Abstract
Overt differences exist between Chinese local pigs and exotic pig breeds, especially in muscle growth rate and meat quality. However, the underlying molecular mechanisms remain unclear. This study aimed to assess muscle fibre types and metabolic enzymes in Bama miniature pigs and Landrace swine. Meat quality traits, including intramuscular fat content, and muscle colour, conductivity, and tenderness, were assessed in these pig breeds. Then, muscle fibre types were classified, and mRNA amounts and activities of lactate dehydrogenase (LDH), succinate dehydrogenase (SDH), and malate dehydrogenase (MDH) assessed, in M. longissimus from the two pig breeds, at various ages. Our data showed significantly higher back fat thickness, muscle conductivity, and intramuscular fat content in samples from Bama miniature pigs compared with the values obtained for Landrace pigs (p < .05). In addition, SDH activity was significantly higher, and LDH activity overtly lower in Bama pigs compared with Landrace swine (p < .05). Furthermore, myosin heavy-chain (MyHC) II A, II B, and II X mRNA levels in Bama miniature pigs at 180 were significantly higher than values obtained for Landrace pigs of the same age. Although MyHC I gene expression levels were similar in Bama miniature and Landrace pigs at 180 days of age, significantly higher amounts were obtained in 300 day old Bama miniature pigs compared with 180 day old Landrace pigs (p < .05). Collectively, these preliminary findings indicated that skeletal muscles from Bama miniature pigs may contain more oxidative fibres compared with those from Landrace pigs, which might explain the meat quality differences between the two pig breeds.
Introduction
The muscle is composed of fibres, intramuscular fat cells, connective tissue, blood vessels, and nerves. Fibres account for 75–90% of muscle tissue, and are important in determining muscle characteristics (Lee et al. Citation2010). A myosin molecule, the main contractile protein found in skeletal muscles, comprises two myosin heavy chain (MyHC) molecules and two pairs of myosin light chain with regulatory functions. Eight myosin isomers have been described in mammalian skeletal and cardiac muscles, with only four types, including I, II A, II B, and II X, identified in adult mammalian skeletal muscles (Chikuni et al. Citation2001; Lefaucheur et al. Citation2002). MyHC has become an important molecular marker in assessing the classification and composition of skeletal muscle fibre types. Previous findings have suggested that various types of muscle fibres greatly differ in their biochemical characteristics, which not only determine the growth and development of skeletal muscles, but also influence physiological characteristics, sensory quality, and meat quality (Pette & Staron Citation2001). Type I muscle fibres, also called slow oxidative muscle fibres, have the highest cytochrome c and myoglobin levels among the four muscle fibre types; in addition, they contain multiple enzymes related to aerobic metabolism such as malate dehydrogenase and succinate dehydrogenase. Type I muscle fibres mainly rely on aerobic metabolism for energy, with slow and long-lasting contractions (Lind & Kernell Citation1991). Type II B muscle fibres, the fast glycolytic type, mainly depend on the glycolytic pathway for energy; they contain large amounts of ATPase and glycolytic enzymes, including lactate dehydrogenase. Indeed, Type II B muscle fibres contract quickly and powerfully, but are rapidly exhausted (Hamalainen & Pette Citation1993). Type II A is a fast-twitch oxido-glycolytic type of muscle fibres, which contains myoglobin and glycogen, and receives energy from aerobic metabolism and glycolysis; II X is an intermediate type between II A and II B (Klosowska & Fiedler Citation2003). The total number of muscle fibres is established before birth in most mammals, and muscle development after birth mainly depends on diameter enlargement and transformation between muscle fibre types (Bee Citation2007).
Swine breeds are different in muscle characteristics, with obvious differences in pork quality. However, the molecular mechanisms involved in the regulation of muscle characteristics in different breeds remain unclear. Skeletal muscle fibre types and the activities of muscle-related enzymes play an important role in pork quality (Chang et al. Citation2003). Guangxi Bama miniature pigs of Southern China originated from Bama Town, Bama County in Guangxi, whose special natural environment combined with long term close inbreeding has yielded this unique breed. Bama miniature pigs are distinctive for small body size, thin skin, red muscle, white fat, and delicious taste (Wang et al. Citation2010). Also, the growth of Bama miniature pigs slows down after 112 days of age, which indicates characteristics of early-maturing, slow growth, and stable weight gain. Landrace pigs originated from Denmark, and are famous lean pigs obtained through long-term breeding; they have fast growth rate and high-lean percentage, but not always satisfactory meat quality. The molecular mechanisms underlying the overt differences between the two breeds remain largely unknown. We hypothesised that meat quality differences between these breeds may lie on distinct properties of muscle fibres. Therefore, this study aimed to assess muscle fibre types and metabolic enzymes in Bama miniature pigs and Landrace swine, using animals of various ages. Our data showed that skeletal muscles in Bama miniature pigs contain more oxidative fibres compared with those of Landrace pigs, which might explain the meat quality differences between these pig breeds.
Materials and methods
Animals
Healthy Bama miniature pigs (n = 18) and Landrace pigs (n = 15) of approximately the same age were purchased from Bama miniature pig breeding centre of Guangxi University. Bama miniature and Landrace pigs were fed according to Guangxi Local Standard DB45/T546-2008 for ‘Experimental animals-miniature pig’ and the American National Research Council’s Nutrient Requirements of Swine, respectively. The study protocol was approved by the Animal Use and Care Committee of Guangxi University (Permit Number: SCXK Gui 20130003). Bama miniature pigs at the ages of 0, 30, 60, 90, 180 and 300 days (n = 3), and Landrace pigs at the ages of 0, 30, 60, 90 180 days (n = 3) were slaughtered. After sacrifice, M. longissimus tissues were isolated within 10 min, washed with saline, snap frozen in liquid nitrogen, and stored at −80 °C.
OPTO-STAR colour measurement
Within 2 h of slaughter, M. longissimus samples parallel to muscle fibres at the 1–2 lumbar spine were collected and sliced into 1.5 × 1 × 1 cm3 pieces. The colour of fresh muscle sections was measured on an OPTO-STAR colour measurement instrument (MATTHAUS, Germany), with luminosity index (L*; Commission Internationale de L’Eclairage) values (Wu et al. Citation2015) obtained in 2–3 s according to the manufacturer’s instructions. A total of 10 points for each pig were assessed, with average values used as final results.
Conductivity measurements
M. longissimus between the tenth and last ribs was selected for conductivity measurements. Two stainless steel electrodes (0.5 cm diameter, separated by 1 cm) of an LF-STAR conductivity metre (MATTHAUS, Germany) were inserted in M. longissimus about 5 mm for reading. A total of 10 points for each pig were measured, with averaged values used as final results (Chang et al. Citation2003), and expressed in μSiemens/cm (μS/cm).
Muscle tenderness evaluation
Muscle tenderness was evaluated using M. longissimus perpendicular to muscle fibres at 1–2 lumbar vertebra. Briefly, muscle samples were cut using a standard muscle shear apparatus, and tenderness was measured with a C-LM3 digital muscle tenderness instrument (Engineering College of Northeast Agricultural University, China) (Han et al. Citation2009). Shear forces of 6–7 samples (cross-section sizes, 1 cm ×1 cm; depth, 1.5 cm) from each pig were obtained, and average values considered final results, with Newton (N) as the unit. The shear force is inversely proportional to muscle tenderness.
Intramuscular fat measurement
M. longissimus at the thoracic junction was selected to determine intramuscular fat content by Soxhlet petroleum-ether (boiling point, 35–60 °C) extraction (Lefaucheur et al. Citation2002). Briefly, weighed muscle samples were extracted with petroleum ether overnight. After drying for 20 h, a final weight was measured and expressed relatively to the fresh wet tissue weight. Total lipid percentage was derived as (lipid weight/fresh wet tissue weight) × 100.
Loin muscle area measurement
The cross-sectional area at the tenth rib was selected as the loin muscle area using a planimeter as suggested previously (Kim et al. Citation2008).
Back fat thickness measurement
Back fat thickness was measured above the dorsal muscle, 4–5 cm to the dorsal midline, at the first and last ribs, as well as lumbar levels; the values were averaged to obtain back fat thickness as previously described (Kim et al. Citation2008).
Enzyme activity measurements
M. longissimus tissue (300–500 mg) was placed into a 5 mL sterile centrifuge tube and weighed. Then, pre-cooled physiological saline was added to the sample at a ratio of 1:9 (mass/volume). The muscle tissue was cut into pieces using ophthalmic scissors, and minced fully with a handheld electric homogeniser on ice. The resulting homogenate was centrifuged at 2500 rpm for 10 min at 4 °C, and the supernatant collected for measurements. Protein content in the supernatant was measured by the Bradford method. Enzyme activities were assessed using commercially available kits (Nanjing Jiancheng Biological Engineering Institute) for lactate dehydrogenase (LDH) (Hu et al. Citation2010), succinate dehydrogenase (SDH) (Zhang & Lin Citation2009) and malate dehydrogenase (MDH) (Zheng et al. Citation2015). Briefly, LDH activity was assessed after 15 min reaction with the substrate at 37 °C, and expressed in units (moles of pyruvate produced) per gram of tissue protein (U/gprot). MDH activity was assessed after 1 min reaction, and expressed in units (moles of substrate transformed) per milligram of tissue protein (U/mgprot). SDH activity was assessed after 1 min reaction, and expressed in units (a 0.01 absorbance decrease represented 1 specific activity unit) per milligram protein (U/mgprot).
Real time RT-PCR (qRT-PCR)
Total RNA from M. longissimus tissue samples from Bama miniature and Landrace pigs of different ages was extracted with RNA Extraction Kit (Invitrogen, Carlsbad, CA), and quantified on a Q5000 ultra micro spectrophotometer (Quawell, San Jose, CA). OD260/280 ratios of all RNA samples were between 1.8 and 2.0, with concentrations over 500 ng/L. First strand cDNA was prepared from 2μg RNA using PrimeScriptTM RT reagent Kit with gDNA Eraser from Takara Biotechnology Co. Ltd. (Dalian, China).
Four MyHC isoforms (I, II A, II B, and II X) were analysed for mRNA expression by real-time quantitative reverse transcription PCR (qRT-PCR). 18S rRNA was used as an internal control, and primers were designed according to NCBI sequences. Specific primers were synthesised by Life Technologies (Shanghai, China), and their properties are shown in Table . cDNA samples were diluted 10 times to obtain concentrations from 10−2 to 10−6, which were used to generate standard curves. Quantitative real-time PCR was performed on CFX 96 Touch Real-Time PCR System (BIO-RAD, Hercules, CA) using SYBR Premix Ex Taq Kit (Takara Biotechnology Co. Ltd.). The PCR reaction consisted of 10 μL of SYBR Premix Ex Taq (2×) mix (Takara Biotechnology Co. Ltd.), 5 μL of cDNA, 4 μL of RNase-Free water, and 1 μL of each primer, for a total volume of 20 μL. PCR amplification was carried out for 5 min at 95 °C, followed by 40 cycles of 95 °C for 10 s and 60 °C for 10 s. After amplification, a melting curve analysis was performed to verify the specificity of the reactions.
Table 1. Primers used for qRT-PCR.
For relative gene expression quantitation, qRT-PCR data were normalised to the house keeping gene 18S rRNA and analysed by the 2-ΔΔCT method, with landrace pig specimens at day 0 considered the reference samples.
Data analysis
Statistical analysis was carried out using the SPSS18.0 software. Paired samples t test was performed to assess the differences within the same breed for various ages; independent samples t test was used to assess the differences between the two breeds for the same age or different ages. The associations of mRNA levels of MyHC subtypes with muscle colour, conductivity, and intramuscular fat were analysed with Pearson linear regression. Data are mean ± standard error of the mean (SEM). p < .05 was considered statistically significant.
Results
Meat quality comparison between Bama miniature pigs and Landrace swine
Meat quality was determined for Bama miniature pigs (180, 300 days old) and Landrace pigs (180 days old). As shown in Table , back fat thickness of 180 day old Bama miniature pigs was significantly higher than that of 180 day old Landrace pigs (p < .05); meanwhile, eye areas of Bama miniature pigs (180 days) were significantly lower than the values obtained for 180 day old Landrace pigs (p < .05). Meat colour was similar in both pig breeds, with no significant differences (p > .05). Muscle conductivity of Bama miniature pigs at 300 days of age was significantly lower than the values obtained for this breed at 180 days (p < .05); meanwhile, muscle conductivity of 180 day old Bama miniature pigs was significantly reduced compared with that of Landrace pigs of the same age (p < .05). Muscle shear force was slightly lower in Bama miniature pigs compared with Landrace pigs, but the difference was not statistically significant (p > .05). Intramuscular fat contents of Bama miniature pigs at 180 days old were significantly higher compared with Landrace pig values (p <.05); meanwhile, 300 day old Bama miniature pigs showed significantly higher intramuscular fat levels compared with younger animals (180 days old) of the same breed (p < .05; Table ).
Table 2. Meat quality data for Bama miniature pigs and Landrace pigs.
Bama miniature pigs and Landrace swine display distinct enzyme activity profiles in muscle samples
As shown in Table , LDH activity in muscle tissues from Bama miniature pigs at 180 days of age was significantly lower compared with that of 180 day old Landrace pigs (p < .05). In addition, 300 day old Bama miniature pigs showed significantly higher LDH activity compared with 180 day old animals of the same breed (p < .05). SDH activity in muscle tissue samples from Bama miniature pigs at 300 days of age was significantly higher than values obtained for 180 day old Bama miniature pigs (p < .05). No significant difference in SDH activity was found between Bama miniature pigs and Landrace pigs at 180 days of age (p > .05). Finally, no statistically significant differences were obtained in MDH enzyme activities among the three pig groups (p > .05).
Table 3. Enzyme activity of muscle tissue.
Bama miniature pigs and Landrace swine show distinct MyHC gene expression profiles
Gene expression levels of MyHC I, II A, II B, and II X in M. longissimus samples from Bama miniature pigs at birth were significantly higher than values obtained for Landrace pigs of the same age (all p < .05), as shown in Figure .
Figure 1. Gene expression trends for myosin heavy-chain (MyHC) I, II A, II B and II X in Bama miniature pigs and Landrace pigs. M. longissimus samples were collected from animals slaughtered at 0, 30, 60, 90 and 180 or 300 days of age for RNA purification. Real-time quantitative reverse transcription PCR (qRT-PCR) was carried out to assess the expression levels of MyHC I (a), II A (b), II B (c) and II X (d) mRNA, with 18S rRNA as an internal control. Data are mean ± SEM (n = 3). *p < .05, **p < .01 Bama miniature pigs vs. Landrace pigs at the same age. #p < .05, ##p < .01 Bama miniature pigs at 300 days of age vs. Landrace pigs at 180 days of age.
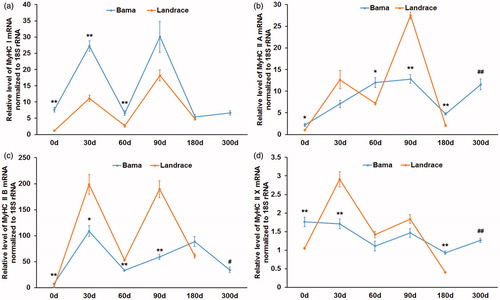
Gene expression levels of MyHC I in 30 day old Bama miniature pigs were overtly higher compared with those of Landrace pigs of the same age (Figure , p < .01), while lower MyHC II B, and II X expression levels were observed in Bama miniature pigs compared with Landrace pigs (p < .05); MyHC IIA was not statistically different between Bama miniature pigs and Landrace pigs of this age (Figure ).
At 60 days of age, MyHC I and II A mRNA levels were higher in Bama miniature pigs than in Landrace pigs (Figure , p < .05), while MyHC II B gene expression levels were reduced compared with the values obtained for Landrace pigs; meanwhile, MyHC IIX gene expression was similar in Bama miniature pigs and Landrace pigs of this age (Figure , all p < .05). In 90–day-old Bama miniature pigs, gene expression levels of MyHC II A and II B were reduced compared with Landrace pig values (Figure ; all p < .05); meanwhile, MyHC I and MyHC IIX mRNA levels were similar in Bama miniature pigs and Landrace pigs of this age (Figure ; p > .005).
MyHC I gene expression levels in Bama miniature pigs at 300 days of age were similar to the values obtained for 180 day old Landrace pigs; in addition, no significant difference was obtained in 180 day old animals of both breeds (Figure , p > .05). Interestingly, MyHC II A and II X levels in Bama miniature pigs at 180 and 300 days of age were significantly higher compared with the values obtained for 180 day old Landrace pigs (Figure , p < .05). Gene expression levels of MyHC II B were significantly lower in muscle samples from Bama miniature pigs at the age of 300 days, compared with the values obtained for 180 day old Landrace pigs (Figure , p < .01); however, slightly higher values were obtained in Bama miniature pigs at the age of 180 days compared with age matched Landrace pigs, although the difference was not statistically significant (Figure , p > .05).
Further, the associations of mRNA amounts of various MyHC subtypes with muscle colour, conductivity and intramuscular fat (IMF) were analysed. Interestingly, IMF was positively correlated with MyHC I expression and negatively correlated with MyHC II B mRNA levels in 180–day-old Bama miniature pigs (Table ).
Table 4. Correlation between expression of MyHC subtypes and muscle colour, conductivity and intramuscular fat in Bama miniature pigs at 180 day of age.
Discussion
As shown above, meat quality was different between Bama miniature pigs and Landrace swine, which was reflected by differences in LDH and SDH activities as well as MyHC gene expression levels. Species, genotype, sex, age, nutrition, and slaughtering conditions can affect meat quality. The skeletal muscle fibre is one of the most important factors defining meat quality; indeed, numerous studies have reported the relationship between muscle fibre types and pork quality (Kim et al. Citation2008). The muscle of pigs mainly contains four types of fibres, divided into I, II A, II B, and II X, according to MyHC subtype; currently, this method of dividing muscle fibre types is widely accepted (Park et al. Citation2009). Interestingly, previous studies using immunohistochemistry and fluorescence quantitative PCR reported a significant difference in type II muscle fibres among different breeds (Lefaucheur et al. Citation2004; Wimmers et al. Citation2008). In this study, the distribution of the four muscle fibre types in M. longissimus from Bama miniature pigs and Landrace swine was assessed by quantitative PCR: MyHC I expression was higher in Bama miniature pigs compared with Landrace pigs except at 180 days of age. It has been suggested that high content of muscle fibre MyHC I results in much higher meat quality (Choi & Kim Citation2009). Meanwhile, we demonstrated that IMF was positively correlated with MyHC I mRNA expression in Bama miniature pigs at 180 days of age. It should be noted that type I fibres contain more triglycerides within them compared with type II fibres, especially type IIB fibres; meanwhile, most IMF is localised in intramuscular adipocytes between fibres and IMF content largely depends on the amount of triglycerides in intramuscular adipocytes (Picard et al. Citation2010). Bama miniature pig meat is delicious, and known as ‘a boiled pork neighbor’s incense, seven miles away from the smell of its taste’. The muscle fibre MyHC II B is involved in muscle development. When the muscle grows faster, the MyHC II B content increases markedly. We demonstrated that muscle fibre MyHC II B amounts in Bama miniature pigs at 30 and 60 days of age were significantly lower compared with Landrace pig values. These findings are consistent with data reported by Yang et al. (Citation2005) who showed that MyHC II B levels in large white pigs older than 90 days are significantly higher than the values obtained for Erhualian pigs (Yang et al. Citation2005). At 180 days of age, MyHC II B mRNA levels in Bama miniature pigs were higher compared with those of Landrace pigs, indicating that muscle development in Bama miniature pigs was still at the production peak, while muscle growth of Landrace pigs at this age was slow, with increasing intramuscular fat deposition. Muscle fibre transformation is similar among breeds, unlike conversion efficiency; a difference is found only in the amount of muscle fibres but not in quality. With age, some slow-twitch fibres will be converted to fast-twitch fibres (Ohlendieck Citation2011); the order is I, II A, II X, and II B. Other parameters, e.g. perimortem energy metabolism and different environmental factors, affect post-mortem transformation from muscle to meat (Karlsson et al. Citation2000); this might contribute to the large variations observed at some time points. Further studies are required to clarify this point.
Bama miniature pigs have a relatively slow growth after 112 days of age (growth curves of Bama miniature pigs and Landrace swine are shown in Supplementary Figure S1). After that age, the growth of Bama miniature pigs is relatively steady and the characteristics of muscles are relative stable. Although weight gain of Bama miniature pigs was observed during growth from 180 to 300 days of age, no significant differences in back fat thickness and eye muscle area between 180 and 300 days of age were observed, which may be due to its premature character.
SDH and MDH are key enzymes of the tricarboxylic acid cycle, and participate in the aerobic oxidation of glucose. LDH is a key enzyme involved in glycolysis, i.e. glucose or glycogen decomposition. Type I muscle fibre contains much more mitochondria, myoglobin, and cytochrome, and is mainly involved in aerobic metabolism; its enzyme activity is high, with slow and lasting muscle contraction. The metabolism of II B muscle fibres is opposite to that of type I cells. II B contains few mitochondria, high glycogen content, and important ATPase activity, participating in anaerobic metabolism: muscle contraction is fast and robust but does not last long. The functions of II A and II X are between those of I and II B (Choe et al. Citation2008). Oxidative muscle fibres contain a lot of capillaries and mitochondria, and have high oxygen metabolic rates and SDH activity, with low-LDH activity; conversely, glycolytic muscle fibres have low myoglobin and SDH activity, and high-LDH activity (Armstrong Citation1996). As shown above, the aerobic metabolism related enzyme SDH had a significantly higher activity in Bama miniature pigs than Landrace animals; meanwhile, the activity of LDH, a glycolysis related enzyme, was significantly lower. These findings indicate that aerobic metabolism may be higher in Bama pigs, which could explain the higher oxidative muscle fibres observed in these pigs. However, we did not assess other important parameters of muscle fibres, e.g. muscle fibre size and area, which profoundly affect muscle metabolism. In addition, this was a preliminary study with only three animals per group. Therefore, further comprehensive experiments with larger sample size are warranted to confirm the current findings.
Conclusions
In conclusion, differential MyHC expression and enzyme activity in muscle fibres are the most important factors affecting pork quality, and likely result in great differences in fat content between Bama miniature pigs and Landrace swine. These preliminary findings provide a basis for improving meat quality.
Supplemental_Figure_S1.tif
Download TIFF Image (117.7 KB)Disclosure statement
The authors declare that they have no conflict of interest.
Additional information
Funding
References
- Armstrong R. 1996. Properties, distribution, and functions of mammalian skeletal muscle fibers. Exercise bioenergetics and gas exchange. Amsterdam: Elsevier/North-Holland Biomedical Press; p. 137–146.
- Bee G. 2007. Birth weight of litters as a source of variation in postnatal growth, and carcass and meat quality. Age. 6:10.
- Chang KC, da Costa N, Blackley R, Southwood O, Evans G, Plastow G, Wood JD, Richardson RI. 2003. Relationships of myosin heavy chain fibre types to meat quality traits in traditional and modern pigs. Meat Sci. 64:93–103.
- Chikuni K, Tanabe R, Muroya S, Nakajima I. 2001. Differences in molecular structure among the porcine myosin heavy chain-2a, -2x, and -2b isoforms. Meat Sci. 57:311–317.
- Choe JH, Choi YM, Lee SH, Shin HG, Ryu YC, Hong KC, Kim BC. 2008. The relation between glycogen, lactate content and muscle fiber type composition, and their influence on postmortem glycolytic rate and pork quality. Meat Sci. 80:355–362.
- Choi Y, Kim B. 2009. Muscle fiber characteristics, myofibrillar protein isoforms, and meat quality. Livest Sci. 122:105–118.
- Hamalainen N, Pette D. 1993. The histochemical profiles of fast fiber types IIB, IID, and IIA in skeletal muscles of mouse, rat, and rabbit. J Histochem Cytochem. 41:733–743.
- Han JC, Yang XD, Zhang T, Li H, Li WL, Zhang ZY, Yao JH. 2009. Effects of 1alpha-hydroxycholecalciferol on growth performance, parameters of tibia and plasma, meat quality, and type IIb sodium phosphate cotransporter gene expression of one- to twenty-one-day-old broilers. Poult Sci. 88:323–329.
- Hu X, Zhou X, He B, Xu C, Wu L, Cui B, Wen H, Lu Z, Jiang H. 2010. Minocycline protects against myocardial ischemia and reperfusion injury by inhibiting high mobility group box 1 protein in rats. Eur J Pharmacol. 638:84–89.
- Karlsson AH, Klont RE, Fernandez X. 2000. Skeletal muscle fibres as factors for pork quality. Livest Prod Sci. 60:255–269.
- Kim NK, Lim JH, Song MJ, Kim OH, Park BY, Kim MJ, Hwang IH, Lee CS. 2008. Comparisons of longissimus muscle metabolic enzymes and muscle fiber types in Korean and western pig breeds. Meat Sci. 78:455–460.
- Klosowska D, Fiedler I. 2003. Muscle fibre types in pigs of different genotypes in relation to meat quality. Anim Sci Pap Rep. 21:49–60.
- Lee SH, Joo ST, Ryu YC. 2010. Skeletal muscle fiber type and myofibrillar proteins in relation to meat quality. Meat Sci. 86:166–170.
- Lefaucheur L, Ecolan P, Plantard L, Gueguen N. 2002. New insights into muscle fiber types in the pig. J Histochem Cytochem. 50:719–730.
- Lefaucheur L, Milan D, Ecolan P, Le Callennec C. 2004. Myosin heavy chain composition of different skeletal muscles in Large White and Meishan pigs. J Anim Sci. 82:1931–1941.
- Lind A, Kernell D. 1991. Myofibrillar ATPase histochemistry of rat skeletal muscles: a “two-dimensional quantitative approach”. J Histochem Cytochem. 39:589–597.
- Ohlendieck K. 2011. Proteomic profiling of fast-to-slow muscle transitions during aging. Front Physiol. 2:105.
- Park SK, Gunawan AM, Scheffler TL, Grant AL, Gerrard DE. 2009. Myosin heavy chain isoform content and energy metabolism can be uncoupled in pig skeletal muscle. J Anim Sci. 87:522–531.
- Pette D, Staron RS. 2001. Transitions of muscle fiber phenotypic profiles. Histochem Cell Biol. 115:359–372.
- Picard B, Berri C, Lefaucheur L, Molette C, Sayd T, Terlouw C. 2010. Skeletal muscle proteomics in livestock production. Brief Funct Genomics. 9:259–278.
- Wang AD, Lan GQ, Guo YF. 2010. Genetic breeding of Guangxi Bama mini-pig. Lab Anim Sci. 1:60–63.
- Wimmers K, Ngu NT, Jennen DG, Tesfaye D, Murani E, Schellander K, Ponsuksili S. 2008. Relationship between myosin heavy chain isoform expression and muscling in several diverse pig breeds. J Anim Sci. 86:795–803.
- Wu F, Zuo JJ, Yu QP, Zou SG, Tan HZ, Xiao J, Liu YH, Feng DY. 2015. Effect of skeletal muscle fibers on porcine meat quality at different stages of growth. Genet Mol Res. 14:7873–7882.
- Yang X, Zhao R, Chen J, Xu Q, Wei X, Chen J. 2005. Developmental changes, species and gender characteristics of the longissimus muscle fiber types. Chin J Vet Sci. 25:89–94.
- Zhang B, Lin S. 2009. Effects of 3,4-dichloroaniline on testicle enzymes as biological markers in rats. Biomed Environ Sci. 22:40–43.
- Zheng S, Jing G, Wang X, Ouyang Q, Jia L, Tao N. 2015. Citral exerts its antifungal activity against Penicillium digitatum by affecting the mitochondrial morphology and function. Food Chem. 178:76–81.