Abstract
This study evaluated the effects of 10% solid-state fermentation (SSF) wheat bran (WB) by Bacillus amyloliquefaciens (BA) and Saccharomyces cerevisiae (SC) in broiler diets, on growth performance, intestinal morphology, and intestinal microbiota and serum characteristics of broiler chickens. The results showed that fermented wheat bran with BA or BA and SC reduced NDF and ADF contents in WB material. A total of 240 male broilers (Ross 308) were randomly allocated into four feeding groups: (1). Basal diet with 10% WB (control treatment). (2). Basal diet that replaced WB with fermented WB by BA (FWBA). (3). Fermented WB by SC (FWSC), and (4). Fermented WB by BA and SC (FWBA + SC), each group was fed these particular diets for 35 d. Results demonstrated that replacement of WB with FWBA and FWBA + SC improved feed conversion ratio (d 1 to d 35) compared to the control group. Moreover, the 10% FWBA group significantly enhanced the lactic acid bacteria count in the ileum of 35 d old broilers. Compared to the control group, the 10% FWBA and 10% FWSC groups, showed significantly increased ileal lactic acid levels, and in the 10% FWSC group, the ileum villus height was significantly increased in 35 d old broilers. However, treatments with 10% FWBA and 10% FWBA + SC had a tendency to reduce serum cholesterol. In conclusion, a 10% FWBA replacement in the diet could ameliorate health status in broilers by improving growth performance, modulating intestinal microbiota and increasing lactic acid in the ileum.
Introduction
Techniques including direct-fed microbials (DFM) and microbial fermentation have been applied to improve growth performance and enhance the health status of poultry. The microorganisms, including Lactobacillus, Bacillus and Saccharomyces cerevisiae (SC), have been studied as potential feed additives due to their outstanding production of extracellular enzymes including protease, amylase, cellulase and lipase (Chen et al. Citation2009; Salim et al. Citation2013). These enzymes may increase the nutrient digestibility of proteins, carbohydrates and lipids in broilers (Salim et al. Citation2013). Bacillus amyloliquefaciens (BA), a member of the genus Bacillus, is a spore-producing gram-positive bacteria. BA can improve the growth performance and modulate the intestine microbiota of broilers (Ahmed et al. Citation2014). SC, one of the most widely commercialised types of yeast, has long been fed to animals, and it has a beneficial effect on growth, modulation of intestinal microbiota and pathogen inhibition in broilers (Mountzouris et al. Citation2015). In contrast, there were reports that showed that body weight gain (BWG) was not affected by the addition of dietary DFM in broiler diets (Lee et al. Citation2010). Because of the unstable effects of supplemented DFM in broiler diets, microbial fermentation of by-products may be a substituted way to enhance the benefit of microbial and improve nutrient availability of by-products (Chen et al. Citation2009).
Recent studies have reported using fungal inoculums for the fermentation of agricultural by-products in order to enhance their nutritional value (Sharma & Arora Citation2010). Solid-state fermentation (SSF) is the growth of organisms on moist substrates in the absence of free-flowing water. The use of SSF for the production of enzymes and other products has many advantages over submerged fermentation. A very important advantage is that it permits the use of agricultural and agro-industrial residues as substrates which are then converted into products with high-commercial values like secondary metabolites (Reda et al. Citation2014). A large number of fungal species were known to grow well on moist substrates in the absence of free-flowing water. As a result, most studies involving SSF have been conducted using fungi. However, there are few reports of bacterial strains or mixtures of fungi and bacteria being used successfully for the improvement of by-product utilisation by using SSF in broilers.
Wheat bran (WB), as the main by-product of wheat flour processing, contains a high amount of total dietary fibre (451 g/kg), and other relevant compounds including protein (160 g/kg), fat (47 g/kg), available carbohydrates (177 g/kg) and minerals (61.5 g/kg) (Souci & Kirchhoff Citation2008). However, it is well known that increasing fibre content negatively correlates with animal feed intake, and affects growth performance. Therefore the use of wheat bran was limited in animal feeding. It is hypothesised that SSF may degrade fibres and eliminate the disadvantage of wheat bran in broiler diets.
The previous studies generally evaluated the effects of ‘fermented complete feed’ on animals (Chen et al. Citation2009), but once composition of complete diets changes, fermenting condition should alter as well. Hence, different formulations of complete diets among animals and feeding periods limited the application of feed fermentation. However, by fermenting a simple feed ingredient, like wheat bran, it could be formulated in different diets which remove the practical limitation from feed fermentation. There are few reports that have focussed on the use of fermented wheat bran in broiler diets. Therefore, this study is intended to demonstrate the effect of the replacement of 10% wheat bran in basal diets by solid-state fermented wheat bran with BA and SC on the growth performance, intestinal microbiota, serum characteristics and intestinal morphology in broiler chickens.
Materials and methods
Solid-state fermentation (SSF)
Wheat bran was mixed with 60% distilled water, and added to a 10% broth culture of BA, 10% broth culture of SC, and a mixture solution of with a 5% broth culture of BA and a 5% broth culture of SC, respectively. The BA and SC used in this study were screened and selected by our laboratory (Teng et al. Citation2017). The broth cultures were prepared by inoculating BA in Lysogeny broth (LB) and SC in Yeast-Mald (YM) at 37 °C for 24 h and the colony-forming units was verified achieving 1 × 107 CFU/mL by plating serial 10-fold dilutions in duplicate into LB agar plates and YM agar plates. After fermentation at 30 °C for 3 d, the fermented wheat brans were dried at 40 °C for 4 d and mashed to formulate broiler diets.
Measurement of total dietary fibre
Total dietary fibre was evaluated using the Megazyme kit (K-TDFR 200A, Megazyme Inc., Chicago, IL). One gram sample was mixed with 50 mL phosphate buffer (pH 6.0), and samples were digested by 50 μL thermostable α-amylase in boiling water bath for 15 min, by 100 μL protease at 60 °C for 30 min, and by 200 μL amyloglucosidase at 60 °C for 30 min, sequentially. After enzyme digestions, soluble dietary fibre was precipitated with 76% (v/v) ethanol. Dietary fibre residues were isolated by filtration through fritted crucibles and corrected by analysing ash and protein contents in the residues.
Radial enzyme diffusion assay
The enzyme activities of were assayed by radial enzyme diffusion method described by Walsh et al. (Citation2005). After fermentation, 5 g fermented samples were mixed with 45 mL RO water to extract crud enzyme in ice bath for 10 min. Crude enzyme extractions were loaded to agar plates containing 1% casein, 0.3% xylan, and 0.1% carboxymethyl-cellulose for protease, xylanase, and cellulase radial diffusions, respectively. RO water was used as negative control group and commercial enzymes were used as positive control groups.
Feeding schedule and dietary composition
The experiment lasted for 35 d during which there were 2 phases: Starter (1 to 21 d) and finisher (22 to 35 d). The diets (in mash form) and water were provided ad libitum. The birds in the control group were fed a diet based on maize-soybean with 10% WB, and the other three groups were provided experimental diets based on the control group’s diet but the WB was replaced with fermented WB by BA (FWBA), with fermented WB by SC (FWSC) and fermented WB by BA and SC (FWBA + SC). Starter and finisher diets were provided to the birds from 1 to 21 d and from 22 to 35 d of age, respectively (). During the entire experimental period (35 d), the diets were formulated to meet the requirements suggested by the Ross Broiler Management Manual (2009). The proximate composition was analysed according to the AOAC (Citation1980) showed no major deviations from the calculated values. The feed mixtures contained no anticoccidial or antibacterial supplements. The method of NDF determination was following the procedure described by Van Soest et al. (Citation1991).
Table 1. Ingredients and chemical composition of the experimental diets for broilers.
Experimental birds and housing
This study was conducted at National Chung Hsing University, Taiwan. The experimental protocol for animal use was approved by the Animal Care and Use Committee. A total of two hundred and forty male broilers (Ross 308) were randomly allocated to four treatments, each of which had three replicate pens per treatment and 20 birds per pen (total of 60 birds per treatment). Initial average body weight (BW) of the birds were similar among different pens (average 46.0 to 46.5 g/bird approximately). All of the birds were raised in the pens (2 *4 m2) with rice husk as litter and housed in a temperature-controlled environment. The temperature was maintained at 33 ± 1 °C until the birds reached 7 d of age, and was gradually decreased to 27 ± 1 °C until the birds reached 21 d of age; after this point, the broilers were maintained at 27 ± 1 °C.
Performance, serum, intestinal content, and faeces collection
The BW of chickens per pen and feed intake was recorded at 1, 21 and 35 d of age. BWG and feed conversion ratio (FCR) were calculated. On Day 35, 12 chickens per treatment (four birds from each pen) close to the average weight were selected for sampling of blood, intestinal content and faeces. Fifty grams of faeces per treatment, excreted over 2h, were collected randomly into three plastic plates twice (six replicates in total) to determine ammonia content. Blood from the brachial vein was collected (5 ml) via cardiac puncture using a vacutainer tube. The blood was centrifuged at 3000 × g for 30 min to obtain the serum, and was stored at −20 °C, then analysed for serum characteristics. After the blood was collected, the birds were euthanized by exsanguination and the ileal and excreta contents were collected in sterile plastic plates for subsequent study.
Ileal microbial populations
One gram of the ileal sample was serially diluted with 9 mL of 0.9% sterile saline (1:10 dilution) and mixed thoroughly. Viable counts of bacteria in the ileal samples were then conducted by plating serial 10-fold dilutions in duplicate into TSC agar (tryptose sulfite cycloserine agar, Difco TM, BD, Franklin Lakes, NJ) plates, and MRS medium (de Man Rogosa and Sharpe agar, Difco 288130, BD, Franklin Lakes, NJ) to isolate the C. perfringens and Lactobacillus spp., respectively. The TSC agar plates and MRS agar plates were then incubated for 48 h at 37 °C under anaerobic conditions. Microbial colonies were immediately counted after removal from the incubator and expressed as log10 colony-forming units (CFU) per gram of ileal contents.
Short chain fatty acid (SCFA) analysis of caecal contents
To determine SCFA levels, including acetate, propionate and butyrate, 1 g of caecal content was mixed with 4mL 25% metaphosphoric acid. Samples were centrifuged at 10,000 × g for 20 min and the supernatant was transferred into a 2 mL tube stored at −20 °C. The concentration of SCFA was determined by gas chromatography with a flame ionisation detector, fuse silica capillary column and nitrogen was used as the carrier gas. Volatile acid standard mixes (SUPELCO, Bellefonte, PA) were used as standard solutions.
Morphometric analysis of the small intestine
At the end of the experiment (day 35), one bird per replicate cage from each treatment group (three birds/treatment in total) was randomly selected and slaughtered by severing the jugular veins. During necropsy, the gastrointestinal tract was removed and a segment of the ileum (from Meckel’s diverticulum to the ileo-caeco-colic junction) was taken from the small intestine. The 3 cm long segments from the centre of each tissue were fixed in 10% formalin for later morphometrical assays. The formalin-fixed gut wall was washed in PBS and embedded in paraffin wax. The 3μm sectioned tissue was stained using haematoxylin and eosin methods. The samples were analysed by light microscopy, and software (Motic image plus 2.0) was used to measure the villus height and crypt depth in fifteen favorably-oriented and representative samples per treatment. The ratio of villus height to crypt depth was also calculated.
Ammonia analysis of ileal, caecal and faecal contents
The assay of ammonia analysis was modified from the methods of Weatherburn (Citation1967). One gram of ileal (three replicates with four birds per replicate in each treatment) and faecal (six replicates in each treatment) sample was mixed with 4 mL 25% metaphosphoric acid and centrifuged at 15,000 × g for 10 min. The 1.5 mL of supernatant was transferred into a 2 mL Eppendolf tube and stored at −20 °C. 25μL of supernatant was mixed with 1 mL reagent A (5 g of phenol with 250 mg of sodium nitroprusside per litre of solution) and 1 mL reagent B (25 g of sodium hydroxide with 16.8 mL sodium hypochlorite per litre of solution). After waiting 15 min for colour development at 37 °C, absorbance was measured at 630 nm. NH4Cl was used as the standard solution.
Lactic acid analysis of ileum and caecal contents
Analysis of lactate was carried out by HPLC (L-4200 UV-VIS Detector, Hitachi, Tokyo, Japan) with Gemini 5 u C6-Phynel 110A 250 × 4.6mm (Phenomenex, Torrance, CL) column. One gram of ileal and caecal sample was mixed with 1 mL de-ionized water and 4mL cyanide methane, and centrifuged at 4000 × g for 4 min. The supernatant was filtered through a 0.2μm filtration film (Sartorius Minisart, NY 25 single-use syringe filter, Sartorius, Göttingen, Germany) and stored at −20 °C. The 10 μL of filtered supernatant was injected into the HPLC to conduct the analysis. The mobile phase was a phosphate buffer (contain 3% methanol) and the rate was set at 1 mL/min. The UV detector wavelength was 220 nm.
Serum characteristic determination
Serum biochemical values, including serum glutamic-oxalocetic transaminase (SGOT), serum glutamic-pyruvic transaminase (SGPT), cholesterol (CHOL), triglyceride (TG), glucose (GLU), creatinine (CREA), uric acid (UA), globulin (GLO), total-protein (T-P) and albumin (ALB), were measured using an automatic biochemical analyser (Hitachi, 7150 auto-analyser, Hitachi, Tokyo, Japan).
Statistical analyses
Data was subjected to ANOVA as a completely randomised design using the GLM function of the SAS software (SAS Institute Citation2004). Determination of significant statistical differences among the mean values of the four treatment groups used Tukey’s honestly significant difference test with a significance level of p < .05.
Results
Approximately analysis of solid-state fermented wheat bran
presents the approximate analysis of solid-state fermentation wheat bran by BA and SC. The results demonstrated that the NDF and ADF levels decreased after solid-state fermentation by BA or BA and SC (NDF: from 38.8% to 18.0 and 18.7%; ADF: from 12.3% to 8.3 and 8.5%). Total dietary fibre of WB, FWBA, and FWBA + SC were 37.4 ± 0.2%, 31.0 ± 1.1% and 33.1 ± 0.8%, respectively. illustrates that BA are able to secrete great amounts of protease, xylanase, and cellulase after 3-day fermentation of WB.
Table 2. Approximately analysis and total dietary fibre of solid-state fermented wheat bran by Bacillus amyloliquefaciens and Saccharomyces cerevisiae.
Growth performance
The effects of 10% WB replacement by FWBA, FWSC and FWBA + SC in diets on the growth performance of 1 to 35-d old broilers are shown in . From 1 to 21 d, 22 to 35d, and 1 to 35 d of age, there were no significant differences in BW, BWG, FI and FCR among all treatment groups (p > .05). From 1 to 35 d, treatments with 10% FWBA and 10% FWBA + SC had better FCR compared to the control treatment (1.41 vs. 1.38 vs. 1.38, p < .05).
Table 3. Effects of solid-state fermentation by Bacillus amyloliquefaciens and Saccharomyces cerevisiae on growth performance of broilers.
Microbial population in ileum
The effects of those diets that had a 10% WB replacement by FWBA, FWSC and FWBA + SC on microbial population in broiler ileum after 35 d are shown in . The treatments had no significant effect on Clostridium perfringens (p > .05). The treatment with 10% FWBA resulted in significantly higher amounts of lactic acid bacteria in the ileal content compared to the control group (p < .05).
Table 4. Effects of solid-state fermentation of Bacillus amyloliquefaciens and Saccharomyces cerevisiae on ileal microbiota concentration of broilers.
Ammonia in ileum, caecum, and excreta
The effects of those diets that had a 10% WB replacement by FWBA, FWSC and FWBA + SC on ileal, caecal and faecal ammonia concentrations are shown in . None of the treatments had a significant effect on ileal, caecal and faecal ammonia levels (p < .05).
Table 5. Effects of solid-state fermentation of Bacillus amyloliquefaciens and Saccharomyces cerevisiae on intestinal and faecal ammonia concentration of broilers.
Lactic acid in ileum and caecum
The effects of those diets that had a 10% WB replacement by FWBA, FWSC and FWBA + SC on ileal and caecal lactic acid concentrations are shown in . There were no significant differences in caecal lactic acid concentration among all treatment groups (p > .05). However, the treatment with 10% FWBA and 10% FWSC significantly increased the ileal lactic acid level compared to the control groups (p < .0001).
Table 6. Effects of solid-state fermentation of Bacillus amyloliquefaciens and Saccharomyces cerevisiae, on intestinal lactic acid concentration of broilers.
Short-chain fatty acid ileum and caecum
The effects of those diets that had a 10% WB replacement by FWBA, FWSC and FWBA + SC on caecal SCFA are shown in . There were no significant differences in acetic acid, propionic acid or butyric acid among all treatment groups (p > .05).
Table 7. Effects of solid-state fermentation of Bacillus amyloliquefaciens and Saccharomyces cerevisiae on caecal individual SCFA concentration of broilers.
Intestinal morphology
The effects of those diets that had a 10% WB replacement by FWBA, FWSC and FWBA + SC on the ileal morphology of broilers are shown in and . There were no significant differences in crypt depth or villi height/crypt depth ratio in the ileum among all treatment groups (p > .05). However, the 10% FWSC group resulted in a higher ileal villi height compared to the control, 10% FWBA and 10% FWBA + SC groups (p < .05).
Figure 2. Photomicrography of ileum of 35 d broiler in treatments with control, 10% FWBA, 10% FWSC, and 10% FWBA + SC. (A) Control (B) 10% FWBA (C) 10% FWSC (D) 10% FWBA + SC. Hematoxylin and eosin stain (40×) (method according to Huang et al. Citation2012).
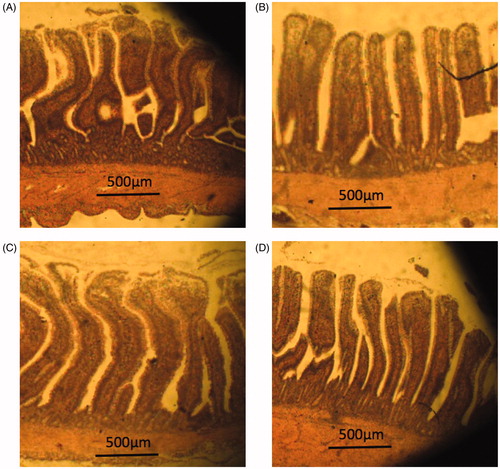
Table 8. Effects of solid-state fermentation of Bacillus amyloliquefaciens and Saccharomyces cerevisiae on ileal morphology of broilers.
Serum characteristics
The effects of those diets that had a 10% WB replacement by FWBA, FWSC and FWBA + SC on serum characteristics are shown in . There were no significant differences in TG, GLU, CREA, UA, GLO, T-P, ALB levels among all treatment groups. However, treatments with 10% FWBA and 10% FWBA + SC had a tendency to reduce serum CHOL compared to the control group (p = .054).
Table 9. Effects of solid-state fermentation of Bacillus amyloliquefaciens and Saccharomyces cerevisiae on serum characteristics of broilers.
Evaluation of fermented wheat bran meal economic benefit
An evaluation of the economic benefits of adding fermented wheat bran meal to the broiler diet is summarised in . The income over feed cost (IOFC) of the control and 10% FSBA + SC groups were 45.3 and 47.0 NT$/bird, respectively.
Table 10. Evaluation of the economic benefit of fermented wheat bran (FWB) supplemented in diet.
Discussion
In the present study, wheat bran fermentation by a mixture of BA and SC can reduce the NDF from 38.8% to 18.7% and ADF from 12.3% to 8.5%, respectively. In the further determination, total dietary fibre contents in wheat bran were decreased 17.1% or 11.5% by fermentation with BA or fermentation with BA and SC. These results can be attributed to outstanding extracellular cellulase and xylananse produced by Bacillus amyloliquefaciens that we found in the present study (). According to the previous study, solid-state fermentation by BA combined with the enzymes production could efficiently degrade non-starch polysaccharides (NSP) to low molecular weight polysaccharides, reducing intestinal viscosity caused by NSP and leading to a higher availability of wheat bran (Castanon et al. Citation1997; Choct Citation2006).
Figure 1. Radial diffusion results of wheat bran added 60% water and 10% BA for 3-day fermentation (A) protease (B) xylanase (C) cellulase.
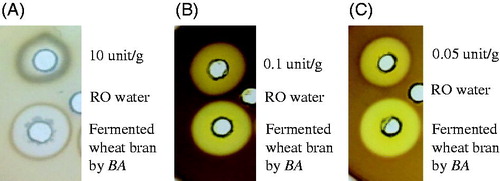
Kraler et al. (Citation2014) pointed out that the use of a 15% solid-stated fermented wheat bran by Lactobacillus paracasei and Lactobacillus plantarum included in basal diet could increase coefficients of total tract apparent digestibility of organic matter (2%), crude fibre (9%), ether extract (40%), and ash (14%) in growing pigs. Decreasing NSP and increasing digestibility may the reason that treatments with 10% FWBA and 10% FWBA + SC improved the FCR of broilers compared to the control group. Nevertheless, Chen et al. (Citation2009) reported fermented total feed by Bacillus and Saccharomyces had no significant difference on the FCR of broilers. The inconsistent results made clear that whole feed fermentation did not improve nutrient availability while 10% fermentation of wheat bran increased it in our study. Wheat bran contained higher fibre content which induces microorganisms secreting plant cell wall-degrading enzymes. These enzymes degrade fibre content which limits nutritional digestibility in gastrointestinal tract. Hence, the wheat bran fermentation improves feed availability and results in better growth performance. On the other hand, total feed is made up of high levels of protein and starch which can be easily utilised by microorganisms. Fibre contents in fermented total feed will not be degraded as much as fermented wheat bran. It might be the reason that fermented total feed by BA and SC had no significant influence on FCR. Furthermore, a replacement of 10% of fermented wheat bran in diets could not only reduce the energy required for fermentation, but also reduce feeding expenses while using 10% by-product in broiler diets.
Complex carbohydrates are poorly metabolised by Lactobacillus (Dworkin et al. Citation2006). However, if complex carbohydrates degraded to low-molecular weight carbohydrates by solid-state fermentation, Lactobacillus could ferment these low-molecular weight carbohydrates to lactic acid (Chen et al. Citation2013). Therefore, in the present study, we could find out that FWBA group increased Lactobacillus and lactic acid in ileum of broilers. Similarly, Chen et al. (Citation2013) also showed that the Lactobacillus count in the small intestine was higher in fermented feed treatment than in the control treatment in Landes geese. Lactobacillus has been reported to produce a variety of antimicrobial substances such as lactic acid, hydrogen peroxide, reuterin and reutericyclin. These substances can inhibit the growth of many enteric pathogens such as Escherichia coli, Salmonella typhimurium and Staphylococcus epidermidis (Hou et al. Citation2015).
In the present study, we found that feeding fermented wheat bran did not produce significant differences in caecal microbiota, individual volatile fatty acid and lactic acid content. Kraler et al. (Citation2015) showed that the fermented wheat bran by L. paracasei and L. plantarum had no effects on acetic acid, propionic acid, and butyric acid in the colonic guts of pigs, which is in agreement with our findings. However, Ahmed et al. (Citation2014) found that the supplementation of 2% of Bacillus probiotic powder in broiler diets might enhance nutrient absorption and inhibit pathogenic bacteria that led to a decrease in non-utilised nutrients, which would have been degraded to ammonia by microorganisms in the faeces. In the current study, the counts of Bacillus in the 10% FWBA treatment might not be sufficient to inhibit pathogenic bacteria in intestinal guts and decrease the concentration of ammonia.
Intestinal morphology plays an important role in nutrient absorption. Longer and wider villus measurements indicate a greater intestinal surface area which may lead to higher feed availability. The crypt is known as the villus factory, and deeper crypts suggest faster cell turnover, producing new epithelial cells which migrate along to the top of the villus, compensating for inflammation or sloughing cells damaged by pathogens or bacterial toxins (Yason et al. Citation1987). We found that the treatment with 10% FWSC could significantly increase ileal villus height and it may be attributed to Saccharomyces cerevisiae. A previous study showed that supplementation of whole yeast or yeast walls could improve intestinal morphology (Muthusamy et al. Citation2011). The cell walls of Saccharomyces cerevisiae, which account for 15 to 25% weight of the cell, are predominantly composed of glucan and mannoproteins, including mannan-oligosaccharide and β-glucan (Lipke & Ovalle Citation1998). These compounds might avoid pathogenic bacteria binding to the villus and protect mucosa from toxic materials. Therefore, the 10% FWSC group had higher villus height in the ileum of broilers compared to other treatments (Zhang et al. Citation2005).
In the current study, the results showed that treatments with 10% FWBA, 10% FWSC, 10% FWBA + SC had a tendency to reduce serum cholesterol compared to the control treatment (p = .054). Pereira and Gibson (Citation2002) reported that the cholesterol in animal guts could be taken into the cellular membrane of Lactobacillus. It may follow that wheat bran fermented by Bacillus increased intestinal Lactobacillus to assimilate cholesterol which might reduce serum cholesterol in broilers. On the other hand, a previous study showed that Saccharomyces cerevisiae was able to remove cholesterol in animal guts (Psomas et al. Citation2003). Paryad and Mahmoudi (Citation2008) also reported that supplementing 1.5% Saccharomyces cerevisiae in diets could reduce the serum cholesterol of broilers, which is similar to our results.
Conclusions
Solid-state fermented wheat bran with BA degraded NDF, ADF, and total dietary fibre, leading to lower FCR in 10% FWBA and 10% FWBA + SC treatments. The 10% FWBA group also increased Lactobacillus and lactic acid in the ileum, while the 10% FWSC group had a greater ileal villus height. In addition, all of the fermented wheat bran treatments had a tendency to decrease the serum cholesterol of broilers compared to the control group.
Disclosure statement
The authors report no conflicts of interest. The authors alone are responsible for the content and writing of this article.
Additional information
Funding
References
- Ahmed ST, Islam M, Mun HS, Sim HJ, Kim YJ, Yang CJ. 2014. Effects of Bacillus amyloliquefaciens as a probiotic strain on growth performance, cecal microflora, and fecal noxious gas emissions of broiler chickens. Poult Sci. 93:1963–1971.
- AOAC (Horwitz W) 1980. Official methods of analysis. Association of Official Analytical Chemists, 13th ed. Washington (DC), USA.
- Castanon JIR, Flores MP, Pettersson D. 1997. Mode of degradation of non-starch polysaccharides by feed enzyme preparations. Anim Feed Sci Technol. 68:361–365.
- Chen KL, Kho WL, You SH, Yeh RH, Tang SW, Hsieh CW. 2009. Effects of Bacillus subtilis var. natto and Saccharomyces cerevisiae mixed fermented feed on the enhanced growth performance of broilers. Poult Sci. 88:309–315.
- Chen W, Zhu XZ, Wang JP, Wang ZX, Huang YQ. 2013. Effects of Bacillus subtilis var. natto and Saccharomyces cerevisiae fermented liquid feed on growth performance, relative organ weight, intestinal microflora, and organ antioxidant status in Landes geese. J Anim Sci. 91:978–985.
- Choct M. 2006. Enzymes for the feed industry: past, present and future. Worlds Poult Sci J. 62:5–16.
- Dworkin M, Falkow S, Schleifer KH, Rosenberg E, Stackebrandt E. 2006. The genera Lactobacillus and Carnobacterium. The prokaryotes. 3rd ed. Vol. 4. New York (NY): Springer-Verlag; p. 320–403.
- Hou C, Zeng X, Yang F, Liu H, Qiao S. 2015. Study and use of the probiotic Lactobacillus reuteri in pigs: a review. J Anim Sci Biotechnol. 6:14.
- Huang CW, Lee TT, Shih YC, Yu B. 2012. Effects of dietary supplementation of Chinese medicinal herbs on polymorphonuclear neutrophil immune activity and small intestinal morphology in weanling pigs. J Anim Physiol Anim Nutr. 96:285–294.
- Kraler M, Schedle K, Domig KJ, Heine D, Michlmayr H, Kneifel W. 2014. Effects of fermented and extruded wheat bran on total tract apparent digestibility of nutrients, minerals and energy in growing pigs. Anim Feed Sci Technol. 197:121–129.
- Kraler M, Schedle K, Schwarz C, Domig KJ, Pichler M, Oppeneder A, Wetscherek W, Prückler M, Pignitter M, Pirker KF, et al. 2015. Fermented and extruded wheat bran in piglet diets: impact on performance, intestinal morphology, microbial metabolites in chyme and blood lipid radicals. Arch Anim Nutr. 69:378–398.
- Lee KW, Lee SH, Lillehoj HS, Li GX, Jang SI, Babu US, Park MS, Kim DK, Lillehoj EP, Neumann AP, et al. 2010. Effects of direct-fed microbials on growth performance, gut morphometry, and immune characteristics in broiler chickens. Poult Sci. 89:203–216.
- Lipke PN, Ovalle R. 1998. Cell wall architecture in yeast: new structure and new challenges. J Bacteriol. 180:3735–3740.
- Mountzouris KC, Dalaka E, Palamidi I, Paraskeuas V, Demey V, Theodoropoulos G, Fegeros K. 2015. Evaluation of yeast dietary supplementation in broilers challenged or not with Salmonella on growth performance, cecal microbiota composition and Salmonella in ceca, cloacae and carcass skin. Poult Sci. 94:2445–2455.
- Muthusamy N, Haldar S, Ghosh TK, Bedford MR. 2011. Effects of hydrolysed Saccharomyces cerevisiae yeast and yeast cell wall components on live performance, intestinal histo-morphology and humoral immune response of broilers. Br Poult Sci. 52:694–703.
- Paryad A, Mahmoudi M. 2008. Effect of different levels of supplemental yeast (Saccharomyces cerevisiae) on performance, blood constituents and carcass characteristics of broiler chicks. Afri J Agri Res. 3:835–842.
- Pereira DIA, Gibson GR. 2002. Cholesterol assimilation by lactic acid bacteria and Bifidobacteria isolated from the human gut. Appl Environ Microbiol. 68:4689–4693.
- Psomas EI, Fletouris DJ, Litopoulou-Tzanetaki E, Tzanetakis N. 2003. Assimilation of cholesterol by yeast strains isolated from infant feces and feta cheese. J Dairy Sci. 86:3416–3422.
- Reda ME, Mohamed SA, Asiri AM, Gomaa AM, Ibrahim IH, Hasan AA. 2014. Solid fermentation of wheat bran for hydrolytic enzymes production and saccharification content by a local isolate Bacillus megatherium. BMC Biotech. 14:29.
- Salim HM, Kang HK, Akter N, Kim DW, Kim JH, Kim M, Na JC, Jong HB, Choi HC, Suh OS, et al. 2013. Supplementation of direct-fed microbials as an alternative to antibiotic on growth performance, immune response, cecal microbial population, and ileal morphology of broiler chickens. Poult Sci. 92:2084–2090.
- SAS Institute. 2004. SAS/STAT Guide for Personal Computers. Version 9.0.1. Cary (NC): SAS Inst. Inc.
- Sharma RK, Arora DS. 2010. Production of lignocellulolytic enzymes and enhancement of in vitro digestibility during solid state fermentation of wheat straw by Phlebia floridensis. Bioresour Technol. 101:9248–9253.
- Souci SW, Kirchhoff E. 2008. Food composition and nutrition tables. 7th ed. Stuttgart Germany: Medpharm Scientific Publ.
- Teng PY, Chung CH, Chao YP, Chiang CJ, Chang SC, Yu B, Lee TT. 2017. Administration of Bacillus Amyloliquefaciens and Saccharomyces Cerevisiae as direct-fed microbials improves intestinal microflora and morphology in broiler chickens. J Poult Sci. 54:134–141.
- Van Soest PJ, Robertson JB, Lewis BA. 1991. Methods for dietary fiber, neutral detergent fiber, and nonstarch polysaccharides in relation to animal nutrition. J Dairy Sci. 74:3583–3597.
- Walsh G, Murphy RA, Killeen GF, Power RF. 2005. Quantification of supplemental enzymes in animal feedingstuffs by radial enzyme diffusion. Appl Microbiol Biotechnol. 67:70–74.
- Weatherburn MW. 1967. Phenol-hypochlorite reaction for determination of ammonia. Anal Chem. 39:971–974.
- Yason CV, Summers BA, Schat KA. 1987. Pathogenesis of rotavirus infection in various age groups of chickens and turkeys: pathology. Am J Vet Res. 6:927–938.
- Zhang AW, Lee BD, Lee SK, Lee KW, An GH, Song KB, Lee CH. 2005. Effects of yeast (Saccharomyces cerevisiae) cell components on growth performance, meat quality, and ileal mucosa development of broiler chicks. Poult Sci. 84:1015–1021.