Abstract
The objective of this experiment was to investigate the effects of dietary supplementation of different levels of soybean oil and exogenous lipase on growth performance, serum biochemical indices and caecal microbial diversity and similarity in weaning pigs. A total of 504 weanling pigs (252 males and 252 females) were randomly assigned to four treatments, with six replicates per treatment and 21 pigs per replicate. Four dietary treatments were arranged in a 2 × 2 factorial arrangement in a completely randomised experiment. The first factor was soybean oil level (2.5% or 5%), and the second factor was lipase level (0 or 200 g/t). In the first 14 days, compared to piglets fed 2.5% soybean oil diets, dietary supplementation of 5% soybean oil improved feed to gain ratio (F/G) (p < .05). On the 14th day, compared to piglets fed 2.5% soybean oil diets, dietary supplementation of 5% soybean oil increased high-density lipoprotein, reduced serum triglycerides and low-density lipoproteins (p < .05). On the 28th day, dietary supplementation of 5% soybean oil increased serum cholesterol and high-density lipoproteins (p < .05). The crypt depth of the duodenum was greater in pigs fed 200 g/t lipase diets compared to those fed non-lipase diets (p < .05). By increasing dietary soybean oil level, bacterial diversity in the caecum was reduced (p < .05). Results from this experiment suggest dietary supplementation of 5% soybean oil could improve growth performance by regulating the glycolipid metabolism and diversity of bacterial in weaning piglets compared to performance on a dietary level of 2.5% soybean oil.
Introduction
Dietary fat, composed of fatty acids and glycerol, is a valuable source of energy and can provide essential fatty acids (linoleic acid, linolenic acid and arachidonic acid) for swine. The actual value of a fat source in providing essential or beneficial fatty acids depends on its’ fatty acid profile which varies among and within sources. Dietary fat is widely used in swine husbandry due to its’ nutritional benefits. Firstly, lipids have high energy content (Batorek-Lukač et al. Citation2016). Secondly, both animal and vegetable fats are highly digestible in swine (Lindblom et al. Citation2017). Thirdly, increasing lipid levels linearly reduces the digesta passage rate (or increases mean digesta retention time) which enhances the digestibility of other nutrients (Mateos et al. Citation1982). Finally, dietary fats enhance the absorption rate of vitamin A, vitamin K, vitamin E and other fat-soluble vitamins (Harrison Citation2005). When low-energy feed ingredients are used, extra fat supplementation is a nutritionally efficient way to increase the dietary energy content. Weaning piglets have fast growth rates, but relatively compact gastrointestinal (GI) tracts and immature digestive systems. Increasing dietary energy content with supplemental fat using appropriate dietary fat sources and concentrations is an efficient way to better insure sufficient energy intake for weaning piglets.
Lipids are a vital component of sow’s milk, providing a large amount of the preweaning calories and reducing the weaning stress in piglets (Campbell et al. Citation2013). Dietary fat is largely hydrolysed in the small intestine by lipolytic enzymes secreted from the pancreas. Pancreatic digestive enzyme activity in piglets from 0 to 4 weeks is significantly increased as piglets start receiving nourishment by suckling (Zhu et al. Citation2012). However, lipase activity in the pancreas is dramatically decreased in the first week post-weaning associated with weaning stress (Hedemann and Jensen Citation2004). Therefore, the addition of exogenous lipolytic enzymes could be an alternative way to compensate for the deficiencies of endogenous lipolytic enzymes during the early post-weaning period. Given the importance of dietary fat and the possible insufficiencies in lipase production during the early post-weaning phase, the objective of this experiment was to investigate the effects of dietary supplementation of different levels of soybean oil and exogenous lipase on growth performance, visceral organ indices, pancreatic lipase activity, serum biochemical indices, and caecal microbial diversity and similarity in weaning pigs.
Materials and methods
Animal care and use
All animal protocols conducted in this research were approved by the South China Agricultural University Animal Care and Use Committee (SCAU-AEC-2010-0416). This experiment was conducted at a commercial pig farm in Jiangmen, Guangzhou (Guangdong Changjiang Food Co., Ltd).
Experimental design
The experiment was conducted as a 2 × 2 factorial arrangement in a completely randomised design. The first factor was the level of soybean oil (2.5% vs. 5%), and the second factor was the level of the lipolytic enzyme (0 vs. 200 g/t). A total of 504 growing pigs (Duroc × Landrace × Yorkshire; 6.36 ± 0.22 kg, 252 males and 252 females) were randomly allocated and stratified by body by weight and gender to 1 of 4 dietary treatments as follows: (1) 2.5% soybean oil +0 g/t lipase, (2) 2.5% soybean oil +200 g/t lipase (3) 5% soybean oil +0 g/t lipase, (4) 5% soybean oil +200 g/t lipase. There were 6 pens per treatment with 21 pigs per pen. The experimental period lasted for 28 d and was divided into two equal phases. The first phase was from d 1 to d 14, and the second phase was from d 15 to d 28. The enzyme complex was provided by Youteer Biochemistry Co., Ltd. (Hunan, China), which contained 30,429 U/g lipase.
Diet and management
The experimental diets were corn–soybean basal diets and were formulated to meet the nutrient requirements of swine (NRC Citation2012). The composition and nutrient content of treatment diets are presented in Table . Each pen was equipped with a one-sided stainless-steel feeder and a nipple drinker that allowed pigs ad libitum access to feed and water according to normal operating procedures of a commercial pig farm.
Table 1. Composition and analysed nutrient content of experimental diets (%, as-fed basis).
Data and sample collection
Body weight per pen was measured on d 1, d 14 and d 28, respectively, to calculate average daily gain (ADG). Feed was weighed and provided daily and consumption was determined on d 14 and d 28 by weighing the feed remaining in the feeder. Average daily feed intake (ADFI) and feed to gain ratio (F/G) of each phase were calculated. The occurrences of health incidents and mortality per pen were also recorded daily.
On d 14 and d 28, at 8:00 am after a 12-h fast-period, one pig approximating pen average body weight was selected from each pen and 5 mL blood per pig was sampled via anterior vena cava puncture in non-heparinized tubes. The blood remained at room temperature for 1 h to allow the clot to form. Samples were centrifuged at 3000 rpm for 15 mins, and serum was pipetted into plastic vials. Serum samples were immediately frozen and stored at −80 °C until analysis. At the end of the experiment, one pig approximating pen average body weight from each pen was selected and sacrificed for estimation of visceral organ indices, intestinal morphology, pancreatic lipase activity and caecal microbial diversity and similarity.
Visceral organ indices
To calculate the cardiac index, liver index, kidney index, pancreatic index and spleen index, heart, liver, pancreas, kidney and spleen weights were obtained immediately after sacrifice. The specific visceral organ index (g/kg) was calculated as visceral organ weight (g)/final body weight before slaughtering (kg).
Blood biochemical indices
Blood biochemical indices were determined using commercial kits (Biosino Bio-Tech, Beijing, China) for glucose (GLU), triglycerides (TG), total cholesterol (CHOL), high-density lipoprotein cholesterol (HDL-C) and low-density lipoprotein cholesterol (LDL-C) according to manufacturer’s instructions.
Intestinal morphology
Samples from each intestinal segment were embedded in paraffin, cut into serial sections, and stained with hematoxylin-eosin for identification. Villi height (V), crypt depth (C) and villi height/crypt depth (V/C) were determined according to the procedure describe by Zhang et al. (Citation2014). Briefly, five well-oriented villi and their associated crypt per section were selected and measured under a light microscope at 40 × magnification and analysed with an Image Analyzer.
Pancreatic lipase activity
Pancreatic lipase activity was determined by using Lipase Assay Kits (Nanjing Jianchen Bioengineering Institution, Nanjing, China) according to manufacturer’s instructions.
DNA isolation and PCR-DGGE analysis
Microbial DNA of the colonic digesta was extracted for denaturing gradient gel electrophoresis (DGGE) analysis according to the instructions of a Stool DNA Kit (Tiangen Biotech, Beijing, China). The bacterial universal V3 region of the 16S RNA gene was amplified by using the following primers: the Muyzer primer F (5′-CGCCCGCCGCGCGCGGCGGGCGGGGCGGGGGCAC GGGGGGCCTACGGGAGGCAGCAG-3′) (including GC clamp) and the Muyzer primer R (5′-ATTACCGCGGCTGCTGG-3′). In brief, the PCR mixture contained 5 μL of genomic DNA, 10 μM (1.5 μL) of each primer and 25 mL EX Taq PCR (Takara, Shiga, Japan). The final volume was adjusted to 50 μL with sterile deionised water. The PCR analysis was conducted as following procedure. The procedure included 5 min at 94 °C; 30 cycles of 30 s at 94 °C, 50 s at 55 °C, and 120 s at 72 °C. The PCR products were analysed by electrophoresis on 1.0% (w/v) agarose gel.
DGGE was performed for separation of PCR amplicons on 8% polyacrylamide gels with a vertical gradient of denaturants of 45–60%. Electrophoresis runs were done for 14 h at 80 V in 1 x TAE buffer at a constant temperature of 60 °C using a DCodeTM Universal Mutation Detection System (Bio-Rad Richmond, CA, USA). DGGE gels were stained by silver for 10 min at room temperature, and photographed with Image LabTM Software (Bio-Rad Richmond, CA) and analysed by Quantity One Basic (Bio-Rad Richmond, CA). Interesting bands that demonstrated differentiation in the denaturing gradient gel electrophoresis profiles were excised and sent for sequencing (Sangon Biotech, Shanghai, China). Homology searches were performed using the BLAST programme in the NCBI database (http://blast.ncbi.nlm.nih.gov).
Statistical analysis
Statistical analysis was done using the SPSS 17.0 software (SPSS Inc, Chicago, IL). Experimental data were averaged across pigs within pen and analysed as a completely randomised design with a 2 × 2 factorial arrangement of treatments. Data were analysed using a mixed model with soybean oil level, lipase level, soybean oil level × lipase level as fixed effects and among pens nested in soybean and lipase levels as random error. Growth data were analysed by period (1–14 d, 15–28 d, 1–28 d) and serum biochemical indices were analysed by day (14 d, 28 d). Pairwise tests of hypotheses were done using LSD multiple range analysis. Effects were considered significant at p < .05 and p < .1 was considered as a trend.
Results
Growth performance
Growth performance of weanling pigs is presented in Table . In the first 14 days, compared to piglets fed 2.5% soybean oil diets, dietary supplementation of 5% soybean oil improved F/G (p < .05) with a strong trend to increase ADG (p < .10). However, extra supplementation of 200 g/t lipase had no effect on ADG, ADFI or F/G (p > .05). From d 15 to d 28, no difference was observed in ADG, ADFI and F/G among the four treatments (p > .05). In the entire experimental period, compared to pigs fed the 2.5% soybean oil +0 g/t lipase diet, F/G was improved when pigs were fed the 5% soybean oil +200 g/t lipase diet (p < .05).
Table 2. Effects of soybean oil and lipase supplementation on growth performance of weanling pigs.
Serum biochemical indices
Serum biochemical indices are shown in Table . At 14 d, lipase supplementation decreased HDL-C and LDL-C at 2.5% soybean oil (p < .05) but not in 5% soybean oil. There was no evidence of lipase × oil interactions for other traits at 14 d (p > .05). Averaged over lipase levels, increased oil supplementation tended to increase GLU (p < .10) and decrease TG (p < .05). At 28 d, there was no evidence of lipase × oil interaction for any trait (p > .05). At day 28, averaged over lipase levels, increase oil supplementation increased CHOL and HDL-C (p < .05).
Table 3. Effects of soybean oil and lipase supplementation on serum biochemical indices of weanling pigs.
Visceral organ indices
Results for visceral organ indices are shown in Table . The relative weights of the heart, liver, kidney, pancreas and spleen were not affected by either soybean oil or lipase levels (p > .05).
Table 4. Effects of soybean oil and lipase supplementation on visceral organ index of weanling pigs (% of body weight).
Pancreatic lipase activity
Pancreatic lipase activity is presented in Table . Supplementation with soybean oil and/or lipase has no effect on pancreatic lipase in weaning piglets (p > .05).
Table 5. Effects of soybean oil and lipase supplementation on pancreatic lipase activity of weanling pigs (U/g prot).
Intestinal morphology
Intestinal morphology of the duodenum, jejunum and ileum are shown in Table . The dietary supplementation of 5% soybean oil decreased the duodenum villous height in piglets compared to those fed 2.5% soybean oil diets (p < .05). The crypt depth of duodenum was greater when piglets were fed 2.5% soybean oil +200 g/t lipase diet compared to those fed 5% soybean oil +0 g/t lipase diet (p < .05) and there was a trend for the crypt depth of jejunum to be greater in the 200 g/t lipase diet compared to those fed 0 g/t lipase (p < .10).
Table 6. Effects of soybean oil and lipase supplementation on intestinal morphology of weanling pigs.
Caecal microbial diversity and similarity
Representative results of the DGGE fingerprints are shown in Figure . Results for microbial diversity and similarity in the caecum are given in Table . Compared to the 2.5% soybean oil treatments, dietary supplementation of 5% soybean oil reduced the band number and diversity of the microbial community in the caecum (p < .05). Compared to 0 g/t lipase treatments, supplementation of 200 g/t lipase tended to increase the diversity of the microbial community in the caecum (p < .10). By gel extraction, most of the strong signal fragments on the denaturing gradient gels were detected and shown to be uncultured microorganisms (Table ).
Figure 1. Example of DGGE fingerprints of PCR products of V3 regions of 16S rDNA from caecal digesta samples taken from piglets. (A) 2.5% soybean oil +0 g/t lipase; (B) 2.5% soybean oil +200 g/t lipase; (C) 5% soybean oil +0 g/t lipase; (D) 5% soybean oil +200 g/t lipase. Arrows (1–16) indicate excised bands that were re-amplified (Table ).
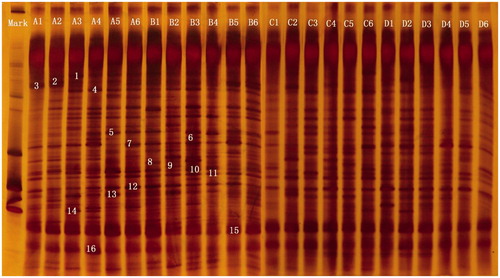
Table 7. Effects of soybean oil and lipase supplementation on the microbial communities in the colon of weanling pigs.
Table 8. Clone relatives to sequences retrieved from the DGGEs bands from the colon digesta of weanling pigs.
Discussion
Effects of supplementation with dietary fat on ADG and feed efficiency in weanling pigs are not consistent in the literature. One study reported dietary fat supplementation (soybean oil) improved ADG and ADFI of weaning pigs (Zeng et al. Citation2012). In other studies, the addition of dietary fat (canola oil) to wheat-soybean diets enhanced F/G but had little effect on ADG (Omogbenigun et al. Citation2004). Some studies reported that neither ADG nor F/G of weaning pigs was enhanced with the supplementation of dietary fat (corn oil) (Cera et al. Citation1988b). In our study, adding 5% soybean in the diet of weanling pig improved F/G and tended to increase ADG from d 1 to d 14 with a trend in improved feed efficiency over the 28 d of the study. However, little difference was observed among the four treatments, which might due to the normal pancreatic endogenous lipase level after the early weaning period and gradually decreased energy requirement of weaning pigs as the pigs are growing. Differences in research results might be attributed to different dietary fat sources or fat concentrations (Frobish et al. Citation1970; Cera et al. Citation1988a) and various weaning ages of piglets used in the studies (Cera et al. Citation1988c).
Vegetable fat has been shown to have higher digestibility than animal fat (e.g. lard or tallow), attributed to the greater percentage of long chain and unsaturated fatty acids (Cera et al. Citation1988c). Therefore, soybean oil used in this research could contribute to better growth performance in this study compared with other studies. It worth mentioning that, in this study, the environmental temperature was lower than is conventional. To decrease the weaning stress when transferring piglets from delivery room to nursing room, piglets were weaned at 31 d. Our results might also be attributed to the comparatively delayed weaning time of piglets in this experiment, leading to a better development of the GI tract and greater pancreatic lipase activity. Well-developed GI systems might explain the limited effects of dietary lipase on growth performance in this experiment.
The increase in blood glucose when feeding piglets with higher-dose fat diets or lipase supplemented diets was consistent with expectations. Fat metabolism affects glucose metabolism and insulin levels in animals (Lichtenstein and Schwab Citation2000). Animals fed high-fat diet exhibited a decrease of activity of glucose transporter system and intracellular glucose metabolism (Olefsky and Saekow Citation1978). Also, a high-fat diet can result in insulin resistance in peripheral tissues (skeleton muscle and brown tissue) and liver (Storlien et al. Citation1986; Ruan et al. Citation2014; Liu et al. Citation2016). In this experiment, pigs fed extra lipase had higher serum GLU level on the 14th day, which may reflect a better utilisation of dietary soybean oil when lipase was supplemented in the diet during this period. However, apparent increases in lipid absorption did not enhance growth performance of piglets in this experiment. During the first 2 weeks after weaning, extra fat supplementation was reported to increase fat retention and decreased protein gain of piglets (Leibbrandt et al. Citation1975; Endres et al. Citation1988). From a carcase gain perspective, the deposition of protein is more efficient than fat as deposition of 1 g protein in the body is associated with the retention of 3 g water. Thus, protein growth in skeletal muscle is a major determinant of growth performance in young animals. These findings indicate that digested and absorbed fat is mostly stored as carcase fat, but might not be efficiently used as energy. Although the underlying mechanism is still unclear, the limited utilisation of fatty acids in the first 28 days after weaning could partly explain the lesser growth performance in piglets fed 5% soybean oil. In this experiment, adding 5% soybean oil increased the serum HDL-C and decreased the LDL-C of piglets on the 14th day and increased HDL-C on the 28th day. Generally, saturated FAs increase plasma LDL (Grundy Citation1987). Diets rich in linoleic acid and oleic acid appear to effectively raise HDL and slightly lower LDL concentration (Nestel et al. Citation1984; Katan et al. Citation1994). Soybean meal is composed of 22.8% oleic acid and 55.1% linoleic acid (NRC Citation2012), which might explain the decrease of serum LDL-C in 5% soybean oil compared with 2.5% soybean oil after 14 d on feed.
Dietary fat can cause sloughing of intestinal villi cells, impairing digestion immediately after weaning (Cera et al. Citation1988d). Similarly, in this trial, adding 5% soybean oil led to a decrease of duodenum villi height and crypt depth, which is consistent with results of previous studies (Cera et al. Citation1988d). The shortened and smooth villi surface could reduce total luminal villus absorptive area and result in inadequate brush border digestive enzymes. In this experiment, adding 5% soybean oil led to the decrease of the villus height only in the duodenum (which is a comparatively small percentage of the total length of the small intestine), but not in the jejunum or the ileum.
Pancreatic lipase, an important endogenous enzyme, mainly participates in fat absorption. Aumaitre and Corring (Citation1978) reported that, from birth to the age of 8 weeks, there was an increase in the total fresh weight of the exocrine pancreas and the level of pancreatic enzymes (trypsin, chymotrypsin, lipase, and amylase). The total luminal enzyme concentrations do not decline upon weaning when pigs are weaned at either 21 or 35 d of age (Cera et al. Citation1990). While enzyme supplementation may influence endogenous enzyme secretion, results of exogenous enzyme supplementation on the expression endogenous enzyme are conflicting (Fan et al. Citation2009; Zhang et al. Citation2014). Previous studies found that pancreatic lipase activity adapts to dietary fat level and responds to the types of fat only below the threshold level (22% in rat) of dietary fat (Gidez Citation1973); no additional increase in lipase levels can be obtained by any further dietary fat supplementation (Sabb et al. Citation1986). These results are likely influenced by diet composition and the dose of enzymes. Neither soybean oil nor lipase supplementation appeared to influence pancreatic lipase regulation in this study.
Visceral organs only account for 15% of total body weight, but account for almost 45% of the oxygen consumption of the whole body (Nyachoti et al. Citation2000). Diet composition can influence the mass of visceral organs (Pond et al. Citation1988; Jørgensen et al. Citation1996). Such effects might lead to a significant increase of energy expenditure in the visceral organs, decreasing the efficiency of converting dietary energy into edible pork products (Nyachoti et al. Citation2000). It was reported that animals on a high-nutrition levels have heavier weights of organs and a greater heat production than animals on low nutrient levels (Koong et al. Citation1982, Citation1985). However, little evidence of differences was observed in visceral organ indices among different treatments in this study since nutritional levels among the different treatments were similar, unlike the studies of Koong et al. (Citation1982) and Koong et al. (Citation1985).
The endogenous gastrointestinal microbial flora plays a fundamentally important role in gut health, immunity and nutrient utilisation (Stappenbeck et al. Citation2002; Bäckhed et al. Citation2004; Mennigen and Bruewer Citation2009). This study demonstrated that increasing dietary soybean oil supplementation from 2.5% to 5% decreased the caecal microbial diversity, which is in agreement with a previous report that microbial diversity decreased with the supplementation of fat (Clavel et al. Citation2014). Similar to other reported research (Laugerette et al. Citation2011). Current knowledge of bacterial diversity is largely based on the use of classical culture-dependent techniques. It is worth noting that most of the bands cut from DGGE gel were bacteria that have not been or cannot be cultured. Culture-based methods only identify a small percentage of bacteria colonising in the intestine since most bacteria are not cultivable (Wang et al. Citation2005). These unknown bacteria could have potential influence on gut function by regulating intestinal permeability, converting primary bile acids into secondary bile acids, impacting on the enterohepatic circulation of bile acids and fat absorption. More research is needed utilising high-throughput sequencing, metaproteome and metabolome analyses to better understand the influence of fat and enzyme supplementation on gut microbial diversity.
Conclusions
In conclusion, dietary supplementation of 5% soybean oil improved growth performance of piglets in the first two weeks after weaning. The effect of lipase supplementation on growth performance was limited in this study. More research is needed to better determine the optimal supplemental dosage and timing of exogenous lipase in piglets. The lack of interaction of soybean oil and lipase levels suggests that neither synergism nor antagonism between these two factors exists under the conditions of this research.
Disclosure statement
All authors declare that they have no competing interest in the present work.
References
- Aumaitre A, Corring T. 1978. Development of Digestive Enzymes in the Piglet from Birth to 8 Weeks. Nutr Metab (Lond). 22:231–243.
- Bäckhed F, Ding H, Wang T, Hooper LV, Koh GY, Nagy A, Semenkovich CF, Gordon JI. 2004. The gut microbiota as an environmental factor that regulates fat storage. Proc Natl Acad Sci USA. 101:15718–15723.
- Batorek-Lukač N, Dubois S, Noblet J, Čandek-Potokar M, Labussière E. 2016. Effect of high dietary fat content on heat production and lipid and protein deposition in growing immunocastrated male pigs. Animal. 10:1941–1948.
- Campbell JM, Crenshaw JD, Polo J. 2013. The biological stress of early weaned piglets. J Anim Sci Biotechnol. 4:19.
- Cera K, Mahan D, Cross R, Reinhart G, Whitmoyer R. 1988a. Effect of age, weaning and postweaning diet on small intestinal growth and jejunal morphology in young swine. J Anim Sci. 66:574–584.
- Cera K, Mahan D, Reinhart G. 1988b. Effects of dietary dried whey and corn oil on weanling pig performance, fat digestibility and nitrogen utilization. J Anim Sci. 66:1438–1445.
- Cera K, Mahan D, Reinhart G. 1988c. Weekly digestibilities of diets supplemented with corn oil, lard or tallow by weanling swine. J Anim Sci. 66:1430–1437.
- Cera K, Mahan D, Reinhart G. 1990. Effect of weaning, week postweaning and diet composition on pancreatic and small intestinal luminal lipase response in young swine. J Anim Sci. 68:384–391.
- Cera KR, Mahan DC, Cross RF, Reinhart GA, Whitmoyer RE. 1988d. Effect of age, weaning and postweaning diet on small intestinal growth and jejunal morphology in young swine. J Anim Sci. 66:574–584.
- Clavel T, Desmarchelier C, Haller D, Gérard P, Rohn S, Lepage P, Daniel H. 2014. High-fat diet alters gut microbiota physiology in mice. ISME JJ. 8:295–308.
- Endres B, Aherne FX, Ozimek L, Spicer H. 1988. The effects of fat supplementation on ileal versus fecal fat digestibilities, performance and body composition of weaned pigs. Can Vet J. 68:225–231.
- Fan C, Han X, Xu Z, Wang L, Shi L. 2009. Effects of β‐glucanase and xylanase supplementation on gastrointestinal digestive enzyme activities of weaned piglets fed a barley‐based diet. J Anim Physiol Anim Nutr (Berl). 93:271–276.
- Frobish L, Hays V, Speer V, Ewan R. 1970. Effect of fat source and level on utilization of fat by young pigs. J Anim Sci. 30:197–202.
- Gidez LI. 1973. Effect of dietary fat on pancreatic lipase levels in the rat. J Lipid Res. 14:169–177.
- Grundy SM. 1987. Monounsaturated fatty acids, plasma cholesterol, and coronary heart disease. Am J Clin Nutr. 45:1168–1175.
- Harrison EH. 2005. Mechanisms of digestion and absorption of dietary vitamin A. Annu Rev Nutr. 25:87–103.
- Hedemann MS, Jensen BB. 2004. Variations in enzyme activity in stomach and pancreatic tissue and digesta in piglets around weaning. Arch Anim Nutr. 58:47–59.
- Jørgensen H, Zhao XQ, Eggum BO. 1996. The influence of dietary fibre and environmental temperature on the development of the gastrointestinal tract, digestibility, degree of fermentation in the hind-gut and energy metabolism in pigs. Br J Nutr. 75:365–378.
- Katan MB, Zock PL, Mensink RP. 1994. Effects of fats and fatty acids on blood lipids in humans: an overview. Am J Clin Nutr. 60:1017S–1022S.
- Koong LJ, Ferrell CL, Nienaber JA. 1985. Assessment of interrelationships among levels of intake and production, organ size and fasting heat production in growing animals. J Nutr. 115:1383–1390.
- Koong LJ, Nienaber JA, Pekas JC, Yen JT. 1982. Effects of plane of nutrition on organ size and fasting heat production in pigs. J Nutr. 112:1638–1642.
- Laugerette F, Furet JP, Debard C, Daira P, Loizon E, Géloën A, Soulage CO, Simonet C, Lefils-Lacourtablaise J, Bernoud-Hubac N. 2011. Oil composition of high-fat diet affects metabolic inflammation differently in connection with endotoxin receptors in mice. Am J Physiol Endocrinol Metab. 302:E374–E386.
- Leibbrandt V, Ewan R, Speer V, Zimmerman DR. 1975. Effect of age and calorie: protein ratio on performance and body composition of baby pigs. J Anim Sci. 40:1070–1076.
- Lichtenstein AH, Schwab US. 2000. Relationship of dietary fat to glucose metabolism. Atherosclerosis. 150:227–243.
- Lindblom S, Dozier W, Shurson G, Kerr B. 2017. Digestibility of energy and lipids and oxidative stress in nursery pigs fed commercially available lipids. J Anim Sci. 95:239–247.
- Liu G, Aguilar YM, Zhang L, Ren WK, Chen S, Guan GP, Xiong X, Liao P, Li TJ, Huang RL, et al. 2016. Dietary supplementation with sanguinarine enhances serum metabolites and antibodies in growing pigs. J Anim Sci. 94:75–78.
- Mateos GG, Sell JL, Eastwood JA. 1982. Rate of food passage (transit time) as influenced by level of supplemental fat. Poult Sci. 61:94–100.
- Mennigen R, Bruewer M. 2009. Effect of probiotics on intestinal barrier function. Ann N Y Acad Sci. 1165:183–189.
- Nestel PJ, Connor WE, Reardon MF, Connor S, Wong S, Boston R. 1984. Suppression by diets rich in fish oil of very low density lipoprotein production in man. J Clin Invest. 74:82–89.
- National Research Council (NRC). 2012. Nutrient Requirements of Swine. 9th rev. ed. Washington (DC): National Academy Press.
- Nyachoti CM, de Lange CF, Mcbride BW, Leeson S, Gabert VM. 2000. Endogenous gut nitrogen losses in growing pigs are not caused by increased protein synthesis rates in the small intestine. J Nutr. 130:566–572.
- Olefsky JM, Saekow M. 1978. The effects of dietary carbohydrate content on insulin binding and glucose metabolism by isolated rat adipocytes. Endocrinology. 103:2252–2263.
- Omogbenigun FO, Nyachoti CM, Slominski BA. 2004. Dietary supplementation with multienzyme preparations improves nutrient utilization and growth performance in weaned pigs. J Anim Sci. 82:1053–1061.
- Pond WG, Jung HG, Varel VH. 1988. Effect of dietary fiber on young adult genetically lean, obese and contemporary pigs: body weight, carcass measurements, organ weights and digesta content. J Anim Sci. 66:699–706.
- Ruan Z, Yang YH, Zhou Y, Wen YM, Ding S, Liu G, Wu X, Liao P, Deng ZY, Assaad H, et al. 2014. Metabolomic analysis of amino acid and energy metabolism in rats supplemented with chlorogenic acid. Amino Acids. 46:2219–2229.
- Sabb JE, Godfrey PM, Brannon PM. 1986. Adaptive response of rat pancreatic lipase to dietary fat: effects of amount and type of fat. J Nutr. 116:892–899.
- Stappenbeck TS, Hooper LV, Gordon JI. 2002. Developmental regulation of intestinal angiogenesis by indigenous microbes via Paneth cells. Proc Natl Acad Sci USA. 99:15451–15455.
- Storlien LH, James DE, Burleigh KM, Chisholm DJ, Kraegen EW. 1986. Fat feeding causes widespread in vivo insulin resistance, decreased energy expenditure, and obesity in rats. Am J Physiol. 251:E576–E583.
- Wang M, Ahrné S, Jeppsson B, Molin G. 2005. Comparison of bacterial diversity along the human intestinal tract by direct cloning and sequencing of 16S rRNA genes. FEMS Microbiol Ecolo. 54:219–231.
- Zeng Z, Yu B, Mao X, Chen D. 2012. Effects of dietary digestible energy concentration on growth, meat quality, and PPARγ gene expression in muscle and adipose tissues of Rongchang piglets. Meat Sci. 90:66–70.
- Zhang G, Yang Z, Wang Y, Yang W, Zhou H. 2014. Effects of dietary supplementation of multi-enzyme on growth performance, nutrient digestibility, small intestinal digestive enzyme activities, and large intestinal selected microbiota in weanling pigs. J Anim Sci. 92:2063–2069.
- Zhu LH, Zhao KL, Chen XL, Xu JX. 2012. Impact of weaning and an antioxidant blend on intestinal barrier function and antioxidant status in pigs. J Anim Sci. 90:2581–2589.