down costs and set new benchmarks for the cost of CSP power. The Middle Eastern and Gulf states are moving into CSP, to complement and offset their dependence on oil, with Saudi Arabia announcing a 25-GW goal to be met by 2030, and Qatar (currently the world's largest gas exporter) targeting to reach 1.8 GW of CSP capacity by 2020; the United Arab Emirates (UAE) is investing in a big way, and has already brought on stream the 100-MW Shams1 plant in Abu Dhabi. In smaller ways, sun-rich countries such as Israel and Australia are also making tentative moves to support CSP.Footnote4
Meanwhile, CSP continues to advance in its traditional strongholds. For example, the US initiated the US$2 billion (in which $1.45 billion come from a federal loan guarantee) Abengoa Solana Project in Arizona in 2010 to create one of the largest parabolic trough solar power plants in the world. This plant utilized an innovative molten salt storage system and began commercial operation in 2013; it has demonstrated a capacity to generate up to 6 hours of dispatchable energy on demand. The Canadian company SHEC (Solar Hydro Energy Corporation) Energy's new Ultra Lite CSP technology demonstrated a materials cost reduction of 89% in its parabolic dish technology and proprietary thermal energy storage system (again, utilizing molten salt technology), in 2013 [Citation106]. Spain was the first European country to introduce a feed-in tariff (FiT) system for CSP, in 2002, and in 2007, FiT regulations were refined, improving remuneration options for CSP plants and driving the diffusion of CSP. However, in January 2012, FiTs were cancelled for new applications, and would not be awarded to CSP plants beyond the 2355 MW approved in 2009 to become operational by 2014. The latest development in terms of the FiT came in July 2013, in which both renewables and cogeneration plants will receive payment for their investment, instead of the former FiT.
Despite the legislative setback in Spain, the new initiatives developed in the US, Canada, China, South Africa and elsewhere are breaking new ground in the quest to reduce the cost of CSP systems and improve generating efficiencies. Even though the emerging countries are seen as aggressively joining the CSP development, the leading CSP countries have significantly enhanced their CSP learning curves through an increasing number of projects. These include, up to September 2014, 62 projects in Spain, 42 in the US, 10 in Italy and 9 in Australia, as derived from CSP World.Footnote[6] Consequently, a series of global value chains for the CSP industry are in the process of being formed. It is also worth mentioning that Japan could become a CSP player, in its post-Fukushima mode, although there is currently only one micro-CSP project operating in Tokyo.Footnote5
The range of CSP technologies and major projects
Modern CSP traces its origins to the series of solar energy generating systems (SEGS) established in the Mojave Desert in California in the 1980s. But the US dithered over supporting such developments at the federal level, and the lead passed to European countries such as Spain which offered FiT support for several facilities. The technologies employed were based on parabolic trough technology but also included power tower facilities and the use of molten salts as a heat transfer fluid (HTF) to provide long-term heat storage and the possibility of providing dispatchable 24/7 power generation. As a result of these and other developments, there is now a well-established range of CSP technologies available – without any yet emerging as a “dominant” technology where cost reduction might be driven by massive roll-out, changing the competitive dynamics in the industry.Footnote6
The original design associated with SEGS in California involved a field array of parabolic mirrors with the capacity to change orientation during the day to capture the maximum solar energy, focusing the sun's energy onto a tube running the length of the field and containing oil as an HTF. The heated oil then turns water into steam to drive a conventional turbine to generate power. This parabolic trough or parabolic mirror (PB) arrangement remains closest to a dominant technology. The major alternatives are fourfold. These are:
The concept of focusing the sun's energy from the field of heliostats onto a single point placed on an elevated tower, or “power tower” – with the advantage that higher temperatures can be secured by focusing the energy on a single point;
A field of parabolic mirrors and a pipe traversing the field carrying a liquid that is heated by the concentrated sun's rays – sometimes with added molten salt storage;
A field of compact linear Fresnel reflectors (CLFR) that provides much lower costs and land use impact in construction;
The concept of modular design utilizing parabolic dish construction, where each dish (rated at around 133 kW) reflects and focuses light on its own collector, and then the high-temperature fluid is brought together for final power generation.
There are leading projects for each of these designs, indicating that they are all moving to the point where mass production industrial dynamics are feasible and imminent. For parabolic trough technology, there are numerous small-scale Spanish installations (e.g., Andasol 1 and 2), while in the US the leading contender is the Solana generating station in Arizona, rated at 280 MW, which went online in October 2013 and generates power night and day through its use of molten salt technology. For power tower technology, there are again some Spanish examples (e.g., Gemasolar, Alcázar and PS10/20), while a leading US contender is in Ivanpah, a 370 MW facility being erected in the Mojave desert and now under the control of BrightSource Energy (BSE), which began commercial operation at the beginning 2014; and there is Crescent Dunes (the first commercial-scale tower CSP plant in the US equipped with molten salt storage), a 110-MW installation developed by Solar Reserve, which was completed in February 2014 and is due to come into commercial operation by the end of 2014. The world's largest linear Fresnel array was opened in India in October 2012 as a desalination plant developed by Empereal-KGDS (KGDS Renewable Energy Private Limited) and funded by the Department of Science and Technology, Government of India; it went into commercial operation in February 2013. In our view, the most important technological development by far is the advance from using oil as an HTF to the use of molten salts, which provide superior heat retention properties and the possibility of supplying dispatchable power. This overcomes the principal problem with solar power, which is that in its PV version it delivers power only during daylight hours. Molten salts (e.g., nitrate salts of sodium or potassium) were utilized as a means of heat absorption in nuclear reactors. It was the Italian nuclear physicist Carlo Rubbia who came up with the original conception of transferring this energy absorption technology from the nuclear realm to that of solar power, where the molten salts would again absorb energy but, in this case, with a view to extracting it later in a power generating device. As Director of the Italian Energy Research Institute (ENEA), Dr. Rubbia had the first molten salts demonstration facility established at the ENEA labs at Casaccia, outside Rome – where it continues to function today.Footnote7
Molten salts technology is now diffusing around the world – with versions developed by the Spanish firm Abengoa as well as the US engineering firm Pratt & Whitney/Rocketdyne (PWR), licensed exclusively to BS). Italy and ENEA retain a strong interest. The world's first fully commercial-scale CSP facility utilizing molten salts technology was the Gemasolar project established in Seville, Spain, by Torresol, a joint venture between SENER and MASDAR from Abu Dhabi. The Torresol Gemasolar plant in Seville, Spain, is a 19.9-MW plant that covers 185 ha; it counts as a baseload plant with heat storage of 15 hours (giving uninterrupted power supply). The plant is operating with a 75% capacity factor, meaning CSP’s capacity factor is quite similar to the CF for coal, nuclear and natural gas facilities.Footnote8 The new benchmark molten salt project is Crescent Dunes in Nevada, rated at 110 MW and providing baseload dispatchable power for up to 7 hours beyond sunset. This plant also sets a benchmark for cost, being provided through a Power Purchase Agreement to the Nevada utility at a cost of US$135/MWh.
This, then, is the technological state of play as the world embarks on the next big push in CSP uptake – where the leaders this time will be coming from China, India, southern Africa and the Middle East. The next phase of the evolution of CSP has created an entirely new industrial sector and supply chain, to produce the heat collecting elements (HCEs) including specially designed and patented piping to carry the HTF. Italy, with its ENEA/Archimede project, was an early starter, with the Angelantoni company producing a specially designed and patented tube called the high-temperature receiver tube, HEMS08, undergoing thorough testing at the Archimede 10-MW demonstration site at Priolo Gargallo in Sicily. On the other hand, in China, the Huiyin Group has developed its vacuum tube HCEs and is now receiving commercial orders. These may be viewed as the beginnings of an important new industrial trajectory.
The new Big Push from emerging industrial powers
Attention is about to shift from European and US projects, important as they have been in establishing the technological options and starting a learning curve process, to new projects in the emerging economies. In particular, both China and India have built up comprehensive CSP industrial value chains, in different modes, and are aggressively engaging in the deployment of CSP as one of the major energy alternatives.
China
China is one of the leaders in the field, announcing in 2011 an ambitious goal of 21 GW for solar power by 2015, including 1 GW for CSP, and then raising the goal (in 2012) to 3 GW for PV and 3 GW for CSP by 2015 [Citation108]. However, due to the short-term to mid-term barriers, including the lower cost of Chinese PV energy, the difficulty of transmitting electricity from the western to the eastern coastal industrial areas and the long periods of water scarcity, heavy brown clouding and sandstorms, the challenge faced by China's CSP industry has led to slippage in these goals, and the target goal is now set at 1 GW in 2015 and 3 GW in 2020 for CSP (and 32 GW for PV by 2020).
Even so, this would still just about double global cumulative capacity in CSP, which had reached around 3.5 GW by 2013. The updated plan still has clearly recognized the potential of CSP, with the longer-term target being raised to 3 GW of commercial-scale activity by 2020, and the cost anticipated to be thereby driven down to something comparable to burning coal, as low as US$90 to $100/MWh. It would therefore be expected to kick-start the learning curve and drive rapid cost reduction – with dramatic impact on further diffusion possibilities.
If these goals are fulfilled (and China has met or exceeded such targets in other fields), then China is likely to emerge shortly as the world's leading CSP proponent. As specified in the 12th Five-Year Plan, the 3 GW of CSP installations by 2020 would be located mainly in Tibet, Inner Mongolia, Gansu, Ningxia, Xinjiang, Qinghai and Yunnan, all provinces with high levels of insolation. None of these projects has yet reached commercial scale. China currently has two pilot solar power towers operating in Beijing – one is the 1-MW Yanqing Solar Power station and the other is the 1.5-MW Beijing Badaling Solar Tower, both completed in August 2012. The two projects are led and developed by the Institute of Electrical Engineering of the China Academy of Sciences (IEECAS). Working together with many Chinese public and private companies, the IEECAS has become the world's second most important source of scientific papers on CSP, second only to the German Aerospace Center (DLR), according to our search on the Web of Science in June 2013. As shown in , it is also noted that another operating demonstration site is the 1.5-MW e-Cube 1 project in Hainan, which is operating with the first modular heliostat technology in the world. The demonstration sites and commercial-scale operations that are being planned or constructed in China (up to September 2014) are also shown as in . However, some of the projects are either waiting for government approval or are under evaluation; further announcements are expected in the near future.Footnote9
Table 2. Concentrating solar power projects in China (up to September 2014).
To illustrate, the Qinghai Delingha CSP project involves the building of a 50-MW parabolic trough plant in the northern Qinghai province, with 7-hours of storage provided by molten salts. The project is jointly financed by the China General Nuclear Power Corp. and the Export-Import Bank of China along with the Asian Development Bank [Citation110]. The latest announcement concerning the Qinghai Delingha Solar Thermal Power generation project came in November 2014, from the US-based BrightSource Energy Inc and Shanghai Electric Group, involving plans to build two 135-MW power tower projects. Construction is to start in 2015 with completion set for 2017. The project will eventually involve six power tower plants utilizing BrightSource technology [Citation111]. The biggest project announced so far in China is a deal struck in January 2010 with the US firm eSolar (headquartered in Pasadena, CA) to build a series of solar thermal farms totaling 2 GW by 2020.Footnote10 The firm eSolar utilizes a modular technology based on 46-MW units involving field arrays of small flat mirrors tilted towards the sun, and a power tower – a unit that can be replicated many times over.Footnote11
To fulfill the national target, China is in the process of establishing a comprehensive CSP industrial value chain, ranging from developers, component supplies, engineering, procurement and construction (EPC) contractors and distributors, as shown in . It is worth noting that the companies along the CSP industrial value chain are mainly domestic Chinese companies or state-owned companies – as contrasted with the case of India (and other emerging countries) where foreign companies essentially dominate the key materials and components supplies (as discussed below). China's world-leading production capacities in glass, steel, concrete and molten salts assure the low cost and independent supplies of CSP raw materials and the country's enormous potential to develop CSP as an industry.Footnote12 China's strength lies in its huge natural resources, manufacturing capacity and competitively priced local equipment, so unless there is an important need for foreign technology equipment, it will be challenging for foreign companies to enter the domestic market. The CSP development market in China is dominated by the so-called Big Five state-owned utility players (i.e., China Guodian, China Huaneng, China Power Investment, China Datang and China Huadian) and China Guangdong Nuclear Company.
Figure 1. The CSP industrial value chain in China. CSP: Concentrating solar power; EPC: Engineering, procurement and construction contractors. Data from CSP Today [Citation8], compiled by the authors.
![Figure 1. The CSP industrial value chain in China. CSP: Concentrating solar power; EPC: Engineering, procurement and construction contractors. Data from CSP Today [Citation8], compiled by the authors.](/cms/asset/569c48a5-5e5f-414e-82d6-c8178c144a72/tcmt_a_987492_f0001_c.jpg)
China adopts the strategy in all its energy dealings of opening up one field of investment after another in a planned sequence, with attention focused on the development of markets as well as the absorption of technology from around the world and the building of local supply chains. This has worked extremely effectively in the related renewable energy fields of wind power and solar PV, and now it is set to prove its worth in CSP as well.
The China CSP developments need to be seen in the context of the country's major push towards renewable energies as a prime means of ensuring energy security, with the green development strategies complementing the black strategies based on coal, and oil and gas. China's strategies all along seem to have been focused on building green energy industries as fast as possible, driving down the costs through mass production, as a principal means of building energy security (based on technological development and domestic renewable energy resources) and for avoiding potential conflicts based on the exploitation and import of fossil fuels. The recent announcements from the National Development and Reform Commission of a cap of 4 billion tonnes on China's coal consumption, and caps on carbon emissions, create further investment certainty in favor of renewables in China – and can be expected to work to support the expansion of the CSP industry.
India
Other newly industrializing countries are moving quickly to take advantage of the new state of play. India missed out on world leadership in the earlier fields of wind power and solar PV, and now seems to be making a determined bid as a strong player in CSP, taking advantage of the country's excellent insolation. Under the Jawaharlal Nehru National Solar Mission (JNNSM), it is anticipated that India will be producing 20 GW of grid-connected solar power by 2020, of which 50% or 10 GW could be CSP.Footnote13 In addition to large Indian companies like Tata Power, which are becoming involved in renewable energies, there are smaller Indian specialist firms such as Coromandal, Electrotherm and Lanco Solar emerging as significant players.Footnote14 Under phase 1 of the JNNSM program, a total of seven CSP projects amounting to 470 MW were awarded – but with PV becoming more cost-effective as well as financing and execution repercussions, many of these are now delayed [Citation115]. The delays were derived from various factors, including the short construction timelines, the current status of the involvement of multiple lenders, the lack of essential components such as HTF, and the large gap between predicted and actual annual direct normal irradiance (DNI). Of the seven projects, only Godawari Green Energy's 50-MW CSP plant was commissioned and connected to the grid in June 2013.
The Indian state of Gujarat issued a Call for Expression of Interest in November 2013 for a 50-MW CSP plant featuring energy storage technology, demonstrating the direction in which Indian initiatives are moving.
By 2013, India had 56 MW of operational CSP plants, together with five CSP plants under construction totaling 254 MW, and three in development totaling 210 MW. Many more are in the planning stages. Despite reports of delays, the Indian support for CSP is substantial and will further help to drive down learning costs. shows the demonstration sites and commercial-scale operations that have been planned or are under construction in India, while indicates that the production of the majority of Indian CSP components is essentially dominated by foreign companies including those from Spain, Germany and the US. However, the local manufacturing capabilities are improving steadily, which provides a promising picture for the future development of the solar program in India. As in China, the key will be the development of local supply chains – as now recognized also by the World Bank [Citation9].
Table 3. Concentrating solar power projects in India (up to September 2014).
Figure 2. The CSP industrial value chain in India (up to May 2013). CSP: Concentrating solar power; EPC: Engineering, procurement and construction contractors. Source: CSP Today [Citation8]
![Figure 2. The CSP industrial value chain in India (up to May 2013). CSP: Concentrating solar power; EPC: Engineering, procurement and construction contractors. Source: CSP Today [Citation8]](/cms/asset/0cc0285f-37de-4487-a8ab-8a67f4bb8178/tcmt_a_987492_f0002_c.jpg)
Middle East and North Africa
The latest new player in CSP is proving to be the Middle East and North Africa (MENA) region, owing to its hunger for industrialization and development of local manufacturing expertise.Footnote15 Saudi Arabia has announced major projects amounting to 25 GW to be phased in by 2032, with its national CSP firm ACWA Power as the major mover. According to the King Abdullah City of Atomic and Renewable Energy (K. A. CARE), this value is part of an overall target of installing 54 GW of renewable energy capacity, comprising 25 GW of CSP, 16 GW of PV and 13 GW of wind energy, geothermal and waste-to-energy power plants. Meanwhile, ACWA won an initial round of bidding to take charge of the 160-MW project being promoted at Ouazazate, in Morocco. shows the demonstration sites and commercial-scale operations that are planned or under constructed in the MENA countries.Footnote16
Table 4. Concentrating solar power projects in Middle East–North Africa countries (up to September 2014).
Southern Africa
Southern Africa is also emerging as a major CSP player, with its excellent solar resource (up to 2900 kWh/m2/annum) and huge land availability. The demonstration potential of CSP projects in southern Africa is vast – estimated at up to 40 GW by South Africa's principal electricity generator, Eskom. To date, all the projects are to be found in South Africa, but there is clearly potential for expansion in Mozambique and elsewhere in southern Africa. The major project in South Africa is aimed at building a 5-GW solar park in the Northern Cape; this would be a 15-km by 3-km area devoted to CSP and PV solar farms (totaling 45 km2).Footnote17 The variable time-of-day tariff introduced in 2013 will also help promote CSP with storage for generating energy during peak hours. In addition, the commitment shown by the government toward CSP, the strong manufacturing industry and land availability are all encouraging factors for the development of CSP. An example is the Saudi Arabian ACWA's Bokpoort project in South Africa, which broke ground in September 2013 and is due to start production in December 2015. All together, informed commercial expectations are for a 100-fold expansion over the next decade and a half, reaching 100 GW by 2025.Footnote18 , below, shows that the demonstration sites and commercial-scale operations have been planned or are under construction in South Africa.
Table 5. Concentrating solar power projects in South Africa (up to September 2014).
South America
The countries of South America, despite their high levels of insolation and water supplies, missed out on the early waves of success with renewables – although it is worth acknowledging that Brazil has always had a high proportion of hydropower in its energy mix. As shown in , a new generation of CSP projects are either coming on line or are under construction in many South American countries. In Chile, for example, remote mining operations are increasingly turning towards CSP as a power source (because of its reliability rather than its cost advantage) and the Atacama Desert is proving to be a “fertile” region for new CSP development. Abengoa, for example, won a tender in January 2014 to build a 110-MW CSP tower plant with sufficient energy storage to provide dispatchable, 24/7 power [Citation118]. There are many more such projects in the planning stage.
Table 6. Concentrating solar power projects in South America (up to September 2014).
Costs and the emergent learning curve
All renewable energy technologies share the feature that their costs are falling, driven by the experience curve or learning curve. This certainly has driven global uptake of wind and solar PV power – and now is starting to exert its effect in CSP as well, along with the drop in CSP capital costs. Most of the data concerning the reality of the learning curves is proprietary and is not being made public as yet. An exception is the consulting firm AT Kearney which, in conjunction with European Solar Thermal Electricity Association (ESTELA), issued an influential report in 2010, in which the likely cost reductions were estimated to be 40–55% and anticipated market expansion was estimated at 100 GW by 2025.Footnote19
The complication in these assessments of likely future trends is the impact of rapid cost reductions in alternative renewables such as solar PV and the unconventional fossil resources (e.g., shale gas, sand sands oil, etc.), and difficulties in accessing financing due to the limited technology maturity and market penetration. These influenced the competitiveness of PV in large-scale power generation systems, turning it into an attractive alternative to CSP. This has been felt in the US, where large projects such as the Blythe Solar Power Project have been downscaled and solar PV substituted for CSP. Likewise, in India, many of the CSP projects awarded under phase 1 of the JNNSM have experienced delays partially due to continuing cost confusion and the rise of solar PV as a competitive alternative. The levelized cost of electricity generated from CSP plants was estimated by the Department of Energy's Sunshot Program to be US$210/MWh in 2010, falling to $130/MWh in 2013 and projected to fall to $60/MWh (or 6 cents per kWh) by 2020.Footnote20
While CSP costs have been dropping significantly, the capital costs for solar PV plants also have dropped by half in just 4 years. The steep cost reductions will be driven by market opportunities, which require further expansion of today's market and the creation of new opportunities on a global scale, contributing positively to the reduction in CSP prices. The Crescent Dunes project in the US is accompanied by a power Purchase Agreement costed at US$135/MWh – a level which sets the benchmark for costings over the next decade. The Crescent Dunes project also features heat storage with molten salts – now emerging as the new industry standard.
Why CSP with molten salts is the way forward
Do the internal cost and external market considerations discussed above mean that CSP will be defeated, after all, by alternative renewables such as concentrated solar PV? We do not think so. The reason is that solar thermal power has the huge advantage (for grid-connected power generation) of dispatchability. This means that such plants can deliver power as and when it is needed – in the same way that conventional baseload power operates.Footnote21
CSP with molten salt technology has the decisive advantage that it delivers baseload, regular power. The key fluctuation with solar power has always been that it is available only during the day, and requires some kind of storage system to carry through to other times. But CSP with molten salt technology produces heat that lasts for 15 hours or beyond, and can be used to generate electric power or industrial heat continuously – and at industrial-scale levels of 100 MW to 1 GW that, when aggregated, is more than enough for baseload power. In this way, gas-fired turbines as back-up would no longer be needed. The idea that solar energy is too diffuse comes from early critiques of solar PV power by some physicists; but concentrated solar power meets that charge head-on by explicitly concentrating the power, thus combating diffuseness. CSP with molten salts (referred to in China as a second-generation technology) has already resolved the issue, and one can only anticipate rapid development as a result. The Chinese CSP literature is already referring to third- and fourth-generation technologies (viz. hot air or gases as HTF and solid state heat conservation systems).Footnote22 We feel that we are on firm ground in predicting that CSP with molten salt thermal storage is on track to become the dominant technology in CSP, with dramatic cost reductions likely to follow.Footnote23
CSP and water requirements
CSP plants require significant amounts of water in order to cool steam turbines and to wash the heliostat arrays. On average, typical CSP plants need as much as 3500 L of water per MWh generated. This compares to 2000 L/MWh for new coal-fired power plants and 1000 L/MWh for more efficient natural gas combined-cycle power plants. A June 2009 Congressional Research Service paper titled “Water issues of concentrating solar power (CSP) electricity in the US Southwest” commented on this issue: “Available freshwater-efficient cooling options…often reduce the quantity of electricity produced and increase electricity production costs, and generally do not eliminate water resource impacts” [Citation121]. However, the problem of the lack of a fresh water supply for CSP plants is now being addressed across a range of innovations. In the United Arab Emirates, for example, Masdar was able to recycle waste water from a nearby sewerage treatment plant and use it as source for wet cooling of its 100-MW Shams 1 parabolic trough development. The leading US contender, BSE, is constructing the world's largest CSP plant with a 370-MW facility at Ivanpah (California), aiming to minimize water consumption. With an air-cooled condenser system, some companies in the US as well as in Spain have announced that they can reduce water usage by more than 90% compared to conventional wet cooling systems [Citation122]. Therefore, we judge that water requirements will not constitute a limiting resource for CSP expansion.
CSP and land requirements
It is important to be precise as to the land requirements needed for commercial CSP plants. The benchmark for CSP is currently the Shams1 plant rated at 100 MW, built in Abu Dhabi and opened in March 2013. The plant concentrates the sun's energy using a field of parabolic trough mirrors covering 250 ha, or 2.5 km2. This plant incorporates many innovative features, including a dry-cooling system to save on water consumption in the desert, and auxiliary burners to provide dispatchable power. But its bare essentials are that it generates power at a land “cost” of 2.5ha/MW, or 40 MW/km2.Footnote24 Scaling that up to 1 GW would require 25 km2; 5 GW would call for 125 km2. When placed in the world's arid and semi-desert regions (where most of the CSP plants would in fact be located), it would be a very small square in a very large area.
Take a country like Taiwan, which is presently almost totally committed to fossil fuels and nuclear power for its energy needs. A good part of Taiwan's power needs could be met over the next 5 years by the construction of 5 GW of CSP, which (according to the Shams1 plant benchmark) would call for no more than 125 km2 – which is negligible given the nation's total land area of 32,260 km2, and comparable to the land currently devoted to science and technology parks. CSP plants operating with molten salt heat storage could easily generate all the power currently produced by the existing nuclear plants operating in Taiwan, as well as generating energy 24 hours a day, 7 days a week, in a way that is infinitely more reliable and safe than nuclear plants. The key point of this approach is that it would catapult Taiwan (for example) into a world leader position in CSP technology and equipment supply, first for national use and then to the rest of the world.
Technology trajectory and patenting trends
One can see two upticks in interest in CSP technologies from the patenting record during the three decades from 1980 to 2011. A first peak occurred in the early 1980s, due largely to the oil crisis of the 1970s. The second patenting surge started in the early 2000s as part of the shift to renewables catalyzed by concerns over global warming (and, in China's case, concerns over energy security). There was a marked increase in 2007–2008, which continued thereafter ().Footnote25
Figure 3. Global concentrating solar power patenting trends, 1980–2011. Source: EPO Espacenet worldwide patent database, compiled by the authors.
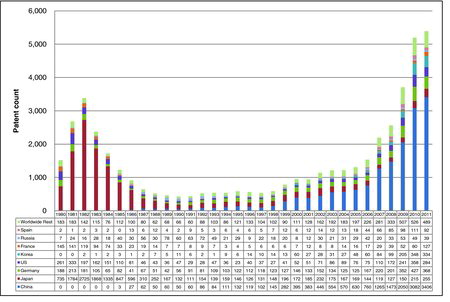
Even more interesting is the analysis of countries involved in this patenting surge. According to our analysis, China, which is (perhaps surprisingly) the leader, was granted 16,387 CSP patents in the period 1980 to 2011 (out of 48,365), according to Espacenet (the European worldwide patent database) – or one third of all CSP patents taken out so far. China is followed by Japan (14,171 patents), Germany (4627), the US (3440), South Korea (1139), Russia (1057, including the former Soviet Union) and Spain (675). These are the countries that are clearly looking to make their mark in the anticipated new wave of CSP investments – China in particular. Clearly China does not intend to be just a “fast follower” in CSP, bringing technologies developed elsewhere to mass production and rapid cost reduction (as done for first-generation solar PV [Citation17]) but rather it intends to be at the cutting edge in developing the CSP technologies itself. European firms are also strong players, with Germany and Spain at the forefront of patenting. In contrast, although Japan acted as the most active patentee in the 1980s and is ranked at the second position in terms of total CSP patent stock, this country has not yet achieved as many accomplishments in the CSP industry as it has had in the solar PV industry.
Concluding remarks
While CSP has shown great promise as an alternative to thermal power stations, its development has been held back by the higher costs involved so far – albeit with full expectation that they will be significantly reduced as technology is diffused and commercialized. What has held back the development of CSP (as well as concentrated PV) has been the issue of energy storage and dispatchability – now solved, in the case of CSP, by the innovation of utilizing molten salts as the HTF, giving up to 15 or 16 hours of heat storage and the capacity to generate power right through the night – in other words, to generate power on a 24/7 basis. Therefore, the real development barrier of CSP is the relative cost of other forms of energy – the competitive cost. For example, the natural gas boom (particularly in the US) has placed more pressure on all forms of renewables. But CSP installations, like solar PV and wind installations, are made from components that are manufactured – and as such they benefit from all the advantages that accrue to manufacturing, such as enjoying increasing returns, as opposed to the diminishing returns associated with extractive activities. This is why CSP will continue to enjoy cost reductions as per the learning curve (along with solar PV and wind), thus reducing costs for renewables overall – while costs for fossil fuels, both traditional and alternative, remain highly uncertain [Citation18,19]. China and other latecomers (especially the Asian latecomers) have advantages in manufacturing and costs reduction; hence, we foresee the CSP industry evolving into a global competitive industry.
In this paper, we have sought to demonstrate that the growth barriers holding back development of the global CSP industry, in terms of costs, technologies and resources, have been circumvented. CSP with molten salt thermal storage, together with the new wave of large-scale investments in emerging economies (led by China), are likely to drive the next wave of CSP and solar power station development. The “Big Lie” that solar power cannot provide baseload and dispatchable power is about to be definitively refuted.
Future perspective
While CSP has shown great promise as an alternative to coal-fired power stations, its development has been held back by the higher costs involved so far. However, the growth barriers holding back development of the global CSP industry, in terms of costs, technologies and resources, have been circumvented. CSP with molten salt thermal storage, together with the new wave of large-scale investments in emerging economies (led by China), are likely to drive the next wave of CSP and solar power station development. Therefore, the real development barrier of CSP is the competitive cost of other forms of energy whereas the competitive advantage lies in the efficient management of the supply chain as a whole.
Notes
1 See the Intergovernmental Panel on Climate Change (IPCC) website for a recent overview of CSP in the context of other renewable technologies and their capacity to mitigate climate change effects of fossil fuel combustion [Citation101].
2 The heated fluid turns water into steam through a heat exchanger, and drives a turbine through the normal power cycle; or, in the organic Rankine cycle, the fluid itself drives the turbine, at lower temperatures suitable for industrial heating or desalination applications.
3 The California Energy Commission (CEC) has approved the conversion of the Blythe Solar Power project in Southern California from CSP to solar PV. For details, see [Citation102].
4 On recent developments involving CSP, see Lilliestam et al. [Citation1] for a review, comparing CSP with CCS (carbon capture and storage); Chien and Lior [Citation2] on prospects for CSP in China; and Vallentin and Viebahn [Citation3] for a review of German contributions to the development of CSP value chains. The NREL (National Renewable Energy Laboratory) Renewable Electricity Futures Study [Citation103] contains a section on CSP in Chapter 10, Solar technologies (Volume 2). The EASAC (European Academies’ Science Advisory Council) study [Citation104] on CSP seriously underestimates the contribution from China and other emerging markets to future CSP activities. The report by A.T. Kearney/ESTELA [Citation105] provides influential estimates of cost reductions up to 2025. As noted above, the IPCC report on Renewables contains a discussion of CSP in Section 3.3.
5 In an article in Asia Pacific Journal: Japan Focus, Andrew DeWit noted that several Japanese prefectures are commissioning new solar thermal (CSP) plants to take over from nuclear power [Citation4].
6 On the emergence of a dominant technology (dominant design) and its impact on competitive dynamics, see Utterback and Suarez [Citation5].
7 The technology has been scaled up through the joint public–private Archimede project, where a 15-MW facility has been established at Priolo Gargallo near Syracuse, in Sicily; it is generating power from an array of parabolic mirrors focusing the sun's rays on a pipe carrying molten salts that runs around the field, and storing the heat in an energy storage unit from which steam is produced that turns a generator to produce electricity. The project was jointly developed by ENEA and Archimede Solar Energy, itself a joint venture (JV) between the Italian industrial engineering firm Angelantoni Industrie and Siemens Energy. See Piemonte et al. [Citation7] for an overview of the Archimede project.
8 This plant is operated by a JV company involving Masdar from Abu Dhabi and SENER, the Spanish engineering and construction firm. See [Citation107].
9 For example, there is a 12-MW (short term)/300-MW (long term) project–collaboration between Xinjiang Qingsong Building Materials and Chemicals (Group) Co. and Guodian Xinjiang Company; a 50-MW project in Tibet by Huaneng Tibet Company; a 100-MW project in Golmud by GD ENERGY; a 100-MW project in Ningxia by Beijing Control Technology Co. Ltd.; a 100-MW project (TBD) by Avic Xi’an Aero-Engine (Group) Ltd.; a 100-MW project (TBD) by Guangdong Kangda; a 100-MW project in Gansu by SETC Tianjin; a 1000-MW project in Qinghai by Lion International Investment Ltd.; and a 2000-MW in Shaanxi by Shandong Penglai Dianli and eSolar [Citation109].
10 See [Citation112]. This came shortly after another US company, First Solar, struck a 2-GW deal to build solar PV farms in China.
11 In August 2011, it was announced that General Electric (GE) would invest US $40 million in eSolar. China Shandong Penglai Electric Power Equipment Manufacturing has announced that they will be developing solar thermal plants using eSolar's technology with investments totaling RMB$5 billion.
12 China's crude steel production is ranked number one in the world, with 46.3% of th global share in 2013, producing 716.5 million tons [Citation113]. China is also the largest glass producer, accounting for over 50% of the global market, and concrete is an easily available construction material in China; for molten salts, China's production accounted for approximately 18% of the global market in 2013 [Citation8].
13 We are somewhat skeptical as to the prospects for this goal being realized. See [Citation113].
14 Lanco Solar is developing multiple solar PV and solar thermal CSP projects, largely under the JNNSM program: http://www.lancogroup.com/DynTestform.aspx?pageid = 87
15 On African developments and their links with adjoining regions, see recent reviews by Pitz-Paal et al. [Citation10] and Py et al. [Citation11].
16 Our reporting of approximately 25–30GW as a (conservative) target for CSP development by 2020 may be compared with the “optimistic–realistic” scenario of Viebahn et al. [Citation12] which predicts a total of 29 GW by 2020.
17 This South African solar park would set the standard for such developments in other countries. There is a debate underway in Taiwan, for example, to substitute renewables for the country's 5-GW nuclear plant fleet; see [Citation115].
18 The 100-GW global projection by 2025 is made by A.T. Kearney and the European Solar Thermal Industry Association, in their investment report Solar thermal electricity 2025 [Citation105]; it is noteworthy that Bill Gross, the founder of eSolar (a key proponent of CSP), is on record as saying that the world needs 2 TW of CSP – or 2000 times current levels [Citation116].
19 International Renewable Energy Agency (IRENA) made its own assessment in a report issued in early 2012 where it estimated that installed costs in 2011 were $4.60/W for parabolic trough technology and $6.30 to $7.50 for power tower technology with 6 to 7.5 hours of heat storage [Citation118].
20 See “Concentrating solar power” at Argonne National Laboratory website: http://energy.gov/eere/sunshot/concentrating-solar-power
21 The CSP Alliance reported that CSP with thermal storage is one of the few studies to highlight the role of heat storage in changing the cost dynamics of CSP [Citation119].
22 The papers referring to third- and fourth-generation CSP technologies are as yet only available in Chinese. On the use of hot CO2 in power generating units of CSP plants, utilizing the Brayton Cycle, see the NREL report, 10 MW super- critical CO2 turbine test – Thermodynamic cycle to revolutionize CSP systems‖ at: http://www.nrel.gov/csp/supercritical_co2.html
23 Comparable analyses are provided by recent contributions including Dunn et al. [Citation13]; Romero and Gonzalez-Aguilar [Citation14] and Burkhardt et al. [Citation15].
24 We note that McKay [Citation16] assumes a lower efficiency and therefore a higher land cost for concentrated solar power. On p. 178, he asserts: “Concentrating solar power in deserts delivers an average power per unit land area of roughly 15 W/m2.” This is 15 MW/km2 – compared with the real power output of the Shams 1 plant of 40 MW/km2.
25 is constructed from an initial survey of CSP patenting results using patent classifications searching in the Espacenet patent database (organized by the European Patent Office). Espacenet offers free access to more than 80 million patent documents published by over 90 global patent offices, including the European Patent Office (EPO), United States Patent and Trademark Office (USPTO), Japan Post Office (JPO), and China's State Intellectual Property Office (China) (SIPO). Through Espacenet, the EPO has made a particular effort to make sustainable technologies available to public viewing, through the Y02 E patent classification, and in particular the Y02E10/ set of technologies, describing energy generation utilizing renewable energy technologies. The list of patent classifications used to retrieve the global CSP-relevant patents for this study, along with the definitions of these patent classifications, is provided in Appendix A.
References
- Lilliestam J, Bielicki JM, Patt AG. Comparing carbon capture and storage (CCS) with concentrating solar power (CSP): potentials, costs, risks, and barriers. Energy Policy 47, 447–455 (2012).
- Chien JC-L, Lior N. Concentrating solar thermal power as a viable alternative in China's electricity supply. Energy Policy 39(12), 7622–7636 (2011).
- Vallentin D, Viebahn P. Economic opportunities resulting from a global deployment of concentrated solar power (CSP) technologies: the example of German technology providers. Energy Policy 38(8), 4467–4478 (2010).
- DeWit A. An emerging Fukushima model? Asia–Pac. J. 11(19), No. 1 (2013).
- Utterback JM, Suarez FF. Innovation, competition and industry structure. Res.Policy 22(1), 1–21 (1993).
- CSP World interactive map available at: http://www.csp-world.com/cspworldmap
- Piemonte V., De Falco M, Tarquini P, Giaconia A. Life cycle assessment of a high temperature molten salt concentrated solar power plant. Solar Energy 85(5), 1101–1108 (2011).
- CSP Today market report 2014. Chapter 9: China, pp.257–297. CSP Today, London, UK (2014).
- World Bank Energy Sector Management Assistance Program. Development of local supply chain: the missing link for concentrated solar power projects in India. ESMAP, Washington DC (2014).
- Pitz-Paal R, Amin A, Bettzuge MOet al. Concentrating solar power in Europe, the Middle East and North Africa: a review of development issues and potential to 2050. J. Sol. Energy Eng. 134(2), 0245011–0245015 (2012).
- Py X, Azoumah Y, Olives R. Concentrated solar power: current technologies, major innovative issues and applicability to West African countries. Renew. Sustain. Energy Rev. 18, 306–315 (2013).
- Viebahn P, Lechon Y, Trieb F. The potential role of CSP in Africa and Europe: a dynamic assessment of technology development, cost development and life cycle inventories until 2050. Energy Policy 39(8), 4420–4430 (2011).
- Dunn RI, Hearps PJ, Wright MN. Molten-salt power towers: newly commercial concentrating solar storage. Proc. IEEE 100(2), 504–515 (2012).
- Romero M, Gonzalez-Aguilar J. Solar thermal CSP technology. WIREs Energy Environ. 3, 42–59 (2014).
- Burkhardt JJ, Heath GA, Turchi CS. Life cycle assessment of a parabolic trough concentrating solar power plant and the impacts of key design alternatives. Environ. Sci. Technol. 45(6), 2457–2464 (2011).
- McKay DJC.Sustainable energy – without the hot air. UIT, Cambridge (2009).
- Wu C-Y. Comparisons of technological innovation capabilities in the solar photovoltaic industries of Taiwan, China, and Korea. Scientometrics 98(1), 429–446 (2014).
- Mathews, JA, Tan, H. Manufacture renewables to build energy security, Nature, 513, 166–168 (2014).
- Mathews, JA, Reinert, E. Renewables: manufacturing, increasing returns, energy security, Futures, 61(Sep), 13–22.
Websites
- Special Report on Renewable Energy Sources and Climate Change Mitigation. Intergovernmental Panel on Climate Change (IPCC). http://srren.ipcc-wg3.de/
- California gives the green light for switch of Blythe solar CSP project to PV. Solar Server. www.solarserver.com/solar-magazine/solar-news/current/2014/kw03/california-gives-the-green-light-for-switch-of-blythe-solar-csp-project-to-pv.html
- Renewable electricity futures study. National Renewable Energy Laboratory (NREL). www.nrel.gov/analysis/re_futures/
- Concentrating solar power: its potential contribution to a sustainable energy future. Halle (Germany): The European Academies Science Advisory Council (EASAC). www.easac.eu/fileadmin/Reports/Easac_CSP_Web-Final.pdf
- Solar thermal electricity 2025. A.T. Kearney/European Solar Thermal Electricity Association (ESTELA). www.estelasolar.eu/fileadmin/ESTELAdocs/documents/Cost_Roadmap/2010-06%20-%20Solar%20Thermal%20Electricity%202025%20-%20ENG.pdf
- Breakthrough solar technology beats estimates by a large margin. PRWeb. www.prweb.com/releases/2013/8/prweb11001473.htm
- Energy plant makes a leap in solar power. New York Times. www.nytimes.com/2011/10/26/business/energy-environment/energy- plant-makes-a-leap-in-solar-power.html
- China to install 3,000MW solar thermal power by 2015. China Daily. www.chinadaily.com.cn/business/2012-11/22/content_15950340.htm
- China to have more 3GW of concentrated solar thermal power (CSP) by 2020. Clean Technica. http://cleantechnica.com/2012/ 03/30/china-to-have-3-gw-of-concentrated-solar-thermal-power-csp-by-2020/
- ADB to finance Qinghai Delingha CSP plant project in China. CSP World. www.csp-world.com/news/20131205/001270/adb-finance-qinghai-delingha-csp-plant-project-china
- BrightSource, Shanghai Electric plan China solar projects, Bloomberg, Nov 10, 2014, at: http://www.bloomberg.com/news/2014-11-10/brightsource-shanghai-electric-plan-china-solar-projects.html
- Pasadena's eSolar lands 2000-megawatt deal in China. LA Times. http://articles.latimes.com/2010/jan/09/business/la-fi-solar9-2010jan09
- Steel statistical yearbook 2013. World Steel Association. www.worldsteel.org/dms/internetDocumentList/statistics-archive/yearbook-archive/Steel-Statistical- Yearbook-2013/document/Steel-Statistical-Yearbook-2012.pdf
- Why India will be a major player in CSP. Renewable Energy World. www.renewableenergyworld.com/rea/news/article/2011/01/why-india-will-be-a-leader-in-csp
- Dust clouds, delays thwart India's $1.4 billion solar plan. Bloomberg BusinessWeek. www.businessweek.com/news/2012-11-15/india-s-1-dot-4-billion-solar-plan-thwarted-by-supply-delays- dust
- China key to Taiwan energy crisis. Taipei Times.’ www.taipeitimes.com/News/editorials/archives/2013/04/15/2003559647
- eSolar secures deal for China's largest concentrated solar power plant. BusinessGreen. www.businessgreen.com/bg/news/1807075/esolar-secures-deal-chinas-largest-concentrated-solar-power-plant
- Abengoa wins first CSP tender in Chile, will build a 110 MW tower plant to generate around the clock. CSP World. www.csp-world.com/news/20140109/001295/abengoa-wins-first-csp-tender-chile-will-build-110-mw-tower-plant-generate
- Renewable energy technologies: cost analysis series. Abu Dhabi (UAE): International Renewable Energy Agency (IRENA). www.irena.org/DocumentDownloads/Publications/RE_Technologies_Cost_Analys is-CSP.pdf
- The economic and reliability benefits of CSP with thermal energy storage. Concentrating Solar Power Alliance (CSPA). www.csp-alliance.org/wp-content/uploads/2012/12/CSPA-Report-Dec-2012-Ver1.0.pdf
- Water issues of concentrating solar power (CSP) electricity in the US Southwest. Congressional Research Service. www.circleofblue.org/waternews/wp-content/uploads/2010/08/Solar-Water-Use-Issues-in-Southwest.pdf
- Cutting water consumption in concentrated solar power plants. CSP World. www.csp-world.com/content/00925/cutting-water-consumption-concentrated-solar-power-plants
- Concentrating solar Power. The US Department of Energy (DOE) http://energy.gov/eere/sunshot/concentrating-solar-power
- 10-Megawatt Supercritical Carbon Dioxide Turbine test - Thermodynamic Cycle to Revolutionize CSP systems. National Renewable Energy Laboratory (NREL). 10 MW super- critical CO2 turbine test – Thermodynamic cycle to revolutionize CSP systems at: http://www.nrel.gov/csp/supercritical_co2.htm