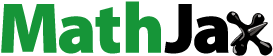
Abstract
The effect of increasing dietary doses of lactose on canine faecal microbiota and apparent digestibility was evaluated. Fourteen adult healthy dogs [1–5 years of age, mean body weight (BW) of 19.0 kg] were fed with an extruded diet containing silica (5 g/kg) as a digestion marker. After a 20 d adaptation period, increasing doses of lactose were added to the dogs’ diet (0.5, 1 and 2 g/kg BW0.75/d) during three consecutive 20-d supplementation periods. Faeces were collected at the end of each period for analyses. Four dogs refused the diet added with lactose at 0.5 g/kg BW0.75/d and were excluded from the trial, as well as two dogs, which developed acute diarrhoea when lactose was fed at 1 g/kg BW0.75/d. Conversely, eight dogs remained healthy throughout the study. Faecal moisture was influenced by lactose (quadratic, p = .001), while faecal pH and ammonia were not affected by treatments. Lactose supplementations tended to linearly decrease isovalerate (p = .051) and quadratically influence n-valerate (p = .056) in canine faeces. No changes in faecal microbial populations were observed. Apparent digestibility of dry matter, Ca, K, Mn and Fe was influenced by lactose supplementation (quadratic, p < .05). Increasing doses of lactose linearly decreased Mg digestibility (p < .05). Furthermore, coefficients of crude protein, crude ash, P, Mg and Zn digestibility were tendentially affected (quadratic, p = .055, .089, .091, .065 and .065, respectively). In conclusion, 8 of 14 dogs displayed a good tolerance (absence of gastrointestinal signs) up to the highest dose of lactose (2 g/kg BW0.75/d). An evident prebiotic effect was not observed.
Introduction
Lactose, a disaccharide composed of glucose and galactose, represents the major carbohydrate in the milk of most mammals, acting as a fundamental source of energy for suckling animals and playing an important role (galactose, in particular) in brain development (Fox et al. Citation2015).
In newborns, ingested lactose is hydrolysed in the small intestine by the lactase-phlorizin hydrolase, an endogenous sz-galactosidase present in the brush border membrane of epithelial cells, which favours an efficient absorption of the two constituent monosaccharides through the intestinal mucosa during the suckling period (Venema Citation2012).
After weaning, in most mammals a genetically programmed decrease in lactase activity gradually occurs. In human species, for example, about the 75% of the world adult population displays this enzymatic reduction, which is strongly linked to ethnicity (Corgneau et al. Citation2015). Lactose maldigestion could lead to a lactose-intolerance condition characterised by intensive bacterial fermentations in the colon, which lead to a huge production of fermentative compounds such as short-chain fatty acids (SCFA) and gasses (mainly carbon dioxide, hydrogen and methane) (Venema Citation2012). The consequent appearance of the characteristic symptoms such as abdominal pain, bloating and osmotic diarrhoea depends not only by the residual lactase activity in the small intestine, but also by several factors such as the dose of lactose ingested and the individual visceral sensitivity (Venema Citation2012). While many studies have been conducted to improve the knowledge on the lactose tolerance threshold in humans (Corgneau et al. Citation2015), little is known about these concerns in companion animals. In particular, beyond some evidences on lactose intolerance of adult dogs and cats when fed diets containing high concentrations of lactose (Bennett and Coon Citation1966; Morris et al. Citation1977; Kienzle Citation1993), few studies have evaluated the effect of adding lower (and more plausible) daily doses of this sugar to companion animals’ diet (Zentek et al. Citation2002; Beynen and Yu Citation2003).
Furthermore, there are evidences that the undigested lactose reaching the hindgut could exert beneficial effects on the gastrointestinal environment, by providing energy for intestinal lactic acid-producing bacteria (Szilagyi Citation2004).
At present, the prebiotic potential of lactose has been estimated in farm animal species such as pigs (Pierce et al. Citation2007; Molino et al. Citation2011) and poultry (Totton et al. Citation2012), but there is a lack of evidences, in this regard, in companion animals like dogs.
Lactose is not traditionally used in pet-food formulations. Nonetheless, whey powder, a low-cost and highly available dairy by-product rich in this sugar and in high-quality proteins, typically used in livestock diets (in particular, in piglets; Mahan et al. Citation2004) and characterised by a pleasant sweet taste, could represent an interesting ingredient for pet-food companies.
The aim of the present study was to evaluate in healthy adult dogs the effects of increasing moderate dietary lactose supplementations on some faecal microbial populations, faecal fermentative end-products and apparent total tract digestibility (ATTD) of the diets. We hypothesised that feeding lactose to dogs would exert a prebiotic effect on their intestinal microbiota.
Material and methods
The study was carried out accordingly to the Italian legislation implementing the European Council Directive 2010/63 on the protection of animals used for scientific purposes. The experimental protocol was reviewed and approved by the Scientific Ethics Committee on Animal Experimentation of the University of Bologna before initiation of the experiment. Informed consent was obtained from all dog owners prior to the beginning of the study.
Animals
Fourteen healthy adult dogs (household dogs, different breeds and living in different environments; between 1 and 5 years of age) were used. The average body weight (BW) ± standard deviation (SD) of the dogs was 19.0 ± 7.6 kg. Each dog was regularly vaccinated, periodically dewormed and had exhibited no clinical signs of gastrointestinal disorders during the previous 12 months. Furthermore, all the dogs were usually fed with commercial diets not containing dairy products among their ingredients.
Diet, feeding trial and samples collection
During a first 20-d adaptation period, dogs were fed with a basal diet consisting in a complete dry extruded food for adult dogs (Effeffe Pet Food S.p.A., Padua, Italy) (Table ), containing dried poultry meat, rice and other cereals, oils and fat, dried eggs by-products, vitamins and minerals and not including any prebiotic ingredient. Silica was included at the dose of 5 g/kg as a source of acid-insoluble ash to be used as digestion marker. After the adaptation period, three increasing concentrations of a lactose powder (lactose monohydrate 99% min.; Brenntag S.p.A., Milan, Italy) were individually dosed for each dog (0.5, 1 and 2 g/kg BW0.75/d) and mixed with the basal diet during three consecutive 20-d supplementation periods (lactose was spread over the kibbles together with a little amount of water to ensure the adhesion of the lactose powder). Dogs were fed twice a day. For this reason, for each dog, the daily dose of lactose was divided and added equally to each of the two planned daily meals. The daily food amount for each dog was calculated on the basis of the energy content of the basal diet and the animals’ daily energy requirements, accordingly to the recommendations for the maintenance of small and medium sized adult dogs: 0.55 MJ/kg BW0.75 [National Research Council (NRC) Citation2006].
Table 1. Chemical composition (g/kg dry matter) of the basal extruded diet fed to dogs during the study.
Each dog owner was asked to avoid any other food or snack and report any modification in faecal quality and BW occurred during the trial.
The experimental design performed during present investigation was addressed to avoid rapid dietary changes in animals. Undoubtedly, a Latin square/crossover design would have been more appropriate in order to withdraw the potential ‘time-effect’, but it would have presented potential ethical limits, as ingesting lactose without an adequate dietary adaptation would have possibly produced undesirable intestinal effects in the dogs. For the same reason, also a wash-out period between the three supplementation periods would have probably exerted detrimental consequences.
From each dog, at the end of the adaptation period (Day 20) and after each supplementation period (at 40, 60 and 80 d) a fresh faecal sample was collected within 15 min from defaecation and frozen at −80 °C for chemical (pH, water content, ammonia, SCFA and biogenic amines) and microbial analyses. Faeces excreted by each dog during the last 5 d of the adaptation period and each supplementation period were collected, pooled and stored at −20 °C for chemical analyses, in order to assess ATTD of the diets.
Chemical analyses and apparent digestibility calculation
Analysis of nutrients in the diet and faecal samples was performed accordingly to the Association of Official Analytical Chemists international standard methods (AOAC Citation2000; method 950.46 for water, method 954.01 for crude protein, method 920.39 for ether extract, method 920.40 for starch, method 942.05 for crude ash, method 962.09 for crude fibre). Acid-insoluble ash was determined according to Vogtmann et al. (Citation1975). For the quantification of minerals, samples were previously diluted with a nitric acid solution (15 M) and processed through microwave mineralisation. Analyses were carried out by inductively coupled plasma-optical emission spectrometry (ICP-OES Optima 2100; PerkinElmer, Waltham, MA). In particular, macrominerals quantification was performed with the torch in radial position and by using a Meinhard nebuliser coupled with a cyclonic spray chamber, while trace elements were assessed with the torch in axial position and with an ultrasonic wave nebuliser (CETAC U5000; Teledyne Cetac Technologies, Omaha, NE). The accuracy of the analytical results was assessed by using an internal standard [Standard Reference Material (SRM) 1546-Meat Homogenate; National Institute of Standards and Technology (NIST), Gaithersburg, MD].
The coefficient of ATTD of dry matter (DM) in diets supplemented with increasing doses of lactose was calculated using the following equation:
The ATTD of each nutrient (macronutrients, macrominerals and trace elements) was calculated using the following equation:
{100–[% nutrient in faeces× (100–% DM digestibility)/% nutrient in the diet]}/100
Faecal pH was measured after diluting samples 1:10 with distilled water. Ammonia was measured using a commercial kit (Urea/BUN–Colour; BioSystems S.A., Barcelona, Spain). The SCFA were analysed according to Biagi et al. (Citation2006). For the determination of biogenic amines, samples were diluted 1:5 with perchloric acid (0.3 M); biogenic amines were later separated by high-performance liquid chromatography and quantified through fluorimetry.
Microbial analyses
Bacterial genomic DNA was extracted and isolated from faecal samples (∼200 mg) using a commercially available kit (QIAamp DNA Stool Mini-Kit; QIAGEN GmbH, Hilden, Germany), according to the manufacturer’s protocol. Isolated DNA concentration and purity were assessed on the basis of spectrophotometric measurements. Template DNA was diluted to 50 ng/μl and stored at −20 °C until further analysis. Quantitative polymerase chain reaction (qPCR) was performed in a real-time PCR instrument (CFX96 Touch thermocycler; Bio-Rad, Hercules, CA) using specific primers for total bacteria, Bifidobacterium genus, Lactobacillus genus, Enterococcus genus and Clostridium perfringens (Table ).
Table 2. Primers used for quantitative polymerase chain reaction analysis.
Amplification was performed in duplicate for each bacterial group within each sample. For amplification, 15 μl final volume containing 7.5 µl of a PCR Master Mix (2× SensiFAST No-ROX PCR Master Mix; Bioline GmbH, Luckenwalde, Germany), 4.8 µl of nuclease-free water, 0.6 µl of each 10 pmol primer and 1.5 µl of template DNA were used. After an initial denaturation at 95 °C for 2 min, the amplification cycle was carried out as follows: 95 °C for 5 s, primer annealing at 55–61 °C for 10 s and at 72 °C for 8 s. The cycle was repeated 40 times. Cycle threshold values were plotted against standard curves for quantification of the target bacterial DNA from faecal samples. For generation of standard curves, 10-fold serial dilutions of purified and quantified PCR products were used. The standard curves of the individual qPCR assays were obtained by PCR using specific primers (Table ) and DNA extracted from the faecal samples. Individual reactions of the standard curves were run in duplicate on each plate for the respective bacterial group. Melting curves were checked after amplification to ensure single product amplification of consistent melting temperature. Results were reported as log10 16S ribosomal DNA gene copies/g fresh matter.
Statistical analysis
Data from dogs that did not end the study were not included in the statistical analysis. Results from microbiological and chemical analysis of canine faecal samples (including ATTD data) collected at the end of each feeding period were analysed by one-way analysis of variance (ANOVA) for repeated measures, with dietary lactose concentration as main factor. Linear and quadratic contrasts were used to determine the nature of the response to the feeding of increasing doses of lactose. Significance and tendency for statistical tests were set at p < .05 and .05 ≤ p ≤ .10, respectively. All the statistical computations were performed with Statistica 10.0 (Stat Soft Italia, Padua, Italy).
Results
During the present study, 6 of the 14 dogs involved at the beginning of the trial were excluded for ethical reasons. In particular, four dogs refused the diet added with lactose at the daily dose of 0.5 g/kg BW0.75 and two dogs developed acute diarrhoea within 24 h from the first ingestion of the diet containing lactose at the dose of 1 g/kg BW0.75/d. Eight dogs concluded the experiment, consuming the entire daily ration provided, without exhibiting palatability problems and remaining healthy throughout the supplementation periods, displaying a good tolerance (absence of gastrointestinal signs) up to the highest dose of lactose (2 g/kg BW0.75/d).
Faecal moisture, pH and concentrations of ammonia, SCFA and biogenic amines are presented in Table . Lactose supplementation influenced water content in faecal samples (quadratic, p = .001). Conversely, dietary treatments did not affect faecal pH and ammonia concentration. Increasing doses of lactose tended to linearly decrease faecal concentrations of isovalerate (linear, p = .051) and to affect faecal concentrations of n-valerate (quadratic, p = .056), but did not influence the faecal concentrations of total SCFA.
Table 3. Chemical analysis of faecal samples from dogs (n = 8) fed increasing doses of lactose.
No changes in faecal microbial populations were observed during lactose supplementation (Table ). Bifidobacteria were detected only in few dogs, moreover, at very low values of log10 16S ribosomal DNA gene copies/g faecal sample (data not shown).
Table 4. Microbial analysis (log10 16S ribosomal DNA gene copies/g fresh matter) of faecal samples from dogs (n = 8) fed increasing doses of lactose.
Coefficients of ATTD of macronutrients, macrominerals and trace elements are reported in Table . Lactose supplementation influenced the digestibility of DM (quadratic, p < .05) and some minerals: Ca, K, Mn and Fe (quadratic, p < .05), whereas the ATTD of crude protein, crude ash, P, Mg and Zn were only tendentially affected, without reaching the statistical significance (quadratic, p = .055, .089, .091, .065 and .065, respectively). Furthermore, Mg digestibility was linearly decreased by lactose supplementation (linear, p < .05).
Table 5. Coefficients of total tract apparent digestibility of nutrients in dogs (n = 8) fed diets supplemented with increasing doses of lactose.
Discussion
The aim of the present study was to evaluate the effects of increasing moderate doses of lactose on intestinal microbial activity and ATTD in adult healthy dogs. In particular, the study design, based on the supplementation with increasing doses of lactose sequentially added to the dogs’ diet in order to avoid rapid dietary changes, has allowed evaluating the lactose tolerance threshold and the cumulative effects on the canine intestinal environment over the time.
Eight of the 14 dogs initially involved in the trial concluded the three planned supplementation periods, displaying to well-tolerate lactose up to the highest dose provided (2 g/kg BW0.75/d). Anyway, only two of the six dogs excluded from the study exhibited acute diarrhoea attributable to a condition of lactose-intolerance, after consuming for the first time the diet supplemented with 1 g of lactose/kg BW0.75, as reported by their owners. Differently, the other four dogs just refused the diet at the beginning of the first supplementation period. In a previous study carried out with four adult Beagle dogs receiving a daily dose of 1 g of lactose/kg BW for 10 d, none of the animals displayed gastrointestinal disorders or palatability problems (Zentek et al. Citation2002). In this latter study, lactose was added to the basal extruded diet during its manufacturing, resulting well-mixed with the other ingredients. On the contrary, in present study, lactose (provided in a powder form) was individually dosed for each dog and spread on the kibbles immediately prior to the mealtime. Canine species is notoriously sensitive to mono- and disaccharides such as lactose, which are perceived sweet and characterised by a pleasant aroma (Bradshaw Citation1991). Therefore, food refusal exhibited by four dogs when lactose was added for the first time to their diet are difficult to explain. Probably, the presence of the lactose powder and/or even the little amount of water used to unsure the lactose adhesion to the kibbles may have negatively influenced in these last animals the ‘texture’ perception, which represents a well-known factor influencing diet palatability (Koppel Citation2014).
Eight dogs concluded the experiment, displaying a good gastrointestinal tolerance to the lactose supplementation up to the daily dose of 2 g/kg BW0.75. This result is in accordance with previous scientific evidences suggesting a lactose tolerance threshold in the adult dog of 1 g/kg BW/day (Meyer and Zentek Citation1998).
During present investigation, faecal quality was not evaluated because dogs continued to live with their owners and an objective assessment would have been difficult. Anyway, dog-owners were specifically asked to report any change in stool consistency noticed during the study.
Dietary lactose supplementation showed an influence on faecal moisture. In particular, increasing doses of lactose from 0 to 1 g/kg BW0.75/d led to higher faecal water content, while this parameter decreased when dogs consumed the highest daily dose of lactose (2 g/kg BW0.75). Anyway, these variations were quantitatively modest and, probably for that reason, no dog-owner described faecal changes during the three supplementation periods. As each lactose-supplemented diet was fed to dogs for a relative long time (20 d), the variation in faecal water content observed throughout the study may be attributable to a sort of slow ‘adaptation’ displayed by the digestive enzymatic system of the dogs, which have been never fed with diets containing lactose prior the beginning of the trial. Moreover, similarly to what happens in ‘lactase non persistent people’ (Szilagyi Citation2015), also in adult dogs the regular consumption of lactose may have led to ‘colonic adaptation’ by the intestinal microbiome. Later, the improvement of lactose digestion may have gradually reduced its by-pass towards the hindgut, where it had firstly exerted a mild osmotic effect (with a consequent increase of the faecal water content of the dogs) during the first two-supplementation periods. The adaptation to the dietary lactose consumption in the dogs could explain the decrease of faecal water content observed in dogs at the end of the trial.
During this study more than half of the adult dogs initially involved (8 of 14) showed to tolerate lactose up to a relative high daily dose (2 g/kg0.75/d), presumably because of a residual lactase activity in their small intestinal tract. In previous studies in canine and feline species, similar moderate doses of lactose added to the diet resulted well-tolerated by the animals (without the appearance of any gastrointestinal signs) and did not affect faecal moisture. For example, a diet containing lactose at 100 g/kg (corresponding to 1.2 g/kg BW/d) fed to eight adult cats for a period of 14 d did not alter daily faecal production and faecal dry matter (Beynen and Yu Citation2003). Furthermore, in the previously cited study by Zentek et al. (Citation2002), carried out with four adult Beagles, a daily dose of 1 g of lactose/kg BW for 10 d did not change consistency, dry matter and unbound water content of faeces. Certainly, future investigations carried out with higher numbers of animals would be useful to better clarify the ‘lactose tolerance’ issue in canine species.
Lactose has been suggested to be a conditional prebiotic in lactose maldigesters. In fact, if this disaccharide is not efficiently digested in the small intestine, it reaches the colon where it could exert beneficial effects on microbial activity (Szilagyi Citation2004), similarly to lactose-derived molecules such as lactitol, galacto-oligosaccharides and lactulose, well-known for their prebiotic properties (Venema Citation2012).
Currently, there is a paucity of studies investigating the prebiotic effects deriving from a lactose supplementation, particularly in companion animals. Traditionally, the prebiotic effects of a substance are assessed through the evaluation of changes in the intestinal microbiota, as a prebiotic is expected to selectively stimulate the growth and activity of intestinal bacteria associated with health and wellbeing (Roberfroid Citation2007). In a previous study by Kienzle (Citation1994), two groups of adult cats receiving different dietary supplementations of lactose (110 and 280 g/kg DM of diet) displayed lower faecal pH, in comparison with the control group fed with a carbohydrate-free diet. Conversely, in the previously cited study by Zentek et al. (Citation2002), a diet supplemented with 1 g of lactose/kg BW did not modify parameters such as pH and concentrations of ammonia and SCFA in faeces of lactose-tolerant dogs. Similarly, in the present study, increasing doses of dietary lactose in eight lactose-tolerant dogs did not modify faecal chemical parameters such as pH, SCFA, ammonia and biogenic amines. Nonetheless, faecal samples are not representative of the intestinal environment, given that, for example, the 95–99% of SCFA produced in the hindgut is rapidly absorbed by the intestinal mucosa, before reaching the distal colon (Wong et al. Citation2006). In regards to parameters such as ammonia and biogenic amines, they are traditionally considered markers of intestinal proteolysis as well as volatile-branched chain fatty acids (isovaleric and isobutyric acids). In particular, ammonia represents a toxic and potentially carcinogenic compound deriving from proteolytic bacterial activity. Furthermore, isovaleric and isobutyric acids derive from the catabolism of branched-chain amino acids valine and leucine, respectively (Blachier et al. Citation2007). Similarly, biogenic amines are nitrogenous molecules principally produced from amino acids and peptides decarboxylation, mainly by proteolytic bacteria such as clostridia and Bacteroides and they are precursors of a well-known carcinogenic compound such as nitrosamine (Blachier et al. Citation2007).
During present study, the faecal concentration of isovaleric acid tended to linearly decrease with increasing doses of lactose. This result (close to a statistical significance) induces to hypothesise a prebiotic outcome deriving from lactose supplementation, as it may reflect a tendency to a reduction of the bacterial protein catabolism in the large intestine of the dogs.
For ethical reasons, the two dogs that experienced diarrhoea when fed with the diet supplemented with 1 g of lactose/kg0.75/d were immediately excluded from the study (and their faeces were not analysed). All other dogs displayed a condition of lactose-tolerance, but it was not possible to assess if lactose was completely digested in the dogs’ small intestine. In this regard, the slightly higher faecal moisture observed when lactose was fed at 0.5 and 1 g/kg0.75/d seems to support the hypothesis that part of the ingested lactose reached the hindgut. Anyway, the lack of variation in faecal parameters induces to suppose that lactose was well digested in the canine intestinal tract.
According to human studies, lactose supplementation is able to exert prebiotic effects such as increase of intestinal beneficial bacteria (bifidobacteria and lactobacilli) (Szilagyi et al. Citation2010), higher production of SCFA (Szilagyi Citation2004) and inhibition of potential pathogenic bacteria such as clostridia (Szilagyi Citation2004) only in lactose intolerant people and not in lactose digesters (discerned by the breath-hydrogen analysis), even at relative high-dose supplementations (50 g/day for 2 weeks; Szilagyi et al. Citation2010).
An improvement of intestinal health attributable to lactose ingestion has been shown also in piglets where lower faecal pH, reduced concentrations of branched chain fatty acids, potential pathogenic bacteria like Coliformes spp., higher total SCFA and butyric acid (Pierce et al. Citation2006) as well as faecal lactobacilli (Molino et al. Citation2011) and bifidobacteria (Pierce et al. Citation2007) have been reported. However, in these last studies, the gastro-intestinal tolerance of the animals to lactose has not been described.
In contrast, during the present study, the ingestion of increasing doses of lactose did not affect the bacterial populations evaluated. In addition, qPCR analysis detected and quantified faecal bifidobacteria (well-known for the ability to use lactose and other milk oligosaccharides for energy purpose; Sela and Mills Citation2010) in only few dogs (moreover, at very low levels). In this regard, the evidences in literature are conflicting. In fact, some studies, in agreement with present results, have demonstrated the rareness of faecal bifidobacteria in healthy dogs (Beloshapka et al. Citation2013). Conversely, other investigations have highlighted a higher presence of this bacterial population in canine faeces (Middelbos et al. Citation2007).
The lack of a prebiotic effect in the eight dogs concluding the present trial could be attributable to inadequate amount of lactose reaching the hindgut, low number of animals involved (from a statistical point of view) or a transient change of microbial parameters along the hindgut which did not manifest in faecal samples.
During the present trial, dogs were kept in their usual living conditions and, consequently, they drank waters characterised by a potentially different mineral content that was not evaluated. Certainly, this aspect represented a limit for the minerals digestibility assessment. Anyway, results concerning mineral ATTD are in agreement with previous investigations in dogs (Zentek et al. Citation2002) and cats (Kienzle Citation1994).
In the present study, lactose influenced the ATTD of some minerals (Ca, K, Mn and Fe) and tended to affect that of other nutrients (crude protein, crude ash, P, Mg and Zn) with a quadratic trend. On the contrary, in the previously cited study with four lactose-tolerating Beagles, the apparent digestibility of all nutrients evaluated (dry matter, crude protein, crude fat, crude fibre, nitrogen-free extracts and macrominerals) was not influenced by lactose (fed at the dose of 1 g/kg BW) (Zentek et al. Citation2002). Similarly, in cats fed with diets supplemented with lactose (110 and 280 g/kg DM) no changes in apparent protein and macrominerals digestibility were observed (Kienzle Citation1994). In the previously cited study in piglets, an improvement of ATTD of all evaluated nutrients (dry matter, crude protein, organic matter, neutral detergent fibre and gross energy) was observed when dietary lactose concentration was increased from 125 to 250 g/kg (Pierce et al. Citation2007). In the present study, the quadratic changes in minerals digestibility in dogs fed with increasing doses of lactose may be attributable (as previously suggested in regard to the modifications in faecal moisture) to an ‘enzymatic adaptation’, with a consequent slow improvement of the digestion of this sugar. On this basis, undigested lactose may have gradually reduced its by-pass towards the hindgut and, consequently, a temporary prebiotic effect (which could explain the enhanced digestibility of minerals during the first supplementation period) may have disappeared during the second part of the study. In this regard, the improvement of intestinal absorption of minerals is a recognised effect deriving from the intestinal fermentation of prebiotic substrates. In particular, among the several supposed mechanisms to explain this effect, there is the acidification of the intestinal environment (consequent to greater SCFA production), which can improve the solubility of minerals (Scholz-Ahrens et al. Citation2007). In this regard, some previous studies have demonstrated a correlation between increased apparent mineral absorption and lower colic pH values in cecectomised rats fed with diets supplemented with fructooligosaccharides (Ohta et al. Citation1995). In a study with dogs, Beynen et al. (Citation2002) reported greater apparent minerals digestibility (Ca, in particular) but no change in faecal pH when animals were fed a diet supplemented with oligofructose. These latter results, as well as those observed in the present study, could be hypothetically the consequence of a temporary acidification of digesta, attributable to prebiotics fermentations by colonic bacteria. As previously underlined, SCFA are rapidly absorbed in the hindgut so this acidifying effect may be transient and not detectable through faecal analysis. If this was the case in the present study, it may be supposed that the undigested lactose reaching the hindgut may have acted as a prebiotic in the lactose-tolerant dogs during the first supplementation period. Moreover, in regards to the increase of apparent Ca digestibility, lactose is known to improve the passive transport of this macromineral across the small intestinal mucosa, through a mechanism independent from the vitamin D endocrine system (Areco et al. Citation2015).
Conversely, the linear decrease of apparent Mg digestibility induced by increasing dietary lactose is difficult to explain. Previous authors described an improving effect on the apparent ATTD of Mg when lactose replaced glucose (at the concentration of 106 g/kg DM) in the diet of rats (Heijnen et al. Citation1993). Contrariwise, in a study with cats, a dietary lactose inclusion of 109 g/kg DM had no effect on apparent Mg digestibility (Beynen and Yu Citation2003).
Conclusions
Eight of the 14 participant dogs in the present study exhibited a good tolerance up to a relative high dietary dose of lactose (2 g/kg BW0.75/d). The quadratic changes in faecal moisture and ATTD of some minerals may suggest that some enzymatic adaptations to lactose took place in the intestinal tract of the dogs during the study, although the small number of the animals and the lack of standardisation of the environmental conditions undoubtedly have represented a limit for the interpretation of the data. In general, an evident prebiotic effect of lactose on the canine intestinal ecosystem was not observed.
Acknowledgements
The authors are thankful to Effeffe Pet Food S.p.A. (Padua, Italy) for providing the commercial diet used in the present study.
Disclosure statement
No potential conflict of interest was reported by the authors.
References
- Areco V, Rivoira MA, Rodriguez V, Marchionatti AM, Carpentieri A, Tolosa de Talamoni N. 2015. Dietary and pharmacological compounds altering intestinal calcium absorption in humans and animals. Nutr Res Rev. 28:83–99.
- AOAC. 2000. Official methods of analysis. Association of Official Analytical Chemists Publ., 17th ed. Gaithersburg (MD): AOAC International.
- Bach HJ, Tomanova J, Schloter M, Munch J. 2002. Enumeration of total bacteria and bacteria with genes for proteolytic activity in pure cultures and in environmental samples by quantitative PCR mediated amplification. J Microbiol Methods. 49:235–245.
- Beloshapka AN, Dowd SE, Suchodolski JS, Steiner JM, Duclos L, Swanson KS. 2013. Fecal microbial communities of healthy adult dogs fed raw meat-based diets with or without inulin or yeast cell wall extract as assessed by 454 pyrosequencing. FEMS Microbiol Ecol. 84:532–541.
- Bennett MJ, Coon E. 1966. Mellituria and postprandial blood sugar curves in dogs after the ingestion of various carbohydrates with the diet. J Nutr. 88:163–168.
- Beynen AC, Baas JC, Hoekemeijer PE, Kappert HJ, Bakker MH, Koopman JP, Lemmens AG. 2002. Faecal bacterial profile, nitrogen excretion and mineral absorption in healthy dogs fed supplemental oligofructose. J Anim Physiol Anim Nutr. 86:298–305.
- Beynen AC, Yu S. 2003. Magnesium balance in adult cats fed a dry food rich in lactose. J Anim Physiol Anim Nutr. 87:245–250.
- Biagi G, Piva A, Moschini M, Vezzali E, Roth FX. 2006. Effect of gluconic acid on piglet growth performance, intestinal microflora, and intestinal wall morphology. J Anim Sci. 84:370–378.
- Blachier F, Mariotti F, Huneau JF, Tomé D. 2007. Effects of amino acid-derived luminal metabolites on the colonic epithelium and physiopathological consequences. Amino Acids. 33:547–562.
- Bradshaw JWS. 1991. Sensory and experiential factors in the design of foods for domestic dogs and cats. Proc Nutr Soc. 50:99–106.
- Collier CT, Smiricky-Tjardes MR, Albin DM, Wubben JE, Gabert VM, Deplancke B, Bane D, Anderson DB, Gaskins HR. 2003. Molecular ecological analysis of porcine ileal microbiota responses to antimicrobial growth promoters. J Anim Sci. 81:3035–3045.
- Corgneau M, Scher J, Ritié-Pertusa L, Le DTL, Petit J, Nikolova Y, Banon S, Gaiani C. 2015. Recent advances on lactose intolerance: tolerance thresholds and currently available solutions. Crit Rev Food Sci Nutr. 57:3344–3356.
- Fox PF, Uniacke-Lowe T, McSweeney PLH, O’Mahony JA. 2015. Lactose, In: Dairy chemistry and biochemistry. 2nd ed. Cham, Switzerland: Springer International Publishing; p. 21–68.
- Heijnen AM, Brink EJ, Lemmens AG, Beynen AC. 1993. Ileal pH and apparent absorption of magnesium in rats fed on diets containing either lactose or lactulose. Br J Nutr. 70:747–756.
- Kienzle E. 1993. Carbohydrate digestion of the cat. 3. Digestion of sugars. J Anim Physiol Anim Nutr. 69:203–210.
- Kienzle E. 1994. Effect of carbohydrates on digestion in the cat. J Nutr. 124:2568S–2571S.
- Koppel K. 2014. Sensory analysis of pet foods. J Sci Food Agric. 94:2148–2153.
- Mahan DC, Fastinger ND, Peters JC. 2004. Effects of diet complexity and dietary lactose levels during three starter phases on postweaning pig performance. J Anim Sci. 82:2790–2797.
- Matsuki T, Watanabe K, Fujimoto J, Miyamoto Y, Takada T, Matsumoto K, Oyaizu H, Tanaka R. 2002. Development of 16S rRNA-gene-targeted group-specific primers for the detection and identification of predominant bacteria in human feces. Appl Environ Microbiol. 68:5445–5451.
- Meyer H, Zentek J. 1998. Nahrungsaufnahme und Verdauung. In: Ernährung des Hundes: Grundlagen-Fütterung-Diätetik. Berlin-Wien: Blackwell Wissenschafts-Verlag; p. 36–43.
- Middelbos IS, Fastinger ND, Fahey GC Jr. 2007. Evaluation of fermentable oligosaccharides in diets fed to dog in comparison to fiber standards. J Anim Sci. 85:3033–3044.
- Molino JP, Donzele JL, de Oliveira RFM, Ferreira AS, de Moraes CA, Haese D, Saraiva A, de Oliveira JP. 2011. Lactose levels in diets for piglets weaned at 21 days of age. R Bras Zootec. 40:1233–1241.
- Morris JG, Trudell J, Pencovic T. 1977. Carbohydrate digestion by the domestic cat (Felis catus). Br J Nutr. 37:365–373.
- NRC, Division on Earth and Life Studies, Board on Agriculture and Natural Resources, Committee on Animal Nutrition, Subcommittee on Dog and Cat Nutrition. 2006. Nutrient requirement of dogs and cats. Washington (DC): National Academies Press.
- Ohta A, Ohtsuki M, Baba S, Takizawa T, Adachi T, Kimura S. 1995. Effects of fructooligosaccharides on the absorption of iron, calcium and magnesium in iron-deficient anemic rats. J Nutr Sci Vitaminol. 41:281–291.
- Pierce KM, Callan JJ, McCarthy P, O’Doherty JV. 2007. The interaction between lactose level and crude protein concentration on piglet post-weaning performance, nitrogen metabolism, selected faecal microbial populations and faecal volatile fatty acid concentration. Anim Feed Sci Technol. 132:267–282.
- Pierce KM, Sweeney T, Callan JJ, Byrne C, McCarthy P, O’Doherty JV. 2006. The effect of lactose and inulin on intestinal morphology, selected microbial population and volatile fatty acid concentration in the gastrointestinal tract of the weanling pig. J Anim Sci. 82:311–318.
- Rinttilä T, Kassinen A, Malinen E, Krogius L, Palva A. 2004. Development of an extensive set of 16S rDNA-targeted primers for quantification of pathogenic and indigenous bacteria in faecal samples by real-time PCR. J Appl Microbiol. 97:1166–1177.
- Roberfroid M. 2007. Prebiotics: The concept revisited. J Nutr. 137:830S–837S.
- Scholz-Ahrens KE, Ade P, Marten B, Weber P, Timm W, Açil Y, Glüer CC, Schrezenmeir J. 2007. Prebiotics, probiotics, and synbiotics affect mineral absorption, bone mineral content, and bone structure. J Nutr. 137:838S–846S.
- Sela DA, Mills DA. 2010. Nursing our microbiota: molecular linkages between bifidobacteria and milk oligosaccharides. Trends Microbiol. 18:298–307.
- Szilagyi A. 2004. Redefining lactose as a conditional prebiotic. Can J Gastroenterol. 18:163–167.
- Szilagyi A. 2015. Adaptation to lactose in lactase non persistent people: effects on intolerance and the relationship between dairy food consumption and evaluation of diseases. Nutrients. 7:6751–6779.
- Szilagyi A, Shrier I, Heilpern D, Je J, Park S, Chong G, Lalonde C, Cote LF, Lee B. 2010. Differential impact of lactose/lactase phenotype on colonic microflora. Can J Gastroenterol. 24:373–379.
- Totton SC, Farrar AM, Wilkins W, Bucher O, Waddell LA, Wilhelm BJ, McEwen SA, Rajić A. 2012. The effectiveness of selected feed and water additives for reducing Salmonella spp. of public health importance in broiler chickens: a systematic review, meta-analysis, and meta-regression approach. Prev Vet Med. 106:197–213.
- Venema K. 2012. Intestinal fermentation of lactose and prebiotic lactose derivatives, including human milk oligosaccharides. Int Dairy J. 22:123–140.
- Vogtmann HP, Frirter P, Prabuck AL. 1975. A new method of determining metabolizability of energy and digestibility of fatty acids in broiler diets. Br Poult Sci. 16:531–534.
- Wang RF, Cao WW, Franklin W, Campbell W, Cerniglia CE. 1994. A 16S rDNA-based PCR method for rapid and specific detection of Clostridium perfringens in food. Mol Cell Probes. 8:131–137.
- Wong JM, de Souza R, Kendall CW, Emam A, Jenkins DJ. 2006. Colonic health: fermentation and short chain fatty acids. J Clin Gastroenterol. 40:235–243.
- Zentek J, Marquart B, Pietrzak T. 2002. Intestinal effects of mannanoligosaccharides, transgalactooligosaccharides, lactose and lactulose in dogs. J Nutr. 132:1682S–1684S.