Abstract
This study examined the milk quality of the autochthonous cattle breed Modenese (MO) in comparison with Italian Friesian (IF), at peak and mid lactation, with the aim to support the interest in sustainability of the local cattle breeds in Europe. Forty-eight individual milk samples were collected from 11 MO and 14 IF pluriparous cows, housed in a free stall barn under similar conditions of feeding and management, at 8 and 21 weeks post-calving; daily milk yield (MY) and body condition score (BCS) were individually recorded. Breed differences were observed, being MY lower in MO cows, but BCS, titratable acidity, freezing point, casein (MC), total calcium (Ca), total phosphorus (P) and colloidal P contents were higher compared to IF cows. Time affected BCS, MY, milk protein (MP), MC, urea, casein P and the micellar content of colloidal Ca and colloidal inorganic P. A factorial analysis was performed and four common factors were obtained with a cumulative explained variance of 77.7% of the total original. MO milk showed a nutritionally interesting mineral profile and processing properties suitable for the production of typical cheese that could be interesting for improving the interest in the conservation of animal genetic resources.
Introduction
The European Union (EU) recognises the importance of autochthonous breeds for the conservation of animal genetic resources: as local cattle breeds are less productive than the breeds selected for milk or meat production, the EU offers economic incentives to most farmers keeping these animals. However, conservation of autochthonous breeds based on public financial support is not practicable for a long time-period; moreover, breeders of local breeds demand help to promote their animal products, even to preserve family and area traditions (Gandini et al. Citation2010). In Italy, efforts are now under way to repopulate the rural areas with the indigenous breeds to ensure genetic variability in livestock, as they are well adapted to the native environment and have special characteristics, as better functional traits and milk composition, that distinguish them from the commercial breeds (Summer et al. Citation2002; Duclos & Hiemstra Citation2010; Martin-Collado et al. Citation2010). An approach for compensating the lower milk yield from autochthonous cattle breeds in comparison with specialised dairy breeds could be raising the prices of traditional high-quality products linked to local breeds (Martin-Collado et al. Citation2010), by emphasising the special quality characteristics of these products. An important traditional activity in Italy is the production of typical cheeses made with milk from local breeds, as the ‘Parmigiano-Reggiano of Modenese’, the main milk product manufactured in the Emilia Romagna Region with milk of Modenese (MO), a dual-purpose local cattle breed included in the endangered-maintained breed list of FAO (Citation2000). Detailed milk composition should be taken into account when developing strategies useful for milk and dairy products valorisation. For example, Ramalho et al. (Citation2012) compared the autochthonous Portuguese cattle breed Minhota with the Holstein and found a pronounced effect of breed on milk fat-soluble vitamins, and considered the higher milk vitamin contents found in Minhota cows as an important nutritional parameter to promote the valorisation of that breed.
Substantial differences among cattle breeds for milk composition, rennet coagulation properties (RCP), mineral content and cheese quality have been observed (Malacarne et al. Citation2006; De Marchi et al. Citation2007; van Hulzen et al. Citation2009) and attributed to genetic factors The colloidal fractions of calcium (Ca), phosphorus (P) and magnesium (Mg) are essential constituents of the micelles, influencing their structure and functionality, with repercussion on milk rennetability and cheese texture (Gaucheron Citation2005; Bijl et al. Citation2013; Malacarne et al. Citation2014). Moreover, minerals are of major importance when considering the nutritional value of milk (Pirilä et al. Citation2011). Indeed, cow’s milk and dairy products, widely consumed by children, are the best sources of Ca in human diet, helping to achieve adequate dietary Ca intake (Gao et al. Citation2006; Pirilä et al. Citation2011); consequently, milk with higher Ca, P and Mg concentrations, being highly bioavailable, is thus desirable. To summarise possible differences in these qualitative features and compare the milk from two different breeds, it is interesting to analyse the presence of a latent structure in the data that reduce the number of variables necessary to describe the variability among the explored samples. The multivariate analysis of data by a Principal Factors Analysis (PFA) may reduce a high number of original variables into a small number of Principal Factors (PF), a new set of variables which are not correlated.
In a previous work (Petrera et al. Citation2015) we demonstrated a different ability of the MO breed to cope with metabolic stress during the peripartum period compared to the specialised dairy breed IF, suggesting the hypothesis that also differences in energy and lipid metabolism could depend on the different merit for milk production between the two breeds.
In the past, as Mariani (Citation1985) as Summer et al. (Citation2002) compared herd milk samples from MO and IF cows reared in the Parmigiano-Reggiano cheese production area and found milk from MO cows had higher concentrations of caseins and minerals than IF breed; however, in their studies the two breeds were fed different diets. The aim of the present study was to estimate the effect of breed on chemical composition and, in particular, on the casein and mineral contents of milk from MO and IF cows fed the same diet (in the Grana Padano cheese production area) and evaluate the effect of the stage of lactation in these two breeds. New perspectives for the use of MO milk may be opened if MO milk has a better milk mineral profile, considering the advantage in mineral gut absorption from the colloidal fraction, as evidenced by Möller et al. (Citation2008).
We aimed, through this zootechnical characterisation, to support the interest in sustainability and conservation of the local cattle breeds reared in Europe and to improve the economic efficiency and the marketability of their animal products by the valorisation of particular nutritional properties.
Materials and methods
Animals and milk sampling
The study was carried out on 25 multiparous lactating cows, 14 Italian Friesian (IF) and 11 MO that calved between February and August 2010. For the description of the barn where the cows were housed, the animal management, feeding and milking, please see the work of Petrera et al. (Citation2015). The characteristics of the cows involved in the present study are reported in Table .
Table 1. Characteristics of the MO and IF breeds in the present study (means ± SD).
Forty-eight individual milk samples (22 from MO and 26 from IF cows) were collected during the morning milking at 8 weeks (T8, 56.8 ± 7.2 DIM) and 21 weeks (T21, 153.2 ± 10.2 DIM) post-calving. Milk yield (MY) was individually recorded during the morning and the evening milking and body condition score was assessed as described in Petrera et al. (Citation2015).
Milk parameters
The analysis on fresh milk samples included fat, protein (MP), lactose (ML) and casein (MC) concentration (Milko-Scan FT 6000, Foss Electric, DK-3400 Hillerød, Denmark) and somatic cell count (SCC, optofluorometric method using Fossomatic 5000, Foss Electric, DK-3400, Hillerød, Denmark).
The pH and titratable acidity (TA) were measured as reported by Malacarne et al. (Citation2008). Briefly, pH was determined with a potentiometer (Crison Instruments, Barcelona, Spain) and TA by titration with 0·25 M NaOH using the Soxhlet-Henkel method and reporting the results as mL of NaOH solution necessary to bring the pH of 50 mL milk to 8.5 (°SH/50 mL). The freezing point (FP) of milk was assessed by a thermistor cryoscope, according to Hortvet (Citation1921).
The rennet coagulation parameters, the contents of dry matter, ash, Ca, Mg (in milk and skimmed milk ultrafiltrate), and P (in milk, skimmed milk ultrafiltrate and milk after treatment with trichloroacetic acid) were determined as reported in Malacarne et al. (Citation2014, Citation2015). Distribution of Ca, P and Mg fractions were calculated according to White and Davies (Citation1958).
Ultrafiltration was carried out in ultrafiltration-stirred cell (Model 8200, Millipore Corporation, Bedford, MA), at room temperature, following the user guide. Polyethersulfone ultrafiltration membranes (nominal molecular weight limit 30,000) were purchased from Millipore (Millipore Corporation, Bedford, MA). The micellar chemical composition parameters were calculated as the ratio to 100 g of casein.
Statistical analyses
Before submission to ANOVA-based procedures, the normality of the distribution for the traits was inspected; the coefficients of skewness and kurtosis were computed by PROC UNIVARIATE (SAS Institute Inc. 2009), to measure the distribution asymmetry and peakedness.
The repeated measurement on each cow (BCS and milk components) was processed using a mixed model (PROC MIXED) as follows:
where yijk is the dependent variable (BCS, milk components), μ is the general mean, Bi is the fixed effect of the ith breed (IF and MO), Tj is the fixed effect of the jth time (T8 and T21), BTij is the first-order interaction between B and T, and ɛijk is the random experimental error. For each variable, five covariance structures were tested to choose (according to the Akaike Information Criteria) the one which best fitted the data: simple, compound symmetry, autoregressive of first order, ante-dependence, and unstructured. Differences were considered significant at p < .05; a trend was considered when p < .10.
Simple (SLR) and multiple linear regressions (MLR) were developed using MP, MC and ML as independent variables and milk Ca, P or Mg as dependent variablse considering data collected at T8, T21 and T8 plus T21 according to the following models:
where yi is the dependent variable (milk Ca, P or Mg as total or colloidal form), x1i and x2i are observations i of independent variables x1 and x2 (MP, MC, and ML), β0, β1 and β2 are regression parameters, and ɛi is the random error.
The independent variable was retained only when its p value was above .15. We considered only equations with adjusted R2 above that attained with simple regression on MP or MC. Pearson’s and partial correlations among milk parameters, parity and age of animals were measured considering data from T8 plus T21.
A principal factor analysis (PFA) was performed considering all the animal and milk variables at T8 plus T21 using the FACTOR procedure of SAS. Using the principal factor component method (PRIN method) and retaining only principal factor components (PCs) with eigenvalues higher than 1.0, four PCs were extracted and rotated by orthogonal Varimax rotation (ROTATE option). This procedure generated loading (correlations between common latent factors and original variables) vectors. Variables with loading vectors higher than 0.50 were considered to load on specific extracted PCs. In particular, this threshold value was obtained by multiplying the critical value of a correlation coefficient at α=.01 for a two-tailed test (i.e. .2) by 2.5, as previously described by Gallo et al. (Citation2013). The extracted PCs were interpreted on the base of loading vectors. Then, regression coefficients (i.e. standardised scoring coefficients) for each original variable on each extracted PC were calculated using the SCORE option of the FACTOR procedure of SAS and attributed to each observation on extracted PC. These scores were analysed by the MIXED procedure according to the above-mentioned design for the single analytical variables, and results were expressed as LSMEANS with their standard error. Significance was declared at p < .05.
Results
Body condition score, MY and its composition are shown in Table . The BCS was higher (p < .0001) in MO cows compared to IF cows at peak (T8) and mid-lactation (T21). On the contrary, daily MY was markedly affected by breed (p < .0001) and time (p = .0009). Milk production was significantly lower in MO than IF, both at T8 (−25%, p = .001) and, especially, at T21 (−35%, p < .001). As expected, a significant drop in milk production was recorded with the progress in lactation both in MO (−27%; p < .01) and IF cows (−16%; p < .05).
Table 2. Body condition score, milk yield and milk composition in MO and IF cows.
The contents of fat, MP, ML, casein index and SCC were similar in the milk of the two cattle breeds, on the contrary, MC resulted significantly higher (+5%, p < .05) in MO milk than IF milk. A significant (p < .001) increase of MP and MC contents was observed in both milks from T8 to T21; on the contrary, a decrease of ML concentration was observed, but only in MO milk (p < .05). On average, the FP was lower in MO compared to IF milk (p < .05), but it did not vary with time in both the breeds.
The traits related to milk coagulation are shown in Table . The values of milk pH and the three rennet coagulation parameters (RCP) did not differ between the two breeds; on the contrary, TA resulted higher (p < .01) in MO milk than IF milk. We did not observe any variation according to the time of sampling for these parameters in both the cattle breeds.
Table 3. Milk coagulation properties in MO and IF cows.
Dry matter (DM), ash, Ca, P and Mg concentrations and the micellar content of Ca, P and Mg in MO and IF milk are shown in Table . Ash, DM and their soluble forms did not differ between breeds or sampling time. On average, total Ca and total P (p < .05) were higher in MO milk than IF milk (117.43 vs 112.87 and 96.71 vs 90.93 mg/100 g, respectively), but no significant differences were seen between breeds in the amount of soluble Ca, total Mg or in its soluble or colloidal form. The colloidal Ca content was only numerically higher in the MO milk than IF one, especially at T21. Concerning the P fractions, the colloidal form was higher (p < .05) in MO milk than IF milk at the peak of lactation, being the value significantly (p < .05) increased at T21 compared to T8 in MO cows. No significant differences between MO and IF milk were reported for soluble P, casein P or colloidal inorganic P (Pi). The stage of lactation affected only the casein P content which increased (p < .05) in the milk of MO from T8 to T21. As regards the composition of the casein micelle, the breed did not affect any of these parameters; on the contrary, we observed a decrease from T8 to T21 for the micellar Ca and the micellar colloidal P content (p < .05) in IF milk.
Table 4. Milk dry matter, ash, mineral concentration and micellar content of Ca, P and Mg in MO and IF cows.
The graphs in Figure describe the dependence of milk total P content on MP (a) and MC (b) contents in both the breeds at T21. The simple regression analysis equations showed higher increase of P content in milk by elevating the MP or MC concentration in MO compared to IF cows.
Figure 1. Estimated simple linear regression between (a) milk total P (mg/100 g milk) and (b) milk protein or casein (g/100 g milk) at 21 weeks post-calving in MO and IF cows.
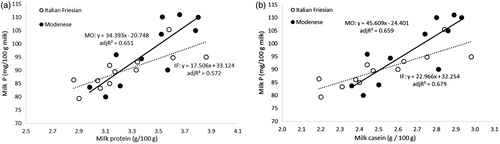
The MLR equations to predict milk P content (mg/100 g milk) counting the effect of MP and ML content (g/100 g milk) at T21 were:
P in MO milk = −104.82 (± 54.737) + 38.154 (± 7.367) * MP +15.17 (± 8.859) * ML; adjusted R2 = 0.713;
P in IF milk = −55.43 (± 30.579) + 14.54 (± 3.3842)* MP +20.803 (± 6.739) * ML, adjusted R2 = 0.769.
The equations describe the relationship between MP plus ML and milk P content showing higher slope for MP in MO than IF. The SLR and MLR analysis developed using milk Ca and Mg as dependent variables explored in our analysis did not perform in a satisfactory way in terms of adjusted R2, so they were neither reported nor discussed.
Table reports the Pearson’s and partial correlations among the milk parameters; this comparison allows us to understand among what original variables the latent structure of data relies.
Table 5. Pearson (above the diagonal) and partial (under the diagonal) correlations among milk parameters considering data from MO and IF cows from T8 plus T21.
Table shows the rotated factor patterns and the communality of the original variables; four variables with a cumulative explained variance of 77.7% of the total original were obtained. Although for a dataset to be suitable for multivariate analysis the optimal overall Kaiser’s value should be over 0.8, the one obtained for the analysed samples (0.59) was close to the results obtained by Macciotta et al. (Citation2012) and quite higher than the lower limit (0.50) proposed by Cerny and Kaiser (Citation1977). Results from ANOVA on the four extracted factors for each observation evidencing for Factor1 a breed (p < .05) and a time (p < .01) effect (−0.140 and 0.868 for MO and −0.638 and 0.077 for IF cows at T8 and T21, respectively) and for Factor3 only a time (p < .001) effect (0.576 and −0.522 for MO and 0.415 and −0.533 for IF at T8 and T21, respectively).
Table 6. Rotated factor pattern and variable communality.
Discussion
The present study compared BCS, MY and its composition between two different cattle breeds at peak (T8) and mid-lactation (T21). Daily MY was higher and BCS lower in IF cows compared to MO cows at both sampling points, as found by the study of Walsh et al. (Citation2008) comparing Holstein Friesian with Montbeliarde and Normande, two breeds selected for milk and meat production. Therefore, we should consider these differences between MO and IF breeds as consequence of the different selection criteria adopted for them: for IF cows the goal was a typical milk yield-based selection; on the contrary, the MO breed was selected as dual-purpose breed, with stronger emphasis on dairy rather than beef traits (Duclos & Hiemstra Citation2010).
In the review of Summer et al. (Citation2002), that summarises the results of several studies carried out in the years 1960–1980, the milk composition of MO cows reared in herds producing milk for Parmigiano-Reggiano cheese was characterised by higher contents of casein, total Ca and particularly P, especially when compared to IF milk.
Differences in the contents of Ca and P are largely dependent on variations in the casein content between the two breeds; as about 66% of Ca and 50% of P are intimately associated with casein (Bijl et al. Citation2013). Differences among cattle breeds for milk mineral composition were in the past evidenced by Cerbulis and Farrell (Citation1976), particularly Jersey cows showed higher values of Ca, P and Mg compared to Holstein cows.
The aptitude of milk to rennet coagulation is the main requisite for milk employed in cheesemaking, especially in the manufacture of hard ripened cheese, as Grana Padano or Parmigiano Reggiano. The three rennet coagulation parameters did not vary significantly between the two breeds, probably because of the high variability between samples, as confirmed by the high MSE observed. However, as in the past milk from MO cows showed worse rennet coagulation parameters than IF ones (Pecorari et al. Citation1987), these results could be viewed as an improvement of the rennet coagulation aptitude of MO milk. We observed an improvement on the secondary phase rennet coagulation in MO milk in this study, i.e. the aggregation of paracasein micelle during curd formation. The improved rennetability of MO milk observed here could depend on the increased content of inorganic P in the casein micelle, as the positive effect of micellar colloidal Ca phosphate (CCP) (Malacarne et al. Citation2014) on rennet-curd formation.
A marked difference with previous studies concerns the degree of mineralisation of the casein micelle of MO milk. In a study comparing the micellar system in the milk of Reggiana, Italian Brown, IF and MO cattle breeds, Mariani (Citation1985) evidenced how the casein micelle of MO milk was characterised by the lowest content of Pi. In our study, we observed an increase in the content of Pi per 100 g of casein compared to Mariani (Citation1985). Besides improved feeding practises and management conditions, differences with past report could depend on changes in the genetic background of the MO cattle breed throughout years. Changes in the gene pool and allele frequencies are probably the results of the decreased number of MO heads from a maximum of 120,000 in 1940, to about 300 cows in 2000, until about 650 cows in 2010 (Duclos & Hiemstra Citation2010).
Concerning the differences between breeds in milk FP, Hanuš et al. (Citation2010) underlined the dependence of milk FP on daily MY, particularly within the Holstein type. The difference seen in the present study agrees with the results from Kędzierska-Matysek et al. (Citation2011), who suggested how this is the result from differences in MP, ML, chloride salts and water-soluble constituents such as Ca, K and Mg.
Correlations among colloidal fractions of Ca, P and Mg were expected, as they interact at a fixed ratio in micellar CCP nanocluster (Holt et al. Citation2013), and are in agreement with those by Bijl et al. (Citation2013). Results from the experiment of Neville and Peaker (Citation1979) that infused labelled phosphate and Ca into the mammary artery of lactating goats, suggest that milk Ca and P in their various forms are secreted into milk by exocytosis of Golgi vesicles, so as protein and lactose. Although the equations developed by Klop et al. (Citation2014) to describe the relationship between milk P concentration and MP and ML contents are similar to our findings, differences observed between the two breeds are difficult to explain.
As reported in Table , the marked difference between Pearson’s and partial correlations coefficients suggests a latent correlation structure among some variables, according to Macciotta et al. (Citation2012).
The partitioning of explained variance was concentrated in the first factor (Table ). According to Macciotta et al. (Citation2012), SCC was characterised by the largest value of uniqueness (1 – communality), because of its random origin of variation. The first common or latent factor was defined as ‘protein–mineral distribution’ because it was linked to MC, MP and mineral concentrations, in terms of total and colloidal contents, and with FP; the second latent factor was defined as ‘animal age’, because it was correlated to parity and age of the cows, and negatively to the titratable acidity. The third factor was defined as ‘mammary secretion’ because it was positively linked to ML and casein index, and negatively to pH and DIM. This pattern suggests how the third factor may be associated with the evolution of the secretory function throughout lactation. The fourth factor was named ‘fat’ because it was essentially linked to milk fat content and milk fat-to-protein ratio.
A part of the variation in the milk mineral contents in MO cows could be under genetic controls and these characteristics could be improved by selection, as the heritability of milk Ca and P content in cattle was estimated to be 0.57 and 0.62, respectively (van Hulzen et al. Citation2009).
Factor1 (called ‘protein-mineral distribution’) seems to synthesise well the features of main interest for human macro-mineral nutrition. The ‘responsiveness’ of this factor to either breed and stage of lactation encourages testing and validating its use in the comparison between other different sources of variation in assessing milk suitability to reach a nutritional target.
Conclusions
The results of this study showed significant differences between the two breeds as regards milk composition, when cows received the same diet and were exposed to the same management conditions. MO milk shows an interesting mineral profile for human nutrition with higher total Ca and P than that of the IF breed, not influenced by the stage of lactation. As consequence, consumption of milk and milk products from MO breed could help to cover the daily requirement of essential minerals, maintaining bone health and preventing osteoporosis. Moreover, milk from specific cattle breeds can play an important role in the diets of children or in populations with very low nutrient intakes (FAO Citation2013).
The higher values of casein and TA observed in MO milk should influence positively its cheese yield. Furthermore, the increased degree of mineralisation of MO milk casein micelle should have positive effect on the rheology of the curd, which in the past was defined as ‘mealy’, especially when compared to the ‘gelatinous’ curd resulting from Reggiana and Brown milk.
The adoption of milk payment based on specific milk characteristics, as casein or mineral contents, should be considered for the valorisation of milk for cheese making. On the other hand, the possibility to label milk and dairy products of local breeds with specific nutritional properties, as high bioavailable Ca and P contents, could help to improve their marketability and the economic efficiency of the farms, contributing to sustain the safeguarding of the autochthonous cattle breeds reared in Italy and Europe.
Acknowledgements
The authors want to thank the farmers of the herd ‘Soc. Sempl. Agricola Cornetti Alessandro e F.lli’, Quinzano d’Oglio (BS) for the on-farm management of the trial. Furthermore, the authors want to thank Conception Ayala for the English Revision.
Disclosure statement
The authors report no conflicts of interest. The authors alone are responsible for the content and writing of this article.
References
- Bijl E, van Valenberg HF, Huppertz T, van Hooijdonk ACM. 2013. Protein, casein, and micellar salts in milk: current content and historical perspectives. J Dairy Sci. 96:5455–5464.
- Cerbulis J, Farrell HM Jr. 1976. Composition of the milks of dairy cattle. II. Ash, calcium, magnesium, and phosphorus. J Dairy Sci. 59:589–593.
- Cerny BA, Kaiser HF. 1977. A study of a measure of sampling adequacy for factor-analytic correlation matrices. Multivariate Behav Res. 12:43–47.
- De Marchi M, Dal Zotto R, Cassandro M, Bittante G. 2007. Milk coagulation ability of five dairy cattle breeds. J Dairy Sci. 90:3986–3992.
- Duclos D, Hiemstra SJ. 2010. State of local cattle breeds in Europe. In: Hiemstra SJ, De Haas Y, Gandini MG, editors. Local cattle breeds in Europe. EU GENRES 870/04 project EURECA. Wageningen, the Netherlands: Academic Publishers; p. 40–57.
- FAO. 2000. World watch list for domestic animal diversity. 3rd ed. Roma, Italy.
- FAO. 2013. Milk and dairy products in human nutrition. Roma, Italy.
- Gallo A, Moschini M, Cerioli C, Masoero F. 2013. Use of principal component analysis to classify forages and predict their calculated energy content. Animal. 7:930–939.
- Gandini G, Avon L, Bohte-Wilhelmus D, Bay E, Colinet FG, Choroszy Z, Díaz C, Duclos D, Fernández J, Gengler N, et al. The EURECA Consortium. 2010. Motives and values in farming local cattle breeds in Europe: a survey on 15 breeds. Animal Genet Resour. 47:45–58.
- Gao X, Valley ML, Tucker KL. 2006. Prospective studies of dairy product and calcium intakes and prostate cancer risk: a meta-analysis. J Natl Cancer Instut. 97:1768–1777.
- Gaucheron F. 2005. The minerals of milk. Reprod. Nutr. Dev 45:473–483.
- Hanuš O, Frelich J, Tomáška M, Vyletělová M, Genčurová V, Kučera J, Třináctý J. 2010. The analysis of relationships between chemical composition, physical, technological and health indicators and freezing point in raw cow milk. Czech J Anim Sci. 55:11–29.
- Holt C, Carver JA, Ecroyd H, Thorn DC. 2013. Invited review: caseins and the casein micelle: their biological functions, structures, and behavior in foods. J Dairy Sci. 96:6127–6146.
- Hortvet J. 1921. The cryoscopy of milk. Ind Eng Chem. 13:198–208.
- Kędzierska-Matysek M, Litwińczuk Z, Florek M, Barłowska J. 2011. The effects of breed and other factors on the composition and freezing point of cow’s milk in Poland. Int J Dairy Technol. 64:336–342.
- Klop G, Ellis JL, Blok MC, Brandsma GG, Bannink A, Dijkstra J. 2014. Variation in phosphorus content of milk from dairy cattle as affected by differences in milk composition. J Agric Sci (Cambridge). 152:860–869.
- Macciotta NPP, Cecchinato A, Mele M, Bittante G. 2012. Use of multivariate factor analysis to define new indicator variables for milk composition and coagulation properties in Brown Swiss cows. J Dairy Sci. 95:7346–7654.
- Malacarne M, Franceschi P, Formaggioni P, Pisani GM, Petrera F, Abeni F, Soffiantini CS, Summer A. 2015. Minerals content and distribution in milk from red deer (Cervus elaphus), fallow deer (Dama dama) and roe deer (Capreolus capreolus). Small Ruminant Res. 130:208–215.
- Malacarne M, Franceschi P, Formaggioni P, Sandri S, Mariani P, Summer A. 2014. Influence of micellar calcium and phosphorus on rennet coagulation properties of cows milk. J Dairy Res. 81:129–136.
- Malacarne M, Summer A, Formaggioni P, Franceschi P, Sandri S, Pecorari M, Vecchia P, Mariani P. 2008. Dairy maturation of milk used in the manufacture of Parmigiano-Reggiano cheese: effects on physico-chemical characteristics, rennet-coagulation aptitude and rheological properties. J Dairy Res. 75:218–224.
- Malacarne M, Summer A, Fossa E, Formaggioni P, Franceschi P, Pecorari M, Mariani P. 2006. Composition, coagulation properties and Parmigiano-Reggiano cheese yield of Italian Brown and Italian Friesian herd milks. J Dairy Res. 73:171–177.
- Mariani P. 1985. Observations on content and distribution of micellar constituents of milk in four cattle breeds. Ann Fac Vet Med Univ Parma. 5:173–183.
- Martin-Collado D, Gandini G, de Haas Y, Díaz C. 2010. Decision-making tools for the development of breed strategies. In: Hiemstra SJ, De Haas Y, Gandini MG, editors. Local cattle breeds in Europe. EU GENRES 870/04 project EURECA. Wageningen, the Netherlands: Academic Publishers; p. 120–140.
- Möller NP, Scholz-Ahrens KE, Roos N, Schrezenmeier J. 2008. Bioactive peptides and proteins from foods: indication for health effects. Eur J Nutrition. 47:171–182.
- Neville MC, Peaker MO. 1979. The secretion of calcium and phosphorus into milk. J Physiol. (Lond). 290:59–67.
- Pecorari M, Sandri S, Mariani P. 1987. Milk coagulation aptitude of Friesian, Brown, Reggiana and Modenese cattle breeds. Scienza Tecnica Lattiero-Casearia. 38:376–384.
- Petrera F, Napolitano F, Dal Prà A, Abeni F. 2015. Plasma parameters related to energy and lipid metabolism in periparturient Modenese and Italian Friesian cows. J Anim Physiol Anim Nutrition. 99:962–973.
- Pirilä S, Taskinen M, Viljakainen H, Kajosaari M, Turanlahti M, Saarinen-Pihkala UM, Mäkitie O. 2011. Infant milk feeding influences adult bone health: a prospective study from birth to 32 years. PLoS One. 6:e19068.
- Ramalho HMM, Santos J, Casal S, Alves MR, Oliveira MBPP. 2012. Fat-soluble vitamin (A, D, E, and β-carotene) contents from a Portuguese autochthonous cow breed-Minhota. J Dairy Sci. 95:5476–5484.
- Summer A, Malacarne M, Martuzzi F, Mariani P. 2002. Structural and functional characteristics of Modenese cow milk in Parmigiano-Reggiano cheese production. Paper presented at: Proceedings of the 53rd Annual Meeting of the European Association for Animal Production; Cairo, Egypt.
- Van Hulzen KJE, Sprong RC, van der Meer R, van Arendonk JAM. 2009. Genetic and nongenetic variation in concentration of selenium, calcium, potassium, zinc, magnesium, and phosphorus in milk of Dutch Holstein-Friesian cows. J Dairy Sci. 92:5754–5759.
- Walsh S, Buckley F, Pierce KN, Patton Byrne J, Dillon P. 2008. Effects of breed and feeding system on milk production, body weight, body condition score, reproductive performance, and postpartum ovarian function. J Dairy Sci. 91:4401–4413.
- White JCD, Davies DT. 1958. The relation between the chemical composition of milk and the stability of caseinate complex. I. General introduction, description samples, methods and chemical composition of samples. J Dairy Sci. 25:236–255.