ABSTRACT
Interleukin-6 (IL-6) is a unique pleiotropic cytokine exhibiting both pro- and anti-inflammatory properties depending on the target cell type. Plasma IL-6 levels are associated with cardiovascular risk. IL-6 elevation in atherosclerosis results in effects on multiple cells involved in lipid processing and plaque formation. IL-6 is also the primary determinant of acute phase protein production. IL-6 has a number of properties that foster development of cardiovascular disease. These include activation of endothelial cells, pro-thrombotic effects on platelets and promotion of smooth muscle proliferation and macrophage lipid accumulation. Despite these overall unfavourable effect on cells involved in atheroma formation, IL-6 also has a positive impact on the lipid handling system through upregulation of ATP binding cassette transporter (ABC)A1, a protein involved in macrophage lipid efflux. Further, IL-6 can inhibit other inflammatory cytokines. Based on its possible role in accelerating atherosclerosis, blockade of IL-6 action with the antibody tocalizumab, a treatment for rheumatoid arthritis and juvenile rheumatoid arthritis, has been evaluated as an atheroprotective agent, but studies are inconclusive. This review discusses multiple aspects of IL-6 effects on parameters related to development of atherosclerosis and highlights their manifestations in cell culture, murine models and human studies.
Introduction
Interleukin (IL)-6 is a pleiotropic cytokine originally identified in 1985 as a B-cell differentiation factor (BSF-2) capable of inducing the maturation of B cells into antibody-producing cells [Citation1]. Cloned in 1986, IL-6 is a 26-kDa glycopeptide whose gene maps to chromosome 7p21 [Citation2]. It was considered a novel interferon (IFN-β2), but studies with recombinant IL-6 and anti-IL-6 antibody demonstrated that IL-6 had no IFN activity [Citation3]. IL-6 is now known to exhibit a broad range of biological activities in multiple systems. IL-6 displays both pro-inflammatory and anti-inflammatory properties depending on the type of injury and the organ or cell in which it is acting [Citation4].
Atherosclerosis, the leading cause of morbidity and mortality worldwide, is both a chronic inflammatory condition and a disorder of lipid metabolism [Citation5]. It involves a complex interplay between resident vascular wall cells and infiltrating immune cells. Plaque progression and later potential rupture may be attributed to vascular inflammation [Citation6,7]. IL-6 is an early and central regulator of inflammation found in high abundance in atherosclerotic plaques. It is synthesised by multiple cells of the artery wall including macrophages, vascular smooth muscle and endothelial cells. The contribution of IL-6 to atherosclerosis is controversial and will be discussed in this review.
IL-6: pro-inflammatory and anti-inflammatory properties
IL-6 is an unusual cytokine with documented pro-inflammatory and anti-inflammatory aspects ().
Table 1. Contrasting pro-inflammatory and anti-inflammatory properties of IL-6.
IL-6 has profound effects on the expansion and activation of T cells. T cells are both a source and a target of IL-6. IL-6 promotes CD8+ T cell differentiation into cytotoxic T cells [Citation8]. IL-6 alone or in combination with other cytokines promotes differentiation of T helper 1, T helper 17 (Th17, which produce IL-17) and T follicular helper (Tfh) cells in lymphoid tissue [Citation9,10]. IL-6, working alongside transforming growth factor (TGF)-β, has a major role in Th17 cell differentiation from naive CD4+ T-cells [Citation11,12]. Tfh, generated from naive T CD4+ cell precursors, participate in differentiation of antigen-specific B lymphocytes into memory and plasma cells [Citation13]. Continuous synthesis of excess IL-6 results in hypergammaglobulinemia and autoantibody production [Citation14]. IL-6 also inhibits TGF-β-induced regulatory T cell (Treg) differentiation [Citation15]. Treg is a subset of CD4+ T cells that maintain peripheral tolerance, inhibit autoimmunity and protect against tissue injury. Overall, IL-6 promotes a pro-inflammatory milieu in which inducible Treg responses are suppressed.
IL-6 is a principal inducer of acute-phase protein synthesis through hepatocyte stimulation which can result in fever and anaemia [Citation16]. Acute-phase proteins stimulated by IL-6 include C-reactive protein (CRP), β2-fibrinogen, amyloid protein, haptoglobin and hemopexin. Amyloid protein and CRP elevations are associated with atherosclerosis [Citation17,18]. The acute phase response is thought to be a beneficial reaction that mobilises host defences against bacterial pathogens [Citation19].
Further evidence of its inflammatory character is the chemotactic activity of IL-6 for neutrophils and macrophages [Citation20]. Combining chemotaxis for monocytes with the ability to activate endothelial cells and induce chemokine production and adhesion molecule expression adds up to high atheroma-promoting and inflammatory potency [Citation21–Citation23]. IL-6 deficient mice have impaired immune and acute-phase responses [Citation16,24]. In these mice, IgG production is compromised upon immunisation with a T cell-dependent antigen.
IL-6 also acts in an anti-inflammatory manner by inhibiting tumor necrosis factor (TNF)-α and IL-1 production [Citation25,26] and by induction of the IL-1 receptor antagonist and the soluble p55 TNF-α receptor [Citation27]. IL-6 inhibits lipopolysaccharide (LPS)-induced production of TNF-α in cultured human monocytes and in the U937 human monocytic cell line [Citation28]. Infusion of recombinant human IL-6 at a low dose into young healthy male volunteers induced two anti-inflammatory cytokines: IL-1 receptor agonist (IL-1ra) and IL-10 [Citation29]. IL-6 also induces tissue inhibitor of matrix metalloproteinase (TIMP) -1, which impedes activity of collagenase and therefore has anti-proteolytic activity [Citation30,31].
IL-6 structure and signalling
The X-ray structure of IL-6 reveals a 4 helix bundle in which helices A and B are oriented in the same direction and C and D in the opposite direction. [Citation32]. IL-6 is the founding member of a family of related cytokines that share this 4 helix structure and interact with the cell surface receptor glycoprotein 130 (gp130) as a signal transducing element in addition to their respective cytokine receptors. Members of this family, also known as the gp130 family of cytokines, include ciliary neurotrophic factor, IL-11, IL-31, leukaemia inhibitory factor, cardiotrophin-1 and oncostatin M [Citation33–Citation35].
IL-6 can activate cells through both membrane-bound (IL-6R, classic pathway) and soluble receptors (sIL-6R, trans-signalling pathway) [Citation36,37] (). IL-6R is cleaved from the cell surface by A disintegrin and metalloproteinase 17 (ADAM17) [Citation38]. IL-6 only displays measurable affinity for IL-6R, but not for gp130 while IL-6R alone also does not bind gp130. The IL-6 complexed to IL-6R must bind 2 monomers of gp130 to form the hexamer (2 IL-6, 2 IL-6R and 2 gp130) that brings about signal transduction [Citation39,40]. Formation of the hexamer leads to activation of Gp130 which then induces the phosphorylation of receptor-associated kinases (JAK1, JAK2 and Tyk2) within the cell. Signal Transducer and Activator of Transcription (STAT) transcription factors are directly phosphorylated and activated by the JAK family tyrosine kinases, leading to the activation of inflammatory STAT transcription targets genes [Citation41–Citation43]. Alternatively, IL-6 signalling can also occur via the SHP2/extracellular signal-regulated kinase pathway.
Figure 1. IL-6 classic and trans-signalling. IL-6 classic-signalling occurs through IL-6R present on the cell membrane.
Notes: In trans-signalling, cells that do not express IL-6R can respond to IL-6 via naturally occurring soluble IL-6R. Abbreviations: ADAM17, A disintegrin and metalloproteinase domain 17; ERK, extracellular signal-regulated kinase; gp130, glycoprotein 130; ICAM-1, intercellular adhesion molecule 1; IL, interleukin; IL-6R, IL-6 receptor; JAK-STAT, janus kinase/signal transducer and activator of transcription; sIL-6R, soluble IL-6 receptor; Th-2, T helper cell 2; VCAM-1, vascular cell adhesion molecule 1.
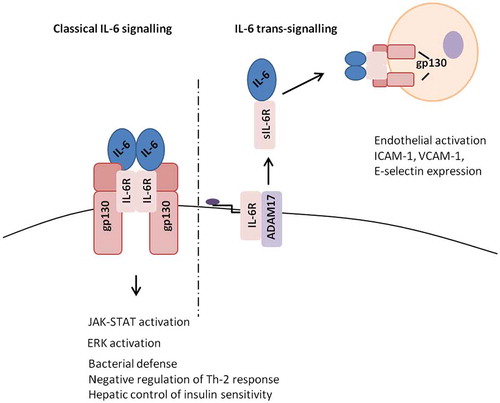
Trans-signalling occurs because sIL-6R enables IL-6 signaling in cells that are unable to express IL-6R. The sIL-6R can be detected in various body fluids and at sites of inflammation. Trans-signalling is believed to promote transition from acute to chronic inflammation while classic signalling is involved in immune defence against pathogens [Citation44,45].
The importance of classical signalling over trans-signalling in infectious processes is highlighted by studies in mice infected with Mycobacterium tuberculosis (Mtb) [Citation46]. In mice, Il-6 plays an active role in mounting resistance against Mtb when infection is introduced intravenously, but not when it is delivered in aerosolised form. When mice given a solubilised GP130 variant that blocks trans-signalling are infected with Mtb intravenously, the protective effect of IL-6 against Mtb is retained, indicating that classical signalling is responsible for IL-6 actions in tuberculosis. Similarly, in studies of mice infected with the Gram positive bacterium Listeria Monocytogenes, blocking IL-6 activity with neutralising antibody resulted in higher titers of L. monocytogenes in spleen and liver while specifically blocking trans-signalling left titers at the level seen in infected controls [Citation47].
In another model of infection, classical signalling is shown to be associated with better outcome in mice. In C57BL/6 J mice with polymocrobial sepsis, selective inhibition of IL-6 trans-signalling was found to yield superior survival compared to total blockade of both classical and trans-signaling, likely due to prevention of healing processes mediated through classical signalling with total blockade [Citation48].
IL-6 and the cardiovascular system
Despite its anti-inflammatory properties, IL-6 acts in the cardiovascular system in a detrimental way overall. IL-6 levels increase with age and are associated with higher mortality in nondisabled persons over age 65 from both cardiovascular and non-cardiovascular causes [Citation49]. IL-6 is elevated in coronary heart disease patients and may be a marker of inflammation related to cardiovascular risk [Citation50–Citation52]. In fact, Lefkou et al. [Citation53] showed that children aged 5–15 years with a positive family history of premature coronary artery disease had significantly higher IL-6 in plasma compared with the control group of children without this family history (p < 0.001). Further, Fisman et al. [Citation54] found that in persons with pre-existing stable coronary artery disease, higher IL-6 was associated with a poorer prognosis over an average follow-up of about 6 years. In this nested, case-control study, each 1 pg/ml increase in IL-6 was associated with a 1.70 (range 1.23–2.45) increased relative odds of subsequent MI or sudden death. In a study of 263 patients admitted to a hospital in Beijing, China with ST-segment elevation myocardial infarction, admission level of IL-6 correlated with cardiovascular mortality over 3 years of follow-up [Citation55]. In addition, immunohistochemical studies show high IL-6 expression in human atherosclerotic arteries in macrophage rich areas of the shoulder regions of coronary plaques [Citation56].
Murine studies have been mixed, with a number showing no protection from infarct damage with elimination of IL-6 effects. In a mouse study of myocardial infarction induced by coronary artery ligation, Fuchs et al. [Citation57] found that neither IL-6 deficiency (knockout mice) nor exogenous IL-6 administration (injection into the femoral vein) impacted infarct size, left ventricular structure, left ventricular function or survival rates, possibly because other compensatory factors come into play when IL-6 is not available. In mice with ischaemia/reperfusion injury induced by 60 min of ligation of the left anterior descending coronary artery, weekly injections of the IL-6R antibody MR 16-1 resulted in no differences in myocardial fibrosis, infarct size or hypertrophy compared to mice given control IgG [Citation58].
Individuals with type 2 diabetes are known to be at elevated risk for atherosclerosis [Citation59]. Oxidative stress mechanisms elevate IL-6 in type 2 diabetes and circulating IL-6 is correlated with insulin resistance [Citation60]. High IL-6 in the setting of type 2 diabetes is an independent predictor of cardiovascular events [Citation61–Citation64].
IL-6 may have direct pro- and anti- atherogenic effects on processes associated with development and progression of atherosclerosis. Pro-atherogenic effects include stimulation of vascular smooth muscle proliferation [Citation65–Citation67], endothelial cell activation [Citation68] and platelet activation [Citation69,70] while atheroprotective effects involve lowering of plasma low density lipoprotein (LDL) via upregulated LDL receptor gene expression [Citation71].
Vascular smooth muscle proliferation is induced in culture via trans-signalling and is accompanied by induction of monocyte chemoattractant protein-1 which can recruit monocytes and smooth muscle to atherosclerotic lesions [Citation72,73].
IL-6 promotes platelet production in mice and humans [Citation74–Citation76]. In patients with elevated markers of inflammation (high erythrocyte sedimentation rate and CRP), platelet and IL-6 levels correlate [Citation77]. IL-6 induces aggregation and activation of platelets, processes associated with clot formation and vessel occlusion in atherothrombosis [Citation69,70]. In mice given exogenous IL-6 by infusion, the number of circulating platelets and the number of activated platelets increases and thrombus formation accelerates [Citation76,78]. Production of fibrinogen, which promotes platelet aggregation and increases plasma viscosity, is stimulated by IL-6 [Citation79,80]. Oleksowicz et al. [Citation81] showed that upon incubation with IL-6, human platelets display increased expression of P-selectin, a marker of activation. However, in contrast to the findings of Oleksowicz et al. a recent study by Marino and colleagues [Citation82] found that IL-6 itself in vitro does not induce platelet expression of P-selectin or platelet aggregation. This seemingly contradicts the findings of Oleksowicz et al. and resolution awaits further study, but may be related to the need for endothelial cell activation of platelets to initiate trans-signalling [Citation82].
IL-6 promotes endothelial cell activation and induces expression of cell adhesion molecules such as ICAM-1, VCAM-1 and E-selectin on endothelial cells by trans-signalling [Citation68,83]. Adhesion molecules produced by endothelial cells lining the arterial wall are a key factor in the development of atherosclerosis because they recruit monocytes that transmigrate, settle in the subendothelium as macrophages and transform into foam cells [Citation84]. A correlation has been observed between IL-6 level and endothelial dysfunction assessed by flow mediated dilatation of the brachial artery in young post- myocardial infarction subjects [Citation85].
Schuett and colleagues [Citation86] used a fusion protein of the IL-6 trans-signalling inhibitor soluble gp130 (sgp130Fc) to block trans-signalling in LDL receptor deficient atherosclerosis-prone mice and found that treatment with this fusion protein reduced atherosclerotic lesion progression and this was accompanied by decreased adhesion molecule expression on the endothelium and lessening of macrophage infiltration in the aorta. This study emphasises the importance of trans-signalling in pro-atherogenic properties of IL-6.
A single nucleotide polymorphism, Asp358Ala, found in exon 9 of the IL-6R gene, results in reduced levels of IL-6R on the cell surface and increased circulating sIL-6R [Citation87]. This leads to impaired classical signalling, reduced CRP and fibrinogen and lowering of coronary heart disease risk [Citation88]. The circulating IL-6R may have buffering properties, rendering some of the IL-6 in the circulation inactive [Citation87]. These findings support the role of inflammation in atherosclerosis, currently being tested in the Cardiovascular Inflammation Reduction (CIRT) trial [Citation89].
IL-6 and macrophage recruitment and polarisation
IL-6 is also secreted at different levels depending on macrophage polarisation. Monocytes that transmigrate into the subendothelial space in the artery may become polarised into the pro-inflammatory classical M1 or anti-inflammatory M2 macrophage subtype. The M1 phenotype, linked to the development of atherosclerosis, produces inflammatory cytokines that trigger acute inflammation while M2 macrophages are involved in healing and resolution [Citation90–Citation92]. The extracellular microenvironment, including inflammatory mediators and cytokines and the cells that produce them, influences phenotype. A number of studies have shown that factors that polarise macrophages towards the M1 phenotype such as native LDL and advanced glycation end products result in increased IL-6 production [Citation93,94].
In contrast, the complement component C1q modulates the properties of lipid-loaded macrophage foam cells towards an anti-inflammatory efferocytic resolving M2 phenotype and this change is associated with reduced IL-6 production [Citation95,96]. Similarly, vitamin D and nitroxyl, a novel nitrogen oxide with atheroprotective properties, each also move macrophages towards the M2 phenotype with concomitant reduction in IL-6 [Citation97,98].
Macrophage infiltration into adipose tissue is an important inflammatory factor affecting insulin resistance and the diabetic state [Citation99]. The macrophages in adipose tissue in the obese condition are predominantly M1 and preventing the accumulation of these macrophages by blocking IL-6 trans-signalling was shown by Kraakman et al. [Citation100] to be beneficial. This group fed C57BL/6 mice a high fat diet for 12 weeks followed by 6 weeks of administration of either sgp130Fc protein to selectively inhibit IL-6 trans-signalling or saline. They found that inhibition of trans-signalling reduced accumulation of macrophages in adipose tissue without undesirable side effects seen with total IL-6 deficiency such as liver inflammation and insulin resistance.
Further studies in mice elucidate the need for classical signalling. Murine studies have shown that under inflammatory conditions such as high fat feeding or endotoxemia, IL-6 moves macrophages in white adipose tissue, brown adipose tissue and liver towards an M2 phenotype and this effect is lost if the mice are bred to have a myeloid-specific IL-6 receptor deficiency [Citation101]. Mice with this lack of the membrane bound IL-6 receptor have more M1 macrophages and less M2 macrophages in these tissues as well as a poorer glucose handling. The M2 polarisation that occurs through IL-6 during inflammation is driven by an enhanced IL-4 response. Without IL-6 classical signalling, the upregulation of the IL-4 receptor, which is a component of the receptor complexes for IL-4 and IL-13, does not occur. In a follow-up study, explants of adipose tissue from obese mice were shown to have M2 macrophages that proliferate at a high rate compared to M2 macrophages from lean mice [Citation102]. High proliferation in the obese mouse explants is due to IL-6 effects and this proliferation is reduced when IL-6 neutralising antibody is introduced. The push towards M2 polarization in adipose tissue is an unexpected example of beneficial effects of IL-6 in an inflammatory state.
Lipids and IL-6
Recognition and internalisation of oxidatively modified lipids such as oxidised LDL by scavenger receptors on macrophages in the vessel wall is a process that leads to foam cell formation [Citation103]. Treating murine RAW264.7 cells with oxidised LDL in culture causes a significant increase in IL-6 mRNA expression compared with mock-treated cells [Citation104]. Macrophage scavenger receptors for oxidised LDL include the scavenger receptor A (SR-A) and CD36 [Citation105]. IL-6 dose-dependently increased mouse peritoneal macrophage CD36 mRNA expression in culture [Citation106]. Hashizume and Mihara [Citation107] found that IL-6 promoted oxidised LDL accumulation in the THP-1 human monocyte/macrophage cell line. In these THP-1 macrophages, IL-6 increased expression of SR-A, but not of the lectin like oxidised LDL receptor (LOX-1). In a separate study, these same authors found that IL-6, in the presence of sIL-6R induced SR-A, but not LOX-1 or CD36, in human arterial endothelium in culture [Citation108]. Further, oxidised LDL-induced production of monocyte chemoattractant protein-1 by this cultured endothelium was enhanced in the presence of IL-6, consistent with a role for IL-6 in endothelial activation.
The results of Hashizume and Mihara’s work in THP-1 stand in direct contrast to earlier findings by Liao et al. [Citation109]. In 1999, Liao’s group found that IL-6 prevented lipid overload by interfering with binding and uptake of acetylated LDL by both THP-1 macrophages and human monocyte-derived macrophages and inhibiting SR-A expression by these macrophages. The difference in outcome in these studies leaves open the question of what happens in vivo in humans. Interestingly, IL-6 can also act in an atheroprotective manner by increasing cholesterol efflux to apolipoprotein A1 (apoA1) in macrophages by upregulating ATP binding cassette transporter (ABC) A1 [Citation110]. ABCA1 mediates active efflux of cholesterol from cells to apolipoproteins (prevents foam cell formation). ABCA1 functions as a rate-controlling protein in the apoA1 – dependent active transport of cholesterol and phospholipids [Citation111]. In this study, ABCA1 moved cholesterol only to ApoA-1 and not to high density lipoprotein (HDL), the atheroprotective lipoprotein monitored in the standard lipid profile. However, IL-6 and HDL do interact. HDL inhibits IL-6 production in human umbilical vein endothelial cells by reducing IL-6 mRNA levels in cells stimulated to produce IL-6 by TNF-α [Citation112]. Further, IL-6 stimulation of bovine endothelial cells induces the endothelium to bind and transport HDL [Citation113].
In human subjects, high IL-6 levels are associated with low HDL, independent of multiple potential confounding variables [Citation114]. Atheroprotective functions of HDL involve transport of cholesterol from the subendothelial intima back to the circulating blood, antithrombotic actions, dampening of inflammation and lowering of oxidative stress [Citation115,116].
HDL also attenuates induction of an M1 inflammatory phenotype in cultured human macrophages [Citation117]. Incubation of human monocyte-derived macrophages under conditions that lead to M1 polarisation (LPS + IFN- γ) in the presence of human HDL leads to diminished expression of M1-related cytokines, including IL-6. Similar results were found in mouse bone marrow-derived macrophages treated with human HDL [Citation118]. In these murine macrophages, human HDL inhibited production of M1 cytokines, including IL-6, while also inducing M2 markers, even in the presence of IFN-γ.
Treatment through IL-6 blockade: cardiovascular risk in rheumatoid arthritis
Cardiovascular disease is the main cause of death in the rheumatoid arthritis population [Citation119]. In persons with rheumatoid arthritis, the most commonly used first line therapy is methotrexate, a drug that is known to reduce cardiovascular risk [Citation120]. Methotrexate is also being evaluated for efficacy in reducing cardiovascular events in persons with type 2 diabetes and metabolic syndrome in the CIRT [Citation121,122]. Tocilizumab, a recombinant humanised anti-human IL-6 receptor monoclonal antibody, has been approved for use in combination with methotrexate or other anti-rheumatic drugs or as monotherapy in rheumatoid arthritis, but it is unclear whether tocalizumab confers cardioprotection [Citation123]. This is an important issue in deciding whether to use tocilizumab as an add on to methotrexate or upon discontinuation of methotrexate (switch strategy). Tocilizumab blocks the binding of IL-6 to the gp80 IL-6R expressed on different cell types, preventing inflammatory manifestations of elevated IL-6 [Citation124]. In persons with inflammatory diseases, tocalizumab alleviates symptoms. In rheumatoid arthritis, tocalizumab administration leads to improved disease activity scores, normalisation of acute phase proteins and decreased joint damage [Citation125]. Unfortunately, tocilizumab can increase body weight and elevate triglycerides and cholesterol [Citation126–Citation128]. A recent proteomic study in 20 female subjects with moderate to severe rheumatoid arthritis showed that tocilizumab changed HDL composition, reducing atherogenic proteins such as serum amyloid A [Citation129]. Tocilizumab can also reduce circulating levels of lipoprotein (Lp)(a), a predictor of vascular events and an independent risk factor for atherosclerotic cardiovascular disease containing a highly glycosylated apolipoprotein (a), covalently attached to apolipoprotein B-100 [Citation130–Citation133]. Lp(a) levels are elevated in persons with high serum IL-6. In rheumatoid arthritis patients, lowering of serum Lp(a) is a specific effect through IL-6 not observed with TNF-α inhibition [Citation133]. After 12 weeks of tocilizumab treatment, patients with rheumatoid arthritis showed reduced ApoLp(a) within LDL particles [Citation129].
Statins may be helpful in mitigating adverse IL-6 effects on lipid profile [Citation134]. A potential cardiovascular benefit of tocalizumab, reduced arterial stiffness, is seen with other biologics as well [Citation135]. Although more data is needed, evidence available at this time does not support a clinically relevant cardioprotective effect for tocalizumab in persons with inflammatory disorders such as rheumatoid arthritis [Citation136].
Conclusions
The data associating IL-6 with atherosclerotic cardiovascular disease are compelling, but there is also information indicating that this pleiotropic cytokine can act to reduce or resolve inflammation and protect the cardiovascular system [Citation137]. Although IL-6 neutralisation with tocalizumab has not been shown to increase clinical cardiovascular events, it does affect the lipid profile adversely by raising LDL and triglycerides [Citation138]. Mouse models are similarly contradictory with studies showing acceleration of atherosclerosis such as that of Huber et al. [Citation80] in which IL-6 injection increased atherosclerotic lesion area vs. saline-treatment and that of Luchtefeld et al. [Citation139] demonstrating that hepatocyte-specific gp130 knockout lessens atherosclerosis. On the other hand, apolipoprotein E and IL-6 deficient double knockout mice have more atherosclerosis as measured by oil red O stain than ApoE single knockouts, arguing for an atheroprotective role of IL-6 [Citation140].
A meta-analysis examining the IL-6R showed that a specific variant in this allele, Asp358Ala, that affects signalling, is associated with decreased CRP and fibrinogen and lower risk of coronary artery disease, leaving the door open to targeting of the IL-6R as a treatment approach to atherosclerosis [Citation141].
Disclosure statement
The authors report no conflicts of interest.
Funding
This work was supported by the American Heart Association under [grant number 16GRNT26430041] and by The Elizabeth Daniell Research Fund.
Acknowledgements
We thank Janet and Robert Buescher for their generous support.
References
- Hirano T, Taga T, Nakano N, et al. Purification to homogeneity and characterization of human B-cell differentiation factor (BCDF or BSFp-2). Proc Nat Acad Sci USA. 1985;82:5490–5494.10.1073/pnas.82.16.5490
- Hirano T, Yasukawa K, Harada H, et al. Complementary DNA for a novel human Interleukin (BSF-2) that induces B lymphocytes to produce immunoglobulin. Nature. 1986;324:73–76.10.1038/324073a0
- Hirano T, Matsuda T, Hosoi K, et al. Absence of antiviral activity in recombinant B cell stimulatory factor 2 (BSF-2). Immunol Lett. 1988;17:41–45.10.1016/0165-2478(88)90099-5
- Kamimura D, Ishihara K, Hirano T. IL-6 signal transduction and its physiological roles: the signal orchestration model. Rev Physiol Biochem Pharmacol. 2003;149:1–38.
- Libby P, Ridker PM, Maseri A. Inflammation and atherosclerosis. Circulation. 2002;105:1135–1143.10.1161/hc0902.104353
- Tedgui A, Mallat Z, Cytokines in Harker JA, et al. Late Interleukin-6 escalates T follicular helper cell responses and controls a chronic viral infection. Science. 2011;334:825–829.
- Tedgui A, Mallat Z. Cytokines in atherosclerosis: pathogenic and regulatory pathways. Physiol Rev. 2006;86:515–581.
- Cimmino G, Loffredo FS, Morello A, et al. Immune-inflammatory activation in acute coronary syndromes: a look into the heart of unstable coronary plaque. Curr Cardiol Rev. 2016;13 Oct. [Epub ahead of print].
- Okada M, Kitahara M, Kishimoto S, et al. IL-6/BSF-2 functions as a killer helper factor in the in vitro induction of cytotoxic T cells. J Immunol. 1988;141:1543–1549.
- Ma CS, Deenick EK, Batten M, et al. The origins, function, and regulation of T follicular helper cells. J Exp Med. 2012;209:1241–1253.10.1084/jem.20120994
- Bettelli E, Carrier Y, Gao W, et al. Reciprocal developmental pathways for the generation of pathogenic effector TH17 and regulatory T cells. Nature. 2006;441:235–238.10.1038/nature04753
- Korn T, Bettelli E, Oukka M, et al. IL-17 and Th17 cells. Ann Rev Immunol. 2009;27:485–517.10.1146/annurev.immunol.021908.132710
- Craft JE. Follicular helper T cells in immunity and systemic autoimmunity. Nat Rev Rheumatol. 2012;8:337–347.10.1038/nrrheum.2012.58
- Yoshizaki K, Matsuda T, Nishimoto N, et al. Pathogenic significance of Interleukin-6 (IL-6/BSF-2) in Castleman’s disease. Blood. 1989;74:1360–1367.
- Bettelli E, Carrier Y, Gao W, et al. Reciprocal developmental pathways for the generation of pathogenic effector TH17 and regulatory T cells. Nature. 2006;441:235–238.10.1038/nature04753
- Kopf M, Baumann H, Freer G, et al. Impaired immune and acute phase responses in Interleukin-6-deficient mice. Nature. 1994;368:339–342.10.1038/368339a0
- Thompson JC, Jayne C, Thompson J, et al. A brief elevation of serum amyloid A is sufficient to increase atherosclerosis. J Lipid Res. 2015;56:286–293.10.1194/jlr.M054015
- Rizzo M, Corrado E, Coppola G, et al. Markers of inflammation are strong predictors of subclinical and clinical atherosclerosis in women with hypertension. Coron Artery Dis. 2009;20:15–20.10.1097/MCA.0b013e3283109065
- Heinrich PC, Castell JV, Andus T. Interleukin-6 and the acute phase response. Biochem J. 1990;265:621–636.10.1042/bj2650621
- Kaplanski G, Marin V, Montero-Julian F, et al. Farnarier C.IL-6: a regulator of the transition from neutrophil to monocyte recruitment during inflammation. Trends Immunol. 2003;24:25–29.10.1016/S1471-4906(02)00013-3
- Cronstein BN. Interleukin-6 – a key mediator of systemic and local symptoms in rheumatoid arthritis. Bull NYU Hosp Jt Dis. 2007;65:S11–S15.
- Wung BS, Ni CW, Wang DL. ICAM-1 induction by TNFalpha and IL-6 is mediated by distinct pathways via Rac in endothelial cells. J Biomed Sci. 2005;12:91–101.10.1007/s11373-004-8170-z
- Kerr R, Stirling D, Ludlam CA. Interleukin 6 and haemostasis. Br J Hematol. 2001;115:3–12.10.1046/j.1365-2141.2001.03061.x
- Kopf M, Ramsay A, Brombacher F, et al. Pleiotropic defects of IL-6-deficient mice including early hematopoiesis, T and B cell function, and acute phase responses. Ann NY Acad Sci. 1995;762:308–318.
- Starkie R, Ostrowski SR, Jauffred S, et al. Exercise and IL-6 infusion inhibit endotoxin-induced TNF-α production in humans. FASEB J. 2003;17:884–886.
- Tilg H, Dinarello CA, Mier JW. IL-6 and APPs: anti-inflammatory and immunosuppressive mediators. Immunol Today. 1997;18:428–432.10.1016/S0167-5699(97)01103-1
- Tilg H, Trehu E, Atkins MB, et al. Interleukin-6 (IL-6) as an anti-inflammatory cytokine: induction of circulating IL-1 receptor antagonist and soluble tumor necrosis factor receptor p55. Blood. 1994;83:113–118.
- Schindler R, Mancilla J, Endres S, et al. Correlations and interactions in the production of Interleukin-6 (IL-6), IL-1, and tumor necrosis factor (TNF) in human blood mononuclear cells: IL-6 suppresses IL-1 and TNF. Blood. 1990;75:40–47.
- Steensberg A, Fischer CP, Keller C, et al. IL-6 enhances plasma IL-1ra, IL-10, and cortisol in humans. Am J Physiol Endocrinol Metab. 2003;285:E433–E437.10.1152/ajpendo.00074.2003
- Lotz M, Guerne PA. Interleukin-6 induces the synthesis of tissue inhibitor of metalloproteinases-1/erythroid potentiating activity (TIMP-1/EPA). J Biol Chem. 1991;266:2017–2020.
- Silacci P, Dayer JM, Desgeorges A, et al. Interleukin (IL)-6 and its soluble receptor induce TIMP-1 expression in synoviocytes and chondrocytes, and Block IL-1-induced collagenolytic activity. J Biol Chem. 1998;273:13625–13629.10.1074/jbc.273.22.13625
- Somers W, Stahl M, Seehra JS. 1.9 A crystal structure of Interleukin 6: implications for a novel mode of receptor dimerization and signaling. EMBO J. 1997;16:989–997.10.1093/emboj/16.5.989
- Dayer J, Choy E. Therapeutic targets in rheumatoid arthritis: the Interleukin-6 receptor. Rheumatology. 2010;49:15–24.10.1093/rheumatology/kep329
- Cron L, Allen T, Febbraio MA. The role of gp130 receptor cytokines in the regulation of metabolic homeostasis. J Exp Biol. 2016;219:259–265.10.1242/jeb.129213
- Garbers C, Hermanns HM, Schaper F, et al. Plasticity and cross-talk of Interleukin 6-type cytokines. Cytokine Growth Factor Rev. 2012;23:85–97.10.1016/j.cytogfr.2012.04.001
- Schaper F, Rose-John S. Interleukin-6: biology, signaling and strategies of blockade. Cytokine Growth Factor Rev. 2015;26:475–487.10.1016/j.cytogfr.2015.07.004
- Yamasaki K, et al. Cloning and expression of the human Interleukin-6 (BSF-2/IFN beta 2) receptor. Science. 1988;241:825–828.10.1126/science.3136546
- Gooz M. ADAM-17: the enzyme that does it all. Crit Rev Biochem Mol Biol. 2010;45:146–169.10.3109/10409231003628015
- Rose-John S, Waetzig GH, Scheller J, et al. The IL-6/sIL-6R complex as a novel target for therapeutic approaches. Expert Opin Ther Targets. 2007;11:613–624.10.1517/14728222.11.5.613
- Waetzig GH, Chalaris A, Rosenstiel P, et al. N-linked glycosylation is essential for the stability but not the signaling function of the Interleukin-6 signal transducer glycoprotein 130. J Biol Chem. 2010;285:1781–1789.10.1074/jbc.M109.075952
- Heinrich PC, Behrmann I, Haan S, et al. Principles of Interleukin (IL)-6-type cytokine signalling and its regulation. Biochem J. 2003;374:1–20.10.1042/bj20030407
- Schindler C, Darnell JE Jr. Transcriptional responses to polypeptide ligands: the JAK-STAT pathway. Ann Rev Biochem. 1995;64:621–652.10.1146/annurev.bi.64.070195.003201
- Garbers C, Aparicio-Siegmund S, Rose-John S. The IL-6/gp130/STAT3 signaling axis: recent advances towards specific inhibition. Curr Opin Immunol. 2015;34:75–82.10.1016/j.coi.2015.02.008
- Wolf J, Rose-John S, Garbers C. Interleukin-6 and its receptors: a highly regulated and dynamic system. Cytokine. 2014;70:11–20.10.1016/j.cyto.2014.05.024
- Gabay C. Interleukin-6 and chronic inflammation. Arthritis Res Ther. 2006;8:S3.10.1186/ar1917
- Sodenkamp J, Waetzig GH, Scheller J, et al. Therapeutic targeting of Interleukin-6 trans-signaling does not affect the outcome of experimental tuberculosis. Immunobiology. 2012;217:996–1004.10.1016/j.imbio.2012.01.015
- Hoge J, Yan I, Jänner N, et al. IL-6 controls the innate immune response against listeria monocytogenes via classical IL-6 signaling. J Immunol. 2013;190:703–711.10.4049/jimmunol.1201044
- Barkhausen T, Tschernig T, Rosenstiel P, et al. Selective blockade of Interleukin-6 trans -signaling improves survival in a murine polymicrobial sepsis model. Crit Care Med. 2011;39:1407–1413.10.1097/CCM.0b013e318211ff56
- Harris TB, Ferrucci L, Tracy RP, et al. Associations of elevated Interleukin-6 and C-reactive protein levels with mortality in the elderly. Am J Med. 1999;106:506–512.10.1016/S0002-9343(99)00066-2
- Mendall MA, Patel P, Asante M, et al. Relation of serum cytokine concentrations to cardiovascular risk factors and coronary heart disease. Heart. 1997;78:273–277.10.1136/hrt.78.3.273
- Ridker PM, Rifai N, Stampfer MJ, et al. Plasma concentration of Interleukin-6 and the risk of future myocardial infarction among apparently healthy men. Circulation. 2000;101:1767–1772.10.1161/01.CIR.101.15.1767
- Zakai NA, Katz R, Jenny NS, et al. Inflammation and hemostasis biomarkers and cardiovascular risk in the elderly: the Cardiovascular Health Study. J Thromb Haemost. 2007;5:1128–1135.10.1111/jth.2007.5.issue-6
- Lefkou E, Fragakis N, Ioannidou E, et al. Increased levels of proinflammatory cytokines in children with family history of coronary artery disease. Clin Cardiol. 2010;33:E6–10.
- Fisman EZ, Benderly M, Esper RJ, et al. Interleukin-6 and the risk of future cardiovascular events in patients with angina pectoris and/or healed myocardial infarction. Am J Cardiol. 2006;98:14–18.10.1016/j.amjcard.2006.01.045
- Fan ZX, Hua Q, Li YP, et al. Interleukin-6, but not soluble adhesion molecules, predicts a subsequent mortality from cardiovascular disease in patients with acute ST-segment elevation myocardial infarction. Cell Biochem Biophys. 2011;61:443–448.10.1007/s12013-011-9209-1
- Schieffer B, Schieffer E, Hilfiker-Kleiner D, et al. Expression of angiotensin II and Interleukin 6 in human coronary atherosclerotic plaques : potential implications for inflammation and plaque instability. Circulation. 2000;101:1372–1378.10.1161/01.CIR.101.12.1372
- Fuchs M, Hilfiker A, Kaminski K, et al. Role of Interleukin-6 for LV remodeling and survival after experimental myocardial infarction. FASEB J. 2003;17:2118–2120.
- Hartman MH, Vreeswijk-Baudoin I, Groot HE, et al. Inhibition of Interleukin-6 receptor in a murine model of myocardial ischemia-reperfusion. Plos One. 2016;11:e0167195.10.1371/journal.pone.0167195
- Haffner SM, Lehto S, Rönnemaa T, et al. Mortality from coronary heart disease in subjects with Type 2 diabetes and in nondiabetic subjects with and without prior myocardial infarction. N Engl J Med. 1998;339:229–234.10.1056/NEJM199807233390404
- Fernandez-Real JM, Ricart J. Insulin resistance and chronic cardiovascular inflammatory syndrome. Endocr Rev. 2003;24:278–301.10.1210/er.2002-0010
- Kado S, Nagase T, Nagata N. Circulating levels of interleukin-6, its soluble receptor and interleukin-6/interleukin-6 receptor complexes in patients with type 2 diabetes mellitus. Acta Diabetol. 1999;36:67–72.10.1007/s005920050147
- Pickup JC, Chusney GD, Thomas SM, et al. Plasma interleukin-6, tumour necrosis factor alpha and blood cytokine production in Type 2 diabetes. Life Sci. 2000;67:291–300.10.1016/S0024-3205(00)00622-6
- Pedersen M, Bruunsgaard H, Weis N, et al. Circulating levels of TNF-alpha and IL-6-relation to truncal fat mass and muscle mass in healthy elderly individuals and in patients with Type-2 diabetes. Mech Ageing Dev. 2003;124:495–502.10.1016/S0047-6374(03)00027-7
- Lowe G, Woodward M, Hillis G, et al. Circulating inflammatory markers and the risk of vascular complications and mortality in people with Type 2 diabetes and cardiovascular disease or risk factors: the advance study. Diabetes. 2014;63:1115–1123.10.2337/db12-1625
- Kahn A, Jing N, Li JH, et al. Role of JAK/STAT pathway in IL-6-induced activation of vascular smooth muscle cells. Am J Nephrol. 2004;24:387–392.
- Ikeda U, Ikeda M, Oohara T, et al. Interleukin 6 stimulates growth of vascular smooth muscle cells in a PDGF-dependent manner. Am J Physiol. 1991;260:H1713–H1717.
- Morimoto S, Nabata T, Koh E, et al. Interleukin-6 stimulates proliferation of cultured vascular smooth muscle cells independently of interleukin-1 beta. J Cardiovasc Pharmacol. 1991;17:S117–S118.10.1097/00005344-199117002-00026
- Wung BS, Hsu MC, Wu CC, et al. Resveratrol suppresses IL-6-induced ICAM-1 gene expression in endothelial cells: effects on the inhibition of STAT3 phosphorylation. Life Sci. 2005;78:389–397.10.1016/j.lfs.2005.04.052
- Davi G, Patrono C. Platelet activation and atherothrombosis. N Engl J Med. 2007;357:2482–2494.10.1056/NEJMra071014
- Oleksowicz L, Mrowiec Z, Zuckerman D, et al. Platelet activation induced by interleukin-6: evidence for a mechanism involving arachidonic acid metabolism. Thromb Haemost. 1994;72:302–308.
- Gierens H, Nauck M, Roth M, et al. Interleukin-6 stimulates LDL receptor gene expression via activation of sterol-responsive and Sp1 binding elements. Arterioscler Thromb Vasc Biol. 2000;20:1777–1783.10.1161/01.ATV.20.7.1777
- Klouche M, Bhakdi S, Hemmes M, et al. Novel path to activation of vascular smooth muscle cells: up-regulation of gp130 creates an autocrine activation loop by IL-6 and its soluble receptor. J Immunol. 1999;163:4583–4589.
- Selzman CH, Miller SA, Zimmerman MA, et al. Monocyte chemotactic protein-1 directly induces human vascular smooth muscle proliferation. Am J Physiol Heart Circ Physiol. 2002;283:H1455–H1461.10.1152/ajpheart.00188.2002
- Ishibashi T, Kimura H, Shikama Y, et al. Interleukin-6 is a potent thrombopoietic factor in vivo in mice. Blood. 1989;74:1241–1244.
- Laterveer L, van Damme J, Willemze R, et al. Continuous infusion of interleukin-6 in sublethally irradiated mice accelerates platelet reconstitution and the recovery of myeloid but not of megakaryocytic progenitor cells in bone marrow. Exp Hematol. 1993;21:1621–1627.
- Ceresa IF, Noris P, Ambaglio C, et al. Thrombopoietin is not uniquely responsible for thrombocytosis in inflammatory disorders. Platelets. 2007;18:579–582.10.1080/09537100701593601
- Heits F, Stahl M, Ludwig D, et al. Elevated serum thrombopoietin and Interleukin-6 concentrations in thrombocytosis associated with inflammatory bowel disease. J Interferon Cytokine Res. 1999;19:757–760.10.1089/107999099313604
- Yan SL, Russell J, Granger DN. Platelet Activation and platelet-leukocyte aggregation elicited in experimental colitis are mediated by Interleukin-6. Inflamm Bowel Dis. 2014;20:353–362.10.1097/01.MIB.0000440614.83703.84
- Tsakadze NL, Zhao Z, D’Souza SE. Interaction of inter-cellular adhesion molecule-1 with fibrinogen. Trends Cardiovasc Med. 2002;12:101–108.10.1016/S1050-1738(01)00157-8
- Huber SA, Sakkinen P, Conze D, et al. Interleukin-6 exacerbates early atherosclerosis in mice. Arterioscler Thromb Vasc Biol. 1999;19:2364–2367.10.1161/01.ATV.19.10.2364
- Oleksowicz L, Mrowiec Z, Isaacs R, et al. Morphologic and ultrastructural evidence of interleukin-6 induced platelet activation. Am J Hematol. 1995;48:92–99.10.1002/ajh.v48.2
- Marino M, Scuderi F, Ponte E, et al. Novel path to IL-6 trans-signaling through thrombin-induced soluble IL-6 receptor release by platelets. J Biol Regul Homeost Agents. 2013;27:841–852.
- Chen Q, Fisher DT, Clancy KA, et al. Fever-range thermal stress promotes lymphocyte trafficking across high endothelial venules via an interleukin 6 trans-signaling mechanism. Nat Immunol. 2006;7:1299–1308.10.1038/ni1406
- Patel SS, Thiagarajan R, Willerson JT, et al. Inhibition of α4 integrin and ICAM-1 markedly attenuate macrophage homing to atherosclerotic plaques in ApoE-deficient mice. Circulation. 1998;97:75–81.10.1161/01.CIR.97.1.75
- Erzen B, Sabovic M, Sebestjen M, et al. Interleukin-6 correlates with endothelial dysfunction in young post-myocardial infarction patients. Cardiology. 2007;107:111–116.
- Schuett H, Oestreich R, Waetzig GH, et al. Transsignaling of Interleukin-6 crucially contributes to atherosclerosis in mice. Arterioscler Thromb Vasc Biol. 2012;32:281–290.10.1161/ATVBAHA.111.229435
- Ferreira RC, Freitag DF, Cutler AJ, et al. Functional IL6R 358Ala allele impairs classical IL-6 receptor signaling and influences risk of diverse inflammatory diseases. PLoS Genet. 2013;9:e1003444.10.1371/journal.pgen.1003444
- Swerdlow DI, Holmes MV, Kuchenbaecker KB, et al. The interleukin-6 receptor as a target for prevention of coronary heart disease: a mendelian randomisation analysis. Lancet. 2012;379:1214–1224. Interleukin-6 Receptor Mendelian Randomisation Analysis (IL6R MR) Consortium.
- Ridker PM. From C-reactive protein to Interleukin-6 to Interleukin-1: moving upstream to identify novel targets for atheroprotection. Circ Res. 2016;118:145–156.10.1161/CIRCRESAHA.115.306656
- Liu YC, Zou XB, Chai YF, et al. Macrophage polarization in inflammatory diseases. Int J Biol Sci. 2014;10:520–529.10.7150/ijbs.8879
- Mantovani A, Garlanda C, Locati M. Macrophage diversity and polarization in atherosclerosis: a question of balance. Arterioscler Thromb Vasc Biol. 2009;29:1419–1423.10.1161/ATVBAHA.108.180497
- El Hadri K, Mahmood DF, Couchie D, et al. Thioredoxin-1 promotes anti-inflammatory macrophages of the M2 phenotype and antagonizes atherosclerosis. Arterioscler Thromb Vasc Biol. 2012;32:1445–1452.10.1161/ATVBAHA.112.249334
- Al-Sharea A, Lee MK, Moore XL, et al. Native LDL promotes differentiation of human monocytes to macrophages with an inflammatory phenotype. Thromb Haemost. 2016;115:762–772.
- Jin X, Yao T, Zhou Z, et al. Advanced glycation end products enhance macrophages polarization into M1 phenotype through activating RAGE/NF-κB pathway. Biomed Res Int. 2015;2015:732450.
- Ho MM, Manughian-Peter A, Spivia WR, et al. Macrophage molecular signaling and inflammatory responses during ingestion of atherogenic lipoproteins are modulated by complement protein C1q. Atherosclerosis. 2016;253:38–46.
- Spivia W, Magno PS, Le P, et al. Complement protein C1q promotes macrophage anti-inflammatory M2-like polarization during the clearance of atherogenic lipoproteins. Inflamm Res. 2014;63:885–893.10.1007/s00011-014-0762-0
- Andrews KL, Sampson AK, Irvine JC, et al. Nitroxyl (HNO) reduces endothelial and monocyte activation and promotes M2 macrophage polarization. Clin Sci (Lond). 2016;130:1629–1640.10.1042/CS20160097
- Yin K, You Y, Swier V, et al. Vitamin D protects against atherosclerosis via regulation of cholesterol efflux and macrophage polarization in hypercholesterolemic swine. Arterioscler Thromb Vasc Biol. 2015;35:2432–2442.10.1161/ATVBAHA.115.306132
- Hill AA, Reid Bolus W, Hasty AH. A decade of progress in adipose tissue macrophage biology. Immunol Rev. 2014;262:134–152.10.1111/imr.2014.262.issue-1
- Kraakman MJ, Kammoun HL, Allen TL, et al. Blocking IL-6 trans-signaling prevents high-fat diet-induced adipose tissue macrophage recruitment but does not improve insulin resistance. Cell Metab. 2015;21:403–416.10.1016/j.cmet.2015.02.006
- Mauer J, Chaurasia B, Goldau J, et al. Signaling by IL-6 promotes alternative activation of macrophages to limit endotoxemia and obesity-associated resistance to insulin. Nat Immunol. 2014;15:423–430.10.1038/ni.2865
- Braune J, Weyer U, Hobusch C, et al. IL-6 regulates M2 polarization and local proliferation of adipose tissue macrophages in obesity. J Immunol. 2016;198:2927–2934.
- Levitan I, Volkov S. Oxidized LDL: diversity, patterns of recognition, and pathophysiology. Antioxid Redox. 2010;13:39–75.10.1089/ars.2009.2733
- Wang R, Zhang Y, Xu L, et al. Protein inhibitor of activated STAT3 suppresses oxidized LDL-induced Cell responses during atherosclerosis in apolipoprotein E-deficient mice. Sci Rep. 2016;6:36790.10.1038/srep36790
- Reiss AB, Glass AD. CD36 and ScR-A: scavenger receptors that mediate uptake of oxidized LDL and foam cell formation. In: Reiss A, Carsons S, Cronstein B, editors. Proteins involved in the pathogenesis of atherosclerosis. Trivandrum, Kerala: Research Signpost; 2006. p. 1–12.
- Keidar S, Heinrich R, Kaplan M, et al. Angiotensin II administration to atherosclerotic mice increases macrophage uptake of oxidized LDL: a possible role for Interleukin-6. Arterioscler Thromb Vasc Biol. 2001;21:1464–1469.10.1161/hq0901.095547
- Hashizume M, Mihara M. Atherogenic effects of TNF-α and IL-6 via up-regulation of scavenger receptors. Cytokine. 2012;58:424–430.10.1016/j.cyto.2012.02.010
- Hashizume M, Mihara M. Blockade of IL-6 and TNF-α inhibited oxLDL-induced production of MCP-1 via scavenger receptor induction. Eur J Pharmacol. 2012;689:249–254.10.1016/j.ejphar.2012.05.035
- Liao HS, Matsumoto A, Itakura H, et al. Transcriptional inhibition by Interleukin-6 of the class A macrophage scavenger receptor in macrophages derived from human peripheral monocytes and the THP-1 monocytic cell line. Arterioscler Thromb Vasc Biol. 1999;19:1872–1880.10.1161/01.ATV.19.8.1872
- Frisdal E, Lesnik P, Olivier M, et al. Interleukin-6 protects human macrophages from cellular cholesterol accumulation and attenuates the proinflammatory response. J Biol Chem. 2011;286:30926–30936.10.1074/jbc.M111.264325
- Voloshyna I, Reiss AB. The ABC transporters in lipid flux and atherosclerosis. Prog Lipid Res. 2011;50:213–224.10.1016/j.plipres.2011.02.001
- Gomaraschi M, Basilico N, Sisto F, et al. High-density lipoproteins attenuate interleukin-6 production in endothelial cells exposed to pro-inflammatory stimuli. Biochim Biophys Acta. 2005;1736:136–143.10.1016/j.bbalip.2005.08.003
- Robert J, Lehner M, Frank S, et al. Interleukin 6 stimulates endothelial binding and transport of high-density lipoprotein through induction of endothelial lipase. Arterioscler Thromb Vasc Biol. 2013;33:2699–2706.10.1161/ATVBAHA.113.301363
- Zuliani G, Volpato S, Blè A, et al. High Interleukin-6 plasma levels are associated with low HDL-C levels in community-dwelling older adults: the inchianti study. Atherosclerosis. 2007;192:384–390.10.1016/j.atherosclerosis.2006.05.024
- Cucuianu M, Coca M, Hancu N. Reverse cholesterol transport and atherosclerosis. a mini review. Rom J Intern Med. 2007;45:17–27.
- Oda MN. High-density lipoprotein cholesterol: origins and the path ahead. Curr Opin Endocrinol Diabetes Obes. 2015;22:133–141.10.1097/MED.0000000000000139
- Lee MK, Moore XL, Fu Y, et al. High-density lipoprotein inhibits human M1 macrophage polarization through redistribution of caveolin-1. Br J Pharmacol. 2016;173:741–751.10.1111/bph.v173.4
- Sanson M, Distel E, Fisher EA. HDL induces the expression of the M2 macrophage markers Arginase 1 and Fizz-1 in a STAT6-dependent process. PLoS One. 2013;8:e74676.10.1371/journal.pone.0074676
- Naz SM, Symmons DP. Mortality in established rheumatoid arthritis. Best Pract Res Clin Rheumatol. 2007;21:871–883.10.1016/j.berh.2007.05.003
- Choi HK, Hernan MA, Seeger JD, et al. Methotrexate and mortality in patients with rheumatoid arthritis: a prospective study. Lancet. 2002;359:1173–1177.10.1016/S0140-6736(02)08213-2
- Everett BM, Pradhan AD, Solomon DH, et al. Rationale and design of the cardiovascular inflammation reduction trial: a test of the inflammatory hypothesis of atherothrombosis. Am Heart J. 2013;166:199–207.10.1016/j.ahj.2013.03.018
- Ridker PM. Testing the inflammatory hypothesis of atherothrombosis: scientific rationale for the cardiovascular inflammation reduction trial (CIRT). J Thromb Haemost. 2009;7:332–339.10.1111/jth.2009.7.issue-s1
- Balsa A, Tovar Beltran JV, Caliz R, et al. Patterns of use and dosing of tocilizumab in the treatment of patients with rheumatoid arthritis in routine clinical practice: the ACT-LIFE study. Rheumatol Int. 2015;35:1525–1534.10.1007/s00296-015-3237-x
- Kaly L, Rosner I. Tocilizumab – a novel therapy for non-organ-specific autoimmune diseases. Best Pract Res Clin Rheumatol. 2012;26:157–165.10.1016/j.berh.2012.01.001
- Hashizume M, Tan SL, Takano J, et al. Tocilizumab, a humanized Anti-IL-6R antibody, as an emerging therapeutic option for rheumatoid arthritis: molecular and cellular mechanistic insights. Int Rev Immunol. 2015;34:265–279.10.3109/08830185.2014.938325
- Hirabayashi Y, Ishii T, Harigae H. Clinical efficacy of tocilizumab in patients with active rheumatoid arthritis in real clinical practice. Rheumatol Int. 2010;30:1041–1048.10.1007/s00296-009-1095-0
- Gabay C, McInnes IB, Kavanaugh A, et al. Comparison of lipid and lipid-associated cardiovascular risk marker changes after treatment with tocilizumab or adalimumab in patients with rheumatoid arthritis. Ann Rheum Dis. 2016;75:1806–1812.10.1136/annrheumdis-2015-207872
- Genovese MC, McKay JD, Nasonov EL, et al. Interleukin-6 receptor inhibition with tocilizumab reduces disease activity in rheumatoid arthritis with inadequate response to disease-modifying antirheumatic drugs: the tocilizumab in combination with traditional disease-modifying antirheumatic drug therapy. Arthritis Rheum. 2008;58:2968–2980.10.1002/art.v58:10
- Lee JS, Chapman MJ, Piraino P, et al. Remodeling of plasma lipoproteins in patients with rheumatoid arthritis: Interleukin-6 receptor-alpha inhibition with tocilizumab. Proteomics Clin Appl. 2016;10:183–194.10.1002/prca.v10.2
- Marcovina SM, Albers JJ. Lipoprotein (a) measurements for clinical application. J Lipid Res. 2016;57:526–537.10.1194/jlr.R061648
- Müller N, Schulte DM, Türk K, et al. IL-6 blockade by monoclonal antibodies inhibits apolipoprotein (a) expression and lipoprotein (a) synthesis in humans. J Lipid Res. 2015;56:1034–1042.10.1194/jlr.P052209
- Schultz O, Oberhauser F, Saech J, et al. Effects of inhibition of Interleukin-6 signalling on insulin sensitivity and lipoprotein (A) levels in human subjects with rheumatoid diseases. PLoS One. 2010;5:e14328.10.1371/journal.pone.0014328
- Nordestgaard BG, Chapman MJ, Ray K, et al. European atherosclerosis society consensus panel. Lipoprotein(a) as a cardiovascular risk factor: current status. Eur Heart J. 2010;31:2844–2853.10.1093/eurheartj/ehq386
- Soubrier M, Pei J, Durand F, et al. Concomitant use of statins in tocilizumab-treated patients with rheumatoid arthritis: a post hoc analysis. Rheumatol Ther. 2016;Nov 29. [Epub ahead of print].
- Kume K, Amano K, Yamada S, et al. Tocilizumab monotherapy reduces arterial stiffness as effectively as etanercept or adalimumab monotherapy in rheumatoid arthritis: an open-label randomized controlled trial. J Rheumatol. 2011;38:2169–2171.10.3899/jrheum.110340
- Welsh P, Tuckwell K, McInnes IB, et al. Effect of IL-6 receptor blockade on high-sensitivity troponin T and NT-proBNP in rheumatoid arthritis. Atherosclerosis. 2016;254:167–171.10.1016/j.atherosclerosis.2016.10.016
- Scheller J, Chalaris A, Schmidt-Arras D, et al. The pro- and anti-inflammatory properties of the cytokine interleukin-6. Biochim Biophys Acta. 2011;1813:878–888.10.1016/j.bbamcr.2011.01.034
- Md Yusof MY, Emery P. Targeting Interleukin-6 in rheumatoid arthritis. Drugs. 2013;73:341–356.10.1007/s40265-013-0018-2
- Luchtefeld M, Schunkert H, Stoll M, et al. Signal transducer of inflammation gp130 modulates atherosclerosis in mice and man. J Exp Med. 2007;204:1935–1944.10.1084/jem.20070120
- Schieffer B, Selle T, Hilfiker A, et al. Impact of Interleukin-6 on plaque development and morphology in experimental atherosclerosis. Circulation. 2004;110:3493–3500.10.1161/01.CIR.0000148135.08582.97
- Sarwar N, Butterworth AS, Freitag DF, et al. Interleukin-6 receptor pathways in coronary heart disease: a collaborative meta-analysis of 82 studies. Lancet. 2012;379:1205–1213. IL6R Genetics Consortium Emerging Risk Factors Collaboration.