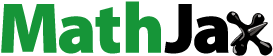
ABSTRACT
Cognitive control is a pivotal aspect of cognition and it is impaired in many clinical populations. To date, several distinct types of cognitive control have been proposed, and prior work demonstrated the instrumental role of basal ganglia, frontal and parietal regions. However, the role of the structural variation of these regions in cognitive control functions is poorly understood. Here, we examined in 39 adults the association between regional brain volume and three major types of cognitive control: (i) stimulus updating, (ii) task-switching, and (iii) distractor filtering. The volume of the globus pallidus was positively correlated with individual variation in task-switching , and was anatomically specific to the left hemisphere. Importantly, this region did not track performance in distractor filtering or stimulus updating. We then aimed to use transcranial direct current stimulation to target the left midline subcortical structures. However, we did not find an effect on task-switching. While the null effect in the brain stimulation prevents us from drawing causal inference from the role of globus pallidus on task-switching, our structural results reveal a novel and highly specific neurostructural mechanism for task-switching and provide a further understanding of the link between cognitive control functions and the human brain.
1. Introduction
Cognitive control is an umbrella term encompassing several cognitive functions including updating and distractor filtering, which are core components of cognition and impaired in various clinical populations such as Parkinson’s disease, attention deficit hyperactivity disorder, schizophrenia, and drug addiction (Cools & D’Esposito, Citation2011). Updating refers to updating of information in working memory and can be classified into two subtypes: (i) updating of low-level information (i.e., stimulus updating); (ii) updating of high-level information such as goals or rules (i.e., task-switching). Distractor filtering refers to the ability to filter out irrelevant information or distractors.
Findings from studies on stimulus updating and task-switching converged in demonstrating the involvement of frontal, parietal and basal ganglia regions (Bledowski et al., Citation2009; Cools et al., Citation2007; Eslinger & Grattan, Citation1993; Lenartowicz et al., Citation2010; Leung et al., Citation2007; Murty et al., Citation2011; Roth & Courtney, Citation2007; Roth et al., Citation2005; Sörqvist & Sætrevik, Citation2010; Takahama et al., Citation2010; Van Holstein et al., Citation2011). Concerning the basal ganglia, bromocriptine, a dopamine D2 receptor agonist, elevated striatal activity during task-switching but not during distractor-filtering in a working-memory task involving faces and scenes (Cools et al., Citation2007). Moreover, in a working memory task featuring maintenance, overwriting and stimulus updating, the latter function compared to the first two revealed a network including caudate, substantial nigra/ventral tegmental area and dorsolateral prefrontal cortex activity (Murty et al., Citation2011). When comparing stimulus updating and task-switching, task-switching preferentially activated the prefrontal cortex (PFC) while stimulus updating preferentially activated the parietal cortex (Montojo & Courtney, Citation2008).
In respect of distractor filtering, failing to filter out irrelevant information leads to unnecessary storage of that information which modulates the parietal lobe (McNab & Klingberg, Citation2008; Vogel et al., Citation2005). However, a preparatory distractor filtering signal in the left globus pallidus which was induced when participants were cued for a forthcoming distractor filtering trial vs. when they were cued for a non-distractor filtering trial, has shown to prevent the unnecessary parietal storage (McNab & Klingberg, Citation2008). These findings have led to the suggestion that the basal ganglia system centered in the left globus pallidus acts as a gatekeeper of information from the environment determining what information is stored and what is filtered out. Apart from basal ganglia and the parietal cortex, other studies demonstrated the involvement of the PFC in distractor filtering (Bledowski et al., Citation2009; Cools & D’Esposito, Citation2011; Cools et al., Citation2007). Taken together, stimulus updating, task-switching and distractor filtering involve subcortical, frontal and parietal regions.
Several studies were conducted on the neurostructural correlates of cognitive control (Alvarez & Emory, Citation2006). A developmental study (Breukelaar et al., Citation2017) identified a negative association between cognitive control and the volume of frontoparietal regions: the left dorsolateral PFC and the bilateral parietal cortex. Moreover, a lesion study identified that individuals with frontostriatal lesions exhibited impaired rule updating (Eslinger & Grattan, Citation1993). In the present study, we aimed to compare the neurostructural contributions of cortical and subcortical regions in stimulus updating, task-switching and distractor filtering using a single task that combines all these three types of cognitive control (), and to examine using brain stimulation the influence of frontal and midline subcortical structures on these three cognitive control components. We aimed to: (i) interrogate whether, as motivated by previous studies, the structure of basal ganglia and frontoparietal regions tracks individual variation in key types of cognitive control, (ii) establish the cognitive specificity of these associations, and lastly (iii) investigate the causal contribution of these regions using transcranial direct current stimulation (tDCS).
2. Methods
2.1. Participants
We recruited 39 participants (17: Stockholm location, 22: Oxford location, age range: 19–46 years) predominantly young adults and university students (Stockholm: mean age = 25.5, standard deviation = 3.6, 9 males, Oxford: mean age = 26.05, standard deviation = 6.5, 16 males). Participants were informed that the study investigated the behavioral and neural mechanisms of spatial memory. In both locations, the completion of the structural acquisition lasted ~20 min, and the completion of the behavioral task lasted ~90 m. In Stockholm, the Karolinska Institute was used as the research location. The task was part of a functional magnetic resonance imaging (fMRI) investigation its aims of which are orthogonal to the current study. The participants from Stockholm did not take part in any stimulation session and did not have any electrodes attached. All the participants in the Oxford location were initially contacted to complete the behavioral task three times, (i) sham session, (ii) DLPFC stimulation session and (iii) midline subcortical structures stimulation session (see below). In Oxford location, 12 additional participants took part in the brain stimulation study but no imaging data were recorded (sample size in the Oxford cohort = 34, from which 22 participants took part to both the imaging and brain stimulation and 12 took part only in the brain stimulation session. Participants in both locations received monetary compensation for their participation. Informed written consent was obtained and the study was approved by the Stockholm’s Ethics Committee and by the University of Oxford’s Medical Sciences Interdivisional Research Ethics Committee (MS-IDREC-C2_2015_016). We excluded missing scores or behavioral or imaging scores that fallen 3 standard deviations beyond the mean.
2.2. MRI data acquisition and pre-processing
In Stockholm, the anatomical high-resolution T1-weighted volume scans (1 mm3) were acquired using a BRAVO sequence (TR = 6.4040s; TE = 2.8080 ms; 180 slices, voxel size=.9375×.9375×1mm). In Oxford, the anatomical high-resolution T1-weighted volume scans (1 mm3) were acquired with a 3 T Siemens MAGNETOM Prisma MRI System equipped with a 32 channel receiver only head coil. Anatomical high-resolution T1-weighted scans were acquired consisting of 192 slices, repetition time (TR) = 1900 ms; echo time (TE) = 3.97 ms; voxel size = 1 × 1 × 1 mm). Since the two studies differed in their image acquisition parameters we controlled for the study location when applicable as discussed below.
The structural analysis was performed by utilizing the recon-all function within FreeSurfer image analysis software v6.0.0, which is documented and freely available for download online surfer.nmr.mgh.harvard.edu. The cortical parcellation was defined based on the standard Desikan-Killiany atlas. Based on the reviewed literature in the introduction, our structural parameters of interest were subcortical and frontoparietal volume (1mm3, see also Supplemental Material 10) of 30 regions (15 per hemisphere). The subcortical regions were the following: caudate, putamen, pallidum; the frontoparietal regions were the following: caudal middle frontal, inferior parietal, lateral orbitofrontal, medial orbitofrontal, pars opercularis, pars orbitalis, pars triangularis, rostral middle frontal, superior frontal, superior parietal, supramarginal, and frontal pole.
2.3. Cognitive control task and dependent variables
The task consisted of three trial-types of interest: (a) information maintenance, (b) distractor filtering, (c) stimulus updating. On a trial-by-trial basis, participants were presented with the following phases:
(i) Preparatory phase: an instruction cue in the form of a geometric shape (square, circle, diamond) signaled the type of cognitive control the participant was about to engage later in the trial. Geometric shapes as instructor cues were utilized in Stockholm. A square instruction cue signaled that the current trial was a ‘maintenance-only’ trial and required maintenance of spatial information but no cognitive control (information maintenance). A triangle signaled a ‘distractor filtering’ trial where participants were asked to maintain spatial information as well as to perform distractor filtering (maintenance+distractor filtering) later in the trial. Lastly, a circle signaled a ‘stimulus updating’ trial in which participants were asked to maintain spatial information and also to update this information (maintenance+stimulus updating). In Oxford, the trial type was instructed with the words ‘baseline’, ‘distractors’, ‘updating’ instead of geometrical shapes. This was done because the participants in Oxford took part in three sessions and the additional requirement of learning to associate geometric shapes with the condition was perceived too taxing and could have led to order effect due to learning. Relatedly, the duration of trial events was shorter in Oxford compared to Stockholm (Figure 1) and was as follows: preparatory (2.5s), encoding (1s), delay 1 (1.5s), executive control (1s), delay 2 (3s).
(ii) Encoding phase: a grid consisted of 16 squares was arranged in a circularly fashion, and depending on the condition (information maintenance, distractor filtering, and stimulus updating), three of these squares were filled with red color, which participants were asked to encode and remember. The main experiment also featured another trial type, maintenance of 5 stimuli, but it was not included in the analyses as it was orthogonal to the aims of the present study.
(iii) Delay 1 phase. The 16 square grid was presented without any stimuli.
(iv) Executive control phase. At this stage, participants were asked to perform maintenance-only (information maintenance), maintenance+distractor filtering (distractor filtering), or maintenance+stimulus updating (stimulus updating), depending on the previously presented instruction cue. During the maintenance-only condition, participants were informed that two of the three previously displayed positions will reappear one in yellow and one in red color. During the distractor filtering condition (distractor filtering) participants were asked to ignore or filter out the red and yellow dots presented during the executive control phase. During the stimulus updating condition, participants were asked to remove the position of the yellow dot from memory and add the position of the newly appeared red dot. The former modification was done to equate the visual input of the executive control phase across the trial types.
(v) Delay 2 phase. The 16 square grid was presented without any stimuli.
(vi) Response phase. A question mark (probe phase) was placed in one of the boxes and participants had a 2s window to select ‘yes’ if the position of the question mark matched the position of the to-be-remembered information or select ‘no’ if the position of the question mark did not match the position of the to be remembered information.
Participants completed 56 trials in each of the following conditions: maintenance-only, distractor filtering and stimulus updating ().
Figure 1. Graphical representation of the three trial types of interest (information maintenance, distractor filtering and stimulus updating) across the sequential trial events (preparatory, encoding, delay 1, executive control, delay 2 and response phases)
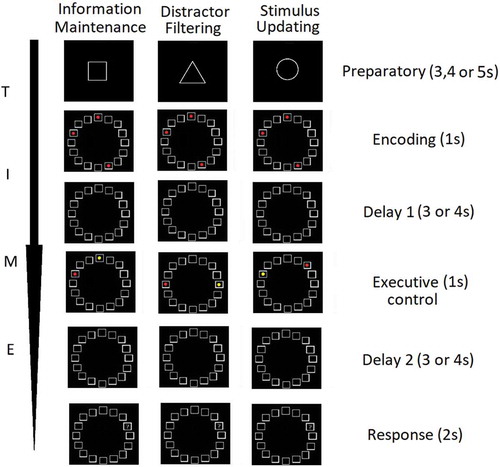
2.3.1. Task-switching
As mentioned above, the experimental task featured three goal-conditions (maintenance-only or information maintenance, distractor filtering and stimulus updating). In several occasions, (i) a given trial was of the same goal-condition as the preceding trial (e.g., maintenance-only in the 30th trial, and maintenance-only in the 29th trial). These cases were termed as ‘stay trials’. In other occasions (ii) a given trial was of a different goal-condition than the preceding trial (maintenance-only in the 30th trial, and stimulus updating in the 29th trial). These cases were termed as ‘switch trials’. Task-switching was calculated by subtracting the mean accuracy of the switch trials from the stay trials. We kept the proportion of each trial type (information maintenance, distractor filtering and stimulus updating) between the stay and switch trials identical (supplemental material 1). Moreover, there was no significant difference between the accuracy of the stay and switch trials (p = .6).
2.3.2. Distractor filtering
Distractor filtering was calculated as the difference between the mean accuracy of the distractor filtering trials (distractor filtering+information maintenance) vs. the mean accuracy of the maintenance-only trials (information maintenance).
2.3.3. Stimulus updating
Stimulus updating was calculated as the difference between the mean accuracy of the stimulus updating trials (stimulus updating+information maintenance) vs. the mean accuracy of the maintenance-only trials (information maintenance).
The abovementioned procedure to calculate task-switching, stimulus updating and distractor updating was established in previous studies (Cools et al., Citation2007; McNab & Klingberg, Citation2008).
2.4. Transcranial direct current stimulation
The brain stimulation only applied to the participants from the Oxford cohort. None of the participants from the Stockholm cohort took part in any stimulation session or had any electrodes attached. We assessed the causal contribution of the left globus pallidus and right dorsolateral prefrontal cortex (DLPFC) in cognitive control (Oxford location only). Apart from the sham condition, which was used for the structural analysis, participants in Oxford location performed the main task () in two tDCS sessions one that aimed to target the midline subcortical structures (‘SC stimulation’) and one to that aimed to target the right DLPFC (‘DLPFC stimulation’). The order of the three stimulation sessions (sham and two brain stimulation) was counterbalanced. During the two active tDCS sessions, 2 mA tDCS was delivered during the first half of each session (20 min) starting with 60s ramp up and ending with a 60s ramp down. The participants completed the task throughout the session namely both during the first half of the session where stimulation was on as well as during the second half of the session where stimulation was off. For analyses on the first and second half separately see Supplemental Material 11 and 12. In the montage that aimed to target the midline subcortical structures the anode electrode was placed on F9 according to the international 10–20 system and the cathode electrode was placed on the right shoulder.
Previous work, using a similar montage, suggested that it could potentially stimulate midline subcortical structures (Clark, Citation2013; Clark et al., Citation2012). In the montage that aimed to target the right DLPFC, we used a high-definition montage similar to the one used previously for the left side (Guo et al., Citation2018). One anode was placed above F4 and four return electrodes were placed over AF4, F6, F2, and FC4. In the sham condition, participants received a 60s ramp up and 60s ramp down current (up to 2 mA) while using either the SC or the DLPFC stimulation configuration. This sham choice was utilized to mimic the physical sensation of the active tDCS conditions so that the participants were blind concerning the three conditions. There was a requirement of at least a 48h period to reduce the likelihood of residual stimulation effects between sessions. The experiment was aimed to be double-blind as the order of the conditions was preprogrammed in advanced. Unfortunately, due to technical errors, the full participants’ blindness reports were not available, therefore we could not confirm that blindness was achieved.
2.5. Statistical analyses
As mentioned above, the present study primarily focused on the structural property of volume measured in mm3 in three subcortical and 12 frontoparietal regions yielding 30 measures across the two hemispheres. The main aim of the study was to examine the extent to which individual variation in regional volume tracks task-switching, stimulus updating and distractor filtering. To examine whether regional volumetric variation tracked specific types of cognitive control, multiple regression analyses were employed where the predicted variable was the performance in task-switching, stimulus updating or distractor filtering and the predictors were the regional volume, the total intracranial volume and the study location. The main effect of interest, and the one which is reported, is the unstandardized regression coefficient (i.e., beta-weight, denoted by b) of the regional volume. The unstandardized regression coefficient represents the amount of change in a dependent variable due to a change of 1 unit of the independent variable. For completeness, we provide additional results including reaction time, age and gender, and brain stimulation results in Supplemental Material.
We first examined the association between performance (i.e., mean accuracy where 1 = 100% correct) in task-switching, stimulus updating and distractor filtering and the volume of subcortical and frontoparietal regions using Equationeq1(1)
(1) .
To examine whether the results were driven by either of the two components of task-switching namely performance of stay or switch trials we run Equationeq2(2)
(2) and Equationeq3
(3)
(3) respectively (see below). The stay trial performance was calculated as the mean accuracy of the stay trials while the switch trial performance was calculated as the mean accuracy of the switch trials.
To examine the cognitive specificity of the results we run multiple regression controlling for the performance of the two other cognitive control types (i.e., stimulus updating and distractor filtering yielding Equationeq4(4)
(4) ).
To examine the regional specificity of the results we run multiple regression controlling for the control regions (e.g, right globus pallidus as a control region for assessing the effect of left globus pallidus yielding Equationeq5(5)
(5) ).
For the brain stimulation analysis, we conducted a paired-sample t-test comparing the mean accuracy of the task-switching in the sham condition vs. the SC tDCS condition. Following recent recommendations (Biel & Friedrich, Citation2018) on null results in brain stimulation studies, when applicable we additionally performed a Bayesian paired sample t-test analogous to the aforementioned one. For this Bayesian analyses, we report the Bayes Factor (BF10) of the experimental model vs. the null model, the one that merely features the intercept. The Bayes Factor is a number that indicated how many time more likely a given model is compared to another model, and in contrast to null results using frequentist statistics, the Bayes Factor can provide evidence in favor of the null model. As we did not have strong informative prior beliefs, we used the JASP default priors, where for the Bayesian t-test we assigned a prior form Cauchy distribution with a scale parameter (λ) = .707 and for the Bayesian multiple regression we assigned a prior from a beta distribution with parameter a = 1 and with parameter b = 1 (JASP, Citation2019).
To assess the relationship between the regional volume and the effect of tDCS on task-switching we performed linear regression with the regional volume as the main predictor, the total intracranial volume as a covariate and the task-switching of the SC tDCS vs. task-switching of the sham tDCS as the dependent variable (Equationequation 6(6)
(6) , see below).
Lastly, we run a similar model to the one in Equationequation 6(6)
(6) where we predicted the SC tDCS task-switching performance from the regional volume, total intracranial volume and sham tDCS task-switching performance (Equationeq 7
(7)
(7) , see below).
As our main interest in Equationequation 6(6)
(6) and Equationequation 7
(7)
(7) was the main effect of the regional volume, the Bayesian equivalents of Equationequation 6
(6)
(6) and Equationequation 7
(7)
(7) , was a model comparison approach that compared different models which included or omitted the regional volume. For this Bayesian analyses, we report the Bayes Factor (BF10) of every possible model vs. the null model, the one that merely features the intercept (see Supplemental Material 4 and 5).
3. Results
Of note, a Bonferroni correction was used at the P < .05 level of significance adjusted by the 90 tests, meaning that a test had to be P < .00055 to be considered significant.
3.1. Behavioral results
The descriptive statistics (mean and standard deviation) of all the conditions, sessions and study locations are displayed in . Please also see Supplemental Material 3 for the correlations between the accuracy in different trial types across the three sessions in the Oxford location.
Table 1. Mean (M) and standard deviation (SD) of the accuracy (1 = 100% accuracy) of all conditions and combination of conditions combined and separately in the two locations. ST = Stockholm, OX = Oxford (sham session), OX DLPFC = Oxford (DLPFC stimulation session), OX SC = Oxford (SC stimulation session)
3.2. Imaging results
We examined the association between the volume of 30 regions (15 per hemisphere) of interest with three cognitive control functions: task-switching, stimulus updating, and distractor filtering. The volume of the left globus pallidus was positively associated with the task-switching, even after correction for multiple comparisons [), b = .000229, t(34) = 4.033, p = .000294, and there was no interaction between site and the volume of the left globus pallidus (b = −.000011, t(33) = −.145, p = .89). For completeness, the full statistical details of the associations between other brain regions and types of cognitive control see Supplemental Material 2. The second strongest association of a regional volume to task-switching was with the right globus pallidus, but this effect was not significant after corrections for multiple comparisons (b = .000185, t(34) = 2.892, p = .0066). To discern whether the unique variance of the left and/or the right globus pallidus is associated to task-switching we utilized Equationeq5(5)
(5) where we predicted task-switching from the left globus pallidus (t(33) = 2.597, b = .000195, t(33) = 2.597, p = .014), the right globus pallidus (b = .000055, t(33) = .701, p = .49), the total intracranial volume and the study location, and the left but not the ride side of the globus pallidus was still a significant predictor.
Figure 2. Scatterplots depicting the associations between individual variation in the left globus pallidus volume (unstandardized residuals when correcting for total intracranial volume and study side is presented) and performance in a) task-switching; b) stimulus updating; c) distractor filtering. d) Coronal view of a segmented brain where the globus pallidus is labeled in blue color. The differences in the y-axis between panel B and panels A and C reflect differences in the performance range of the three functions studied, task-switching, stimulus updating and distractor filtering
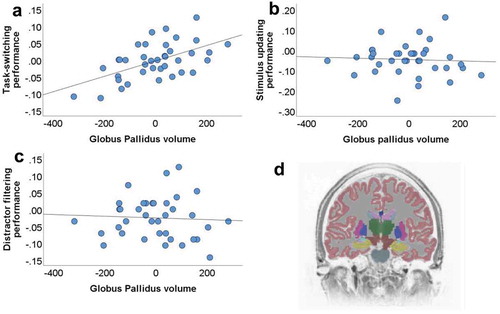
3.2.1. The effect of the left globus pallidus is not associated with either of the two determinants of task-switching
Next, we examined whether this main finding was driven by either of the two components of task-switching namely stay-trials performance (Equationeq2(2)
(2) ) or switch-trials performance (Equationeq3
(3)
(3) ). The volume of the left globus pallidus was neither associated with the accuracy of the stay trials (b = .000114, t(33) = 1.1, p = .28) nor switch trials (b = −.000113, t(33) = −1.23, p = .23) trials. Therefore, it was the difference between stay and switch trials that was predicted by the left globus pallidus volume.
3.2.2. The effect of the left globus pallidus is specific to task-switching
To examine the cognitive specificity of the main association we run multiple regression controlling for the performance of the two other cognitive control types (i.e., stimulus updating and distractor filtering, see Equationeq4(4)
(4) in Methods section). The main effect of the left globus pallidus in predicting task-switching performance was still significant (b = .000248, t(30) = 4.397, p = .000127). Taken together, our results suggest a specific association between the left globus pallidus and task-switching.
3.3. tDCS did not alter task-switching behavior
After establishing the relationship between the left globus pallidus volume and task-switching, we assessed whether tDCS in a montage that aimed to target the left midline subcortical structures will induce changes in task-switching behavior. This was done by performing a paired-sample t-test comparing the sham vs. SC tDCS condition on the task-switching. However, no significant effects were found (t(28) = −.71, p = .48, M = −.009, SD = .066, BF10 = .249). To relate the left globus pallidus volume with the null stimulation effect we performed Equationeq6(6)
(6) and Equationeq7
(7)
(7) . EquationEq6
(6)
(6) was used to assess the relationship between the regional volume and the effect of tDCS on task-switching in a linear regression with the regional volume as the main predictor with the total intracranial volume as a covariate and the task-switching of the SC tDCS vs. task-switching of the sham tDCS as the dependent variable. In Equationeq7
(7)
(7) , we predicted the SC tDCS task-switching performance from the regional volume, total intracranial volume and sham tDCS task-switching performance.
Neither Equationeq6(6)
(6) , (b = −.000173, t(17) = −1.419, p = .17) nor Equationeq7
(7)
(7) (b = −.000082, t(16) = −.757, p = .46) yielded a significant effect. Similar results were obtained using Bayesian analyses (Supplemental Material 4–5), where the models featuring the left globus pallidus were weaker than the null model. Similarly, DLPFC stimulation did not significantly impact accuracy or reaction time (Supplemental Material 7–8 and 12).
4. General discussion
In the present study, we examined the association between the volume of 30 regions of interest with three cognitive control functions: task-switching, stimulus updating, and distractor filtering. Three main findings emerged from this study: (i) the positive link between the left globus pallidus volume and task-switching, (ii) the cognitive specificity of this finding; only task-switching was significantly associated to the left globus pallidus volume, (iii) and null results showing that tDCS with a montage that aimed to target the midline subcortical structures did not impact task-switching performance.
The main finding of the current study was the positive association between the volume of the left globus pallidus and task-switching. This finding is in agreement with previous work involving basal ganglia regions and dopamine in task-switching. Previous lesion studies identified that individuals with striatal lesions exhibited impaired rule updating (Eslinger & Grattan, Citation1993). The involvement of basal ganglia in task-switching was also documented in pharmacological, electrophysiological and neuroimaging investigations in animals (Floresco & Magyar, Citation2006) and humans (Cools et al., Citation2007; Eslinger & Grattan, Citation1993; Lenartowicz et al., Citation2010; Van Holstein et al., Citation2011). However, the importance of basal ganglia was shown beyond task-switching as it was additionally demonstrated in stimulus updating (Murty et al., Citation2011) and distractor filtering (McNab & Klingberg, Citation2008). Taken together, previous work suggests that the involvement of basal ganglia may execute multifarious effects on different types of cognitive control. Our findings challenge the general role, at least, of the left globus pallidus, by showing that its volume was not specific to stimulus updating or distractor filtering or even to the performance of the stay or switch trials separately, but was associated with differences in the performance of the stay vs. switch trials. This is particularly important because the relative comparison of stay vs. switch trials isolates the component of preparing and executing task-switching while equating for the load (i.e., 3) and critically also for the same trial types (i.e., maintenance-only, distractor filtering and stimulus updating trials).
Despite the neurocognitive specificity, tDCS in a montage that was suggested to target the midline subcortical structures (Clark, Citation2013) did not exert task-switching alterations at the behavioral level. If any, our Bayesian analysis provided evidence in favor of the null model. This inability to provide a causal contribution of midline subcortical structures on task-switching can be explained by several factors. First, the montage to target the midline subcortical structures might inadvertently reach regions beyond the basal ganglia and thus compromising the net effect of the left globus pallidus stimulation on task-switching. Indeed, previous studies that used such montage produced a more general effect beyond the basal ganglia (Clark et al., Citation2012; Coffman, Trumbo, Clark et al., Citation2012; Coffman, Trumbo, Flores et al., Citation2012). Second, given the importance of the individuals’ baseline dopamine levels and the inverted U shaped relationship between dopamine and cognitive control (Cools & D’Esposito, Citation2011), it is possible that SC tDCS may be effective in altering task-switching only when the initial baseline dopamine levels of the participants are taken into account. If this assumption is correct, the initial baseline dopamine levels can be utilized in future studies to generate a personalized brain stimulation regime tailored to each individual. Third, even though we demonstrated that task-switching is associated with the volume of the left globus pallidus, it does not necessarily follow that basal ganglia are critical for task-switching. The relationship between structure and function is complex and brain structure does not fully determine the workings at the network level (Hagmann et al., Citation2008; C. Honey et al., Citation2009; C. J. Honey et al., Citation2007; Rubinov et al., Citation2009). Indeed, the association between task-switching and left globus pallidus volume could be mediated by other factors. Therefore, it is of interest for future research to identify potential mediators to increase our mechanistic understanding.
Compared to globus pallidus, our study showed that the contribution of the volume of frontal and parietal regions was not as strong in tracking task-switching. This may seem at odds with previous studies documenting the contribution of prefrontal cortex in task-switching behavior (Dove et al., Citation2000; Sohn et al., Citation2000). Even though the structural property of globus pallidus volume may be a stronger predictor over the structure of frontal and parietal regions, this does not contradict the neurofunctional involvement of these regions in task switching. Indeed, task-switching involves the updating of an abstract or high-level component rather than sensory representations of working memory stimuli, therefore the PFC may be the most suitable mediator candidate. This is because the prior work suggested that higher-order information, such as task rules, goals, or abstract representations are tracked in the PFC compared to stimulus-specific information represented by sensory-specific areas (Chen et al., Citation2012; Freedman et al., Citation2001, Citation2003; Lee et al., Citation2013; Meyers et al., Citation2008; Riggall & Postle, Citation2012; Sreenivasan et al., Citation2014). Taken this accumulative evidence together, we speculate that regions within the PFC are likely to participate in a network involving the volume of the left globus pallidus underpinning task-switching behavior. Anatomical pathways that link basal ganglia with the frontal cortex are the frontostriatal pathway (Darki & Klingberg, Citation2015). Future tractography investigations can examine whether the structural integrity of the aforementioned dopaminergic can determine task-switching behavior.
An open question concerns the exact mechanism underlying task-switching behavior which was underpinned by the volume of the left globus pallidus. For example, individual variation in task-switching might merely reflect errors on switch trials per se, or failure to adequately update the rule from the previous trial. If the latter mechanism is correct one would expect that responses would have been correct if the previous rule (from the preceding trial) had been active. However, because of the fixed contingencies between the instructions and stimuli positions between the different conditions of maintenance-only, distractor filtering and stimulus updating (see Methods section), the empirical interrogation between these two putative mechanisms is not possible in our study.
In sum, by investigating three key types of cognitive control with brain imaging, the present study allowed the identification of a specific basal ganglia marker, the volume of the left globus pallidus, for task-switching. Given the importance of task-switching on effective functioning, these findings pave the way for further studies into the causal role of the neural networks determining types of cognitive control which vary in the typical population and are impaired in different clinical populations.
Revision3_Supplemental_Material_CLEAN.docx
Download MS Word (50.3 KB)Acknowledgments
We are grateful to all the participants who took part in our study. The Wellcome Centre for Integrative Neuroimaging is supported by core funding from the Wellcome Trust (203139/Z/16/Z). This work was supported by the European Research Council (Learning & Achievement 338065).
Disclosure statement
RCK serves on the scientific advisory boards of Neuroelectrics Inc. and Tech InnoSphere Engineering Ltd.
Supplemental material
Supplemental data for this article can be accessed here
Additional information
Funding
References
- Alvarez, J. A., & Emory, E. (2006). Executive function and the frontal lobes: A meta-analytic review. Neuropsychology Review, 16(1), 17–42. https://doi.org/10.1007/s11065-006-9002-x
- Biel, A. L., & Friedrich, E. V. (2018). Why you should report bayes factors in your transcranial brain stimulation studies. Frontiers in Psychology, 9, 1125. https://doi.org/10.3389/fpsyg.2018.01125
- Bledowski, C., Rahm, B., & Rowe, J. B. (2009). What “works” in working memory? Separate systems for selection and updating of critical information. Journal of Neuroscience, 29(43), 13735–13741. https://doi.org/10.1523/JNEUROSCI.2547-09.2009
- Breukelaar, I. A., Antees, C., Grieve, S. M., Foster, S. L., Gomes, L., Williams, L. M., & Korgaonkar, M. S. (2017). Cognitive control network anatomy correlates with neurocognitive behavior: A longitudinal study. Human Brain Mapping, 38(2), 631–643. https://doi.org/10.1002/hbm.23401
- Chen, A. J.-W., Britton, M., Turner, G. R., Vytlacil, J., Thompson, T. W., & D’Esposito, M. (2012). Goal-directed attention alters the tuning of object-based representations in extrastriate cortex. Frontiers in Human Neuroscience, 6, 187. https://doi.org/10.3389/fnhum.2012.00187
- Clark, V. P. (2013, October 8). TDCS for cognitive enhancement [Video]. YouTube. /https://www.youtube.com/watch?v=dUMUIXNeBRQ
- Clark, V. P., Coffman, B. A., Mayer, A. R., Weisend, M. P., Lane, T. D., Calhoun, V. D., … Wassermann, E. M. (2012). TDCS guided using fMRI significantly accelerates learning to identify concealed objects. NeuroImage, 59(1), 117–128. https://doi.org/10.1016/j.neuroimage.2010.11.036
- Coffman, B. A., Trumbo, M., Flores, R., Garcia, C. M., Van Der Merwe, A., Wassermann, E. M., … Clark, V. P. (2012). Impact of tDCS on performance and learning of target detection: Interaction with stimulus characteristics and experimental design. Neuropsychologia, 50(7), 1594–1602. https://doi.org/10.1016/j.neuropsychologia.2012.03.012
- Coffman, B. A., Trumbo, M. C., & Clark, V. P. (2012). Enhancement of object detection with transcranial direct current stimulation is associated with increased attention. BMC Neuroscience, 13(1), 108. https://doi.org/10.1186/1471-2202-13-108
- Cools, R., & D’Esposito, M. (2011). Inverted-U–shaped dopamine actions on human working memory and cognitive control. Biological Psychiatry, 69(12), e113–e125. https://doi.org/10.1016/j.biopsych.2011.03.028
- Cools, R., Sheridan, M., Jacobs, E., & D’Esposito, M. (2007). Impulsive personality predicts dopamine-dependent changes in frontostriatal activity during component processes of working memory. Journal of Neuroscience, 27(20), 5506–5514. https://doi.org/10.1523/JNEUROSCI.0601-07.2007
- Darki, F., & Klingberg, T. (2015). The role of fronto-parietal and fronto-striatal networks in the development of working memory: A longitudinal study. Cerebral Cortex, 25(6), 1587–1595. https://doi.org/10.1093/cercor/bht352
- Dove, A., Pollmann, S., Schubert, T., Wiggins, C. J., & Von Cramon, D. Y. (2000). Prefrontal cortex activation in task switching: An event-related fMRI study. Cognitive Brain Research, 9(1), 103–109. https://doi.org/10.1016/S0926-6410(99)00029-4
- Eslinger, P. J., & Grattan, L. M. (1993). Frontal lobe and frontal-striatal substrates for different forms of human cognitive flexibility. Neuropsychologia, 31(1), 17–28. https://doi.org/10.1016/0028-3932(93)90077-D
- Floresco, S. B., & Magyar, O. (2006). Mesocortical dopamine modulation of executive functions: Beyond working memory. Psychopharmacology, 188(4), 567–585. https://doi.org/10.1007/s00213-006-0404-5
- Freedman, D. J., Riesenhuber, M., Poggio, T., & Miller, E. K. (2001). Categorical representation of visual stimuli in the primate prefrontal cortex. Science, 291(5502), 312–316. https://doi.org/10.1126/science.291.5502.312
- Freedman, D. J., Riesenhuber, M., Poggio, T., & Miller, E. K. (2003). A comparison of primate prefrontal and inferior temporal cortices during visual categorization. Journal of Neuroscience, 23(12), 5235–5246. https://doi.org/10.1523/JNEUROSCI.23-12-05235.2003
- Guo, H., Zhang, Z., Da, S., Sheng, X., & Zhang, X. (2018). High‐definition transcranial direct current stimulation (HD‐tDCS) of left dorsolateral prefrontal cortex affects performance in Balloon Analogue Risk Task (BART). Brain and Behavior, 8(2), e00884. https://doi.org/10.1002/brb3.884
- Hagmann, P., Cammoun, L., Gigandet, X., Meuli, R., Honey, C. J., Wedeen, V. J., Sporns, O., & Friston, K. J. (2008). Mapping the structural core of human cerebral cortex. PLoS Biology, 6(7), e159. https://doi.org/10.1371/journal.pbio.0060159
- Honey, C., Sporns, O., Cammoun, L., Gigandet, X., Thiran, J.-P., Meuli, R., & Hagmann, P. (2009). Predicting human resting-state functional connectivity from structural connectivity. Proceedings of the National Academy of Sciences, 106(6), 2035–2040. https://doi.org/10.1073/pnas.0811168106
- Honey, C. J., Kötter, R., Breakspear, M., & Sporns, O. (2007). Network structure of cerebral cortex shapes functional connectivity on multiple time scales. Proceedings of the National Academy of Sciences, 104(24), 10240–10245. https://doi.org/10.1073/pnas.0701519104
- JASP, T. (2019). JASP (Version 0.11. 1)[Computer software]. University of Amsterdam.
- Lee, S.-H., Kravitz, D. J., & Baker, C. I. (2013). Goal-dependent dissociation of visual and prefrontal cortices during working memory. Nature Neuroscience, 16(8), 997. https://doi.org/10.1038/nn.3452
- Lenartowicz, A., Escobedo-Quiroz, R., & Cohen, J. D. (2010). Updating of context in working memory: An event-related potential study. Cognitive, Affective & Behavioral Neuroscience, 10(2), 298–315. https://doi.org/10.3758/CABN.10.2.298
- Leung, H.-C., Oh, H., Ferri, J., & Yi, Y. (2007). Load response functions in the human spatial working memory circuit during location memory updating. NeuroImage, 35(1), 368–377. https://doi.org/10.1016/j.neuroimage.2006.12.012
- McNab, F., & Klingberg, T. (2008). Prefrontal cortex and basal ganglia control access to working memory. Nature Neuroscience, 11(1), 103. https://doi.org/10.1038/nn2024
- Meyers, E. M., Freedman, D. J., Kreiman, G., Miller, E. K., & Poggio, T. (2008). Dynamic population coding of category information in inferior temporal and prefrontal cortex. Journal of Neurophysiology, 100(3), 1407–1419. https://doi.org/10.1152/jn.90248.2008
- Montojo, C. A., & Courtney, S. M. (2008). Differential neural activation for updating rule versus stimulus information in working memory. Neuron, 59(1), 173–182. https://doi.org/10.1016/j.neuron.2008.05.012
- Murty, V. P., Sambataro, F., Radulescu, E., Altamura, M., Iudicello, J., Zoltick, B., … Mattay, V. S. (2011). Selective updating of working memory content modulates meso-cortico-striatal activity. NeuroImage, 57(3), 1264–1272. https://doi.org/10.1016/j.neuroimage.2011.05.006
- Riggall, A. C., & Postle, B. R. (2012). The relationship between working memory storage and elevated activity as measured with functional magnetic resonance imaging. Journal of Neuroscience, 32(38), 12990–12998. https://doi.org/10.1523/JNEUROSCI.1892-12.2012
- Roth, J. K., & Courtney, S. M. (2007). Neural system for updating object working memory from different sources: Sensory stimuli or long-term memory. NeuroImage, 38(3), 617–630. https://doi.org/10.1016/j.neuroimage.2007.06.037
- Roth, J. K., Serences, J. T., & Courtney, S. M. (2005). Neural system for controlling the contents of object working memory in humans. Cerebral Cortex, 16(11), 1595–1603. https://doi.org/10.1093/cercor/bhj096
- Rubinov, M., Sporns, O., van Leeuwen, C., & Breakspear, M. (2009). Symbiotic relationship between brain structure and dynamics. BMC Neuroscience, 10(1), 55. https://doi.org/10.1186/1471-2202-10-55
- Sohn, M.-H., Ursu, S., Anderson, J. R., Stenger, V. A., & Carter, C. S. (2000). The role of prefrontal cortex and posterior parietal cortex in task switching. Proceedings of the National Academy of Sciences, 97(24), 13448–13453. https://doi.org/10.1073/pnas.240460497
- Sörqvist, P., & Sætrevik, B. (2010). The neural basis of updating: Distinguishing substitution processes from other concurrent processes. Scandinavian Journal of Psychology, 51(5), 357–362. https://doi.org/10.1111/j.1467-9450.2010.00817.x
- Sreenivasan, K. K., Vytlacil, J., & D’Esposito, M. (2014). Distributed and dynamic storage of working memory stimulus information in extrastriate cortex. Journal of Cognitive Neuroscience, 26(5), 1141–1153. https://doi.org/10.1162/jocn_a_00556
- Takahama, S., Miyauchi, S., & Saiki, J. (2010). Neural basis for dynamic updating of object representation in visual working memory. NeuroImage, 49(4), 3394–3403. https://doi.org/10.1016/j.neuroimage.2009.11.029
- van Holstein, M., Aarts, E., van der Schaaf, M. E., Geurts, D. E., Verkes, R. J., Franke, B., … Cools, R. (2011). Human cognitive flexibility depends on dopamine D2 receptor signaling. Psychopharmacology, 218(3), 567–578. https://doi.org/10.1007/s00213-011-2340-2
- Vogel, E. K., McCollough, A. W., & Machizawa, M. G. (2005). Neural measures reveal individual differences in controlling access to working memory. Nature, 438(7067), 500. https://doi.org/10.1038/nature04171