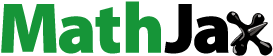
Abstract
Promising new pharmacological strategies for the enhancement of cognition target either nicotinic acetylcholine receptors (nAChR) or N-methyl-D-aspartate receptors (NMDAR). There is also an increasing interest in low-dose combination therapies co-targeting the above neurotransmitter systems to reach greater efficacy over the monotreatments and to reduce possible side effects of high-dose monotreatments. In the present study, we assessed modulatory effects of the α7 nAChR-selective agonist PHA-543613 (PHA), a novel α7 nAChR positive allosteric modulator compound (CompoundX) and the NMDAR antagonist memantine on the in vivo firing activity of CA1 pyramidal neurons in the rat hippocampus. Three different test conditions were applied: spontaneous firing activity, NMDA-evoked firing activity and ACh-evoked firing activity. Results showed that high but not low doses of memantine decreased NMDA-evoked firing activity, and low doses increased the spontaneous and ACh-evoked firing activity. Systemically applied PHA robustly potentiated ACh-evoked firing activity with having no effect on NMDA-evoked activity. In addition, CompoundX increased both NMDA- and ACh-evoked firing activity, having no effects on spontaneous firing of the neurons. A combination of low doses of memantine and PHA increased firing activity in all test conditions and similar effects were observed with memantine and CompoundX but without spontaneous firing activity increasing effects. Our present results demonstrate that α7 nAChR agents beneficially interact with Alzheimer’s disease medication memantine. Moreover, positive allosteric modulators potentiate memantine effects on the right time and the right place without affecting spontaneous firing activity. All these data confirm previous behavioral evidence for the viability of combination therapies for cognitive enhancement.
Introduction
The fundamental brain structures and pathways involved in cognition, especially those related to attention, learning and memory, have been widely explored in the last few decades mainly driven by the growing unmet medical need of new potent disease modulatory therapies and treatments for neurocognitive disorders (Farlow, Citation2009; Lockhart & Lestage, Citation2003; Sarter, Citation2006). Earlier data clearly proved that the cholinergic neurotransmission (via both muscarinic and nicotinic receptors) in the central nervous system (CNS) play an important and deterministic role in the formation of new memories and in the successful recall of earlier memory traces (Hasselmo, Citation2006). The glutamatergic system also plays a crucial role in the fundamental steps of learning and memory, and it is also critically affected in acute and chronic neurocognitive diseases (Barnes et al., Citation1996; Collingridge & Singer, Citation1990).
According to these facts, efficient targeting of the cholinergic and/or glutamatergic neurotransmission alone or simultaneously may prove as a relevant pharmacological strategy for cognitive enhancement. Acetylcholinesterase (AChE) inhibitors have been widely applied for decades for the treatment of mild to moderate cognitive impairment. However, other cholinergic agents including selective agonists, antagonists and positive allosteric modulators (PAM) of specific cholinergic receptor subtypes have also been intensively studied as potential drug-candidates for the treatment of neurocognitive disorders with little or no success to reach therapeutic use (Lockhart & Lestage, Citation2003). The only approved glutamatergic agent for the treatment of cognitive decline, so far, is memantine, an uncompetitive, voltage-dependent NMDA receptor antagonist with low-to-moderate affinity, strong voltage dependency, and rapid blocking/unblocking receptor kinetics (Danysz et al., Citation2000; Frankiewicz & Parsons, Citation1999; Parsons et al., Citation2007). In the past decades several other receptor targets of memantine in the CNS were also discovered, such as the serotonergic 5-HT3 receptor, the dopaminergic D2 receptor and the σ1 receptor (Rogawski & Wenk, Citation2006). Furthermore, memantine has been suggested to act as an antagonist on the α7 nicotinic acetylcholine receptor (nACh) (Aracava et al., Citation2005; Banerjee et al., Citation2005).
In a recent electrophysiological study, the direct in vivo effects of α7 nAChR specific agonist, PAM and antagonist compounds were investigated and compared on the spontaneous and NMDA-evoked firing rate of hippocampal CA1 neurons by means of local microiontophoretic administration of the agents into the vicinity of the neurons (Bali et al., Citation2019b). The results suggested that α7 nAChR specific PAM compounds (NS-1738 and PNU-120596) increase neuronal excitability more potently than the orthosteric agonist PHA-543613 (N-(3R)-1-azabicyclo[2.2.2]-Oct-3-yl-furo[2,3-c]pyridine-5-carboxamide). Surprisingly, PHA-543613 exerted both facilitatory and inhibitory effects on the firing activity with similar occurrence. The inhibitory effects of the α7 nAChR agonist could have probably been accounted for the desensitization of the receptor that may also explain some of the previously reported neuroprotective effects of α7 nAChR agonists (Mudo et al., Citation2007).
Based on the affinity of memantine to α7 nAChR and its similar effects to some α7 nAChR ligands, it was hypothesized that memantine and other α7 nAChR targeting compounds may have pharmacological interactions that may be beneficial in the treatment of cognitive impairments. This hypothesis was supported by recent behavioral studies that demonstrated the superior effectiveness of combination treatments with memantine and PHA-543613 over their monotreatments in experimental rodent models of cognitive decline (Bali et al., Citation2019a; Bruszt et al., Citation2021).
In the present electrophysiological study, our major aim was to investigate the cellular basis of the earlier observed synergistic cognitive enhancer action of memantine and α7 nAChR compounds by studying their effects on the firing activity of hippocampal neurons. Memantine and two different α7 nAChR compounds, the orthosteric agonist PHA-543613 and a novel drug candidate PAM compound, referred to as CompoundX (CPDX; a proprietary compound of Gedeon Richter Plc., Hungary; described in the patent WO 2020/012423 A1) were systemically applied both in monotreatments and in co-administration while the firing activity of hippocampal pyramidal neurons were recorded. Furthermore, we also analyzed the effect of the applied mono- and combination treatments on the responsiveness of pyramidal neurons to local iontophoretic stimulation using glutamatergic agonist NMDA and the natural cholinergic agonist ACh. Our experiments were designed to shed light on the neuroelectrophysiological background of the procognitive effects of memantine and α7 nAChR compounds alone or in combined applications.
Methods
Animals and Housing
Two hundred and seven male Wistar rats (289–599 g) were used in the experiments. Animals were purchased from a commercial vendor (Toxi-Coop, Budapest, Hungary). The rats were pair-housed and were kept in the conventional animal house of the Institute with a 12 h light/dark cycle (light ON from 7 a.m. to 7 p.m.). Tap water and standard laboratory rat food were available ad libitum.
Surgery
Surgical preparations were performed as described previously (Bali et al., Citation2019b, Citation2017; Nagy et al., Citation2021). Briefly, rats were initially anesthetized with a single intraperitoneal injection of chloral hydrate (400 mg/kg b.w., Molar Chemical, Halásztelek, Hungary), and stable anesthesia was further maintained by continuous intravenous administration of the anesthetic via a jugular vein cannula (100 mg/kg/h initially, later adjusted if needed). Tail-flick test was performed regularly to control the depth of anesthesia. Stereotaxic surgery was performed in a stereotaxic frame to allow the insertion of a multi-barrel carbon fiber microelectrode (Carbostar-3, -4 or -7S, Kation Scientific Ltd., Minneapolis, MN, USA) (Budai & Molnár, Citation2001) in the CA1 region of the dorsal hippocampus in conformity with the rat brain atlas by Paxinos and Watson (Citation2014): AP 3.3–5.5, ML 1.5–2.5 from bregma, and DV 1.8–3.8 from dura. At the end of the recording sessions, rats were sacrificed by intravenous administration of pentobarbital (Euthanimal 40%, Alfasan, Netherlands).
In Vivo Electrophysiological Recording and Microiontophoresis
The firing activity of CA1 neurons was recorded extracellularly through the central carbon fiber of the multi-barrel microelectrode. The electrophysiological signal was amplified and band-pass filtered between 400 and 3500 Hz by analog electrophysiological amplifiers (BioAmp, Supertech Ltd., Pécs, Hungary, and NeuroLog, Digitimer Ltd., Welwyn Garden City, UK). The signal was digitized at 25 kHz with an analog-to-digital converter unit (CED Power 1401) using the Spike2 software (both manufactured by Cambridge Electronic Design Ltd., Cambridge, UK). During recordings, microiontophoretic drug delivery was performed using the glass pipettes surrounding the central carbon-fiber recording channel of the microelectrode. One of the glass capillaries of the multi-barrel electrode was filled with 50 mM NMDA (Sigma-Aldrich, St. Louis, Missouri, USA) and the other was filled with 100 mM ACh (Sigma-Aldrich, St. Louis, Missouri, USA). N-methyl-D-aspartate was delivered using a negative ejection current (from −33 to −2 nA), while ACh was delivered using a positive ejection current (from +2 to +15 nA). For iontophoretic delivery, a constant current generator was used (Neurophore BH-2 System, Medical System Corp., Greenvale, NY). Between individual drug administrations, a low retention current with the opposite charge was applied onto the microiontophoresis pipettes to avoid leakage of the compounds.
Experimental Protocol and Test Conditions
The experimental protocol is depicted in . N-methyl-D-aspartate was periodically ejected for 5 s in every 2 min during the whole recording session. After stable firing responses of the neurons to NMDA were established, ACh was injected for 70 sec. Subsequent ACh ejections occurred in every 10 min, thus, four NMDA deliveries preceded the next ACh ejection. In the context of the iontophoretic delivery events, three different test conditions were defined: (1) spontaneous firing activity (average firing rate in a 60 s long interval without the iontophoretic delivery of any compounds); (2) NMDA-evoked firing responses (average of firing rates during during three NMDA-evoked excitation events before ACh ejection); (3) ACh-evoked firing responses (average firing rate in the first 60 s of the iontophoretic ACh delivery). After measuring a stable spontaneous, NMDA-evoked and ACh-evoked control firing activity (T0), different pharmacological treatments were administered systemically with subcutaneous (s.c.) injection. After the systemic treatment, the NMDA and ACh iontophoretic delivery were repeated periodically in the whole recording, which normally lasted 60 min.
Figure 1. (A) Representative electrophysiological recording of CA1 hippocampal pyramidal cells with the protocol of local microinotophoretic delivery. The horizontal bars on the firing rate histogram indicate iontophoretic deliveries of NMDA or ACh. (B) Schematic timeline of systemic drug administration in one recording session.
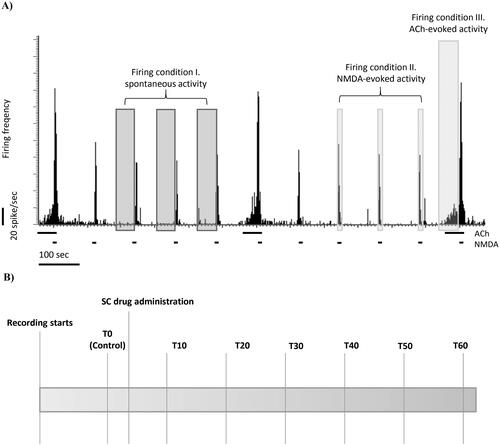
Systemic Pharmacological treatments
Systemic pharmacological treatments were summarized on . Memantine hydrochloride (Cat. No. 0773, Tocris) was dissolved in 0.9% saline and applied in four different doses: 0.01 mg/kg (MEM0.01), 0.1 mg/kg (MEM0.1), 1.0 mg/kg (MEM1.0), 3.0 mg/kg (MEM3.0). PHA-543613 hydrochloride (Cat. No. 3092, Tocris) was dissolved in 0.9% saline and applied in three different doses: 0.1 mg/kg (PHA0.1), 1.0 mg/kg (PHA1.0) and 3.0 mg/kg (PHA3.0). CPDX (a proprietary compound of Gedeon Richter Plc., Hungary, described in the patent WO 2020/012423 A1, synthesized by Gedeon Richter Plc.) was dissolved in 5% Tween 80 in phosphate-buffered saline (PBS) applied in three different doses: 0.3 mg/kg (CPDX0.3), 1.0 mg/kg (CPDX1.0) and 3.0 mg/kg (CPDX3.0). The combination treatments were categorized as low-dose and high-dose combinations. Low-dose combinations were: 0.01 mg/kg memantine + 0.1 mg/kg PHA-543613, and 0.01 mg/kg memantine + 0.3 mg/kg CPDX. High-dose combinations were: 3.0 mg/kg memantine + 3.0 mg/kg PHA-543513, and 3.0 mg/kg memantine + 3.0 mg/kg CPDX. Control experiments were performed by injecting the vehicle of the given test compounds (saline or 5% Tween 80).
Data Analysis
Analysis of electrophysiological data was performed offline. Data of 18 of 207 total recordings (18 animals) were excluded from further analysis because of various technical reasons (e.g., dripping micropipettes, non-stable anesthesia, electrical noise, etc.). The final corresponding sample sizes are described in Supplementary Figure 1. Extracellular action potentials (spikes) were detected from the continuous waveform data (raw data) with a template matching algorithm using the Spike2 software and applying a threshold of five times the root-mean-square of noise. Only those spike clusters were included in further analyses, which showed the typical characteristics of a complex-spiking cell (putative pyramidal cell) based on autocorrelation analysis (Csicsvari et al., Citation1998; Bali et al., Citation2014). Next, we normalized the firing rate frequency data to the corresponding pre-treatment control firing rate (T0 time point).
The effectiveness of the test compounds on the spontaneous, NMDA- and ACh-evoked firing rate were separately tested using linear mixed-effects models in IBM SPSS Statistics V25 (SPSS). In the linear mixed-effects model, neuron ID was used as a random intercept to consider the correlation between repeated measurements. Significance (p-values) corresponding to estimated marginal mean differences between post-injection time points (T10–T60) and the control measurement (T0) were corrected for multiple comparisons using Holm’s method (Holms, Citation1979). Furthermore, the efficacies of the test compounds were compared to the corresponding vehicle control. First, regression slopes of firing rate change over the time course of the recording session were fitted, then the slopes were compared using interaction terms of the following linear mixed-effects model:
where, FIRING is the resulted firing rate in Hz, TIME is a continuous predictor meaning the time elapsed from the injection, TREATMENT[1]-TREATMENT[n] are the test compounds in the given experiment, b0-b2n+1 are the parameters to be estimated, vi is the random intercept and e is the error term. Indices correspond to the i-th subject and j-th repeated measurement. After finding a significant interaction of TIME and TREATMENT main effects (ANOVA), significant interaction term parameters (i.e., bn+2 to b2n+1) indicated that the slope of firing rate as a function of time in the given treatment group significantly differs from the slope of firing rate after vehicle administration.
Results
Effects of Memantine on the Firing Activity of Hippocampal Neurons
Spontaneous Activity
We found that memantine dose dependently increased the spontaneous firing rate over time (MEM0.01: F(6, 71.1)=3.257, p = .007) as the observed firing rate was significantly higher compared to pre-injection control values (T0: 1.07 ± 0.40 Hz) already at 30 min after the administration of MEM0.01 (T30: 2.98 ± 0.73 Hz; normalized firing rate: 1.94 ± 0.73; p < .001) and further increased by the end of the session (T60: 3.40 ± 1.14 Hz; normalized firing rate: 2.35 ± 1.14; p < .001) (). In addition, the higher dose of memantine (MEM3.0) also increased the spontaneous firing activity at 40 min (T40: 2.36 ± 0.55 Hz; normalized firing rate: 1.15 ± 0.55; p = .032) compared to the T0 control value (T0: 1.33 ± 0.52 Hz; MEM3.0: F(6, 70.2)=4.872, p < .001). There was no difference between the effect sizes of the vehicle and the different test compound treatments on spontaneous firing activity (TIME × TREATMENT: (F(4, 325.4)=1.481, p = .208).
Figure 3. (A) Spontaneous firing activity of hippocampal CA1 pyramidal neurons before (T0) and after (T10–T60) the administration of 1 ml/kg saline (0.9% NaCl) and four doses of memantine (0.01 mg/kg; 0.1 mg/kg; 1 mg/kg; 3 mg/kg). (B) NMDA-evoked firing activity of hippocampal pyramidal cells before (T0) and after (T10–T60) the administration of 1 ml/kg saline (0.9% NaCl) and four doses of memantine (0.01 mg/kg; 0.1 mg/kg; 1 mg/kg; 3 mg/kg). (C) ACh-evoked firing activity of hippocampal pyramidal cells before (T0) and after (T10–T60) the administration of 1 ml/kg saline (0.9% NaCl) and four doses of memantine (0.01 mg/kg; 0.1 mg/kg; 1 mg/kg; 3 mg/kg). Neuronal activity was expressed as a normalized firing rate. Data were normalized using the mean pre-injection control firing rate values. Data points and error bars represent mean and SEM, respectively. Asterisks show significance levels of the Holm-corrected post-hoc comparisons to the corresponding T0 within a certain treatment group: *p < .05, **p < .01, ***p < .001. Hash symbol marks a significant difference between the regression slope of different test compounds: ##p < .01
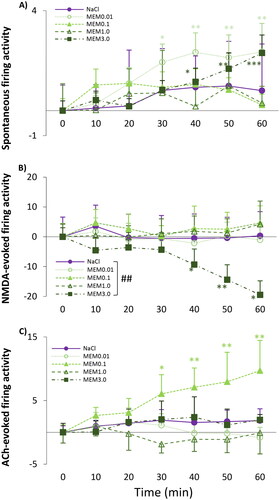
NMDA-Evoked Firing Activity
Only the highest memantine dose, MEM3.0 decreased the NMDA-induced firing rate (MEM3.0: F(6, 65.9)=2.762, p = .019) as the mean sensitivity of the neurons to NMDA was significantly lower first at 40 min after the administration of memantine (T40: 26.86 ± 5.24 Hz vs T0: 36.21 ± 3.02 Hz; normalized firing rate: −9.34 ± 5.24; p = .02) and further decreased in the next 20 min, until the end of the recording sessions (T50: 31.81 ± 6.7 Hz vs T0: 36.21 ± 3.02 Hz; normalized firing rate: −14.41 ± 5.02; p = .02; T60: 27.53 ± 7.19 Hz vs T0: 36.21 ± 3.02 Hz; normalized firing rate: −19.48 ± 4.7; p = .001) (). Comparing the regression slope of saline and MEM3.0, the significant interaction of TIME and TREATMENT confirmed the effectiveness of memantine compared to the corresponding vehicle treatment (TIME × TREATMENT: (F(4, 339.6)=4.255, p = .002; parameter estimates: TIME × [MEM_3.0]: −0.22 ± 0.08, df = 339.4, t = −2.793, p = .006).
ACh-Evoked Firing Activity
Memantine significantly increased ACh-evoked firing rate of the neurons at a dose of 0.1 mg/kg (MEM0.1: F(6, 575.5) = 2.948, p = .014) as there was a significant difference between ACh-evoked pre-treatment control firing rate (T0: 7.41 ± 1.16 Hz) and ACh-evoked firing rate after 30 min (T30: 13.47 ± 3.0 Hz, normalized firing rate: 6.06 ± 3.0; p = .023) (). However, there was no difference between the effect of the vehicle and memantine over time (TIME × TREATMENT: F(4, 290.3) = 4.788, p = 0.058).
Effects of PHA-543613 on the Firing Activity of Hippocampal Neurons
Spontaneous Activity
The α7 nAChR selective agonist PHA-543613 influenced spontaneous activity in every applied dose over the time (): PHA0.1, PHA1.0 and PHA3.0 all increased spontaneous firing activity compared to the pre-treatment control (PHA0.1: F(6, 59.944)=3.901, p = .002; PHA1.0: F(6, 53.726)=3.314, p = .008; PHA3.0: F(6, 61.186)=3.382, p = .006). The firing rate appeared to be significantly higher first at 30–40 min after administration of PHA-543613 and remained high until the end of the recordings. The slope of the effect of PHA1.0 was significantly higher compared to the corresponding saline treatment indicating a significant effect of PHA1.0 over its vehicle (TIME × TREATMENT: F(3, 254.0)=4.991, p = .002; parameter estimates: TIME × [PHA1.0]: 0.06 ± 0.02, df = 255.3, t = 3.702, p < .001).
Figure 4. (A) Spontaneous firing activity of hippocampal CA1 pyramidal neurons before (T0) and after (T10–T60) the administration of 1 ml/kg saline (0.9% NaCl) and three doses of PHA-543613 (0.1 mg/kg; 1 mg/kg; 3 mg/kg). (B) NMDA-evoked firing activity of hippocampal pyramidal cells before (T0) and after (T10–T60) the administration of 1 ml/kg saline (0.9% NaCl) and three doses of PHA-543613 (0.1 mg/kg; 1 mg/kg; 3 mg/kg). (C) ACh-evoked firing activity of hippocampal pyramidal cells before (T0) and after (T10–T60) the administration of 1 ml/kg saline (0.9% NaCl) and three doses of PHA-543613 (0.1 mg/kg; 1 mg/kg; 3 mg/kg). Firing activity was expressed as a normalized firing rate. Data were normalized using the mean pre-injection control firing rate values. Data points and error bars represent mean and SEM, respectively. Asterisks show significance levels of the Holm-corrected post-hoc comparisons to the corresponding T0 within a certain treatment group: *p < .05, **p < .01, ***p < .001. The hash symbol marks a significant difference between the regression slope of different test compounds: ###p < .001
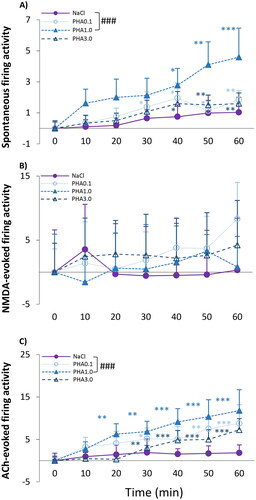
NMDA-Evoked Firing Activity
None of the applied doses of PHA-543613 significantly affected the excitatory firing responses evoked by locally applied NMDA, and there was no difference between the regression slopes of saline and specific PHA-543613 doses ().
ACh-Evoked Firing Activity
The α7 nAChR agonist PHA-543613 remarkably increased the ACh-evoked firing response rate over time in all applied doses (PHA0.1: F(6, 57.3) = 3.369, p = .007; PHA1.0: F(6, 63.0) = 5.567, p < .001; PHA3.0: F(6, 54.0)=11.716, p < .001) (). Furthermore, the slope of the effect of PHA1.0 was significantly higher than that of the control saline treatment indicating a significant effect of PHA1.0 over saline (TIME × TREATMENT: F(3, 249.7) = 5.406, p < .001; parameter estimates: TIME × [PHA_1.0]: 0.19 ± 0.05, df = 251.5, t = 1,071, p < .001).
Effects of CPDX on the Firing Activity of Hippocampal Neurons
Spontaneous Activity
The α7 nAChR positive allosteric modulator CPDX had only weak firing rate increasing effect over time on the spontaneous firing activity at 0.3 mg/kg dose (CPDX0.3: F(6, 29.9) = 2.577, p = .027) (). The firing rate was significantly higher than the pre-treatment control only at 40 min after administration (T40: 4.30 ± 0.98 Hz vs T0: 2.16 ± 0.72 Hz; normalized firing rate: T40: 1.00 ± 0.44; p = .04). Other doses of CPDX did not affect spontaneous firing activity.
Figure 5. (A) Spontaneous firing activity of hippocampal CA1 pyramidal neurons before (T0) and after (T10–T60) the administration of Tween 80 and three doses of CPDX (0.1 mg/kg; 1 mg/kg; 3 mg/kg). (B) NMDA-evoked firing activity of hippocampal pyramidal cells before (T0) and after (T10–T60) the administration of Tween 80 and three doses of CPDX (0.1 mg/kg; 1 mg/kg; 3 mg/kg). (C) ACh-evoked firing activity of hippocampal pyramidal cells before (T0) and after (T10–T60) the administration of Tween 80 and three doses of CPDX (0.1 mg/kg; 1 mg/kg; 3 mg/kg). Firing activity was expressed as normalized firing rate. Data were normalized using the mean pre-injection control firing rate values. Data points and error bars represent mean and SEM, respectively. Asterisks show significance levels of the Holm-corrected post-hoc comparisons to the corresponding T0 within a certain treatment group: *p < .05, **p < .01. Hash symbol marks a significant difference between the regression slope of different test compounds: #p < .05, ##p < .01, ###p < .001.
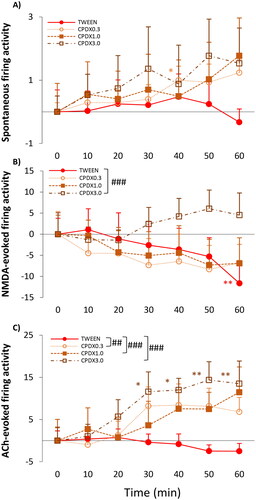
NMDA-Evoked Firing Activity
Although NMDA-evoked firing rate did not increase over time as a result of CPDX3.0 treatment, we found a significant difference between the regression slopes of CPDX3.0 and its vehicle, Tween 80 (TIME × TREATMENT: F(3, 268.2)=4.877, p = .003; parameter estimates: TIME × [CPDX_3.0]: 0.30 ± 0.08, df = 268.3, t = 3.622, p < .001) (). Interestingly, Tween 80 decreased NMDA-evoked firing acitivity (TWEEN: F(6, 76.0)=2.889, p = .014) at 60 min after its administration (T60: 26.44 ± 3.78 Hz vs T0: 38.08 ± 4.63 Hz; normalized firing rate: −11.64 ± 4.63; p = .003).
ACh-Evoked Firing Activity
Also, CPDX had significant effect on the ACh sensitivity of the neurons. CPDX3.0 increased ACh-evoked firing frequency over time (CPDX3.0: F(6, 49.2) = 3.114, p = .011) (). The firing rate was significantly higher than the pre-treatment control first at 30 min after administration (T30: 24.25 ± 4.70 Hz vs T0: 12.66 ± 3.19 Hz; normalized firing rate: 11.60 ± 4.70; p = .021) and remained high until the end of the recording sessions (T60: 31.48.11 ± 7.11 Hz; normalized firing rate: 18.82 ± 7.11; p = .005). Comparisons of regression slopes revealed that all of the CPDX doses robustly increased ACh-evoked firing responses compared to the vehicle group treated with Tween 80 (TIME × TREATMENT: F(3, 268.4)=6.728, p < .001; parameter estimates: TIME × [CPDX_0.3]: 0.22 ± 0.07, df= 265.2, t = 2.959, p = .003; TIME × [CPDX_1.0]: 0.24 ± 0.08, df = 265.9, t = 3.184, p = .002; TIME × [CPDX_3.0]: 0.34 ± 0.08, df = 272.9, t = 4.092, p < .001).
Effects of Combined Treatments on the Firing Activity of Hippocampal Neurons
Spontaneous Activity
Low-dose combination treatment with memantine and PHA-543613 (MEM0.01 + PHA0.1) significantly increased spontaneous firing rate over time (MEM0.01+ PHA0.1: F(6, 66.7) = 6.352, p < .001) (). Compared to the pre-treatment control, the firing rate was higher at 30 min after administration of the combination (T30: 3.81 ± 1.25 Hz vs T0: 1.24 ± 0.22 Hz; normalized firing rate: 2.57 ± 1.25; p = .005) and this effect lasted until the end of the recording sessions (T50: 4.35 ± 1.20 Hz vs T0: 1.24 ± 0.22 Hz, normalized firing rate: 3.10 ± 1.20; p < .001; and T60: 5.25 ± 1.33 Hz vs T0: 1.24 ± 0.22 Hz, normalized firing rate: 4.01 ± 1.33; p < .001). Moreover, the slopes of the effect of low-dose memantine and PHA-543613 combination was significantly higher than that of saline, indicating a significant effect of this combined treatment over its vehicle (TIME × TREATMENT: F(2, 194.8)=16.429, p < .001; parameter estimates: TIME × [MEM0.01 + PHA0.1]: 0.05 ± 0.01, df = 195.6, t = 3.848, p < .001). High-dose combination of memantine and PHA-543613 (Mem3 + PHA3) did not affect the spontaneous firing rate.
Figure 6. (A,B) Spontaneous firing activity of hippocampal CA1 pyramidal cells before (T0) and after (T10-T60) the administration of vehicles and different combined treatments (MEM0.01 + PHA0.1, MEM3.0 + PHA3.0, MEM0.01 + CPDX0.3, MEM3.0 + CPDX3.0). (C,D) NMDA-evoked firing activity of hippocampal pyramidal cells before (T0) and after (T10–T60) the administration of vehicles and different combined treatments. (E,F) ACh-evoked firing activity of hippocampal pyramidal cells before (T0) and after (T10–T60) the administration of vehicles and different combined treatments. Firing activity was expressed as a normalized firing rate using the mean pre-injection control firing rate values. Data points and error bars represent mean and SEM, respectively. Asterisks show significance levels of the Holm-corrected post-hoc comparisons to the corresponding T0 within a certain treatment group: *p < .05, **p < .01, ***p < .001. Hash symbol marks a significant difference between the regression slope of different test compounds: #p < .05, ###p < .001.
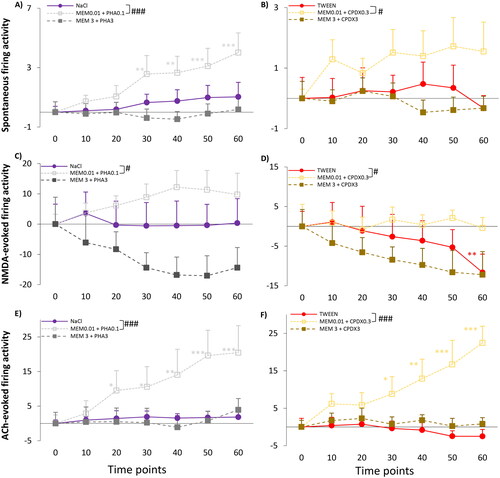
Similarly, only the low-dose combination of memantine and CPDX (Mem0.01 + CPDX0.3) was found effective on spontaneous firing activity. There was a difference in the slope of the effect between MEM0.01 + CPDX0.3 combination treatment and vehicle-treatment (TIME × TREATMENT F(2, 194.1)=18.958, p < .001; parameter estimates: TIME × [MEM0.01 + CPDX0.3]: 0.06 ± 0.01, df = 194.5, t = 4.333, p < .001) (). However, the spontaneous firing rate was not significantly higher after MEM0.01 + CPDX0.3 in any of the measurement points compared to the pre-treatment control (MEM0.01 + CPDX0.3: F(6, 54.0)=1.595, p = .166).
NMDA-Evoked Firing Activity
Also, NMDA-evoked firing activity was only modulated by the low-dose combination of memantine and PHA-543613 or CPDX. Comparison of regression slopes indicated a significant difference between MEM0.01 + PHA0.1 treatment and vehicle treatment (TIME × TREATMENT: F(2, 200.2) = 3.851, p = .023; parameter estimates: TIME × [MEM0.01 + PHA0.1]: 0.02 ± 0.01, df = 199.3, t = 2.328, p = .021) (). Also, a difference of regression slopes between the effect of MEM0.01 + CPDX0.3 and Tween 80 (vehicle) was found (TIME × TREATMENT: F(2, 189.9) = 3.049, p = .05; parameter estimates: TIME × [MEM0.01 + CPDX0.3]: 0.19 ± 0.08, df = 188.8, t = 2.411, p = .017) (). However, neither MEM0.01 + PHA0.1 nor MEM0.01 + CPDX0.3 treatments resulted in the increase of NMDA-evoked firing rate over time compared to the pre-treatment control condition (MEM0.01 + PHA0.1: F(6, 61.4) = 1.567, p = .172; MEM0.01 + CPDX0.3: F(6, 54.0) = 0.397, p = .878).
ACh-Evoked Firing Activity
Combined MEM0.01 + PHA0.1 treatment increased (MEM0.01 + PHA0.1: F(6, 60.4) = 6.349, p < .001) ACh-evoked firing activity at 20 min after administration (T20: 22.63 ± 5.73 Hz vs T0: 13.11 ± 2.44 Hz; normalized firing rate: 9.52 ± 5.73; p = .044) compared to the pre-treatment control, and this effect lasted until the end of the recording sessions (T60: 39.16 ± 6.45 Hz; normalized firing rate: 20.46 ± 7.81; p < .001) (). Additionally, the regression slope after MEM0.01 + PHA0.1 treatment was also different from the slope of the firing rate changes after vehicle (saline) treatment. (TIME × TREATMENT: F(2, 184.1)=18.625, p < .001; parameter estimates: TIME × [MEM0.01 + PHA0.1]: 0.38 ± 0.07, df = 184.8, t = 5.223, p < .001). Similarly, MEM0.01 + CPDX0.3 treatment increased the responsiveness of the neurons to direct delivery of ACh (MEM0.01 + CPDX0.3: F(6, 43.4)=5.666, p < .001) at 30 min after administration (T30: 14.63 ± 4.31 Hz vs T0: 6.52 ± 1.69 Hz; normalized firing rate: 8.80 ± 4.67; p = .043) compared to the pre-treatment control condition. Neuronal responsiveness kept further increasing until the end of the 60 minutes long recording (T40: 16.47 ± 4.70 Hz vs T0: 6.52 ± 1.69 Hz; normalized firing rate: 12.90 ± 5.21; p = .012; T50: 18.10 ± 5.24 Hz vs T0: 6.52 ± 1.69 Hz; normalized firing rate: 16.69 ± 6.45; p = .005; T60: 21.92 ± 4.90 Hz vs T0: 6.52 ± 1.69 Hz; normalized firing rate: 22.45 ± 4.46; p < .001) (). Furthermore, the slope of the effect of MEM0.01 + CPDX0.3 treatment was significantly higher than that of the vehicle treatment (TIME × TREATMENT: F(2, 185.7)=29.761, p < 0.001; parameter estimates: TIME × [MEM0.01 +PHA0.1]: 0.38 ± 0.05, df = 184.6, t = 7.531, p < .001).
Short Summary of Treatment Effects
summarizes the overall effects of the test compounds. Memantine increased the spontaneous firing activity of the neurons both in low and high doses, while a low dose of memantine also increased the ACh-evoked firing activity. On the other hand, a high dose of memantine decreased NMDA-evoked firing activity. Alpha7 nAChR agonist PHA-543613 increased both spontaneous and ACh-evoked firing activity, while it did not modulate the NMDA-evoked firing activity. Alpha7 nAChR PAM CPDX increased the firing rate in all firing conditions: the low dose of CPDX increased the spontaneous firing activity, the high dose increased NMDA-evoked firing activity, and all doses increased the ACh-evoked firing activity. Surprisingly, the vehicle of CPDX, Tween 80 decreased the NMDA-evoked firing activity of the neurons. Low dose combinations of memantine and PHA-543613 or CPDX increased the firing rate in all firing conditions, while high-dose combinations of the test materials did not modulate the activity of the neurons in any of the firing conditions.
Figure 7. Summary of the different drug treatment effects on in vivo firing activity of pyramidal neurons in the rat hippocampus. (A) Significant effects (p < .05) at any post-injection time points (T10–T60) compared to the T0 control time point within the given treatment. (B) Significant differences (p < .05) between the regression slopes of the effect of the given treatment and the corresponding vehicle control.
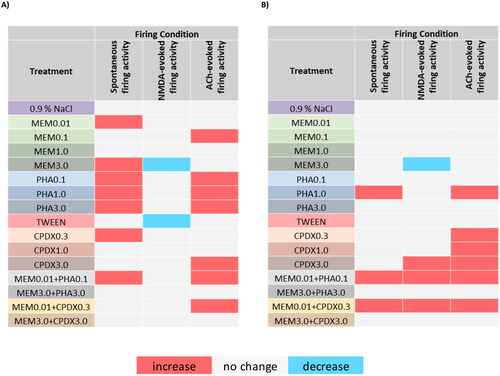
Discussion
In the present study, we investigated how behaviorally relevant doses of different cognitive enhancer compounds modulate the firing activity of CA1 hippocampal neurons in vivo. Our experimental design enabled to measure the effects of the systemically administered test compounds on both spontaneous, and NMDA or ACh-evoked firing activity of the neurons.
First, we showed that memantine modulated the firing rate of hippocampal CA1 neurons in every test condition (spontaneous, NMDA-evoked, and ACh-evoked firing). However, only the highest memantine dose (3.0 mg/kg) decreased the NMDA-evoked firing activity. This finding is in accordance with the known NMDA receptor blocking effect of memantine that was earlier demonstrated elsewhere under in vivo conditions using high doses of memantine (Szegedi et al., Citation2010). Low memantine doses (0.01–1.0 mg/kg) had no effect on NMDA-evoked firing activity. However, recent behavioral results showed a marked cognitive enhancer effect of memantine in rats in a dose range of 0.1–1.0 mg/kg (Bali et al., Citation2019a; Bruszt et al., Citation2021). Our present results together with the previous findings suggest that the cognitive enhancer effect of memantine does not necessarily require the direct antagonism of NMDARs. According to previous literature data, the lack of effects of low memantine doses on NMDA-evoked firing is not surprising, since memantine is a weak antagonist of the NMDARs and in vitro studies showed its higher affinity to other targets, including α7 nAChRs (Aracava et al., Citation2005; Rogawski & Wenk, Citation2006). In addition, the facilitatory effects of low-dose memantine on ACh-evoked firing was very similar to the effects of α7 nAChR ligands.
Interestingly, low-dose (0.1 mg/kg) memantine treatment effectively increased the responsiveness of neurons to locally delivered ACh that may at least partly explain the previously observed cognitive enhancer effects of memantine in low dose ranges in memory tasks in rats, where a low dose of memantine and PHA-543613 successfully reversed scopolamine-induced memory impairment (Bali et al., Citation2019a; Bruszt et al., Citation2021).
In the present experiments, systemic injection of PHA-543613 exerted robust facilitating effects on the spontaneous firing activity of neurons and on their responsiveness to ACh, but it did not influence the NMDA-evoked firing activity. The effect of PHA-543613 on spontaneous and on ACh-evoked firing activity showed an inverted U-shaped dose-effect relationship, which is also typical for α7 nAChR compounds in behavioral tests (Pismataro et al., Citation2021; Weed et al., Citation2017; Yang et al., Citation2013). Similar results were reported in a recent electrophysiological paper, where locally administered PHA-543613 had significant facilitating effects on the spontaneous firing activity of approximately half of the tested neurons, while the α7 nAChR agonist did not modulate their responses to NMDA (Bali et al., Citation2019b). Earlier behavioral studies also showed that the same dose of PHA-543613 (1.0 mg/kg) which increased spontaneous and ACh-evoked firing activity of hippocampal neurons in our present experiments was also behaviorally effective against MK-801 induced memory impairment in rodents (Bali et al., Citation2015; Ning et al., Citation2017).
It is generally accepted that direct action of nAChR agonist agents, especially during chronic administration, may generate unfavorable side-effects that are related to receptor desensitization or receptor upregulation (Papke & Horenstein, Citation2021). Therefore, PAM compounds of α7 nAChRs may have great therapeutic potential in future pharmacological treatment strategies (Wang et al., Citation2020). In the present study, we tested the effects of the α7 nAChR PAM CPDX and observed only a slight facilitating effect on the spontaneous activity of hippocampal neurons, while CPDX robustly increased the responsiveness of neurons to locally delivered ACh. Thus, the present results indicate the putative therapeutically beneficial property of CPDX, as CPDX only modulated neuronal activity in the presence of the endogenous ligand (ACh), which may be further utilized to minimize off-target therapeutic effects of cholinomimetic agents. Such modulatory mechanism may also facilitate cholinergic signaling while preserving the physiological activation pattern made by α7 nAChRs following spatially and temporally regulated endogenous ACh release (Lewis et al., Citation2017). Our present results are also in correspondence with earlier findings that showed a robust facilitatory effect of the α7 nAChR-specific PAM NS-1738 in the presence of the endogenous agonist ACh in vitro (Timmermann et al., Citation2007), and with earlier findings that demonstrated the neuronal firing rate increasing effect of locally applied NS-1738 in vivo (Bali et al., Citation2019b). It is also important to mention that systemic administration of the PAM agent CPDX, but not the orthosteric agonist agent PHA-543613, was shown to moderately increase the NMDA-evoked firing activity of hippocampal neurons. The present results are also in line with previous findings where the test compounds were administered via direct iontophoresis in the vicinity of the neurons and where such agents similarly increased NMDA-evoked firing activity of the neurons in the hippocampus (Bali et al., Citation2019b). Interestingly, in the present study, TWEEN 80 decreased the NMDA-evoked firing activity of hippocampal pyramidal neurons at 60 min after administration. Indeed, TWEEN 80 has previously been shown to inhibit α7 nACh receptors (Oz et al., Citation2004), and presumably affected the NMDA sensitivity of the neurons via an indirect mechanism (for instance via the modulation of interneuron activity or via collateral inhibition). In summary, we can state that the α7 nAChR agonist PHA-543613 has a strong cholinergic facilitatory effect and the α7 nAChR PAM CPDX enhanced the neuronal activity in all firing conditions independently of the vehicles used.
Memantine is also known to produce side-effects during long-term (chronic) treatment, furthermore, as administering memantine for longer periods of time may lead to stimulation of compensatory mechanisms which could reduce its effectiveness. These long-term consequences can be attenuated by decreasing the applied memantine dose, but this may also limit the therapeutic effects of the drug (Schmitt et al., Citation2004). One possible strategy for preserving the beneficial therapeutic effects of memantine while decreasing the applied dose would be the introduction of combination treatments which may offer a higher efficacy by multi-targeting and possible synergistic effects without inducing unfavorable side effects and long-term tolerance (Woodruff-Pak et al., Citation2007). Combining drugs approved for the treatment of AD, for example the NMDA antagonist memantine with various cholinesterase inhibitor agents (e.g., donepezil, rivastigmine, etc.) has been a possible option for combination therapies in neurocognitive decline, however, preclinical studies did not confirm their beneficial pharmacological interactions so far (Deardorff & Grossberg, Citation2016; Molino et al., Citation2013; Wenk et al., Citation2000; Woodruff-Pak et al., Citation2007). In addition, recently, there have been promising preclinical experimental results published investigating behavioral effects of combinations of α7 nAChR compounds and memantine in animal models. These studies showed superior efficacy of the combination treatments over monotreatments for the enhancement of cognitive performance (Bali et al., Citation2019a; Bruszt et al., Citation2021; Busquet et al., Citation2012; Nikiforuk et al., Citation2016; Potasiewicz et al., Citation2020). In the present study, the combination of low doses of α7 nAChR agonist PHA-543613 and PAM CPDX with memantine (MEM0.01 with either PHA0.1 or PAM0.3) showed clear facilitating effects in every test condition (spontaneous, NMDA-evoked, or ACh-evoked firing) indicating a clear beneficial interaction between the NMDA antagonist memantine and the α7 nAChR agonist and PAM compounds.
It also has to be noted, that the combination of PHA-543613 and memantine exerted more remarkable effects on spontaneous firing activity than the combination of CPDX and memantine. This is in line with their presently reported monotreatment effects and their pharmacodynamic properties, namely that for exerting their facilitating effects, PAM compounds need the presence of an orthosteric agonist agent of the same receptor (Timmermann et al., Citation2007). In addition, a combination of high doses of memantine and α7 nAChR compounds counteracted the inhibitory effects of memantine on NMDA-evoked firing activity. Although the attenuation effects of high doses of cholinomimetics may decrease the efficacy of the combination treatments, such interaction may be beneficial by blocking the over-enhanced antagonist effects on NMDARs and enabling physiological long-term potentiation and memory formation (Grüter et al., Citation2015; Newcomer & Krystal, Citation2001). Nevertheless, the present findings comparing low-dose and high-dose cognitive enhancer treatment combinations indicate that the selection of the best doses for combination therapies requires thorough screening of the underlying cellular and behavioral interactions and their consequences on the effectiveness of the given therapeutic regimen. To sum up the findings of combined treatments, low dose combinations of memantine and α7 nAChR compounds (agonist or PAM) showed facilitatory effects on the neuronal activity in the hippocampus which is in line with the previously reported beneficial interactions of these compounds on cognitive performance.
To date, the precise mechanism of the cellular-level interaction between combined drug treatments using memantine and α7 nAChR agents has not been fully explored. One possible mechanism may be that memantine also acts on the α7 nAChRs. As it was demonstrated in this study, memantine interacted with the spontaneous and the ACh-evoked firing rate in almost all applied doses, while only the highest applied dose decreased the NMDA-evoked firing activity. This is in line with previous findings showing that memantine more potently acts on α7 nAChRs compared to NMDARs (Aracava et al., Citation2005).
The present findings further support the evidence of the long suggested beneficial interactions between memantine and α7 nAChR compounds and substantiate the prominent role of α7 nAChRs in the procognitive effects of memantine. Results also facilitate the understanding of the cellular mechanisms underlying the behavioral manifestations of cholinergic and glutamatergic interplay in the mammalian brain.
Ethics Statement
All procedures were conducted at the electrophysiological laboratory of the Institute of Biology, University of Pécs in full compliance with the European Union legislation Directive 2010/63/EU and national legal requirements of the decree no. 40/2013. (II.14.) ‘On animal experiments’ issued by the Ministry of Agriculture of the Government of Hungary. The study was approved by the Local and National Ethical Committees on Animal Research and the Department of Animal Health and Food Control of the Country Government of the Ministry of Agriculture (License no.: BA02/2000-80/2017). All possible measures were taken to minimize pain and discomfort of the animals involved.
Authors’ Contributions
L.V.N.: Conceptualization, Methodology, Investigation, Visualization, Formal analysis, Data curation, Writing
Z.K.B.: Conceptualization, Methodology, Formal analysis, Writing
I.L.: Conceptualization, Writing
Z.N.: Conceptualization, Writing
B.L.: Conceptualization, Writing
I.H.: Conceptualization, Methodology, Supervision, Writing, Resources, Funding acquisition
Disclosures Statement
IL, ZN, and BL are employees of Gedeon Richter Plc. This does not alter the authors’ adherence to journal policies on sharing data and materials. The remaining authors are academic researchers and declare that the research was conducted in the absence of any commercial, non-financial, or financial relationships that could be construed as a potential conflict of interest.
Supplemental Material
Download MS Word (60.9 KB)Acknowledgements
Proprietary agent CompoundX, a potent positive allosteric modulator (PAM) of α7 nicotinic acetylcholine receptors was provided as a courtesy of Gedeon Richter Plc., Hungary (Patent No. WO 2020/012423 A1). The authors would like to thank Mrs. Jánosné Antal for her assistance in animal care. Microelectrodes were provided by courtesy of Dr. Dénes Budai (Kation Scientific Ltd., Minneapolis, MN).
Data Availability Statement
The datasets generated during and/or analyzed during the current study are available from the corresponding author on reasonable request.
Additional information
Funding
References
- Aracava, Y., Pereira, E. F. R., Maelicke, A., & Albuquerque, E. X. (2005). Memantine blocks α7* nicotinic acetylcholine receptors more potently than N-methyl-D-aspartate receptors in rat hippocampal neurons. Journal of Pharmacology and Experimental Therapeutics, 312(3), 1195–1205. https://doi.org/10.1124/jpet.104.077172
- Bali, Z. K.,Budai, D., &Hernádi, I. (2014). Separation of electrophysiologically distinct neuronal populations in the rat hippocampus for neuropharmacological testing under in vivo conditions. Acta Biologica Hungarica, 65(3), 241–251. 10.1556/ABiol.65.2014.3.125194728
- Bali, Z. K., Bruszt, N., Tadepalli, S. A., Csurgyók, R., Nagy, L. V., Tompa, M., & Hernádi, I. (2019a). Cognitive enhancer effects of low memantine doses are facilitated by an alpha7 nicotinic acetylcholine receptor agonist in scopolamine-induced amnesia in rats. Frontiers in Pharmacology, 10, 73. https://doi.org/10.3389/fphar.2019.00073
- Bali, Z. K., Inkeller, J., Csurgyók, R., Bruszt, N., Horváth, H., & Hernádi, I. (2015). Differential effects of α7 nicotinic receptor agonist PHA-543613 on spatial memory performance of rats in two distinct pharmacological dementia models. Behavioural Brain Research, 278, 404–410. https://doi.org/10.1016/j.bbr.2014.10.030
- Bali, Z. K., Nagy, L. V., Budai, D., & Hernádi, I. (2019b). Facilitation and inhibition of firing activity and N-methyl-D-aspartate-evoked responses of CA1 hippocampal pyramidal cells by alpha7 nicotinic acetylcholine receptor selective compounds in vivo. Scientific Reports, 9(1), 9324. https://doi.org/10.1038/s41598-019-45796-7
- Bali, Z. K., Nagy, L. V., & Hernádi, I. (2017). Alpha7 nicotinic acetylcholine receptors play a predominant role in the cholinergic potentiation of N-methyl-D-aspartate evoked firing responses of hippocampal CA1 pyramidal cells. Frontiers in Cellular Neuroscience, 11, 271. https://doi.org/10.3389/fncel.2017.00271
- Banerjee, P., Samoriski, G., Gupta, S., Aracava, Y., Pereira, E. F. R., Maelicke, A., & Albuquerque, E. X. (2005). Comments on “memantine blocks α7* nicotinic acetylcholine receptors more potently than N-methyl-D-aspartate receptors in rat hippocampal neurons” (multiple letters). Journal of Pharmacology and Experimental Therapeutics, 313(2), 928–929. https://doi.org/10.1124/jpet.104.081976
- Barnes, C. A., Danysz, W., & Parsons, C. G. (1996). Effects of the uncompetitive NMDA receptor antagonist memantine on hippocampal long-term potentiation, short-term exploratory modulation and spatial memory in awake, freely moving rats. European Journal of Neuroscience, 8(3), 565–571. https://doi.org/10.1111/j.1460-9568.1996.tb01241.x
- Bruszt, N., Bali, Z. K., Tadepalli, S. A., Nagy, L. V., & Hernádi, I. (2021). Potentiation of cognitive enhancer effects of Alzheimer’s disease medication memantine by alpha7 nicotinic acetylcholine receptor agonist PHA-543613 in the Morris water maze task. Psychopharmacology, 238(11), 3273–3281. https://doi.org/10.1007/s00213-021-05942-4
- Budai, D., & Molnár, Z. (2001). Novel carbon fiber microeletrodes for extracellular electrophysiology. Acta Biol Szeged, 45(1), 65–73.
- Busquet, P., Capurro, V., Cavalli, A., Piomelli, D., Reggiani, A., & Bertorelli, R. (2012). Synergistic effects of galantamine and memantine in attenuating scopolamine-induced amnesia in mice. Journal of Pharmacological Sciences, 120(4), 305–309. https://doi.org/10.1254/jphs.12166SC
- Collingridge, G. L., & Singer, W. (1990). Excitatory amino acid receptors and synaptic plasticity. Trends in Pharmacological Sciences, 11(7), 290–296. https://doi.org/10.1016/0165-6147(90)90011-V
- Csicsvari, J.,Hirase, H.,Czurko, A., &Buzsáki, G. (1998). Reliability and state dependence of pyramidal cell-interneuron synapses in the hippocampus: An ensemble approach in the behaving rat. Neuron, 21(1), 179–189. 10.1016/s0896-6273(00)80525-59697862
- Danysz, W., Parsons, C. G., Möbius, H.-J., StÖffler, A., & Quack, G. (2000). Neuroprotective and symptomatological action of memantine relevant for Alzheimer’s disease—A unified glutamatergic hypothesis on the mechanism of action. Neurotoxicity Research, 2(2–3), 85–97. https://doi.org/10.1007/BF03033787
- Deardorff, W. J., & Grossberg, G. T. (2016). A fixed-dose combination of memantine extended-release and donepezil in the treatment of moderate-to-severe Alzheimer’s disease. Drug Design, Development and Therapy, 10, 3267–3279. https://doi.org/10.2147/DDDT.S86463
- Farlow, M. (2009). Treatment of Mild Cognitive Impairment (MCI). Current Alzheimer Research, 6(4), 362–367. https://doi.org/10.2174/156720509788929282
- Frankiewicz, T., & Parsons, C. G. (1999). Memantine restores long term potentiation impaired by tonic N-methyl-D-aspartate (NMDA) receptor activation following reduction of Mg2+ In hippocampal slices. Neuropharmacology, 38(9), 1253–1259. https://doi.org/10.1016/S0028-3908(99)00060-X
- Grüter, T., Wiescholleck, V., Dubovyk, V., Aliane, V., & Manahan-Vaughan, D. (2015). Altered neuronal excitability underlies impaired hippocampal function in an animal model of psychosis. Frontiers in Behavioral Neuroscience, 9, 117. https://doi.org/10.3389/fnbeh.2015.00117
- Hasselmo, M. E. (2006). The role of acetylcholine in learning and memory. Current Opinion in Neurobiology, 16(6), 710–715. https://doi.org/10.1016/j.conb.2006.09.002
- Holms, S. (1979). A simple sequentially rejective multiple test procedure. Scandinavian Journal of Statistics, 6, 65–70.
- Lewis, A. S., Van Schalkwyk, G. I., & Bloch, M. H. (2017). Alpha-7 nicotinic agonists for cognitive deficits in neuropsychiatric disorders: A translational meta-analysis of rodent and human studies HHS Public Access. Progress in Neuro-Psychopharmacology and Biological Psychiatry, 75, 45–53. https://doi.org/10.1016/j.pnpbp
- Lockhart, B. P., & Lestage, P. J. (2003). Cognition enhancing or neuroprotective compounds for the treatment of cognitive disorders: Why? When? Which? Experimental Gerontology, 38(1–2), 119–128. https://doi.org/10.1016/S0531-5565(02)00163-8
- Molino, I., Colucci, L., Fasanaro, A. M., Traini, E., & Amenta, F. (2013). Efficacy of memantine, donepezil, or their association in moderate-severe Alzheimer’s disease: A review of clinical trials. The Scientific World Journal, 2013, 1–8. https://doi.org/10.1155/2013/925702
- Mudo, G., Belluardo, N., & Fuxe, K. (2007). Nicotinic receptor agonists as neuroprotective/neurotrophic drugs. Progress in molecular mechanisms. Journal of Neural Transmission, 114(1), 135–147. https://doi.org/10.1007/s00702-006-0561-z
- Nagy, L. V., Bali, Z. K., Kapus, G., Pelsőczi, P., Farkas, B., Lendvai, B., Lévay, G., & Hernádi, I. (2021). Converging evidence on D-amino acid oxidase-dependent enhancement of hippocampal firing activity and passive avoidance learning in rats. International Journal of Neuropsychopharmacology, 24(5), 434–445. https://doi.org/10.1093/ijnp/pyaa095
- Newcomer, J. W., & Krystal, J. H. (2001). NMDA receptor regulation of memory and behavior in humans. Hippocampus, 11(5), 529–542. https://doi.org/10.1002/hipo.1069
- Nikiforuk, A., Potasiewicz, A., Kos, T., & Popik, P. (2016). The combination of memantine and galantamine improves cognition in rats: The synergistic role of the α7 nicotinic acetylcholine and NMDA receptors. Behavioural Brain Research, 313, 214–218. https://doi.org/10.1016/j.bbr.2016.07.023
- Ning, H., Cao, D., Wang, H., Kang, B., Xie, S., & Meng, Y. (2017). Effects of haloperidol, olanzapine, ziprasidone, and PHA-543613 on spatial learning and memory in the Morris water maze test in naïve and MK-801-treated mice. Brain and Behavior, 7(8), 1–6. https://doi.org/10.1002/brb3.764
- Oz, M., Spivak, C. E., & Lupica, C. R. (2004). The solubilizing detergents, Tween 80 and Triton X-100 non-competitively inhibit alpha 7-nicotinic acetylcholine receptor function in Xenopus oocytes. Journal of Neuroscience Methods, 137(2), 167–173. https://doi.org/10.1016/j.jneumeth.2004.02.016
- Papke, R. L., & Horenstein, N. A. (2021). Therapeutic targeting of α7 nicotinic acetylcholine receptors. Pharmacological Reviews, 73(3), 1118–1149. https://doi.org/10.1124/pharmrev.120.000097
- Parsons, C. G., Stöffler, A., & Danysz, W. (2007). Memantine: A NMDA receptor antagonist that improves memory by restoration of homeostasis in the glutamatergic system – Too little activation is bad, too much is even worse. Neuropharmacology, 53(6), 699–723. https://doi.org/10.1016/j.neuropharm.2007.07.013
- Paxinos, G., & Watson, C. (2014). The rat brain in stereotaxic coordinates (7th ed.). Elsevier Academic Press.
- Pismataro, M. C., Horenstein, N. A., Stokes, C., Dallanoce, C., Thakur, G. A., & Papke, R. L. (2021). Stable desensitization of α7 nicotinic acetylcholine receptors by NS6740 requires interaction with S36 in the orthosteric agonist binding site. European Journal of Pharmacology, 905, 174179. https://doi.org/10.1016/j.ejphar.2021.174179
- Potasiewicz, A., Krawczyk, M., Gzielo, K., Popik, P., & Nikiforuk, A. (2020). Positive allosteric modulators of alpha 7 nicotinic acetylcholine receptors enhance procognitive effects of conventional anti-Alzheimer drugs in scopolamine-treated rats. Behavioural Brain Research, 385, 112547. https://doi.org/10.1016/j.bbr.2020.112547
- Rogawski, M. A., & Wenk, G. L. (2006). The neuropharmacological basis for the use of memantine in the treatment of Alzheimer’s disease. CNS Drug Reviews, 9(3), 275–308. https://doi.org/10.1111/j.1527-3458.2003.tb00254.x
- Sarter, M. (2006). Preclinical research into cognition enhancers. Trends in Pharmacological Sciences, 27(11), 602–608. https://doi.org/10.1016/j.tips.2006.09.004
- Schmitt, B., Bernhardt, T., Moeller, H.-J., Heuser, I., & Frölich, L. (2004). Combination therapy in Alzheimer’s disease: A review of current evidence. CNS Drugs, 18(13), 827–844. https://doi.org/10.2165/00023210-200418130-00001
- Szegedi, V., Juhász, G., Parsons, C. G., & Budai, D. (2010). In vivo evidence for functional NMDA receptor blockade by memantine in rat hippocampal neurons. Journal of Neural Transmission, 117(10), 1189–1194. https://doi.org/10.1007/s00702-010-0471-y
- Timmermann, D. B., Grønlien, J. H., Kohlhaas, K. L., Nielsen, E., Dam, E., Jørgensen, T. D., Ahring, P. K., Peters, D., Holst, D., Chrsitensen, J. K., Malysz, J., Briggs, C. A., Gopalakrishnan, M., & Olsen, G. M. (2007). An allosteric modulator of the α7 nicotinic acetylcholine receptor possessing cognition-enhancing properties in vivo. Journal of Pharmacology and Experimental Therapeutics, 323(1), 294–307. https://doi.org/10.1124/jpet.107.120436
- Wang, X., Bell, I. M., & Uslaner, J. M. (2020). Activators of α7 nAChR as potential therapeutics for cognitive impairment. In Current topics in behavioral neurosciences (pp. 209–245). Springer Science and Business Media Deutschland GmbH. https://doi.org/10.1007/7854_2020_140
- Weed, M. R., Polino, J., Signor, L., Bookbinder, M., Keavy, D., Benitex, Y., Morgan, D. G., King, D., Macor, J. E., Zaczek, R., Olson, R., & Bristow, L. J. (2017). Nicotinic alpha 7 receptor agonists EVP-6124 and BMS-933043, attenuate scopolamine-induced deficits in visuo-spatial paired associates learning. PLOS One, 12(12), e0187609. https://doi.org/10.1371/journal.pone.0187609
- Wenk, G. L., Quack, G., Moebius, H. J., & Danysz, W. (2000). No interaction of memantine with acetylcholinesterase inhibitors approved for clinical use. Life Sciences, 66(12), 1079–1083. https://doi.org/10.1016/S0024-3205(00)00411-2
- Woodruff-Pak, D. S., Tobia, M. J., Jiao, X., Beck, K. D., & Servatius, R. J. (2007). Preclinical investigation of the functional effects of memantine and memantine combined with galantamine or donepezil. Neuropsychopharmacology, 32(6), 1284–1294. https://doi.org/10.1038/sj.npp.1301259
- Yang, Y., Paspalas, C. D., Jin, L. E., Picciotto, M. R., Arnsten, A. F. T., & Wang, M. (2013). Nicotinic α7 receptors enhance NMDA cognitive circuits in dorsolateral prefrontal cortex. Proceedings of the National Academy of Sciences, 110(29), 12078–12083. https://doi.org/10.1073/pnas.1307849110