Abstract
Carbon dioxide removal (CDR) is a necessary complement to emissions reductions to achieve a state of global net zero emissions and stabilise future warming. Despite its utility, CDR remains poorly understood. Due to a range of constraints, CDR is a fundamentally finite resource, which we currently do not have enough of to achieve and go beyond global net zero. This has wide-ranging but underexplored implications for the technical and economic feasibility of our collective net zero transition. At the same time, both the opportunity and obligation to undertake CDR are not equally distributed amongst actors and geographies. As a result, there are ongoing questions as to how we can increase the supply of quality CDR whilst at the same time ensure equitable distribution of that same CDR, both within and between countries and non-state actors. To explore these phenomena, we introduce and define the concept of a Carbon Removal Budget (CRB), illustrate how it can apply to different contexts and scales, and distinguish it from the related but distinct concept of the carbon budget. We further estimate the global CRB, review its constraints and quality considerations and outline potential utilisation pathways and principles. We then examine the potential application of the CRB as a tool on which both public and private decision-makers can use to assess the feasibility of their nationally determined contributions and/or net-zero transition plans. In this manner, we illustrate how CRB forecasts can be used today to help build the net zero future of tomorrow.
Introduction
Net zero is the defining paradigm of climate governance today. The “zero” element requires reducing emissions as much as possible, while the “net” element refers to anthropogenic removals required to neutralise the residual hard-to-abate emissions. Anthropogenic removals, in turn, can be defined as the “withdrawal of greenhouse gases (GHGs) from the atmosphere as a result of deliberate human activities” [Citation1,p.1795]. As methods to remove the full range of other GHGs remains nascent, the vast majority of anthropogenic removals focus on carbon dioxide removal (CR) [Citation1,p.1261]. Such CDR could include improving existing biological sinks, such as afforestation and wetland restoration as well as more novel methods such as direct air capture and storage (DACCs), bioenergy carbon capture and storage (BECCS) and ocean-based CDR techniques [Citation1, p.1795].
Active stewardship of the nascent CDR industry is essential to scale it to the quantities needed to reach net zero. Public and private actors alike currently lack the tools to adequately gauge the available supply of CDR and make the necessary investments to build out its future potential. Currently, most carbon is removed through passive natural sequestration in the biosphere, which can and should be distinguished from anthropogenic CDR [Citation2]. But how much anthropogenic CDR is possible, over what time period, and with what level of certainty? Therefore, it is imperative to establish what could constrain or grow CDR supply over time. A finite supply of CDR also raises a number of equity issues. How should access to CDR be allocated? To whom, and on what terms?
Towards this end, this article introduces and defines the Carbon Removal Budget (CRB): a conceptual tool that can be used to estimate, allocate and manage the finite global potential of CDR. Section “The role of carbon removal in achieving global net zero” provides context for the analysis by reviewing the global goal of net zero, the role of CDR within it, and how budgeting approaches can help lend clarity as to meeting it. Section “Introduction to the Carbon Removal Budget” defines the CRB, illustrates the scales and contexts it can apply to and considers its limitations. Section “Sizing the global Carbon Removal Budget” models three Paris-aligned CRBs and explores their interrelationship with their corresponding global carbon budgets. Section “Growing the global Carbon Removal Budget” reviews the constraints on quality CDR and considers levers to grow the global CRB. Section “consuming the global Carbon Removal Budget” considers the various demand pressures on the global CRB, potential utilisation pathways to develop the CRB and the principles through which it could be equitably allocated. Section “The implications of Carbon Removal Budgeting” explores the potential applications and implications of removal budgeting for countries and companies alike. Section “Conclusion” summarises and offers direction for future research.
The role of carbon removal in achieving global net zero
Net zero requires us to take significant near-term steps to reduce emissions and scale CDR. As this section explores, while a budgeting approach has long been applied to steward the former, it is comparatively novel in conceptualising the latter.
The global goal of net zero
The IPCC defines net zero carbon dioxide as a state in which “anthropogenic carbon dioxide (CO2) emissions are balanced by anthropogenic CO2 removals over a specified period” [Citation1,p.1809]. The notion of net zero as a global goal can be found in Article 4(1) of the Paris Agreement, which states the Parties’ intention to achieve a balance between the sources and sinks of GHGs by mid-century [Citation3]. Just as the Paris Agreement establishes net zero as a global goal, it also recognises that it is a goal that can only be achieved through commitments from a range of individual actors [Citation3: preamble]. To this end, it is significant that 151 countries have now set some form of net zero targets to the extent that 88% of global emissions, 92% of GDP and 89% of the global population are now covered [Citation4]. Various non-state actors have also set net zero targets in parallel, including through the United Nations’ Race to Zero Campaign, whose membership now exceeds 11,300 + [Citation5]. The last decade has, therefore, seen a growing awareness of the importance of reaching net zero in combination with a proliferation of net zero commitments from a wide range of state and non-state actors.
Both emissions reductions and CDR are essential to achieving these net zero commitments. To this end, the mitigation hierarchy is a concept central to determining the appropriate levels of CDR required to achieve and go beyond net zero in a sustainable manner. It requires first avoiding your emissions, before taking steps to reduce and ultimately compensating for any residual emissions, including through CDR. Reaching net zero requires identifying the ongoing activities that will generate ongoing hard-to-abate residual emissions that require CDR abatement [Citation6]. It is the view of some that steps taken to enhance the deployment of compensatory CDR may threaten other avenues of climate mitigation, including emissions reductions [Citation7] and net-negative emissions [Citation6]. For this reason, there are calls for some CDR to be generated to reach minimum levels of CDR needed to achieve climate goals sustainable development [Citation6] and alternatively for CDR “to complement rather than replace ambitious mitigation policies before mid-century” [Citation7]. Governments have signalled that CDR has a role in accelerating near-term mitigation prior to the achievement of a net zero target, and subsequently in achieving net-negative emissions–still the focus appears to be initially on compensating for residual emissions rather contributing to drawdown [Citation8]. As a result, notwithstanding the risk of potential mitigation deterrence, it is well accepted that there is an imperative to scale CDR.
Carbon dioxide removal
CDR provides the net in net-zero. There are several techniques to enhance natural sequestration or chemical absorption of CO2, all of which constitute CDR. Conventional CDR tends to enhance the natural ability of carbon sinks to sequester carbon and could include projects that undertake afforestation & reforestation (AR) or peatland and wetland restoration. Novel CDR, in contrast, includes a diverse range of technological solutions that work to remove and store carbon. Such techniques include direct air carbon capture and storage (DACCS) and ocean electrolysis. Between these two poles lie CDR techniques that harness both conventional and novel processes to capture and store CO2, for example, enhanced weathering, biochar and bioenergy and carbon capture and storage (BECCS). Each technique has limitations and trade-offs, including uncertainty over its effectiveness, making it potentially risky to rely on a select few CDR techniques [Citation9,Citation10]. Still, many integrated assessment models are only beginning to consider broader CDR portfolios in their projections [Citation8].
While a diverse portfolio of CDR offers the best chance of scaling CDR to meet net zero, actors can face challenges doing so due to constraints on the permanence, scalability, and affordability of CDR [Citation11]. Fundamentally, there is a finite capacity to store carbon within the biosphere (terrestrial and oceanic sinks) and lithosphere [Citation12]. Even if there is capacity to store carbon, how long that carbon is stored is also important. Depending on the timescale in question, all carbon sinks ultimately re-release CO2 as part of the varying carbon cycles. Yet some carbon sinks face a more ready risk of reversal. Mitigating this risk of reversal requires significant and ongoing governance and financial resources, which has attendant consequences for the choice of CDR undertaken [Citation13]. Conventional CDR tends to rely on storage in carbon stocks that have a high risk of reversal over the course of the century. That said, through good governance and replenishment it may still be possible to achieve a state of “dynamic permanence” among such stocks [Citation14].Footnote1 This is necessary to shift such options from being “CDR-capable’ interventions to being actual CDR [Citation6].
Novel CDR tends to have a lower risk of reversal over the next century. Indeed, in some circumstances, such techniques can store CO2 in excess of 10,000–100,000 years [Citation2,p.15]. Yet, like conventional CDR, such CO2 stores also require monitoring–at least in the near-term– to evidence that leakage risks are low [Citation15,Citation16]. Consequently, both the cost of the initial CDR and its ongoing storage present an additional constraint on the feasible CDR supply. The cost per ton of CDR cost can fluctuate significantly– ranging from less than USD $1/tCO2 for some conventional CDR projects up to USD $1610/tCO2 for the most complex novel CDR [Citation17,Citation18]. Oftentimes actors that seek to purchase CDR for offsetting purposes require thousands–even millions–of tons at a time to address their ongoing emissions. The significant cost of high-quality CDR in the significant volumes it is often needed can thus make it prohibitively expensive for many actors to access. At the same time, the technical feasibility of scaling CDR also remains a challenge. Various resource inputs are needed to set up viable CDR projects, from land availability to electricity and industrial equipment. It can take time for ecosystem restoration efforts to be fully effectual and for the infrastructure to enable subterranean storage of CO2 to be constructed. All of these factors contribute to a finite supply of conventional and novel CDR available at any one time. In turn, this requires careful forecasts of both CDR supply and demand to ensure that CDR potential is both developed and utilised.
Budgeting approaches
A state of net zero could theoretically be achieved at any point in the future where sufficient CDR to balance residual emissions was deployed. Yet to influence the overall warming signal, it matters what timescale this CDR occurs for and the degree to which it is paired with emissions reductions [Citation19]. To this end, the carbon budget has been a vital concept to model the relationship between cumulative emissions and temperature increase [Citation20]. Carbon budgets describe the “total quantity of CO2 emissions that is consistent with remaining below a given level of global temperature” [Citation21, p.3]. In 2021, the IPCC set out three carbon budgets that illustrated the cumulative emissions that we need to stay within to avoid breaching the Paris Agreement’s temperature goal of “well below 2 °C” [Citation3: Art 2(1)(a)]. The carbon budgets to achieve a temperature target of 1.5 °C, 1.7 °C and 2 °C with a probability of 67% from 2020 are 400, 700 and 1150 GtCO2 respectively [Citation22, p.29]. These carbon budgets present clear developmental choices, both in terms of what end temperature is in sight as well as how the trade-offs between near-term emissions reductions and the longer-term potential of CDR will be managed. Fundamentally, they illustrate that the more we emit, the faster the remaining carbon budget will be utilised. The overreliance on future CDR in climate mitigation strategies can threaten vital near-term emissions reductions [Citation23]. Such approaches also confirm that our ability to reduce CO2 is far less constrained than our ability to remove it. Therefore, while the carbon budget provides a useful tool for identifying appropriate timeframes to reach net zero, more specific attention needs to be paid to the supply and demand levels of CDR required to achieve it.
Budgeting approaches clearly offer merit in helping coordinate necessary actions to reach net zero. Although there is a clear understanding of the utility of carbon budgets in this context, there is not yet the same clarity as to the role of CDR within them. Employing a CRB in parallel to a carbon budget could change this, both adding rigour to the mitigation calculus and presenting a clear case for equitable investment in and use of a finite removal supply. A CRB could help make microeconomic decisions about the balance between emissions reduction and CDR at the entity level to reach net zero; in turn, revealing the necessary near-term investments in CDR supply needed today to offset tomorrow’s residual emissions. A CRB could likewise help calibrate macroeconomic decisions by countries to stimulate the development of the requisite regulatory and fiscal infrastructure to ensure that sufficient CDR is available in the time frames needed in the future. As a result, the CRB is a tool that could be used to provide decision-useful guidance and guardrails to inform the net zero transition of public and private actors alike.
Introduction to the Carbon Removal Budget
This section builds on calls to develop a realistic CDR budget across all CDR methods [Citation24]. Specifically, we propose a CRB that can be used to help forecast supply and demand for CDR in line with temperature targets. We first define the CRB and its key elements. We then illustrate how the CRB can be downscaled to a micro-level and upscaled to a macro-level as appropriate in order to render decision-useful insights. Yet, as with most tools, it has some limitations which should also be considered.
Definition
We define the “carbon removal budget” as the “cumulative amount of carbon dioxide removal available to contribute to the realisation of global temperature goals”. The available CDR describe the total capacity for economically and technologically viable CDR at time T.Footnote2 For the purposes of the global CRB estimated in the next section, we assume that CDR available at <$100 USD per ton is economically viable at 2050 in line with the IPCC WG III’s projections.Footnote3 A second factor relevant to determining available CDR supply is their technological viability. We account for this by adjusting CDR economic potentials by their technological readiness levels (TRLs) through a “Realisation Rate”.Footnote4 This is important because while the economic viability and TRLs of different forms of CDR will improve with time, decisions about developing and using CRBs need to be taken today against a backdrop of uncertainty. CDR demand or consumption is the total volume of CDR that IPCC projections indicate will have already be used to reach a given temperature target and is therefore no longer part of the “available” CRB (See Section “Top-down: IPCC demand estimates”). The concept of “used” CDR implies that any conventional CDR is dynamically replenished, and so future capacity is adding to CDR deployment rather than replacing existing carbon stocks that may have seen reversals. Importantly, the CRB is calibrated to reflect a given temperature target which can and does requiring reaching net-zero, and in overshoot scenarios, moving into net-negative. As demonstrates functionally, the CRB thus equates to:
Figure 1. Simplified CRB Equation. NB: the CRB is expressed as a positive integer, whereas some models present CDR potential as a negative integer.
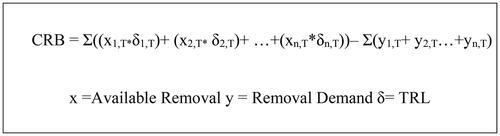
Given the extant uncertainties in forecasting a CRB, one should always indicate the range of uncertainty that it involves. It is important to gauge the full range of possibilities when exploring the range of CRB estimates. On the lower end, this involves comparing between high CDR demand/ lowest CDR supply scenarios [“MIN”], and on the upper end, the high CDR supply/ lowest CDR demand scenarios [“MAX”]. It is also important to appreciate that demand for CDR at a given price range ultimately enables its ability to scale. Indeed, the majority of CDR technologies to date lie above the threshold of $100 USD per tCO2 even when at scale, illustrating the need for access to CDR being provided investment at varied price points in order to realise the full potential of the CRB. That said, the threshold for economic viability can and should be calibrated depending on the context of the CRB and the time of deployment, especially as the cost of novel CDR remains significantly higher than conventional CDR.
The evolution of the global CRB can also be conceived in terms of fluxes of CO2 emissions and removals. presents a stylised demonstration of this for (1) CRB deficit (2) a neutral CRB (3) and a CRB surplus.
While net zero is often the first target, a surplus CRB can also be used to reach net zero and beyond to net negative scenarios [Citation6]. However, as the presence of any surplus remains highly speculative, and dependent on the extent (or lack of) emissions reduction actions, a potential future surplus in future should not be relied on as a safety valve.
Applications of the carbon removal budget
Budgets are tools for an effective allocation of scarce resources. With CDR being both a finite and essential resource, demand for it will likely exceed supply [Citation6]. This requires distributional trade-offs resulting in key questions about who has the right to access already feasible CDR as well as who bears the onus of developing further potential [Section “Consuming the global Carbon Removal Budget”]. For this distribution to occur in an efficient manner it is necessary to have a more granular overview of the CRB than possible at the global level. As shows through application to a range of contexts, CRBs can help tease out the specific role of actors as contributors to and users of the global CRB and the many interrelationships and interdependencies that exist in this regard. reveals the differing scales on which the CRB could be determined, illustrating how it can be attuned to aid decision-makers at a range of scales.
Table 1. Scale options for a CRB.
It is also possible to apply the CRB as a tool to explore trends geographically (e.g. comparing the CRBs of Country A and Country B), temporally (e.g. comparing the global CRB in 2020 with one in 2050) and by technology type (e.g. comparing the CRB for AR with one for DACCS). In this way, the inputs to a CRB can be calibrated in such a way as to have a range of potential contextual applications and, by extension, implications for CDR development. A multiscalar view is necessary because whilst most development and deployment is occurring at a ton for ton basis, this will need to change and evolve in future [Citation6]. Despite its potential utility in this regard, this is not to say that all actors will necessarily be willing to develop their own CRB or accept the modelled outcomes. Similar to the international treatment of carbon budgets, allocating a finite CRB could become highly politicised and informed by sometimes conflicting principles [Section “Consuming the Global Carbon Removal Budget”].
Limitations
There are limitations to estimating the CRB which should be borne in mind. There is nuance approximating the CRB in this manner, particularly regarding the risk of reversal. The reality today is that most CO2 is stored in stocks with a high risk of reversals. Yet, true net zero alignment requires a transition to CO2 storage in sinks with a lower risk of reversal [Citation25,Citation26]. A limitation of the model of the CRB this paper presents is that it is dependent on an assumption that conventional CDR will be replaced. There will also likely be a mismatch in demand and supply for both conventional and novel CDR resulting in under-utilisation of potential; for instance, by demand outstripping supply for lower-cost conventional CDR and supply outpacing demand for higher-cost novel CDR, as seen on the voluntary carbon market today.
At the same time, investment at a sufficient price point is also critical to unlocking the full extent of CDR, in particular, investment to bring down the cost per ton of novel CDR to reach the levels of deployment possible at $100 USD p/tCO2. The CRB modelling also assumes that all possible deployment will occur up to this threshold–and at scale. While there is evidence of some actors investing at a higher cost per ton basis for novel CDR, the absolute volume of this trend tends to occur on remains small compared to the much cheaper prospects for conventional CDR. The CRB is also subject to a lag in data availability, especially concerning the deployment of CDR, as well as its technological and economic viability.Footnote5 From a demand perspective, there are significant gaps in the inclusion of CDR in IPCC’s integrated assessment models, which limits the ability to develop a more granular CRB per CDR technique type [Citation9]. For example, no integrated assessment model currently includes ocean-based CDR techniques; instead tending to focus heavily on a more limited range of CDR techniques such as AR, BECCS and DACCs [Citation27]. Recognising these limitations, CRBs can and should be continuously reviewed as CDR supply and demand curves shift.
Summary
Devising a CRB has the potential to transform how we conceive the role of CDR, in relation to Paris-aligned temperature scenarios. The definition of a CRB identifies the elements intrinsic to how CDR are available and consumed, and how this may change over time as costs come down. At the same time, it provides a concept sufficiently flexible to be adapted to a range of scales and contexts, meaning it can generate insights for decision-makers. Even so, due caution must be had not to rely on CRB modelling in isolation from other input factors, especially as input factors are in a continual state of flux.
Sizing the global Carbon Removal Budget
This section sizes the global CRB for 202–2100 based on supply and demand projections of CDR across the range of Paris-aligned temperature goals: 1.5 °C with low to no overshoot, 1.5 °C with high overshoot and 2 °C based on a feasible price point of up to $100 USD/tCO2 [Supplementary Materials].
Bottom-up: viable removal supply
CDR techniques contribute different volumes of available CDR to a global CRB. The first step in calculating the global CRB is to aggregate the supply of viable CDR. To calculate global CRB, the IPCC estimate of 2050 supply volumes available at a cost of <$100 USD per tCO2 was used [Citation1,pp.751–791;1266–1268].Footnote6 Supply volumes were then calibrated to increase linearly from 2025 to reach their 2050 IPCC estimates. From this point on, both conventional and novel CDR techniques were modelled to stabilise at their annual rates in 2050 [Supplementary Materials]. These supply volumes of each CDR type were then adjusted using their respective TRLs to give a CRB Realisation Rate-adjusted CDR supply volume. This resulted in an overall annual CDR economically and technically feasible supply of 10 GtCO2 yr-1 (4–17 GtCO2 yr-1) in 2100 consisting of 5 GtCO2 yr-1 (2–9 GtCO2 yr-1) of feasible conventional CDR deployment and 5 (2–9 GtCO2 yr-1) of feasible novel CDR deployment.Footnote7 That said, almost all novel CDR units are priced above that on the current market, and factoring in the full cost of dynamic permanence [Citation11]. Therefore, significant investment is required to reach the full estimated supply to the global CRB that this section estimates.
Top-down: IPCC demand estimates
IPCC projections provide a number of temperature scenarios and pathways that reflect differing levels of CDR supply and demand. Such projections are becoming increasingly sophisticated, including in the degree to which they factor in the trade-offs between CDR and emissions reductions. For this reason, while they do not necessarily focus on the biophysical and geophysical limits of CDR deployment [Citation28], they can be used to forecast approximate CDR demand given different temperature targets. Shared socioeconomic pathways that reveal the range of policy choices could also be used to forecast an even greater range of CRBs in the future.Footnote8 Yet for this initial examination, we forecast the global CRB using the three major Paris-aligned projections used in Version One of the State of CDR Report: 1.5 °C with low to no overshoot, 1.5 °C with high overshoot and 2 °C [Citation2]. By 2100 this resulted in an annual CDR demand of 11 GtCO2 yr-1 (8–17 GtCO2 yr-1) under a 1.5 °C with low to no overshoot scenario, 17 GtCO2 yr-1 (13–21 GtCO2 yr-1) under a 1.5 °C with high overshoot scenario and 13 GtCO2 yr-1 (9–19 GtCO2 yr-1) under a 2 °C scenario.
Global CRB forecast 2025–2100
The sum of the estimated volumes of CDR supply and demand over the course of the next century resulted in an overall cumulative CRB forecast for 2025–2100 of − 49 GtCO2 (with a MIN/MAX range of −712 to 657 GtCO2) under a 1.5 °C with low to no overshoot scenario, −185 GtCO2 (with a MIN/MAX range of −772 to 560 GtCO2) under a 1.5 °C with high overshoot, scenario and 12 GtCO2 (with a MIN/MAX range of −627 to 716 GtCO2) under a 2 °C scenario as mapped in .
Figure 3. Global CRB cumulative forecast 2025–2100 under three Paris-aligned IPCC projections, with minimum and maximum range estimates. Source of Paris-aligned average IPCC C1-C3 projections [2, data annex].
![Figure 3. Global CRB cumulative forecast 2025–2100 under three Paris-aligned IPCC projections, with minimum and maximum range estimates. Source of Paris-aligned average IPCC C1-C3 projections [2, data annex].](/cms/asset/f8c902d9-0909-42fb-a2e9-10cc0174d663/tcmt_a_2374515_f0003_c.jpg)
CRBs can also be mapped at regular intervals, providing decision-useful information on the viability of CDR utilisation pathways [Section “Utilisation Pathways”]. As reveals, an annualised view suggests that the likelihood of a CRB deficit is significant in the latter half of the century–even when assuming maximum utilisation of all feasible CDR under $100 USD/tCO2.
Insights
We need CDR both before and beyond a net zero target date. All three global CRBs examined indicate annual deficits where the models already assume a higher volume of CDR being deployed than is already occurring at some stage. This reveals the need to continue efforts to rapidly reduce emissions. It further shows that IPCC scenarios are based on a higher quantity of CDR to be available in future than presently looks possible, requiring significant efforts to raise the levels of available CDR. The CRB forecasts presented here illustrate that the extent of this deficit differs significantly, revealing the significant latent uncertainties that remain concerning the economic and technical viability of CDR, and its ultimate deployment. Limiting temperature rise to 1.5 °C with overshoot places the greatest demand on CDR supply to remove excess CO2, thus presenting the greatest CRB deficit from 2025 to 2100. The only positive cumulative CRB between 2025 and 2100 is seen under the 2 °C scenario due to higher volumes of atmospheric CO2 predicted, and lower demand for CDR in turn. As all pathways are possible right now, the pre-2030 window will be the most critical for shaping future levels CDR supply and demand. Robust near-term decisions as to how to grow and allocate the CRB are in this way vital to enable more high-quality CDR options in the latter half of the century to reach and go beyond net zero.
Contrasts between the carbon removal budget and carbon budget
Actors should consider both carbon budgets and CRBs alongside each other in future. Although both budgets share similarities, there are important differences in their nature and, accordingly, how each should be interpreted. At a fundamental level, as shows–if managed appropriately– the CRB could grow to reach a cumulative surplus, while the remaining carbon budget shrinks. As reveals, intuitively when the CRB is in surplus, it can add an annual buffer to the carbon budget. Likewise, when the CRB is in deficit, the CRB further reduces the remaining carbon budget.
Figure 5. Stylised interplay between the carbon budget and the carbon removal budgets. Source of Carbon Budget Estimates [1,Table SPM.2]. Here the CRB surplus is presented as negative integer, and the forecast CRB deficit as a positive. One example of this relationship between the deficit and surplus is illustrated for the 2 degree pathway. However, it is important to note that the carbon budget envelope has continued to shrink since [Citation29].
![Figure 5. Stylised interplay between the carbon budget and the carbon removal budgets. Source of Carbon Budget Estimates [1,Table SPM.2]. Here the CRB surplus is presented as negative integer, and the forecast CRB deficit as a positive. One example of this relationship between the deficit and surplus is illustrated for the 2 degree pathway. However, it is important to note that the carbon budget envelope has continued to shrink since [Citation29].](/cms/asset/90082881-e425-43a6-9712-e8012abb5086/tcmt_a_2374515_f0005_c.jpg)
While carbon budgets and CRBs can be quantified using the same unit of tCO2 one should not draw false carbon, geographical, and temporal CO2 equivalencies [Citation30]. In a carbon budget, units of emitted CO2 are inherently fungible. This stands in contrast to units in a CRB, which have various characteristics, including durability and co-benefits, making them often inherently non-fungible [Citation31]. From a temporal perspective, due to the effect of cumulative radiative forcing, the reduction of one ton of CO2 is not the same as the later removal of that same ton. Gruberta and Talatib term the asymmetry between 1 tonne of CO2 emitted and removed as the “sufficiency” threshold [Citation6]. As a result, while the carbon budget provides a starting point for understanding the extent of CDR needed to reach a given temperature goal, it does not provide sufficient granularity on how to unlock the full potential of CDR to contribute to climate mitigation efforts. Likewise, the additional space (in instances of surplus) or constriction (in instances of a deficit) that a CRB adds to a carbon budget highlights that. Nevertheless, presenting the two together is a further reminder that there is much more available space to constrain emissions than to scale CDR.
Summary
Sizing the global CRB illustrates that there is a near-term CRB deficit, whereby the need for CDR outstrips forecasted deployment. This calls into focus the need to scale investment into CDR pre 2030. At the same time, any such efforts need to be contextualised by the relationship between the global CRB and the global carbon budget, in particular that it remains a greater window to reduce emissions than to remove them.
Growing the global Carbon Removal Budget
As the CRB forecasts presented in Section “Sizing the global Carbon Removal Budget” illustrate: our choice of emissions pathway has a direct correlation on CDR demand, and thus whether or not we are in a surplus or deficit from a CRB perspective. As illustrates CRB deficits are forecast through to mid-late century for all three major Paris-aligned temperature pathways. Yet even then this is assuming that all technically feasible CDR that is <$100 USD per tCO2 is being deployed between 2025 and 2100. Given that even this generous assumption as to what economic CDR will be available in future still tends to result in a CRB deficit, it is incumbent on global decision-makers to gain a precise overview of a feasible CRB and work to grow it over time. One option for this is undertaking swift emissions reductions, reducing the demand for CDR and extending the available carbon budget to avoid breaching a given Paris-aligned temperature goal. Yet efforts to reduce emissions on par with Paris-aligned scenarios continue to be slow. Scaling the supply of CDR must also be prioritised. Before one considers how to scale CDR, one must recognise that the quality of CDR units can vary greatly. At the same time, numerous constraints affect potential CDR supply. Examining these quality considerations and supply constraints, in turn, reveals the levers through which it may be possible to sustainably grow the global CRB in future.
Quality considerations
Unlike a carbon budget where one ton of CO2 is ostensibly fungible with another, the global CRB comprises of varying qualities of units.Footnote9 There are four key considerations for a quality CDR include its permanence, monitoring, verification, and reporting (MRV), additionality and co-benefits and risks.
Permanence
The major factor affecting the quality of a removal unit in a CRB is the permanence of the CDR in question. Low-quality CDR only temporarily stores carbon from the atmosphere, whereas high-quality CDR can store CO2 durably on timescales relevant to temperature stabilisation [Citation32]. Both conventional and novel CDR can contribute to the CRB, but to do so, conventional CDR has to be replaced when reversed to achieve dynamic permanence over time. Practices of using buffer pools and/or employing carbon insurance products can help protect against reversal risks [Citation33]. However, such practices also increase the cost of CDR, potentially making a given technique not economically viable [Citation13].
Robust monitoring, verification, and reporting
High-quality CDR is monitored, verified and reported. For conventional CDR, robust MRV can help minimise the risk that the sequestration abilities of a given natural carbon sink have been overestimated. In this way, MRV can help ameliorate the risk that an incorrect methodology or assumptions to estimate the CO2 a given project has taken up. Method-specific MRV tools and procedures can help combat these risks, resulting in a higher-quality CRB [Citation34,Citation35]. Yet such techniques are still under development, and more needs to be done to ensure the broader infrastructure relating to MRV is accessible for robust modelling of the CRB [Citation36]. It is also vital that MRV factors in the full lifecycle emissions of a given unit of CDR, and ultimately transitions to a system able to measure CDR volumes and fluxes on a gigaton rather than ton-for-ton basis [Citation6].
Additionality
CDR units must be additional. Determining additionality requires establishing a baseline and a rigorous counterfactual. For instance, financial additionality means that the CDR would not have been generated but for the finance provided by the investor–be they a government to achieve its nationally determined contribution or a company to achieve its net zero target [Citation6]. Additionality is particularly crucial for CDR that relies on land or oceanic-based carbon sinks where baselines and counterfactuals may be complex to establish [Citation37,Citation38]. Financial additionality can also be important to consider on the basis of potential prospects for utilisation of by-products, for example, biochar can be used as a fertiliser making it potentially cost-effective in its own right. For this reason, careful consideration of which types of CDR need investment–and to what extent–is fundamental to develop the CRB. Institutional frameworks are also important when establishing additionality: for instance, to ensure the CDR is additional to what otherwise has been or was committed to. It is important, particularly for novel CDR that additionality is considered on a lifecycle basis, as technologies that require electricity or significant transport can result in a significant carbon cost [Citation6].
Co-benefits & risks
While not necessarily for a quality CDR unit, an assessment of the co-benefits and risks of CDR provides essential context for their deployment. In the context of a CRB, co-benefits and risks are not distributed evenly amongst CDR types. Although the presence or lack of co-benefits and risks between CDR types should be factored into investment decisions [Citation7,Citation39] it should not deter investment. After all, there can be an inherent tension between co-benefits and other qualities such as permanence and additionality. As a result, suggesting that while the co-benefits and risks of a given unit of CDR should be weighed against another unit of the same CDR technique to ensure an “apples to apples” comparison of co-benefits and risks.
Constraints on removal supply
Beyond the considerations affecting the quality of a given unit of CDR, there are very real constraints on CDR supply more broadly [Citation1,Citation6]. The global CRB estimated in Section “Sizing the global Carbon Removal Budget” factors applies technical and economic constraints to availability. These are imperfect proxies for a far more diverse array of factors constraining feasible levels CDR potential. It is essential to consider the impact of geophysical, environmental, technological, economic, institutional and sociocultural limitations in turn:
Geophysical
There is a limited physical capacity for the lithosphere and biosphere to store carbon. This limit is reached far sooner in the latter case, due to persistent uncertainties relating to the feedback loops from terrestrial and oceanic sinks [Citation40]. Here physical climate change itself becomes a barrier, as could sudden alterations to the volume of global CDR being undertaken [Citation40]. By contrast, the lithosphere has a finite, albeit vast, ability to store carbon Yet, at the same time, it tends to require more inputs to store CO2 within it, for instance, pipelines that transport carbon from a BECCS point source to its final aquifer-based storage. The scarcity of these resource-based inputs thereby also presents a geophysical constraint on CDR potential. As a result, while there is likely no geophysical limit to our ability to store carbon geologically, there are still many geophysical constraints on CDR.
Environmental
Several environmental factors further constrain the CRB. CDR could also significantly impact biodiversity, for instance, if undertaking AR with single, non-native species [Citation24]. Some CDR techniques also threaten water quality and quantity and cause eutrophication, while others may generate toxic waste or require mining which has its own associated environmental risks. Air pollution can also result from some CDR techniques, for example, from fine particulate matter produced by enhanced rock weathering. As a result, the environmental impact of CDR strongly affects the tractability of a given approach, therefore presenting a constraint.
Technological
Several path dependencies affect the trajectory and effectiveness of CDR from a technological perspective. The IPCC recognises a full spectrum of TRL scores of CDR techniques exhibited from 1 (largely unproven) to 9 (well developed) [Citation1, pp.1275–1276]. With more than half of CDR techniques possessing a TRL of <5, technological feasibility presents another constraint. Some types of CDR have highly complex processes that depend on a number of resource inputs. Path dependencies arise from decisions regarding the construction of facilities, transportation networks, storage capacity, and the availability of necessary resources and materials. There is a similar live prospect of competition for these resources, including from other decarbonisation efforts [Citation6]. Due to these technological constraints, scaling up a given CDR technique efficiently can be challenging.
Economic
The level of market demand for CDR and its standalone economic viability are also significant path dependencies that constrain the CRB. Factors such as carbon pricing, market incentives, subsidies, and the cost of alternative mitigation options can influence the commercialisation and adoption of CDR. The availability of public and private sector investment, whether directly or indirectly through market mechanisms, can shape the economic viability of CDR. Similarly, while some CDR techniques generate by-products capable of economic use [Citation41] most do not. This results in highly variable pricing range estimates for CDR, making it difficult to attract crucial investment [Citation1, pp.1275–1276, Citation42]. For this reason, economics can be a major constraint on a CRB.
Institutional
The design and implementation of institutional frameworks also play a crucial constraint on the deployment of CDR. Policies can create incentives, support funding mechanisms, and establish regulatory frameworks that facilitate or hamper the adoption and deployment of CDR. On the one hand, at COP28 the first Global Stocktake recognised the need to scale up carbon removal [Citation43,28(e)]. At the same time, governance constraints in other areas of international regulation affect the development and deployment of some CDR, particularly novel variants. For instance, the Convention on Biological Diversity invites governments to ensure that “no climate-related geoengineering activities that may affect biodiversity take place until there is an adequate scientific basis on which to justify such activities” [Citation44,8(w)]. The nature and extent of government support and the alignment of policies with long-term climate goals can constrain the pace and scale of CDR. Political acceptance can be an additional constraint on CDR supply, as can the institutional, legal, and administrative capabilities necessary to deploy it.
Socio-cultural
Public awareness, acceptance, and perception of CDR are further dependencies related to CDR. Community acceptance, trust in technology, and understanding of the potential risks and benefits of CDR can all play a constraining role in this regard. This risk can be particularly acute if there is a real or perceived risk to human health or other unequal distributional impact, for example, the displacement of food production for CDR [Citation28].Footnote10 Given the location-based nature of CDR, socio-cultural constraints often have the greatest impact on the community level [Citation45]. CDR, particularly novel CDR, may also be co-located or enabled by oil and gas infrastructure which can further limit its perceived social licence to operate, presenting yet another constraint on available supply [Citation6].
Levers to expand CDR supply
As the CRB is essentially a product of the constraints on CDR, it is important to consider how these constraints can be addressed systematically to increase the global CRB. Expanding CDR supply requires the use of all available levers, some of which are more readily deployable than others [Citation46]. Research, Development and Innovation (RDI) and early-stage funding models are the most likely to grow the CRB in the near term. In the long term, building institutional capacity and infrastructure for CDR will equally be key.
Catalytic funding models
Fundamentally, while there are many CDR that are technically possible, many cannot be feasibly accessed due to price constraints. There tends to be convergence on $100 t/CO2 as a threshold that demonstrates economic feasibility, yet this masks a range of CDR techniques with varying levels of feasibility [Citation23]. The range of CDR techniques and the different contexts in which they are used lead to a menu of possible funding options to address the economic feasibility of CDR, including market and government-led options, or a combination thereof [Citation42]. A promising example of one such measure is advanced market commitments (AMCs), which seek to provide forward investment certainty for CDR developers. Although typically implemented by governments, the most significant AMC for CDR was initiated by the private sector, illustrating its dexterity as a catalytic funding mechanism.Footnote11 There is also the possibility of introducing CDR into compliance markets through integration with emissions trading schemes at national and regional levels. However, at present, such schemes are generally not well adjusted to the non-fungible nature of CDR compared to units of emission reduction. The purchase of carbon credits through the voluntary carbon market can also provide a flexible form of funding, a tool which emerging proposals show can be “net zero aligned” [Citation10]. However, there is also a non-offsetting alternative to CDR financing in the form of beyond value chain mitigation [Citation47]. The Milkywire Fund, which acts as a platform through which companies can invest in durable CDR outside the voluntary carbon market, provides one such example of this [Citation48]. This highlights that while private sector investment into CDR is typically linked to offsetting claims, it does not have to be–catalytic investment in all forms is required to scale CDR supply and thus grow the CRB.
Research, development and innovation
The level of RDI can significantly affect the path dependencies CDR faces. Collaborative research efforts and data sharing can accelerate technological advances, improve best practices, and inform policy decisions. CDR has already attracted some USD $4 billion in RDI funding to date, yet more is likely needed to reach the 1300x scaling of novel CDR Paris-aligned scenarios require [Citation2,p.11]. While RDI principally helps address technological constraints on CDR supply, it could also lead to more confidence in CDR in general, addressing sociocultural concerns in tandem. RDI could also lead to a further understanding of geophysical limitations on CDR, such as the availability and quality of lithospheric storage. In this way, it is possible that RDI can help address a wide range of constraints that prevent CDR deployment and thus limit the growth of the CRB.
Communities of practice
CDR presents complex risks and opportunities. Insights generated from RDI can prepare institutions to govern CDR, but without the institutional capacity to regulate CDR, decisionmakers are unlikely to be fully effective in this regard. Building institutional capacity first requires a sufficient evidence base for decision-making as well as the necessary support to implement it. A robust community of practice can be a means through which decision-makers can access both to develop institutional capacity. While there is early evidence of this occurring through the European Union’s carbon removal certification scheme, much CDR regulation remains nascent [Citation49]. Adequate governance of CDR will be crucial to scaling supply in the future, meaning that such communities of practice will only become ever more critical.
Removal infrastructure
CDR can require significant levels of human and physical infrastructure. Such infrastructure could be related to the CDR itself, as well as its broader transport and storage needs. Indeed, there are real-world examples that this long-term planning for CDR infrastructure is beginning to occur. The United States has, for instance, begun to develop CDR infrastructure by creating CDR “hubs” [Citation50]. The European Union and the United Kingdom are also setting up comparable “clusters” for carbon capture, use, and storage [Citation51,Citation52]. However, the timescales for pooled removal infrastructure in this manner can be lengthy, requiring considerable lead-in time. Therefore, the development of CDR infrastructure is likely to benefit the global CRB in the medium to long term.
Summary
There is an evident need to grow the global CRB. Doing so not only affords consumers more options to take up a diverse portfolio of high-quality CDR options, but it also helps ensure that CDR is available on the timescales and levels needed to reach global net zero. There are a range of constraints on the CRB that the above levers can help address. Technological readiness and economic feasibility remain the two main constraints on the CRB. To this end, it is natural that RDI and catalytic funding models remain the two primary levers to grow the supply of CDR in the near term. Improvements in these areas could help create a virtuous circle where an increase in buy-in from public and private investors improves investment certainty for CDR developers and reduces the marginal cost of CDR. In the longer term, institutional capacity and physical infrastructure development will also be essential to increasing CDR supply over time, particularly novel CDR. All in all, growing the global CRB is essential for enabling more choices as to its utilisation and consumption.
Consuming the global Carbon Removal Budget
The CRB is in continual flux as more feasible capacity is both added and utilised. While the absolute size of the global CRB throughout the twenty first century remains subject to much uncertainty, given that the CRB remains highly constrained in the near term under all temperature scenarios, it is vital to consider how the CRB is consumed. Disaggregating the global CRB from the perspective of its users reveals a complex patchwork of pressures on and opportunities from CDR demand. These factors translate into a range of potential utilisation pathways through which the global CRB could be managed. Having regard to these options, it is important to consider how the CBR may be allocated, including how the principles underpinning this assessment could differ from those applied to the global carbon budget [Citation9,Citation31,Citation50,Citation53]. Bearing these factors in mind enables us to now consider what an optimal allocation of the global CRB could look like [Citation54].
Factors driving CDR demand
At a macro level demand for CDR is driven by the need to reach and go beyond net zero emissions [Citation6,Citation55]. Globally some 150 countries, 147 regions, 252 cities and 939 of the largest companies have made net zero commitments [Citation18]. Despite this range of commitments, there has not been extensive evidence of emissions cuts necessary to achieve net zero goals [Citation56]. As a result, there is often an implicit and extensive dependence on CDR to abate residual emissions to reach net zero [Citation8]. Some countries have begun to evidence the scale of their CDR demand by signalling clear CDR targets in their nationally determined contributions and long-term strategies under the Paris Agreement [Citation8]. These countries, including the United States and several Nordic nations, have a comparatively high ability to pay for CDR, but also have contributed significantly to historical emissions.
By contrast, countries with comparatively low historical emissions but growing contemporary emissions–such as China and India– have not yet shown the same explicit demand for CDR [Citation8]. One can also consider this latent CDR from a sectoral perspective. Here it is evident that the oil and gas sector has made the most significant share of net zero commitments and thus has the greatest implicit or explicit reliance on CDR being widely available in the future, especially when considering their significant volumes of scope 3 emissions.Footnote12 However, it is the technology sector evidencing the greatest CDR demand, particularly for novel CDR [Citation53]. As a result, it is sectors with significant residual emissions, which are likely to need to consume the majority of the CRB, are often not the same generating CDR demand [Citation57]. This question is also highly salient given that such industries, such as agriculture often have the least ability to pay for CDR but support the livelihoods of many of the world’s most climate-vulnerable people [Citation6]. These various factors that can affect CDR demand, in turn, prompts consideration of how the opportunity and/or obligation to undertake CDR may change in future through utilisation pathways.
Utilisation pathways
Utilisation pathways refer to the various ways that the CRB could be managed from the demand side. As this section explores, there are both active and passive choices options for utilisation pathways.
First-come, first-served
This is an unmanaged CRB utilisation pathway that sees unconstrained use of the CRB. Indeed, this pathway is where feasible CDR is utilised on a first-come, first-served basis, is the current status quo. In the near term, this pathway heightens the risk of overreliance on CDR by entities or companies with unrealised emissions reduction or avoidance potential. Faced with unconstrained consumption opportunities across all CDR types, users of the CRB would likely continue their preference for conventional CDR due to its lower-cost and higher availability. This could also have the attendant effect of weakening the prospects for investment in novel, durable CDR. In the longer-term, unconstrained use would quickly lead to exhaustion of CDR supply. This could leave significant volumes of residual hard to abate emissions that it is not possible to counterbalance with durable CDR, jeopardising attempts to attain or maintain global net zero in line with the Paris Agreement. In this way, a “first come, first served” approach is likely not effective as a utilisation pathway.
Geographic utilisation
This is a managed pathway sees the global CRB utilised along geographic lines, This means utilisation of the CRB within a given country or regional basis. In general terms this would see higher use of novel CDR to Europe and North America and more conventional CDR in Asia, South America and Africa [Citation7,Citation53]. This utilisation pathway would likely result in near-term exhaustion of domestic or regional CRBs, and significant underutilisation of it in others. This is especially true as geographic distribution may not reflect the absolute or feasible levels of CDR deployment, or CDR needed [Citation7]. In the long term, this pathway would be effective at preserving a portion of the existing global CRB but, importantly, it would not likely not be developing further feasible capacity within the global CRB. Consequently, geographical utilisation is likely not an optimal utilisation pathway.
CDR pricing
This is a managed utilisation pathway where access to the global CRB is mediated through price. This would occur by having a global price floor for CDR that could, like comparable carbon pricing models, be designed to set a high price for CDR. This price could be fixed threshold such as $100 per tCO2, progressively increased over time from a benchmark, or pegged to the average cost of CDR. Different price thresholds could also be set based on type of CDR or durability threshold. In the near term, setting a minimum price of CDR would ensure a much greater degree of adherence to the mitigation hierarchy whereby emissions are reduced first prior to any abatement via CDR being sought. However, at the same time, it could price many actors out of being able to afford CDR, leaving them unable to neutralise any hard-to-abate residual emissions they have. In the long-term this would likely lead to significantly more investment in novel CDR and less in conventional CDR. It could thus be a risky utilisation pathway should terrestrial CO2 sinks be put at risk in the near term and novel CDR strategies fail to materialise due to technological constraints [Citation24].
Hard to abate emissions only
This managed pathway allocates more of the CRB to actors with significant hard to abate residual emissions. This could be sectorally focused and align with proposed sectoral carbon budgets or could instead map directly onto levels of hard to abate residual emissions. There is a risk with this pathway of underdevelopment of the CRB as the ability to pay for CDR currently does not match with demand [Citation53]. Without conditions attached to CDR use, a preference for lower-cost CDR could drive investment in conventional CDR at the expense of developing novel CDR potential. Given that these hard-to-abate CO2 emissions are almost exclusively fossil-related, this could result in a deficit of investment into more durable forms of novel CDR, thereby constraining a significant portion of the future global CRB. Consequently, it does not appear to be an optimal utilisation pathway.
Like for like utilisation
This managed pathway sees demand for CDR being matched with its counterpart of CO2 emissions. Under this model, biogenic emissions would be removed by conventional CDR and fossil-fuelled emissions with novel CDR stored in the lithosphere [Citation58]. This would drive near-term significant demand for novel CDR but could risk underinvestment in conventional CDR. Generally, the sectors and countries with a higher ability to pay are also the ones whose emissions are principally fossil-based whilst those with a greater proportion of biogenic emissions tend to have a lower ability to pay. This could result in a greater distribution of CDR demand which matches countries’ changing emissions profiles [Citation39]. Implementing this utilisation pathway would significantly increase the cost of CDR for fossil-based industries, preserving the CRB, but potentially also not enabling its full development.
Summary
While these utilisation pathways present a range of indicative options, they are not mutually exclusive and can be applied in various combinations. This is necessary because allocating a global CRB would likely require extensive negotiations that balance an array of scientific, equity, economic, and political factors. With this in mind, it is important to consider what underlying principles could also dictate the optimal allocation of a CRB.
Allocating a carbon removal budget
Given the range of possible utilisation pathways, it is essential to directly consider how the CRB could be allocated. CDR potential is not evenly distributed: the technical potential and feasibility of different approaches varies considerably across the world. As one might expect, both the opportunity and the obligation to undertake CDR are also not homogeneous. This has led to attempts to allocate a “quota” for CDR both globally and sectorally based on principles such as responsibility, capability and equality as well as “ability to pay” and “cumulative per capita emissions” [Citation9,Citation31,Citation50,Citation53]. While this literature sheds light on options for how CDR could be allocated, it is less clear how these allocations could stimulate or hamper CDR potential itself, including regarding the utilisation pathways above [Citation53].
Historical emissions
The historical contributions of countries or sectors to cumulative CO2 emissions could be considered a principle on which to allocate a global CRB. Under such an approach, the allocation would be proportional to historical emissions rather than inversely proportional as allocations of the carbon budget tend to be. From a country perspective, this would allocate a greater proportion of the CRB to historically significant emitters, such as the United States and European Union and a smaller amount to emerging and developing economies. From a sectoral perspective, this would result in greater allocation to emissions-intensive industries such as the oil and gas and agriculture sectors. However, these countries and sectors have different abilities to pay, and different sources of CO2: fossil and biogenic respectively. Without an attendant obligation to conduct like for like CDR allocation based on historical emissions alone could result in consumption of the conventional part of the CRB by fossil-based emitters, which would have a negative effect on unlocking future, largely novel, CDR potential on the frontiers of the CRB [Citation58].
Per capita
Another approach is to equally distribute access to the CRB among the global population [Citation24]. Allocation via this means aims to address equity concerns by accounting for the population size of countries or regions. This would result in larger allocations of the global CRB to the world’s most populous countries, resulting in countries with significant populations with low per capita emissions, like India, with significant CRB surplus, while smaller populations with high per capita emissions, like Qatar, with a significant CRB deficit. From a sectoral perspective, most of the world’s emissions-intensive industries are based in countries with large populations, meaning that per capita allocation could imply a greater licence for ongoing emissions. It could also result in carbon leakage from countries with limited CRBs, to ones with larger CRBs. As a result, it is likely that per capita distribution is too blunt a principle to equitably allocate a global CRB.
Developmental needs
A CRB could also be allocated on the basis of the differing developmental amongst countries. As Article 9 of the Paris Agreement recognises, developing countries or regions require additional resources to transition to low-carbon futures [Citation2]. Allocating the CRB based on development needs could give developing countries more carbon operating space whilst restricting the carbon operating space for developed economies. Still, CDR costs money. Therefore, unless this allocation is combined with significant financial transfers, it is likely that the global CRB would remain significantly under-utilised. This is because, unlike developed countries, developing countries are unlikely to have the fiscal space to invest in addressing hard-to-abate emissions to reach net zero, especially if their fair share of those residual emissions is growing rather than shrinking [Citation39]. Consequently, this principle alone would likely not result in efficient utilisation of the global CRB.
Ability to pay
The financial capacity of different countries or sectors could also be considered when allocating the CRB [Citation6]. This principle acknowledges that entities with more economic resources also possess a greater ability to invest in and scale CDR. The simplest measure to do so on a country or sector basis would be to review the prices of units traded under emissions trading schemes (ETS). To ensure there is no perverse incentive to cancel an ETS or not adopt one in the first place, there could still be a minimum price floor for CDR, which recent developments suggest could be possible in the future.Footnote13 The same could be applied to sectors, whereby revenue per ton could similarly be used to allocate access to a CRB. While these price bands would fluctuate frequently, parties could instead negotiate these thresholds annually under a dedicated CDR stream under international climate negotiations [Section “Climate negotiations”]. Allocation along the lines of “ability to pay” could also exclude exempt countries from the obligation to undertake CDR, otherwise known as “CDR liability” on the basis that they are not fiscally able to invest in CDR capacity [Citation28]. Yet too, it could make CDR a “luxury” good displacing “deployment for compensatory removals in high priority but low wealth applications” [Citation6]. Therefore, allocation based on ability to pay alone does not enable wholly equitable access to the global CRB [Citation6].
Optimal allocation of the carbon removal budget to drive effective utilisation
No one principle explored in Sections “Historical emissions”–“Ability to pay” results in optimal allocation of the CRB. Yet it is clear that certain combinations of them can help to leverage the most promising utilisation pathways explored in Section “Utilisation pathways”. Currently, the unmanaged utilisation pathway, otherwise termed the “market based industry model” is critically insufficient in scaling the CDR and doing so in an equitable manner [Citation6,Citation28]. Countries have different comparative advantages in CDR. They also have a choice as to whether this CDR is utilised domestically or transferred internationally [Citation9,p.840]. Yet without careful guidance, this could lead to a “race to the bottom” for CDR projects, as has been seen on the voluntary carbon market to date which has largely only attracted investment into conventional rather than novel CDR. At the same time, investment into novel CDR demonstrates that a narrow focus on the most profitable techniques could limit the future CRB and lead to undemocratic decisions about this resource allocation [Citation6].
Allocation of the global CRB should instead stimulate a “race to the top” where CDR is available for hard-to-abate emissions at a range of price points to balance access with sustainable investment opportunities. Rather than a narrow focus on a ton for ton basis, this system should be capable of managing million and billion ton scale required by the Paris aligned pathways. The thresholds for these price points and volumes within the global CRB could be negotiated annually by Parties to the Paris Agreement [Citation59]. This would enable decisionmakers to balance the many relevant factors outlined in Sections “Historical emissions”–“Ability to pay” affecting optimal allocation in a manner that reflects the need for CDR at a given time without promoting overreliance on it [Citation6]. The opportunity to allocate a global CRB via negotiations could also help to balance the right to development which is often excluded from equity-based distributions of CDR. In sum, optimal allocation of the CRB is one that maximises the mitigation potential CDR can offer to enable us to achieve a full range of Paris-aligned pathways, including limiting warming to 1.5 °C and does so in an inclusive manner.
The implications of Carbon Removal Budgeting
Given the nascency of governance and institutional structures for CDR today, carbon removal budgeting carries several potential implications. For one, a finite CRB is further evidence of the need for actors– be they countries or corporations–to respect the broader mitigation hierarchy, where emissions should be avoided or reduced first before looking to CDR. As this section explores, clear targets and accounting of emissions and CDR can help avoid this and drive much-needed incentives for CDR deployment, including through international mechanisms such as Article 6 of the Paris Agreement [Citation60]. A CRB could also be used to scrutinise the credibility of corporate transition plans.
Domestic CDR targets and implementation
Carbon removal budgeting could be performed on an individualised country basis yielding important insights as to its current and future CDR potential, and whether this is reasonable given their emissions trajectory [Citation8]. Most countries rely on CDR to reach their climate mitigation targets under the Paris Agreement.Footnote14 Yet, very few outline removal targets or ensure robust guardrails as to the use of CDR towards their nationally determined contributions or long-term strategies [Citation8]. Setting a clear CDR target in the context of a finite CRB enables one to see projections of CDR potential supply and demand: helping to plan future required capacity additions as well as helping ensure that CDR is used to supplement rather than undermine emissions reductions [Citation55,p.73]. This is particularly important as it is the path dependencies established by institutions and governance that will likely be deterministic as to where CDR investments, and ultimately breakthroughs will be made [Citation6].
A CRB could also assist with attempts to integrate CDR into domestic ETS or setting up an alternative domestic CDR trading scheme [Citation56]. There are several options for integrating CDR into ETS. One option is governments allowing the blending of a certain amount of voluntary carbon credits which could include but are not limited to CDR credits– with the allowances required under ETS in order to discharge obligations under a domestic ETS.Footnote15 Another potential system is a “Carbon Removal Trading Scheme”, which instead of an emissions cap and trade system, establishes a CRB system that can allocate the responsibility to generate future CDR. Conceiving a finite CRB could help drive demand for CDR development for compensatory purposes as well as to reach goals of net negative [Citation6].
Climate negotiations
There has been a notable lack of attention to CDR as a public good in today’s international policy decisions [Citation6]. The global CRB is likely to change over time, as will what constitutes an equitable allocation of that potential [Citation24]. A finite global CRB could inform future international climate negotiations relating to the determination and allocation of global CDR potential [Citation8]. For this to occur there is a need to gain consensus on the principles on which a finite CRB could be developed and allocated. Such an opportunity could occur under the Paris Agreement with the creation of a dedicated track to negotiate the management of the global CRB, including any conditions attached to its use. This opportunity is vital given the compensatory promise of CDR and the imprecision with which residual emissions are often defined [Citation8,Citation23]. This could include setting an end-of-century CDR target, with graduated intervals before that or undertaking a bottom-up analysis of CDR demand based on planned residual emissions, tailored to Parties’ national circumstances.
Whether or not there could be the global will to initiate such a system depends on the extant incentives that countries have. As the global CRB could also be a source of economic opportunity for countries with significant CDR potential, it could see less resistance than carbon budget models, as shown by the ready interest in Article 6. Within this context, a CRB could help create demand for CDR in the new Article 6.4 market mechanism in a manner that reflects the principle of environmental integrity. The need for Parties to examine CDR potential more directly in this manner is also supported by the recent call of the Subsidiary Body for Scientific and Technological Advice to the Paris Agreement for the research community to address the risks and opportunities related to upscaling CDR [Citation61]. The G7, who have strong sway in the climate negotiations, also recently released a statement “importance of significant increase in the pace and scale of deployment of carbon management technologies and infrastructure, as well as promoting development of export/import mechanisms for CO2” [Citation62]. As a result, there could well be appetite in future for CDR to obtain its own negotiations track. Within this context, it is clear to see how quantifying global CRB, and collectively determining its utilisation and pathways can help bolster its efficient and equitable development and use of CDR potential.
Corporate transition planning
A wide range of companies have set net zero targets [Citation4]. To reach net zero a company’s residual emissions must be counterbalanced with durable CDR. Doing so in a coherent way depends on a robust transition plan. Some companies are already demonstrating considerable leadership in this space [Citation63]. As a first step towards this, companies and/or sectors should establish their own CRBs–noting how their planned and actual use of CDR–based offsets aligns with the constraints of a Paris-aligned CRB. Such an analysis will reveal how much a given company or sector is in “overdraft” of their CRB allocation through excessive reliance on CDR. Initial regulatory oversight, leading to ultimate enforcement of such targets and transition plans may be considered, for instance, within the evolving climate disclosure and regulation that requires actors to provide a “detailed and realistic implementation plan that shows how those commitments and targets will be achieved and that allocates resources to that end” [Citation64].
A company can and should also perform stress testing of the CRB for added surety. This could mean developing bespoke scenarios where their CDR demand increases or supply decreases (and thus availability decreases and prices rise). This is critical as both factors can impact the viability of a corporate transition plan; for instance, if a company is relying on the more accessible low-cost units of the CRB or a CDR technique that has not yet been proven at scale, its transition plan could easily be knocked off course. In this way, a corporate-level CRB can help corporates to prioritise value-chain decarbonisation, whilst exploring how they can assist in the development and efficient use of the global CRB.
Conclusion
Investing in CDR potential is essential to achieve the Paris Agreement’s temperature goal of limiting warming to “well below 2 degrees” [3:Art 2(1)(a)]. Timely investment in CDR potential can help reduce its associated transitional and economic costs, through “rightsizing it” [Citation65]. Yet, many barriers currently stand in the way of unlocking this investment. Much of this challenge results from a false equivalency between 1 tCO2 emitted and removed [Citation30,Citation39]. Unlike CO2 emissions that are fungible in the atmosphere, CO2 removal is nonfungible. Storage in carbon reservoirs–geological, terrestrial, or oceanic–is fundamentally limited, and each has differing levels of reversal risk. Aside from the physical capacity for removal and storage, a wide range of economic, institutional, and technological constraints affect the feasibility of CDR–making it a scarce resource that requires budgeting.
As a conceptual tool, the CRB can be deployed to yield actionable insights on micro, meso or macro scales. It can render insights into how CDR supply changes over time whilst also providing a tool to allocate removal supply fairly along geographical and sectoral lines. The range of constraints that apply to the CRB, in turn, provide insights as to how it can be grown. Depending on the context for which a CRB is estimated, a wide range of factors may be relevant in determining its optimal allocation and utilisation. On this point, there are similarities and differences with the carbon budget. In general, an optimal utilisation pathway for a CRB is likely to be one that unlocks the full development potential by equitably allocating the opportunity and obligation to undertake CDR. For this to be effective, more work is needed to address the constraints on CDR. Actors operating over and above their fair allocation of the global CRB, whether countries, sectors, or companies, should take the lead in addressing these constraints on CDR [Citation11]. Realising this objective requires more work to identify pressures on the CRB and how they may be obviated.
Building on this, future research could size CRBs for different contexts and provide insight into the trends and trade-offs that are faced over time as CDR potential is developed and utilised. It will also be necessary to regularly review CRBs as CDR demand and supply scenarios become clearer, as will the viability and role of different forms of CDR as part of the global CRB. Research could further shed light on the dependencies and opportunities created by the challenge of equitably developing and allocating the globe’s finite CDR potential. In this way, it could directly inform the net zero transition planning of both countries and corporates alike.
Consequently, carbon removal budgeting has implications for both state and non-state actors alike. For states, it draws attention to the CDR component of the net zero equation, including the need to implement national CDR targets and transparently negotiate a fair allocation of CDR obligation and opportunity internationally–potentially through the UNFCCC. For private actors CRB can reveal areas of strength and weakness in sectoral or corporate transition plans. In this way, it can be a powerful driver of private market investment in developing CDR potential further as a finite good. In this way, it is possible to conceive how CRB can lend confidence to the long-term investment landscape for CDR whilst illustrating that the near-term priority for all actors should and must remain reducing emissions [Citation7]. This is particularly so as the CRB forecast reveals a near-term deficit: requiring scaling CDR and paring back demands on what is a finite resource. Whether explicit or not, managed or unmanaged, a global CRB will always be in existence Whether or not we harness its potential to the fullest to stimulate its development and equitably allocate though is an active choice.
Supplemental Material
Download MS Excel (1.2 MB)Acknowledgements
With thanks to reviewers Adrien Rose and Johanna Arlinghaus from the University of Oxford.
Disclosure statement
No potential conflict of interest was reported by the author(s).
Data availability statement
The data that support the findings of this study are available from the corresponding author, IJ, upon reasonable request.
Additional information
Funding
Notes
1 Dynamic permanence requires management practices to be continued even when the sink is saturated. Smith P, Haszeldine R and Smith S. Preliminary assessment of the potential for, and limitations to, terrestrial negative emission technologies in the UK. Environmental Science: Processes & Impacts. 2016;.18:1400–1405. doi: 10.1039/C6EM00386A.
2 There are however a much broader range of constraints on the CRB. Indeed, the IPCC in AR6 outlines a number of constraints which are equally applicable to the range of CDR techniques in greater detail, including geophysical, technical, environmental, institutional, socio-cultural, and economic feasibility. See [Citation1] at Box TS.15.
3 In line with the maximum feasible price consistently applied by the IPCC CDR projections in its AR6 WG III Report [Citation1]. $100 USD per ton is also a price where compliance markets could also see convergence around especially as the EU ETS already exceeded this threshold at times throughout 2023.
4 The TRL levels are taken from IPCC AR6 WG III, see [Citation1] at pp.1275–1276.
5 See the Supplementary Materials for a full breakdown and assumptions. See also Chapters 7 and 12 of [Citation1].
6 This assumption strikes a balance between the uncertainties related to the as yet unknown feedbacks from the global carbon cycle that could result if terrestrial and oceanic sinks becoming net sources, as well as the likelihood that novel CDR capacity will continue to see growth. See: Keller D, Lenton A, Littleton E et al. The effects of carbon dioxide removal on the carbon cycle. Current climate change reports. 2018;4: 250–265. doi: 10.1007/s40641-018-0104-.
7 The ranges referred to here are the minimum (informed under by a scenario with maximum CDR demand and minimum CDR supply) and the maximum (informed under by a scenario with minimum CDR demand and maximum CDR supply).
8 For example, the focus on removals shared socio-economic pathway would yield insights into a high-demand CDR scenario, and the focus on renewables shared socio-economic pathway a comparatively lower one.
9 A range of emerging standards, methodologies and disclosures are being developed to address these quality concerns most of which will also be applicable to removal units within a given CRB. Carbon ratings are provided by a growing range of agencies including Be Zero, Calyx Global and Sylvera. From the supply side the Integrity Council for the Voluntary Carbon Market’s Core Carbon Principles also reveal further insights as to qualities of removal-based credits.
10 The total area of land needed to meet the projected demand through country commitments under the Paris Agreement amounts to almost 1.2 billion hectares (the near entirety of the area of current global cropland). Dooley K, Keith H, Larson A et al. The Land Gap Report 2022; Melbourne: 2022.
11 This commitment by the Frontier consortium now exceeds $1 Billion USD of future purchases of CDR via offtake agreements. An advance market commitment to accelerate carbon removal [Internet]; Frontier; 2023 [cited 2023 July 8]. Available from:. https://frontierclimate.com/
12 This is despite carbon-intensive industries being among the most likely to set net ero targets, with ⅔ oil and gas companies globally having set them. Net zero targets among world's largest companies double, but credibility gaps undermine progress [Internet] Net Zero Tracker; 2023 [cited 2023 Sep 1]. Available from: https://zerotracker.net/insights/net-zero-targets-among-worlds-largest-companies-double-but-credibility-gaps-undermine-progress.
13 As revealed by Berkeley’s Voluntary Registry Offsetting Database, pure removals constitute less than 5% of total available and retired credits, with no novel CDR having been traded on the voluntary carbon market at large.
14 23 of 29 long-term strategies posit that removals are needed to reach their targets, including net zero targets. Jacobs H, Gupta A, Möller I. Governing-by-aspiration? Assessing the nature and implications of including negative emission technologies (NETs) in country long-term climate strategies. Global Environmental Change. 2023; 81, p.102691. doi: 10.1016/j.gloenvcha.2023.102691
15 Singapore’s proposal to allow 5% of its compliance carbon tax to come from eligible offsets procured on the VCM from 2024. Carbon Tax [Internet] Strategy Group Prime Minister’s Office (Singapore); 2023 [cited 23 July 2023]. Available from: https://www.nccs.gov.sg/singapores-climate-action/mitigation-efforts/carbontax/.
References
- Shukla P, Skea J. Climate Change 2022 Mitigation of Climate Change Working Group III Contribution to the Sixth Assessment Report of the Intergovernmental Panel on Climate Change. Geneva IPCC; 2022.
- Smith S, Geden O, Nemet G, et al. The State of Carbon Dioxide Removal. 1st ed.; 2023.
- Paris Agreement. UN Doc. FCCC/CP/2015/10/Add.1 Decision 1/CP.21 (12 December); 2015.
- Zero Tracker. 2023 [Internet]; [cited 2023 Oct 21]. Available from https://zerotracker.net/.
- Race to Zero Campaign. [Internet]. UNFCCC. 2022; [cited 2023 Oct 21]. Available from: https://unfccc.int/climate-action/race-to-zero-campaign.
- Grubert E, Talati S. The distortionary effects of unconstrained for-profit carbon dioxide removal and the need for early governance intervention. Carbon Manage. 2024;15(1):11. doi: 10.1080/17583004.2023.2292111.
- Ampah JD, Jin C, Liu H, et al. Prioritizing non-carbon dioxide removal mitigation strategies could reduce the negative impacts associated with large-scale reliance on negative emissions. Environ Sci Technol. 2024;58(8):3755–3765. doi: 10.1021/acs.est.3c06866.
- Smith HB, Vaughan NE, Forster J. Long-term national climate strategies bet on forests and soils to reach net-zero. Commun Earth Environ. 2022;3(1):305. doi: 10.1038/s43247-022-00636-x.
- Rueda O, Mogollón JM, Tukker A, et al. Negative-emissions technology portfolios to meet the 1.5° C target. Global Environ Change. 2021;67:102238. doi: 10.1016/j.gloenvcha.2021.102238.
- World Business Council for Sustainable Development. Removing Carbon Responsibly. Geneva: WBSCD; 2023.
- Fyson C, Baur S, Gidden M, et al. Fair-share carbon dioxide removal increases major emitter responsibility. Nat Clim Chang. 2020;10(9):836–841. doi: 10.1038/s41558-020-0857-2.
- Mitchell-Larson E, Allen M. Prosets: a new financing instrument to deliver a durable net zero transition. Clim Change. 2022;174(1-2):15. doi: 10.1007/s10584-022-03423-x.
- Prado A, Mac Dowell N. The cost of permanent carbon dioxide removal. Joule. 2023;7(4):700–712. doi: 10.1016/j.joule.2023.03.006.
- Wijnand S. Principles for carbon negative accounting. NEGUM (Horizon 2020 Report, Grant Agreement no. 869192).
- Hauber G. Norway’s Sleipner and Snøhvit CCS: industry models or cautionary tales?. Cleveland Institute for Energy Economics and Financial Analysis; 2023.
- Kelemen PB, Matter J, Streit EE, et al. Rates and mechanisms of mineral carbonation in peridotite: natural processes and recipes for enhanced, in situ CO2 capture and storage. Annu Rev Earth Planet Sci. 2011;39(1):545–576. doi: 10.1146/annurev-earth-092010-152509.
- Mei B. Carbon offset as another driver of timberland investment returns in the United States. JForBusRes. 2023;2(1):1–19. doi: 10.62320/jfbr.v2i1.20.
- CDR.Fyi. 2023. [Internet]; cited 2023 Oct 24]. Available from: https://www.cdr.fyi/.
- Zickfeld K, Azevedo D, Mathesius S, et al. Asymmetry in the climate–carbon cycle response to positive and negative CO2 emissions. Nat Clim Chang. 2021;11(7):613–617. doi: 10.1038/s41558-021-01061-2.
- Allen M, Frame D, Huntingford C, et al. Warming caused by cumulative carbon emissions towards the trillionth tonne. Nature. 2009;458(7242):1163–1166. doi: 10.1038/nature08019.
- Matthews H, Landry J, Partanen A, et al. Estimating carbon budgets for ambitious climate targets. Curr Clim Change Rep. 2017;3(1):69–77. doi: 10.1007/s40641-017-0055-0.
- Masson-Delmotte V, Zhai P, Pirani A, et al. Climate Change 2021: the physical science basis. Contribution of Working Group I to the Sixth Assessment Report of the Intergovernmental Panel on Climate Change- Summary for Policymakers. IPCC; 2021.
- Buck H, Carton W, Lund J, et al. Why residual emissions matter right now. Nat Clim Chang. 2023;13(4):351–358. doi: 10.1038/s41558-022-01592-2.
- Deprez A, Leadley P, Dooley K, et al. Sustainability limits needed for CO2 removal. Science. 2024;383(6682):484–486. doi: 10.1126/science.adj6171.
- Axelsson K, et al. The Oxford principles for net zero aligned carbon offsetting. Oxford: University of Oxford; 2024.
- Fankhauser S, Smith SM, Allen M, et al. The meaning of net zero and how to get it right. Nat Clim Chang. 2022;12(1):15–21. doi: 10.1038/s41558-021-01245-w.
- Powis CM, Smith SM, Minx JC, et al. Quantifying global carbon dioxide removal deployment. Environ Res Lett. 2023;18(2):024022. doi: 10.1088/1748-9326/acb450.
- Yang P, Mi Z, Wei Y, et al. The global mismatch between equitable carbon dioxide removal liability and capacity. Natl Sci Rev. 2023;10(12):nwad254. doi: 10.1093/nsr/nwad254.
- Forster PM, Smith CJ, Walsh T, et al. Indicators of Global Climate Change 2022: annual update of large-scale indicators of the state of the climate system and the human influence. Earth Syst Sci Data. 2023;15(6):2295–2327. doi: 10.5281/zenodo.8000192.
- Carton W, Lund J, Dooley K. Undoing equivalence: rethinking carbon accounting for just carbon removal. Front Clim. 2021;3:130. doi: 10.3389/fclim.2021.664130.
- Macinante J, Ghaleigh N. Regulating removals: bundling to achieve fungibility in GGR removal units. CCLR. 2022;16(1):3–17. doi: 10.21552/cclr/2022/1/4.
- Cullenward D. A framework for assessing the climate value of temporary carbon storage. Brussels: Carbon Market Watch; 2023.
- Michaelowa A, Honegger M, Poralla M, et al. International carbon markets for carbon dioxide removal. PLoS Clim. 2023;2(5):e0000122. doi: 10.1371/journal.pclm.0000118.
- Technology. [Internet] 2023; Pachama; [cited 2023 Oct 1]. Available from: https://pachama.com/technology/.
- Puro Standard. [Internet] Puro. 2023; [cited 2023 Sep 15]. Available from: https://puro.earth/puro-standard-carbon-removal-credits/.
- Strefler J, Bauer N, Humpenöder F, et al. Carbon dioxide removal technologies are not born equal. Environ Res Lett. 2021;16(7):074021. doi: 10.1088/1748-9326/ac0a11.
- Bach LT, Tamsitt V, Gower J, et al. Testing the climate intervention potential of ocean afforestation using the Great Atlantic Sargassum Belt. Nat Commun. 2021;12(1):2. doi: 10.1038/s41467-021-22837-2.
- Rosentreter JA, Al-Haj A, Fulweiler RW, et al. Methane and nitrous oxide emissions complicate coastal blue carbon assessments. Global Biogeochem Cycles. 2021;35(2):e2020GB006858. doi: 10.1029/2020GB006858.
- Yuwono B, Yowargana P, Fuss S, et al. Doing burden-sharing right to deliver natural climate solutions for carbon dioxide removal. Nature-Based Solut. 2023;3:100048. doi: 10.1016/j.nbsj.2022.100048.
- Keller D, Lenton A, Littleton E, et al. The effects of carbon dioxide removal on the carbon cycle. Curr Clim Change Rep. 2018;4(3):250–265. doi: 10.1007/s40641-018-0104-3.
- Hepburn C, Adlen E, Beddington J, et al. The technological and economic prospects for CO2 utilization and removal. Nature. 2019;575(7781):87–97. doi: 10.1038/s41586-019-1681-6.
- Hickey C, Fankhauser S, Smith SM, et al. A review of commercialisation mechanisms for carbon dioxide removal. Front Clim. 2023;4:1101525. doi: 10.3389/fclim.2022.1101525.
- UNFCCC. Outcome of the first global stocktake. FCCC/PA/CMA/2023/L.17 (13 December 2023).
- Convention on Biodiversity. Biodiversity and climate change. Decision. 2010;X/(33):8(w).
- Förster J, Beck S, Borchers M, et al. Framework for assessing the feasibility of carbon dioxide removal options within the national context of Germany. Front Clim. 2022;4:28. doi: 10.3389/fclim.2022.758628.
- Pozo C, Galán-Martín Á, Reiner D, et al. Equity in allocating carbon dioxide removal quotas. Nat Clim Chang. 2020;10(7):640–646. doi: 10.1038/s41558-020-0802-4.
- Net zero. Urgent Beyond Value Chain Mitigation Is Essential [Internet]; Science Based Target Initiative, 2022; [cited 2023 September 2]. Available from: https://sciencebasedtargets.org/blog/net-zero-urgent-beyond-value-chain-mitigation-is-essential.
- Leading Tech Companies Unite Against Climate Change. Change. 2023 [cited 2023 Aug 2]. Available from: [Internet] Milkywire; https://www.milkywire.com/articles/leading-tech-companies-unite-against-climate-change.
- Carbon Removal Certification [Online] European Union. 2023; [cited 2023 July 25]. Available from: https://climate.ec.europa.eu/eu-action/sustainable-carbon-cycles/carbon-removal-certification_en.
- Biden Administration Launches $3.5 Billion Program To Capture Carbon Pollution From The Air. [Internet] United States Department of Energy. 2022; [cited 2023 July 23]. Available from: https://www.energy.gov/articles/biden-administration-launches-35-billion-program-capture-carbon-pollution-air-0.
- Industrial Clusters. ; 2023 [Internet] Realise CCUS; 2023; [cited 2023 July 23]. Available from: https://realiseccus.eu/ccus-and-refineries/industrial-clusters.
- Cluster sequencing for carbon capture, usage and storage (CCUS): Track-2. [Internet] HM Government. 2023; [cited 2023 Aug 3]. Available from: https://www.gov.uk/government/publications/cluster-sequencing-for-carbon-capture-usage-and-storage-ccus-track-2.
- McMullin B, Price P, Jones M, et al. Assessing negative carbon dioxide emissions from the perspective of a national “fair share” of the remaining global carbon budget. Mitig Adapt Strateg Glob Change. 2020;25(4):579–602. doi: 10.1007/s11027-019-09881-6.
- Lee K, Fyson C, Schleussner C. Fair distributions of carbon dioxide removal obligations and implications for effective national net-zero targets. Environ Res Lett. 2021;16(9):094001. doi: 10.1088/1748-9326/ac1970.
- Hale T, Smith S, Black R, et al. Assessing the rapidly-emerging landscape of net zero targets. Climate Policy. 2022;22(1):18–29. doi: 10.1080/14693062.2021.2013155.
- Zero Tracker. Net Zero Stocktake 2023. London: Zero Tracker; 2023.
- Honegger M, Poralla M, Michaelowa A, et al. Who is paying for carbon dioxide removal? Designing policy instruments for mobilizing negative emissions technologies. Front Clim. 2021;3:672996. doi: 10.3389/fclim.2021.672996.
- Hoglund R, Mitchell-Larson E, Delerce S. How to avoid carbon removal delaying emissions reductions. Carbon Gap. 2023.
- Mace MJ, Fyson CL, Schaeffer M, et al. Large‐scale carbon dioxide removal to meet the 1.5° C limit: key governance gaps, challenges and priority responses. Global Policy. 2021;12(S1):67–81. doi: 10.1111/1758-5899.12921.
- La Hoz Theuer S, Hall M, Eden A, et al. Offset use across emissions trading systems. Berlin: ICAP; 2023.
- Subsidiary Body for Scientific and Technological Advice. Research and systematic observation Draft conclusions proposed by the Chair, [8] and [9]; 2023.
- G7. Climate, Energy and Environment Ministers’ Meeting Communiqué. (Torino, April 29–30, 2024).
- Microsoft, for example, has committed to being carbon-negative by 2030 and eventually removing all of its historical emissions. Carbon Removal Program [Internet] Microsoft. 2023; [cited 2023 Aug 23]. Available from: https://www.microsoft.com/en-us/corporate-responsibility/sustainability/carbon-removal-program.
- European Parliament. Empowering consumers for the green transition (17 January 2024). Available from: https://www.europarl.europa.eu/doceo/document/TA-9-2024-0018_EN.html.
- Field CB, Mach KJ. Rightsizing carbon dioxide removal. Science. 2017;356(6339):706–707. doi: 10.1126/science.aam9726.