Abstract
This study was conducted to evaluate the dietary supplementation of Trichoderma pseudokoningii enzyme powder (EP) and its effect on the growth performance, intestinal morphology and antioxidative status of broilers. In vitro experiments showed that xylanase and cellulase activities of EP were 487.96 ± 14.14 U/g and 18.55 ± 0.54 U/g, respectively. Total phenolic and ferulic acid content was 4.79 ± 0.35 mg GAE/g DW and 4.38 ± 0.32 μM/g DW, respectively. A total of 240 d-old Ross 308 male broiler chicks were randomly subjected to one of four treatments, namely diets supplemented with dry EP at 0% (control), 0.1%, 0.2% or 0.4% for 35d. Results showed that in the starter phase (1–21 d), the EP supplemented groups had improved body weight, weight gain and FCR compared to the control group. The villus:crypt ratio of both jejunum and ileum in all EP-treated groups was significantly higher than that of the control group. When testing serum antioxidant activity, results showed that 0.2% and 0.4% EP supplementation significantly increased superoxide dismutase activity in both the finisher phase and the overall experimental period. In addition, catalase activity was elevated in all EP supplemented groups in both the finisher phase and throughout the experimental period. 0.1% EP seemed to have no effect during the starter period. Serum malondialdehyde concentration was not affected by EP supplementation. These results suggest that EP is potential feed additive in terms of improving broiler performance and enhancing antioxidative status, while also providing an optimal intestinal environment.
Introduction
Non-starch polysaccharides (NSPs) were general component in plant-derived feedstuffs. The major concern of NSPs is their resistance to the monogastric digestive enzymes, causing anti-nutritional effects and thus contributing to restricted utilisation of nutrition in animals. Therefore, NSP-degrading enzymes (NSPase) derived from microorganisms play an important role in the animal feed industry. They have been supplemented exogenously to animals to prevent adverse effects due to NSP-rich feedstuffs, such as a wheat-based diet. However, researchers recently found that the addition of NSPase to the corn-soybean based diet of broilers improved nutrient availability (Cowieson and Ravindran Citation2008), since broken down cell wall materials enhanced the access of endogenous enzymes and released nutritive contents. Xylanase has been reported to improve lactobacilli and bifidobacteria counts in the caecum of broilers fed a corn-soybean based diet (Nian et al. Citation2011). Passos et al. (Citation2015) also proved that supplementation with xylanase improved nutrient digestion in pigs fed a corn-soybean based diet.
Filamentous fungi have been found to exert antioxidative properties by producing phenolic compounds during fermentation, suggesting they could be a promising source of natural antioxidants (Sugiharto et al. Citation2015). Exogenous antioxidants such as vitamin C or selenium powder were provided to synchronise the redox balance and endogenous antioxidants were replenished to avert negative factors caused by oxidative stress (Noori Citation2012), further preventing economic losses (Lara and Rostagno Citation2013).
Trichoderma spp. produce cell wall-degrading enzymes (xylanase and cellulase) that have been applied to soil borne pathogen-inhibiting biocontrol agents and industrial enzyme production (Harman et al. Citation2004). Goyal et al. (Citation2008) produced xylanase with T. viride from xylan-rich lignocellulosic waste via submerged culture. Cacais et al. (Citation2001) also produced xylan-degrading enzymes by submerged culture of T. harzianum. With regard to antioxidant production, Singh et al. (Citation2010) discovered the ability of T. harzianum to modulate natural antioxidants in a soybean seed matrix by increasing the release of polyphenol. Due to this particularity, Trichoderma species are suitable for producing antioxidants and NSP enzymes. However, there are still limited studies utilising Trichoderma spp. in situ enzyme production on farm animals.
Our previous results showed that substituting 10% of a basal diet with Trichoderma pseudokoningii fermented wheat bran improved the growth performance and intestinal morphology of broilers (Chu et al. Citation2016), indicating the potential of T. pseudokoningii to mitigate high feed costs. However, before this fungal strain could be used as an exogenous enzyme-providing feed additive, the variety of substrate qualities and the cumbersome fermentation process would need to be further investigated, in order to confirm its consistent quality, convenience and efficiency.
This paper established the method of producing enzyme powder (EP) with antioxidant properties by T. pseudokoningii in situ enzyme production using solid-state fermentation (SSF). Furthermore, after dietary supplementation with EP, the growth performance and serum antioxidative parameters of broilers were tested in order to evaluate its potential as a functional feed additive.
Materials and methods
Micro-organisms
The T. pseudokonigii used for this study were kindly provided by the Department of Biotechnology, National Formosa University, Yunlin, Taiwan, and were capable of fermenting lignocellulose-rich materials. The micro-organisms were routinely maintained on a potato dextrose agar (PDA) plate at 25 °C with regular sub-cultivation (no longer than 1 week). An appropriate amount of sterilised 20% molasses solution was added to the plate before gently shaking it by hand to retrieve the spore suspension. The concentration of T. pseudokoningii spores in suspension was diluted to 1 * 107–5 * 107 spores per mL for subsequent usage as inoculum to prepare enzyme powder (EP). Wheat bran purchased from the Formosa Oilseed Processing Corporation, Taichung, Taiwan, was chosen as the substrate for SSF.
SSF
The techniques for large-scale production of EP are similar to SSF in the lab. About 50 g of wheat bran were weighed into a plastic bag and the moisture content was adjusted to 50% with Toyama’s solution. The contents of each bag were thoroughly mixed before being autoclaved at 121 °C ± 1 °C for 30 min. The autoclaved wheat bran was inoculated with 0.2 mL of T. pseudokoningii spore suspension per gram of wheat bran. The wheat bran was fermented aerobically under environmentally controlled conditions and maintained at 25 °C for 7 d. All samples were dried at 40 °C for 2 d then ground in a mill before being packed in plastic bags, sealed and stored at ambient temperature.
Enzyme extraction and assays
The 3 g of EP was weighted and diluted 10-fold by the buffer and stirred for 30 min at 4 °C to extract the extracellular enzyme solution. The solution was than centrifuged at 3000 rpm at 4 °C for 10 min and filtered through filter paper (Advantec No. 1, Tokyo, Japan), before detecting cellulase and xylanase activities. Both enzymes were assayed using the dinitrosalicylic acid (DNS) method (Miller Citation1959) to determine the reducing sugar content. Briefly, 0.1 mL of diluted enzyme solution was added to 0.9 mL of substrate and vortexed. Subsequently, the mixture was left to react for 10 min in a water bath. The reaction was stopped by adding 1 mL DNS and boiled a further 5 min. The colours that developed were measured spectrophotometrically at 540 nm. One international unit (IU) of cellulase and xylanase activity was defined as the quantity of enzyme required to liberate 1 μm of reducing sugar (glucose/xylose) of crude filtrate per minute under standard assay conditions (50 °C, pH 4.8 for cellulase and 55 °C, pH 5.3 for xylanase).
Bioactive compound extraction and determination
Air-dried EP powder was weighed and added to distilled water, which was then left to stand at 95 °C for 1 h. The solution was filtered (Advantec No. 1, Tokyo, Japan) to obtain the EP extract (EPE), which was stored at −20 °C for further analysis. The total phenolic contents were determined using a Folin–Ciocalteu reagent according to the method described by Kujala et al. (Citation2000). The Folin–Ciocalteu reagent was mixed evenly with EPE before adding the sodium carbonate by using the equation obtained from the standard gallic acid graph, the phenolic compounds of the EPE (microgram of the gallic acid equivalent, mg GAE), was then determined.
Ferrous chelating capacity assay
The ferrous ion chelating activity of the EPE was determined as per the methods of Dinis et al. (Citation1994). Briefly, 0.25 mL EPE was mixed with 0.025 mL 2 mM ferrous chloride solution and 0.925 mL methanol. After 30 min incubation at room temperature (RT), 0.05 mL 5 mM ferrozine was added to initiate the reaction and the mixture was then left to stand at RT for 10 min. When the reaction was complete, the absorbance of the mixture was measured spectrophotometrically at 562 nm. The inhibiting percentage of ferrozine–Fe2+ complex formation was calculated according to the following formula:
where A0 is the absorbance of the control and A1 is the absorbance of the EPE and EDTA. The control contained ferrous chloride, ferrozine and complex formation molecules.
Determination of reducing power
The reducing power of the EPE was measured using the methods described by Oyaizu (Citation1986). Briefly, the EPE was mixed with phosphate buffer (0.2 M, pH 6.6) and potassium ferricyanide (1%). The mixture was incubated at 50 °C for 20 min. before the reaction was terminated by adding 2.5 mL 10% trichloroacetic acid. About 5 mL of the solution was then mixed with the same volume of distilled water and 0.1% ferric trichloride. After 10 min of incubation, the absorbance was detected at 700 nm by spectrophotometer; ascorbic acid was used as a control. The increase in the reaction mixture absorbance indicated greater reducing power.
1,1-Diphenyl-2-picrylhydrazyl (DPPH) free radical scavenging capacity assay
The free radical scavenging ability of the EPE was investigated according to the methods of Blois (Citation1958). Briefly, DPPH ethanol solution (0.1 mM) was prepared and added to the EPE at different concentrations. After 30 min incubation, the absorbance was measured at 517 nm against ascorbic acid. The lower absorbance of the reaction mixture indicated higher scavenging capacity. The scavenging effect was calculated according to the following equation:
where A0 is the absorbance of the control reaction and A1 is the absorbance in the presence of EPE.
Experimental birds and housing
The animal experiment was approved by the Animal Care and Use Committee of the National Chung Hsing University, Taiwan. A total of 240 1-d-old commercial Ross 308 male broiler chicks were randomly allocated to one of four treatments: 0% (control), 0.1%, 0.2% or 0.4% EP dietary supplementation, with four replicates and 15 birds per pen (a total of 60 birds/treatment). Room temperature was maintained at 34 ± 1 °C for the first 7 d, then gradually decreased to 26 °C ± 1 °C until the birds reached 21 d of age. A temperature of 26 °C was maintained until the end of the 35 d. The experimental period included two phases: starter phase (1–21 d) and finisher phase (22–35 d). At the beginning of the feeding trial, the average body weight of the birds was similar for all pens (approximately 43.4 ± 0.17 g/bird). All chicks were raised in a temperature-controlled house. The birds were kept in floor pens (2.5 × 4.0 m) with wire floors and rice bran litter material. In the starter phase (1–21 d), two fountain drinkers and one feed tray were installed per pen. In the finisher phase (22–35 d), these were replaced with nipple drinkers and a feeder. Vaccines for Marek’s disease, Newcastle disease and infectious bronchitis were provided to chicks immediately after birth. Moreover, vaccines for Newcastle disease and infectious bursal disease were administered on day 14. Water and feed were supplied ad libitum to the broilers. The diets (Table ) were formulated to meet or exceed the nutrient requirements of broilers (NRC Citation1994). The proximate composition was analysed according to the AOAC (Citation1980). Starter and finisher diets were offered to the birds from 1 to 21 d and from 22 to 35 d of age, respectively. Neither anti-coccidial nor anti-bacterial supplements were added to the feed mixtures.
Table 1. Ingredients and chemical composition of the basal diets of broilers.
Performance, serum and intestinal content collection
The body weight of each broiler was measured at 1, 21 and 35 d of age. Feed consumption was recorded at the end of starter phase (21 d) and finisher phase (35 d). Overall feed cost and meat profit of each group were counted at the termination of the experimental period. Body weight gains and feed conversion ratios (FCR) and income over feed cost (IOFC) were calculated from these data. At 35 d, four birds were randomly selected from each pen for sampling. The birds were bled via the brachial vein and 5 mL blood samples were collected from the wing veins. Samples were centrifuged at 2000 × g for 15 min and the serum was stored at −20 °C until lipid profile evaluation. Catalase (CAT) activity, superoxide dismutase (SOD) activity and serum malondialdehyde (MDA) levels were measured calorimetrically using a spectrophotometer. The procedures were conducted using an assay kit purchased from Cayman Chemical Co. (Ann Arbor, MI). Antioxidant enzyme activities were expressed as units (U) per millilitre of serum. After the blood samples were collected, the chickens were euthanized by exsanguination and their abdominal cavities were opened. For each treatment group, the livers, hearts, bursae, leg muscles and contents of the ilea and caeca of three birds were collected for study.
Microbial populations of intestinal content
Ileum and caecum digesta were collected and diluted serially in PBS solution for enumeration of microbial populations. Escherichia coli was cultured with chromogenic medium agar (CHROMagarTM ECC) under aerobic condition at 37 °C for 24 h and lactic acid bacteria was cultured with Difco™ Lactobacilli MRS Agar under anaerobic condition at 37 °C for 48 h. The microflora numbers were calculated and the bacterial populations were expressed as log10 CFU per gram of intestinal contents.
Intestinal morphology
On days 21 and 35 of the experiment, one bird per replicate cage from each treatment group (four birds/treatment in total) was randomly selected and sacrificed. The jejunum (from the pancreatic loop to Meckel’s diverticulum) and ileum (from Meckel’s diverticulum to the ileo-caeco-colic junction) from each bird were sectioned. Approximately 3 cm of the segments were fixed in 10% formalin for the morphometrical assays. The formalin-fixed tissue was washed in PBS and embedded in paraffin wax. Three micrometre-sectioned tissue was stained using haematoxylin and eosin methods. Samples were analysed by light microscopy and computer software (Motic image plus 2.0, Motic Inc., Wetzlar, Germany) was used to measure the villus height and crypt depth in fifteen favourably oriented and representative samples per treatment. The ratio of villus height to crypt depth was also calculated.
Determination of lactic acid concentration in the intestinal digesta
The concentrations of lactic acid in the ileal and caecal digesta were analysed using a high-performance liquid chromatography (HPLC) system (Hitachi, Tokyo, Japan) according to the methods of Grigsby et al. (Citation1992).
Statistical analysis
Data were subjected to ANOVAs as a completely randomised design using the GLM function of the SAS software (SAS Citation2004). Determination of the significant statistical differences among the mean values of the four treatment groups (0%, 0.1%, 0.2% or 0.4% EP dietary supplementation) used Tukey’s honestly significant difference test, with a significance level of p < .05.
Results
Bioactive compounds and enzyme activities
The bioactive compounds and enzyme activities of wheat bran (WB) solid state fermented with T. pseudokoningii are summarised in Table . Total phenolic content of EP was 4.79 ± 0.35 mg QE/g DW, which increased approximately 1.6- to 1.7-fold after fermentation compared to the untreated WB. The xylanase and cellulase activities detected in EP were 487.96 ± 1 4.14 and 18.55 ± 0.54 U/g DW, respectively.
Table 2. The content of bioactive compounds and enzyme activities of Trichoderma pseudokoningii fermented enzyme powderTable Footnotea.
DPPH radical scavenging capacity
Figure illustrates the DPPH radical scavenging capacity of EPE. Compared with 0.1 mg/mL BHT, adding 10 mg/mL EPE exerted the equivalent of a 35% scavenging effect, while WBE only had a 25% chelating effect. The scavenging capacity of both EPE and WBE decreased when the concentration exceeded 10 mg/mL and only increased slightly as concentration increased.
Reducing power
The reducing power of EPE is shown in Figure . Ascorbic acid reached the maximum reducing power (A700 value) at 0.5 mg/mL and did not increase further with increasing concentration. The reducing powers exhibited when 50 mg/mL EPE and WBE were added were equivalent to 60% and 40% of the value associated with 0.5 mg/mL ascorbic acid, respectively.
Ferrous iron chelating capacity
Figure shows the ferrous iron chelating capacity of the EPE. At 12.5 mg/mL, the chelating ability of EPE was 80%, while WBE exerted 50% compared with 0.1 mg/mL EDTA.
Growth performance
Table shows the growth performance of 1- to 35-d-old broiler chickens supplemented with EP. The body weight, feed consumption and weight gain of all broilers supplemented with EP were significantly higher than the control group from 1 to 21 d of age (p < .05), leading to significantly better FCR overall during this period (p < .05). However, from 22 to 35 d of age, there were no significant differences in the growing traits. The overall growth performance showed the same tendency.
Table 3. Effect of Trichoderma pseudokoningii fermented enzyme powder supplemented in diet on growth performance of 1–35 d-old broilers.
Lactate concentration of intestinal contents
The effect of EP supplementation on the lactate concentration in the intestinal contents of the broiler chickens is shown in Table . The lactate concentration in the caecal content of all EP supplemented groups was significantly higher from 35 d of age compared with the control group. Overall, 0.2% EP supplementation caused the greatest elevation in caecal lactate concentration while EP seemed to have no effect on ileal lactate concentration.
Table 4. Effect of Trichoderma pseudokoningii fermented enzyme powder (EP) supplemented in diet on lactate concentration of intestinal content of 1–35 d-old broilers.
Microbial population in ileum and caecum
The effects of EP dietary supplementation on the microbial population in broiler ilea and caeca after 35 d are presented in Table . The lactic acid bacteria population was increased in the caeca of the 21 d old chickens that received EP supplementation, in which 0.2% supplementation produced the best results. EP supplementation significantly lowered the coliform population throughout the entire experimental period; however, no difference in the lactic acid bacteria population was observed.
Table 5. Effect of Trichoderma pseudokoningii fermented enzyme powder (EP) supplemented in diet on microbial parameter in intestinal content of 1–35 d-old broilers.
Intestinal morphology
Table demonstrates the effects of dietary EP supplementation on the intestinal morphology of the broiler chickens. Jejunal villus height was increased by EP supplementation during both the starter and finisher periods. The villus:crypt ratio in the jejunum and ileum were improved in all EP supplemented groups compared to the control group in both 21- and 35-d-old chicken (Figure ).
Figure 2. Photomicrography of jejunum and ileum of 21 and 35 d old broiler fed with control and fermented wheat brans. (A)–(D) Jejunum of 21 d broiler, control; 0.1% EP; 0.2%; 0.4% EP. (E)–(H) Jejunum of 35 d broiler, control; 0.1% EP; 0.2%; 0.4% EP. (I)–(L) Ileum of 21 d broiler, control; 0.1% EP; 0.2%; 0.4% EP. (M)–(P) Ileum of 35 d broiler, control; 0.1% EP; 0.2%; 0.4% EP haematoxylin and eosin stain. 40×.
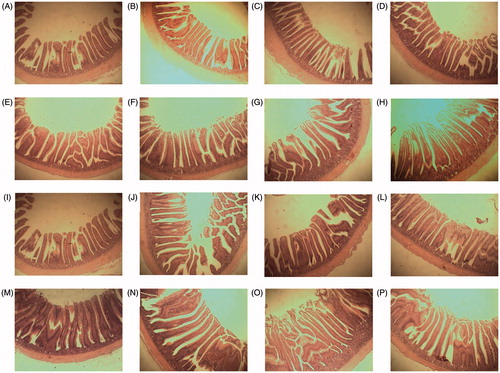
Table 6. Effect of Trichoderma pseudokoningii fermented enzyme powder (EP) supplemented in diet on intestinal morphology of 1–35 d-old broilers.
Serum antioxidant enzyme activities
Table shows the effects of dietary EP supplementation on serum antioxidant enzyme activity in broiler chickens at different periods. CAT activity was significantly elevated in the 0.2% and 0.4% EP supplemented groups from 1 to 21 d of age. From 22 to 35 d of age, 0.4% and 0.2% EP supplementation significantly raised SOD and CAT activity, respectively. Overall, SOD and CAT activities were increased by EP supplementation, with 0.4% supplementation producing the best effect.
Table 7. Effects of Trichoderma pseudokoningii fermented enzyme powder (EP) supplemented in diets on the serum antioxidant enzymes activities and MDA levels of 1–35 d-old broilers.
Economic benefits
An evaluation of the economic benefits of adding EP to the broiler diet is summarised in Table . The income over feed cost (IOFC) of the control, 0.1%, 0.2% and 0.4% EP groups were 55.6, 58.7, 58.4 and 58.1 NT$/bird, respectively.
Table 8. Evaluation of the economic benefit of Trichoderma pseudokoningii-fermented enzyme powder (EP) supplemented in diet.
Discussion
Trichoderma spp. are known to produce NSPase by fermenting various lignocellulosic substrates with different features. Pang et al. (Citation2006) produced xylanase from local isolated Trichoderma spp. FETL c3-2 via SSF with sugar cane bagasse and palm kernel cake, and retrieved 75.0 U/mg glucosamine xylanase activity after 4 d fermentation. Azin et al. (Citation2007) recorded xylanase activity of 592.7 U/g substrate after fermenting wheat bran and wheat straw with Trichoderma longibrachiatum. Our previous study also found that T. pseudokoningii produced 179.7 ± 6.62 U/g and 15.19 ± 0.15U/g of xylanase and cellulase activity, respectively, by fermenting wheat bran (Chu et al. Citation2016). However, it was widely recognised that microbial enzyme activity during fermentation was affected by the form and property of the substrates. Based on our previous study, this indicated the potential of T. pseudokoningii fermented WB producing higher enzyme activity. Kalogeris et al. (Citation2003) studied the optimal incubation environment of Thermoascus aurantiacus to produce xylanase and determined that enhanced extracellular enzyme activity was produced from carbon sources with a finer particle size (< 0.5 mm). In this study, the higher xylanase activity (487.96 ± 14.14 U/g), compared with our previous study (179.7 ± 6.62 U/g), was due to the modification of particle size (< 0.5 mm), day of incubation and the addition of Toyama’s mineral solution (1% (NH4)2SO4, 0.3% KH2PO4, 0.05% MgSO4·7H2O and 0.05% CaCl2·2H2O, pH 5.5).
According to our results, EP supplementation caused a significant improvement in body weight, feed consumption, weight gain and FCR in broilers during the starter period, compared with the control group. However, there were no significance differences during the finisher period or in the overall period. Xylanase supplementation has been shown to be beneficial to the performance of feed-restricted broilers (Pinheiro et al. Citation2004). Nian et al. (Citation2011) also demonstrated that the addition of xylanase to a corn–soybean-based diet in broilers significantly improved feed energy as well as FCR. This improvement could be due to the ability of NSPase to break down the encapsulation of starch and proteins in botanical resources. The lack of significant improvements in the finisher period and the overall experiment can be explained by the complementary development of digestion enzymes and the ability of older chickens to utilise feed nutrients (Noy and Sklan Citation1995).
In this study, we observed an improvement in gut microflora by supplementation of broiler diet with EP, which was in agreement with Nian et al. (Citation2011), who found that lactobacilli and bifidobacteria counts increased with the supplementation of xylanase in broilers. Bedford (Citation2000) also showed that exogenous enzymes could alter microbial populations indirectly within the GI tract through their utilisation of the substrates that bacteria use as a carbon source. Furthermore, lactic acid concentration in the caecum was significantly increased by the addition of EP in the finisher period. This was in consistent with the findings of Pluske et al. (Citation2001), who reported carbohydrase supplementation increased the proportion of lactic and organic acids and promoted gut health by suppressing the growth of pathogens. The increasing lactic acid concentration, caused by the increasing population of lactic acid bacteria, contributed to an acidic environment in the GI tract that partially influenced the growth of pathogenic microorganisms (Yang et al. Citation2015). These studies supported the ability of dietary EP supplementation to enhance gut microflora and inhibit the growth of E. coli as well.
Many of the reports attributed the improvement of intestinal morphology to NSPase dampening the deleterious effects of NSP-rich feedstuffs on the intestinal villi (Wang et al. Citation2005; Luo et al. Citation2009). However, in our study, this effect should be regarded as a beneficial effect of EP addition as it caused pathogenic bacteria inhibition as well as beneficial bacteria promotion. The increase in villi height represents an improvement in the area available for nutrient absorption in the intestine (Xu et al. Citation2003). The crypt region is the origin of the villi epithelial cells and the depth of the crypts represents the cell turnover rate. With regard to farm animal feed efficiency, low depth was favoured in order to save energy for animal growth (Parsaie et al. Citation2007). Stressors presented in the digesta can cause dynamic changes in the intestinal mucosa, due to the close proximity of the mucosal surface and the intestinal contents (Giannenas et al. Citation2010). In our study, EP supplemented to the broilers diet was found to be phenolic-rich and capable of preventing oxidative stress, which may be one of the reasons why gut morphology improved. Similar results were showed in the study of Viveros et al. (Citation2011), suggesting dietary polyphenol-rich grape pomace concentrate (60 g/kg) could improve gut morphology (villus height:crypt depth) and increase feed efficiency in broiler chicks. Lai et al. (Citation2015) also found that fermented soybean hulls containing phenolic-rich Pleurotus eryngii stalk residues could increase ileum villus height:crypt depth.
Phenolic content had been proven to contribute to the antioxidant capacity of fungal mycelia (Wu and Hansen Citation2008). Antioxidants can be classified into four categories according to their operating mechanisms: chain breaker, peroxide decomposer, metal chelator and reactive oxygen scavenger (Krinsky Citation1992). The phenolic content of EP was first detected followed by several in vitro assays determining different aspects of antioxidant capacities in EP. DPPH is a stable free radical (Eklund et al. Citation2005); decolouring them represents the ability of a sample to directly remove free radicals. Hydrogen donating ability was indicated in the reducing power assay, which was reported to be one of the antioxidant abilities of mushrooms (Shimada et al. Citation1992). Ferrous iron (Fe2+) could initiate many radical reactions by transferring single electrons, indicating the important role of iron chelation agents as antioxidants. Trichoderma spp. had been shown to enhance the antioxidant defence of plants by secreting fungitoxic phenolic compounds (Mastouri et al. Citation2012; Bernal-Vicente et al. Citation2014). However, few studies have examined such filamentous fungi exerting antioxidant capacities on animals.
In animal cells, non-enzymatic and enzymatic antioxidant systems are developed to limit the potential toxicity of ROS. Enzymatic antioxidants are synthesised and regulated endogenously, which are also essential indicators of the oxidative status of animal tissues (Jiang et al. Citation2007; Giannenas et al. Citation2010). SOD and CAT are two of the endogenous antioxidant enzymes that make up the antioxidant cellular enzymatic system (Blokhina et al. Citation2003). SOD catalyses the dismutation of O2- to H2O2 and O2, and also functions in conjugation with CAT, which converts H2O2 to H2O (Weiss Citation1986). Filamentous fungi have been shown to produce secondary metabolites possessing antioxidant abilities (Yadav et al. Citation2014; Wang et al. Citation2017), and are also capable of improving broiler oxidative status (Wang et al. Citation2017). In our study, the improved CAT and SOD activity of broilers supplemented with EP was due to the antioxidant properties of polyphenols produced by T. pseudokoningii during fermentation (Table ). Wang et al. (Citation2017) supplemented broilers with white rot fungi fermented wheat bran and claimed the phenolic content improved serum CAT activity. Increased broiler CAT and SOD activities mediated by the phenolic content of Pleurotus eryngii stalk residues and soybean hulls fermented by Aureobasidium pullulans were also found by Lai et al. (Citation2015), suggesting that fungi phenolic content is able to improve animal oxidative status, which is in consistent with the current findings.
Conclusions
In conclusion, supplementing the corn-soybean based diet of broilers with T. pseudokoningii fermented enzyme powder improves their performance in the starter phase, as well as optimising the intestinal environment by enhancing intestinal microflora and gut morphology. The antioxidant properties exerted by T. pseudokoningii also contributed to the increased in SOD and CAT activities and improved broiler oxidative status. Based on our results, we recommend 0.4% as the optimal amount to add to broiler feed.
Disclosure statement
No potential conflict of interest was reported by the authors.
Additional information
Funding
References
- Association of Official Analytical Chemists (AOAC). 1980. Official methods of analysis, 457 13th ed. Washington (DC): Association of Official Analytical Chemist.
- Azin M, Moravej R, Zareh D. 2007. Production of xylanase by Trichoderma longibrachiatum on a mixture of wheat bran and wheat straw: optimization of culture condition by Taguchi method. Enzyme Microb Technol. 40:801–805.
- Bedford MR. 2000. Exogenous enzymes in mono-gastric nutrition: their current value and future benefits. Anim Feed Sci Technol. 86:1–13.
- Bernal-Vicente A, Pascual J, Tittarelli F, Hernández J, Diaz-Vivancos P. 2014. Trichoderma harzianumT-78 supplementation of compost stimulates the antioxidant defense system in melon plants. J Sci Food Agric. 95:2208–2214.
- Blois MS. 1958. Antioxidant determinations by the use of a stable free radical. Nature. 26:1199–1200.
- Blokhina O, Virolainen E, Fagerstedt KV. 2003. Antioxidants, oxidative damage and oxygen deprivation stress: a review. Ann Bot. 91:179–194.
- Cacais AOG, Silveira FQP, Ferreira Filho EX. 2001. Production of xylan-degrading enzymes by a Trichoderma harzianum strain. Braz J Microbiol. 32:141–143.
- Chu YT, Lo CT, Chang SC, Lee TT. 2016. Effects of Trichoderma fermented wheat bran on growth performance, intestinal morphology and histological findings in broiler chickens. Ital J Anim Sci. 16:82–92.
- Cowieson AJ, Ravindran V. 2008. Effect of exogenous enzymes in maize-based diets varying in nutrient density for young broilers: growth performance and digestibility of energy, minerals and amino acids. Br Poult Sci. 49:37–44.
- Dinis TCP, Madeira VMC, Almeida LM. 1994. Action of phenolic derivates (acetoaminophen, salycilate, and 5-aminosalycilate) as inhibitors of membrane lipid peroxidation and as peroxyl radical scavengers. Arch Biochem Biophys. 315:161–169.
- Eklund PC, Langvik OK, Warna JP, Salmi TO, Willfor SM, Sjoholm RE. 2005. Chemical studies on antioxidant mechanisms and free radical scavenging properties of lignans. Org Bimol Chem. 21:3336–3347.
- Giannenas I, Pappas IS, Mavridis S, Kontopidis G, Skoufos J, Kyriazakis I. 2010. Performance and antioxidant status of broiler chickens supplemented with dried mushrooms (Agaricus bisporus) in their diet. Poult Sci. 89:303–311.
- Goyal M, Kalra KL, Sareen VK, Soni G. 2008. Xylanase production with xylan rich lignocellulosic wastes by a local soil isolate of Trichoderma viride. Braz J Microbiol. 39:535–541.
- Grigsby KN, Kerley MS, Paterson JA, Weigel JC. 1992. Site and extent of nutrient digestion by steers fed a low-quality bromegrass hay diet with incremental levels of soybean hull substitution. J Anim Sci. 70:1941–1949.
- Harman GE, Howell CR, Viterbo A, Chet I, Lorito M. 2004. Trichoderma species-opportunistic, avirulent plant symbionts. Nat Rev Microbiol. 2:43–56.
- Jiang ZY, Jiang SQ, Lin YC, Xi PB, Yu DQ, Wu TX. 2007. Effects of soybean isoflavone on growth performance, meat quality, and antioxidation in male broilers. Poult Sci. 86:1356–1362.
- Kalogeris E, Christakopoulos P, Katapodis P, Alexiou A, Vlachou S, Kekos D, Macris BJ. 2003. Production and characterization of cellulolytic enzymes from the thermophilic fungus Thermoascus aurantiacus under solid state cultivation of agricultural wastes. Process Biochem. 38:1099–1104.
- Krinsky NI. 1992. Mechanism of action of biological antioxidants. Proc Soc Exp Biol Med. 200:248–254.
- Kujala TS, Loponen JM, Klika KD, Pihlaja K. 2000. Phenolics and betacyanins in red beetroot (Beta vulgaris) root: distribution and effect of cold storage on the content of total phenolics and three individual compounds. J Agric Food Chem. 48:5338–5342.
- Lai LP, Lee MT, Chen CS, Yu B, Lee TT. 2015. Effects of co-fermented Pleurotus eryngii stalk residues and soybean hulls by Aureobasidium pullulans on performance and intestinal morphology in broiler chickens. Poult Sci. 94:2959–2969.
- Lara LJ, Rostagno MH. 2013. Impact of heat stress on poultry production. Animals (Basel). 3:356–369.
- Luo D, Yang F, Yang X, Yao J, Shi B, Zhou Z. 2009. Effects of xylanase on performance, blood parameters, intestinal morphology, microflora and digestive enzyme activities of broilers fed wheat-based diets. Asian-Aust J Anim Sci. 22:1288–1295.
- Mastouri F, Bjorkman T, Harman GE. 2012. Trichoderma harzianum enhances antioxidant defense of tomato seedlings and resistance to water deficit. Mol Plant Microbe Interact. 25:1264–1271.
- Miller GL. 1959. Use of dinitrosalicylic acid reagent for determination of reducing sugar. Anal Chem. 31:426–428.
- Nian F, Guo YM, Ru YJ, Péron A, Li FD. 2011. Effect of xylanase supplementation on the net energy for production, performance and gut microflora of broilers fed corn/soy-based diet. Asian-Australas J Anim Sci. 24:1282–1287.
- Noori S. 2012. An overview of oxidative stress and antioxidant defensive system. J Clin Cell Immunol. 1:413.
- Noy Y, Sklan D. 1995. Digestion and absorption in the young chick. Poult Sci. 74:366–373.
- National Research Council (NRC). 1994. Nutrient requirements of poultry. 9th Rev. ed. Washington, DC: National Academy Press.
- Oyaizu M. 1986. Studies on product of browning reaction prepared from glucose amine. Jpn J Nutr. 44:307–315.
- Pang PK, Darah I, Poppe L, Szakacs G, Ibrahim CO. 2006. Production of cellullolytic enzymes by a newly isolated, Trichoderma sp. FETL c3-2 via solid state fermentation grown on sugar cane baggase: palm kernel cake as substrates. Pak J Biol Sci. 9:1430–1437.
- Parsaie S, Shariatmadari F, Zamiri MJ, Khajeh K. 2007. Influence of wheat-based diets supplemented with xylanase, bile acid and antibiotics on performance, digestive tract measurements and gut morphology of broilers compared with a maize-based diet. Br Poult Sci. 48:594–600.
- Passos AA, Park I, Ferket P, von Heimendahl E, Kim SW. 2015. Effect of dietary supplementation of xylanase on apparent ileal digestibility of nutrients, viscosity of digesta, and intestinal morphology of growing pigs fed corn and soybean meal based diet. Anim Nutr. 1:19–23.
- Pinheiro DF, Cruz VC, Sartori JR, Vicentini Paulino ML. 2004. Effect of early feed restriction and enzyme supplementation on digestive enzyme activities in broilers. Poult Sci. 83:1544–1550.
- Pluske JR, Kim JC, McDonald DE, Pethic DW, Hampson DJ. 2001. Non-starch polysaccharides in the diets of young weaned piglets. In: The Weaner Pig: nutrition and management. Wallingford: CABI Publishing; p. 81–112.
- SAS Institute (SAS). 2004. SAS/STAT guide for personal computers. Version 9.01. SAS Inst. Inc., Cary, NC.
- Singh HB, Singh BN, Singh SP, Nautiyal CS. 2010. Solid-state cultivation of Trichoderma harzianum NBRI-1055 for modulating natural antioxidants in soybean seed matrix. Bioresour Technol. 101:6444–6453.
- Shimada K, Fujikawa K, Yahara K, Nakamura T. 1992. Antioxidative properties of xanthan on the autoxidation of soybean oil in cyclodextrin emulsion. J Agric Food Chem. 40:945–948.
- Sugiharto S, Yudiarti T, Isroli I. 2015. Functional properties of filamentous fungi isolated from the Indonesian fermented dried cassava, with particular application on poultry. Mycobiology. 43:415.
- Viveros A, Chamorro S, Pizarro M, Arija I, Centeno C, Brenes A. 2011. Effects of dietary polyphenol-rich grape products on intestinal microflora and gut morphology in broiler chicks. Poult Sci. 90:566–578.
- Wang CC, Lin LJ, Chao YP, Chiang CJ, Lee MT, Chang SC, Yu B, Lee TT. 2017. Antioxidant molecular targets of wheat bran fermented by white rot fungi and its potential modulation of anti-oxidative status in broiler chickens. Br Poult Sci. DOI:10.1080/00071668.2017.1280772
- Wang ZR, Qiao SY, Lu WQ, Li DF. 2005. Effects of enzyme supplementation on performance, nutrient digestibility, gastrointestinal morphology, and volatile fatty acid profiles in the hindgut of broilers fed wheat-based diets. Poult Sci. 84:875–881.
- Weiss SJ. 1986. Oxygen, ischemia and inflammation. Acta Physiol Scand Suppl. 548:9–37.
- Wu XJ, Hansen C. 2008. Antioxidant capacity, phenolic content, and polysaccharide content of Lentinus edodes grown in whey permeate-based submerged culture. J Food Sci. 73:1–8.
- Xu ZR, Hu CH, Xia MS, Zhan XA, Wang MQ. 2003. Effects of dietary fructooligosaccharide on digestive enzyme activities, intestinal microflora and morphology of male broilers. Poult Sci. 82:1030–1036.
- Yadav M, Yadav A, Yadav J. 2014. In vitro antioxidant activity and total phenolic content of endophytic fungi isolated from Eugenia jambolana Lam. Asian Pac J Trop Biomed. 7:S256–S261.
- Yang F, Hou C, Zeng X, Qiao S. 2015. The use of lactic acid bacteria as a probiotic in swine diets. Pathogens. 4:34–45.