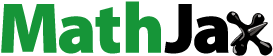
Abstract
In hydrothermal liquefaction (HTL) of biomass, only the oil and the char phases are considered imperative while the aqueous phase is discarded. However, the aqueous phase is rich in phenolic compounds like guaiacol and vanillyl alcohol that can be upgraded to vanillin. Commercially vanillin is synthesized from phenolic compounds derived from fossil fuels using chemical synthesis methods. In this investigation, the method was developed to use raw materials and enzymatic conversion of substrates which is sustainable and renewable. The purpose of this investigation is to evaluate the applicability of enzymatic vanillin synthesis within the HTL aqueous phase major components. Vanillyl alcohol oxidase was expressed in E. coli BL21 (DE3) bacteria cells and purified to characterize the production of vanillin from vanillyl alcohol. The effect on the enzyme reaction of each of the major components of the aqueous phase as detected by HPLC (High Performance Liquid Chromatography) analysis was evaluated. The components evaluated in this study included different phenolic compounds namely guaiacol, vanillic acid, vanillyl alcohol, phenol, catechol and acetic acid. These components were evaluated individually as well as in different combinations. Vanillic acid increased the reaction rate while guaiacol inhibited the reaction rate. Acetic acid exhibited the largest inhibition of the enzyme reaction after 15 min. It is known that acids are often used to stop enzyme reactions as it results in precipitation of the enzyme. Therefore, to successfully convert vanillin from vanillyl alcohol using a recombinantly expressed vanillyl alcohol oxidase, the acetic acid would first need to be removed from the HTL aqueous phase.
Introduction
VAO (vanillyl alcohol oxidase) is an aromatic alcohol oxidase that is covalently bound to Flavin adenine dinucleotide (FAD) [Citation1]. The role of the enzyme is dependent on this covalently bound cofactor. In Penicillium simplicissimum, 4-(methoxymethyl)phenol can induce the expression of VAO [Citation2]. Several studies have described different enzyme reactions since the first isolation of VAO from Penicillium simplicissimum [Citation2, Citation3]. Vanillin and conferyl alcohol are some of the major products generated from VAO reactions from phenolic substrates [Citation3].
Interest in the recombinant production of VAO resulted in the sequencing of the vanillyl alcohol oxidase gene (VaoA) [Citation4] and VAO was subsequently successfully expressed in bacteria cells [Citation3]. In a later study it was shown that eugenol could be converted to ferulic acid at a conversion rate of 91% in 15 h with a concentration of 8.6 g/L [Citation5]. A study that evaluated the effect of single mutations on the VAO active site showed that Asp170 is the critical amino acid responsible for the binding of the first substrate to the active site as well as hydration of the initially formed p-quinone methide intermediate [Citation6].
This study aims to convert phenolic substances in the aqueous phase of the biomass from the HTL (hydrothermal liquefaction) plant [Citation7–9] using enzymatic reactions. Each biomass has a unique composition of different phenolic composition ratios. The biomass analysed in this study contains guaiacol and vanillyl alcohol as major phenolic compounds [Citation10–12]. Guaiacol has a similar structure to vanillin, the only difference being the additional aldehyde group on the benzene ring in vanillin. Guaiacol can be converted to vanillyl alcohol using a chemical formylation reaction [Citation3, Citation13, Citation14]. This will increase the concentration of vanillyl alcohol in the aqueous phase resulting in the increased yield of vanillin from VAO.
The substrate of VAO, vanillyl alcohol, is one of the major components of the HTL aqueous phase from sewage sludge [Citation12]. Enzyme reactions can be affected by several conditions including pH, temperature and media compositions. This study investigated whether the phenolic compounds must be removed from the aqueous phase to maximize enzyme activity. A recombinant VAO enzyme was expressed using a bacterial host [Citation3]. The enzyme was purified and the enzyme activity evaluated in the presence of different phenolic compounds. The main aim of this study is to determine whether vanillyl alcohol can be converted to vanillin thus making naturally labelled flavor compounds [Citation15] from waste products of HTL. Based on literature, the aqueous phase from HTL have not been considered necessary since the HTL is still an emerging way of formation of biodiesel and oils from biomass to subsidies fossil fuels. However, after analysing the contents of the aqueous phase it was realised that plenty of organic substances are being lost which includes before mainly phenolic compounds from sewage sludge.
Materials and methods
Enzyme expression and purification
The plasmids and bacterial strains used in this study were acquired from the UFS (University of Free State, Bloemfontein Campus, Department of Microbial, Biochemical and Food Biotechnology, Biocatalysis Group, South Africa). The PsVAO (Penicillium simplissimum VAO) plasmid contained a VAO gene (VaoA) cloned in pET28 via Nhel and HindIII restriction enzymes under the control of T7 promoter. The protein was transformed and expressed as reported previously by [Citation3], with a replacement of RbCl2 with CaCl2 [Citation16] in the expression media. For protein purification, cell lysis was done by ultrasonic sound sonication, followed by nickel column under native conditions purification as described by [Citation17]. The enzyme concentration was measured using the Bicinchoninic acid assay (Protocol-Mitochondria Lab, North-West University Biochemistry Department). VAO activity was determined at 25 °C in 50 mM potassium phosphate buffer containing 1 mM vanillyl alcohol and 50 µg/ml of a purified enzyme. The absorbance over time was measured at 340 nm using Libra S12 spectrophotometer (Thermo Fisher Scientific) [Citation4].
Determining the composition of the HTL aqueous phase
Hydrothermal liquefaction of sewage sludge biomass was performed according to [Citation12] for 20 min. The aqueous phase product of the liquefaction was used for this investigation. The concentration of each known compound in the HTL aqueous phase was determined using HPLC analysis and Inductively Coupled Plasma (ICP). This included mainly phenolic, sugars and metal ions. A synthetic aqueous phase containing combinations of these compounds was then prepared to evaluate the effect of each compound on the enzyme reaction ().
Table 1. Representation of the concentration of phenolic compounds and acetic acid in the aqueous phase and calculated volume of synthetic aqueous phase making 1 mM vanillyl alcohol volume ratio in 210 µL volume.
Determination of enzyme activity in the synthetic HTL aqueous phase
A vanillin standard curve was prepared using 98% pure vanillin standard with an estimated maximum absorption at 340 nm. Samples were prepared in a linear working range from 0.005 g/L to 0.152 g/L. This was used to relate the concentration of the product to absorbance as the reaction progress. A total of 11 different reactions were setup in triplicate as shown in . The phosphate buffer pH 8, constitutes a concentration of 50 mM in each reaction treatment. The reaction was initiated by adding the enzyme after a 10 min incubation period. A spectrophotometer (microplate reader) was set at 30 °C and allowed to shake for 10 s before each reading. The absorbance was set at 340 nm [Citation4]. The time was set at 5 min intervals, reading for a time frame of 45 min. After 10 min, automatically the plate comes out and the enzyme is added. After enzyme addition the plate was taken in and the reaction started.
Table 2. Representation of the volumes of each component in each of the 11 reactions to make 210 µL in each well of the microplate.
Results and discussion
Enzyme expression and purification
The sequence of the VAO gene (VaoA) cloned in the pET28 vector was confirmed using Sanger sequencing. The sequencing results showed a mutation at base pair position 1011, however, it was determined that this mutation did not alter the amino acid sequence as both codons (GAT and GAC), codes for the same amino acid asparagine [Citation18]. This is consistent with the results reported by [Citation3] under the heterogeneous expression of vanillyl alcohol oxidase.
The expression time was optimized by comparing the level of protein expression at 24, 36 and 48 h. In this study as in previously reported studies, a protein band of 65 kDa was observed on the SDS-PAGE (sodium dodecyl sulfate polyacrylamide gel electrophoresis) [Citation2]. A total time frame of up to 36 h resulted in the highest concentration of protein being produced. The solubility of the expressed protein was analyzed by dividing the cell lysate into the total fraction and soluble fraction (). This result shows both successful cell lysis and solubility of the enzyme [Citation20].
Figure 1. Total fraction and soluble fraction of duplicate samples A and B from the same expression for 36 h using the ultrasonic sound sonication method of cell lysis.
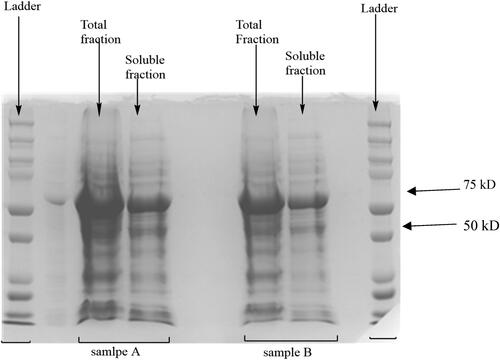
The ProBond purification kit was used for protein purification, where a nickel resin column binds to the six His tag terminal of the protein at low imidazole concentration. The protein is then eluted at higher concentrations of imidazole [Citation17]. The protein elution step resulted in a single high-intensity band at the expected 65 kDa ().
Figure 2. A small scale (8 mL culture) SDS-PAGE gel after purification under native conditions of the sample expressed over 36 h.; (b) Wells 1-8 are elution aliquots.
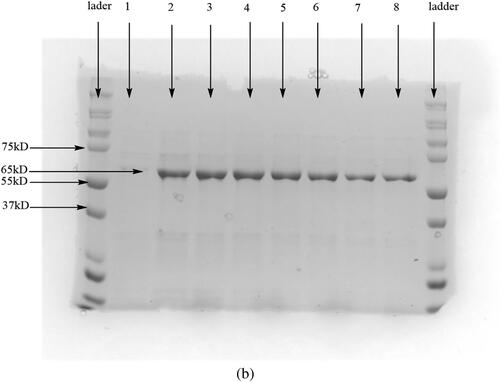
From the 50 mL culture used for expression, 8 mL of the enzyme was obtained. The enzyme concentration determination was through the BCA method using BSA as a standard [Citation22]. A concentration of 2.2 mg/mL of the enzyme was obtained. Following protein quantification, the activity of the enzyme was monitored at different concentrations of the enzyme using the same concentration of substrate.
From these reactions, the enzyme units calculated gave 0.92 U/mg of enzyme. This was higher than the data reported by [Citation3], namely 0.017 U/mg and 0.028 U/mg. However, the high specific enzyme activity seen in this study is due to the purification of the enzyme using a His fusion tag as opposed to previous authors [Citation3] that used cell free extracts.
Determination of enzyme activity in the synthetic HTL aqueous phase
A series of 11 reactions were setup representing the components of the HTL aqueous phase in their ratios. These ratios have been detailed in and . These reactions were used to determine which combinations of compounds inhibit the enzyme reaction. ().
Figure 3. The quantity of product produced at each time interval from the enzyme with different treatments of the HTL aqueous phase components. Error bars represent the standard error of the mean at 95% confidence level. The abbreviations on the series are represented as follows: VA: vanillyl alcohol; VAc: vanillic acid; Ph: phenol; Cat: catechol; Gua: guaiacol; Ac: acetic acid; Enz: enzyme.
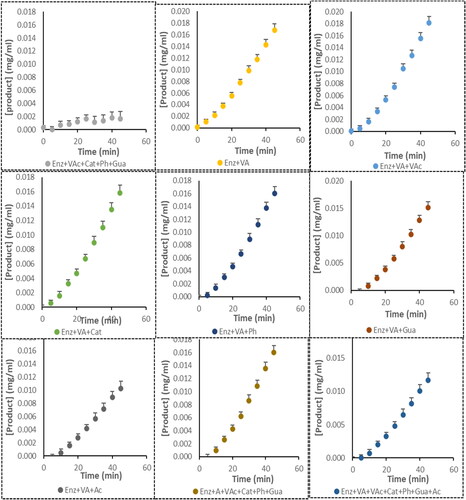
Reactions 1 (no enzyme) and 2 (no substrate), as described in , are the control reactions and showed no enzyme activity. Reaction 3, first in the chart, without vanillyl alcohol (Enz + VAc + Cat + Ph + Gua) shows only a slight increase in product from 0 to 0.0016 mg/mL in 45 min. From this observation two possibilities can be deduced, either phenolic compounds other than vanillyl alcohol are converted into a product (vanillin) or a different product that absorbs light at the same wavelength of 340 nm is present [Citation23]. The second assumption is that some or one of the phenolic compounds decomposed slightly in aqueous solution to form vanillin [Citation23]. In an aqueous solution, there is a possibility of slight dissociation (reduction) of the COOH group into CHO and/or CH2OH [Citation24]. Therefore, the resulted product can be ascribed to dissociation and not to enzyme action on any of the components other than vanillyl alcohol.
Reactions 4 – 11, containing the substrate and the enzyme, showed an increase in product concentration over 45 min irrespective of the other components in the same solution. The substrate does not inhibit the reaction as there is a steady increase in the rate of the enzyme reaction over the 45 min period.
The concentration of the product was quantified and compared between the 11 reactions to determine if there were any significant differences at each time interval (). Statistically significant differences are shown by overlapping positive error bars while non-overlapping error bars show non-significant differences. At times 0 to 10 min there was no significant difference between the 11 different treatments.
Figure 4. Each graph shows the concentration of product in each of the eleven reaction mixtures per given time. Error bars represent the standard error at a 95% confidence level. Each one of the graphs has a different scale of concentration for clarity’s sake, therefore the graphs are not drawn to scale. The key shows the corresponding concentrations of each number in the x-axis.
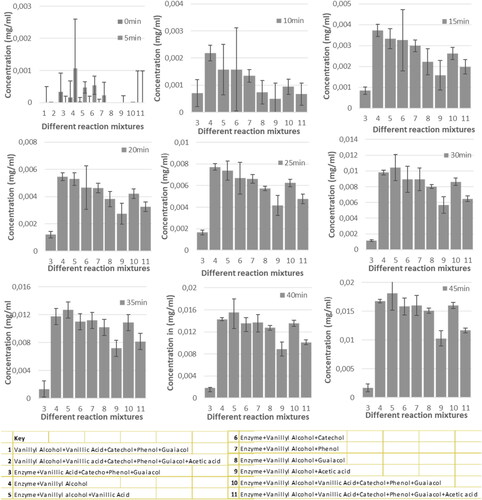
For the enzyme containing reactions, a difference was observed for reaction 9 (Enz + VA + Ac), which contains acetic acid in addition to the substrate from 15 min. This is shown by the non-overlapping error bars of this reaction treatment with all the others. However, from 20 min for the enzyme reactions containing acetic acid a significant difference to the other treatments were observed as shown in . There was also a difference in the six enzyme and vanillyl alcohol treatments with no acetic acid. The vanillic acid containing reaction showed a significant difference to the reference reaction from 30 min. From this observation, paying attention to the products of the oxidation of vanillyl alcohol to vanillin, hydrogen peroxide is one of the products. Under alkaline conditions, hydrogen peroxide decomposes in solution. Hydrogen peroxide at higher amounts in the reaction leads to deep oxidation of vanillin leading to vanillic acid formation [Citation26]. As more hydrogen peroxide accumulates with time, vanillin formed may be converted to vanillic acid [Citation26]. For this reaction, there is plenty of vanillic acid in solution considering the equilibrium laws [Citation27], it will be difficult for hydrogen peroxide to favor the formation of vanillyl alcohol. Thus, the vanillin formed remains constant with time, while in the standard without vanillic acid, the rate is slower during the reaction progress. This is because vanillin formed is channeled towards vanillic acid with the effect of hydrogen peroxide produced.
The phenol and catechol reactions are the only treatments that have no significant difference to the control reaction. There is a significant difference in guaiacol containing treatments. Guaiacol has a similar structure to vanillyl alcohol (the substrate), only differing in that vanillyl alcohol has an additional CH2OH group. From the reaction mechanism of VAO [Citation28], it is the proton on the OH group attached to the ring that is first attacked by amino acid ASP170 in the enzyme active site [Citation1]. Both guaiacol and vanillyl alcohol has this OH group in a similar confirmation, therefore, there is a possibility of guaiacol inhibiting the enzyme reaction as it can also enter the active site. Thus, when guaiacol enters the active site, it can participate in the first step of the reaction, but no further reaction will occur as it lacks the para-CH2OH group. Guaiacol can therefore act as a competitive inhibitor of VAO. In previous studies, the aqueous phase was utilized through successive recycling to extract the important components back into the bio-oil [Citation31–33], alternatively for algal growth where the algae is used as biomass for bio-oil production [Citation34]. However, in this study a new method which aims to recover all the phenolic compounds with minimum energy use i.e. in the recycle steps, was initiated. This study targeted only vanillyl alcohol, but more work needs to be done on other phenolic components so that multiple enzymes can be used for the recovery of the total phenolic compounds. There was no specific work which can be referenced for comparison on the utilization of aqueous phase through bio-upgrade.
Conclusions
This study evaluated whether vanillyl alcohol can be converted to vanillin in the HTL aqueous phase using a recombinant expressed VAO enzyme. The VAO enzyme was successfully expressed, purified, and characterized using vanillyl alcohol as substrate. The main goal was to observe the effect of each component in the HTL aqueous phase on the reaction rate of VAO with vanillyl alcohol to yield vanillin. This is a step towards the upgrade of the aqueous phase rich in vanillyl alcohol (0.52 g/L) to bio-based vanillin. From the results obtained, all the phenolic components of the HTL aqueous phase permitted the reaction to occur albeit at different rates. The reaction containing vanillic acid showed an increased reaction rate, while for phenol and catechol there was not much deviation from the normal vanillyl alcohol and VAO reaction. Acetic acid and guaiacol significantly inhibited the rate of product formation with acetic acid having a greater inhibitory effect. Acids are often used to stop enzyme reactions as they result in the precipitation of the enzyme. Therefore, due to its higher effect on the reaction rate, a recommendation may be that acetic acid should be removed from the aqueous phase prior to the enzyme reaction [Citation19, Citation21, Citation25, Citation29, Citation30].
Disclosure statement
No potential conflict of interest was reported by the authors.
Additional information
Funding
References
- Ewing TA, Fraaije MW, Mattevi A, et al. The VAO/PCMH flavoprotein family. Arch Biochem Biophys. 2017;632:104–117.
- De Jong E, van Berkel WJH, van der Zwan RP, et al. Purification and characterization of vanillyl‐alcohol oxidase from Penicillium simplicissimum: a novel aromatic alcohol oxidase containing covalently bound FAD. Eur J Biochem. 1992;208(3):651–657.
- Van Rooyen N. 2012. Identification, cloning andHeterologous expression of fungal vanillyl-alcohol oxidases [doctoral dissertation]. Bloemfontein: University of the Free State.
- Benen JAE, Sánchez-Torres P, Wagemaker MJM, et al. Molecular cloning, sequencing, and heterologous expression of the VaoA gene from penicillium simplicissimum CBS 170.90 encoding vanillyl-alcohol oxidase. J Biol Chem. 1998;273(14):7865–7872.
- Overhage J, Steinbüchel A, Priefert H. Highly efficient biotransformation of eugenol to ferulic acid and further conversion to vanillin in recombinant strains of Escherichia coli. Appl Environ Microbiol. 2003;69(11):6569–6576.
- Van Den Heuvel RHH, Fraaije MW, Ferrer M, et al. Inversion of stereospecificity of vanillyl-alcohol oxidase. Proc Natl Acad Sci USA. 2000;97(17):9455–9460.
- Kang S, Li X, Fan J, et al. Classified separation of lignin hydrothermal liquefied products. Ind Eng Chem Res. 2011;50(19):11288–11296.
- Gollakota ARK, Kishore N, Gu S. A review on hydrothermal liquefaction of biomass. Renewable Sustainable Energy Rev. 2018;81:1378–1392.
- Beims RF, Hu Y, Shui H, et al. Hydrothermal liquefaction of biomass to fuels and value-added chemicals: products applications and challenges to develop large-scale operations. Biomass Bioenergy. 2020;135:105510.
- Li C, Yang X, Zhang Z, et al. Hydrothermal liquefaction of desert shrub Salix psammophila to high value-added chemicals and hydrochar with recycled processing water. BioResources. 2013;8(2):2981–2997.
- Zhu Z, Toor SS, Rosendahl L, et al. Influence of alkali catalyst on product yield and properties via hydrothermal liquefaction of barley straw. Energy. 2015;80:284–292.
- Sanette M, Venter RJ, Louw A, et al. Upgrading of the aqueous product stream from hydrothermal liquefaction: simultaneous removal of minerals and phenolic components using waste-derived hydrochar. Biomass Bioenergy. 2021;151:106170.
- Bolognini M, Cavani F, Dal Pozzo L, et al. Guaiacol hydroxyalkylation with aqueous formaldehyde: role of surface properties of H-mordenites on catalytic performance. Appl Catal A Gen. 2004;272(1–2):115–124.
- Ardizzi LMM, Cavani F, Dal Pozzo L. 2005. Hydroxymethylation of 2-methoxyphenol with formaldehyde to yield vanillic alcohols: a comparison between homogeneous and heterogeneous acid catalysis. In: John J, Sowa R, editor. Catalysts of organic reactions. 1st ed. Bologna, Italy: CRC Press. p. 357–361.
- Banerjee G, Chattopadhyay P. Vanillin biotechnology: the perspectives and future. J Sci Food Agric. 2019;99(2):499–506.
- Mayer M. A new set of useful cloning and expression vectors derived from pBlueScript. Gene. 1995;163(1):41–46.
- Thermofisher. 2012. ProBond TM purification system for purification of polyhistidine-containing recombinant proteins. https://assets.thermofisher.com/TFS-Assets/LSG/manuals/xprpur_man.pdf.
- Benyó B, Biro JC, Benyó Z. Codes in the codons: construction of a codon/amino acid periodic table and a study of the nature of specific nucleic acid—protein interactions. In: The 26th Annual International Conference of the IEEE Engineering in Medicine and Biology Society. Vol. 26; 2014. p. 2860–2863.
- Sundar IK, Sakthivel N. Advances in selectable marker genes for plant transformation. J Plant Physiol. 2008;165(16):1698–1716.
- McCormick AM, Jarmusik NA, Endrizzi EJ, et al. Expression, isolation, and purification of soluble and insoluble biotinylated proteins for nerve tissue regeneration. J Vis Exp. 2014;83:1–11.
- Gygli G, Berkel WJHv Communication. Vanillyl alcohol oxidases produced in komagataella phaffii contain a highly stable noncovalently bound anionic FAD semiquinone. Biocatalysis. 2017;3(1):17–26.
- Scientific T. 2007. BCATM protein assay kit.
- Bisswanger H. Enzyme assays. Perspect Sci. 2014;1(1–6):41–55.
- Zimmerman J. Physical chemistry for the biosciences: Chang, Raymond. Biochem Mol Biol Educ. 2005;33(5):382–382.
- Skoog DA, West DM, James Holler F, et al. 1992. Fundamentals of analytical chemistry. 6th ed. New York (NY): College Publishing.
- Chang R. 2005. Enzyme kinetics in physical chemistry for the biosciences. Sausalito (CA): University Science Books.
- Elamathi P, Kolli MK, Chandrasekar G. Catalytic oxidation of vanillyl alcohol using FeMCM-41 nanoporous tubular reactor. Int J Nanosci. 2018;17(01n02):1760010.
- Ghirardi M, Marchetti F, Pettinari C, et al. Implementing an equilibrium law teaching sequence for secondary school students to learn chemical equilibrium. J Chem Educ. 2015;92(6):1008–1015.
- Mattevi A, Fraaije MW, Mozzarelli A, et al. Crystal structures and inhibitor binding in the octameric flavoenzyme vanillyl-alcohol oxidase: the shape of the active-site cavity controls substrate specificity. Structure. 1997;5(7):907–920.
- Husnaeni H, Maruddin F, Malaka R, et al. 2019. Study on the use of various concentration of acetic acid and different precipitation duration on casein characteristics. In: IOP Conference Series: Earth and Environmental Science.
- Das P, Khan S, AbdulQuadir M, et al. Energy recovery and nutrients recycling from municipal sewage sludge. Sci Total Environ. 2020;715(1):136775.
- Edmundson S, Huesemann M, Kruk R, et al. Phosphorus and nitrogen recycle following algal bio-crude production via continuous hydrothermal liquefaction. Algal Res. 2017;26(1):415–421.
- Leng L, Li J, Wen Z, et al. Use of microalgae to recycle nutrients in aqueous phase derived from hydrothermal liquefaction process. Bioresour Technol. 2018;256:529–542.
- Leng L, Zhang W, Leng S, et al. Bioenergy recovery from wastewater produced by hydrothermal processing biomass: progress, challenges, and opportunities. Sci Total Environ. 2020;748:142383.
Appendix A.
Supplementary data
The following are the Supplementary data to this article:
The recombinant plasmid was synthesized from the University of Free State, South Africa, Bloemfontein campus, Department of Microbial, Biochemical and Food Biotechnology, Biocatalysis Group.
The error bars in show the LSD for the 11 data points was calculated per given time. The standard error of each data point at 95% level () was first calculated, then the average of these errors gives the LSD: