Abstract
To research the isolation, identification, physiological property of Clostridium butyricum strain using lactic acid from pig and its effects on intestinal function of weaned piglets, the C. butyricum strain LY33 was isolated with an anaerobic culture method from the intestinal contents of healthy piglets fed with lactic acid bacteria fermented feeds. The strain LY33, which was identified as Clostridium butyricum according to morphological, physiological, biochemical and 16S rRNA sequence analyses, was found to be efficient in lactic acid utilisation and butyric acid conversion. Strain LY33 was non-toxic and resistant to heat, gastric acid and bile salts. It utilised lactic acid efficiently when cultured in LADM liquid medium (Lactic acid decomposition medium) under the initial conditions of pH 5.5 and a lactic acid concentration of 132 mmol. Strain LY33 also had inhibitory effects on Escherichia coli, Salmonella, and other pathogens. Conversely, it promoted the growth of lactic acid bacteria. Compared to the control group fed the basal diet, the indexes of intestinal function of piglets in the experimental group fed the strain LY33 preparation improved markedly, and for most of these indicators, a difference at p < .05 was achieved.
In this study, one Clostridium butyricum strain LY33 was isolated from intestinal contents in healthy piglets which were fed lactic acid bacteria fermented feed, and was identified as Clostridium butyricum based on its morphological, physiological, biochemical and 16S rRNA sequence analysis.
The strain LY33 had better ability of utilising lactic acid, had a certain inhibitory effect on Escherichia coli, Salmonella and other pathogens, can promote the growth of lactic acid bacteria, and the preparation of Clostridium butyricum had a good effect on improving the intestinal function of weaned piglets.
Highlights
Introduction
Clostridium butyricum, or C. butyricum, is a gram-positive anaerobic bacillus (Lawson Citation2016) that has been found to play an important role in regulating the intestinal tract when cultivated in anaerobic conditions by producing butyric acid (Whelan and Nasr Citation1951). The ability of C. butyricum to inhibit pathogenic bacteria in the intestinal tract and promote the growth of beneficial bacteria such as Bifidobacterium and Lactobacillus has found use in animal husbandry, mainly as a feed additive (Hamer et al. Citation2008; Yang et al. Citation2012; Kelsic et al. Citation2015). Current research has shown that the colonisation of C. butyricum plays an important role in livestock and poultry intestines, such as promoting intestinal development by strengthening intestinal functions (Gao et al. Citation2011; Mansuy Citation2012; Liao et al. Citation2015).
Considering today’s reduction in the weaning time of piglets (Niekamp et al. Citation2007; Smith et al. Citation2010; Xiao et al. Citation2014) and its effect on the animals’ underdeveloped immune and digestive systems, probiotic fermented feed additives have been gradually included in piglet feeding practices. These additives are safe, non-toxic and efficient; therefore, they have become a popular topic of discussion and study among animal nutritionists (Le et al. Citation2016; Missotten et al. Citation2016;) Among them, fermented lactic acid bacteria feed has been the most widely used in recent years (Missotten et al. Citation2009; Lin et al. Citation2010). However, researchers have found that piglets weaned using a large amount of fermented lactic acid bacteria feed were prone to diarrhoea. The reason is quite likely that lactic acid bacteria generate lactic acid, which accumulates in the intestines of the piglets and causes some difficulty in their ability to absorb water, and then large amounts of water reverse-infiltrate into the intestinal cavity and cause permeable diarrhoea (Hayden et al. Citation1998; Tsukahara and Ushida Citation2001). Consequently, the effect of fermented lactic acid bacteria feeds has been questioned, and this has affected its wider promotion and application (Wang et al. Citation2015).
Using lactic acid as the sole source of carbon and energy, this study seeks to isolate C. butyricum strains that produce butyric acid from lactic acid from the intestines of healthy piglets fed with fermented lactic acid feed and explores the physiological characteristics of this bacteria, its ability to transform lactic acid and the characteristics of a co-culture of lactic acid bacteria and pathogenic bacteria. This study also explores the effect fermented preparations have on the intestines of weaned piglets to elucidate the mechanism of the function of C. butyricum, the production of lactic acid bacteria and C. butyricum co-fermented feed, and the improvement of treatments for the adverse reactions of livestock and poultry intestines caused by too much lactic acid. The following findings may counterbalance the inaction of other probiotics and provide a theoretical basis for the research, development, and promotion of probiotic fermented feed.
Materials and methods
Materials
Isolated samples of C. butyricum strains were collected from piglets’ intestinal contents obtained from the Fujian Province of China. These piglets (25–28 days of age) were fed 4% fermented lactic acid bacterial feed without any antibiotics.
Co-cultured strains, Escherichia coli (E. coli), Salmonella enteritidis, and Lactobacillus acidophilus, were provided by the animal medical research centre of Longyan University.
PCR-related reagents were purchased from Tiangen Company (Beijing, China). Primers were designed and synthesised by Sangon Biotech Co., Ltd (Shanghai, China). The artificial gastric juice (asepsis, refer to the Chinese Pharmacopoeia, which consists of pepsin and hydrochloric acid, etc) and artificial intestinal juice (asepsis, refer to the Chinese Pharmacopoeia, which consists of trypsin and phosphate, etc.) were all purchased from Bjbalb Technology Co., Ltd (Beijing).
Medium
In this study, a lactic acid decomposition medium (LADM medium) was used, which consisted of 10 g casein, 2.5 g yeast steeped in liquid, 1 g cysteine; 0.45 g KH2PO4, 0.9 g NaCl, 0.09 g MgSO4·7H2O, 0.09 g CaCl2, 10 mg haem, 10 µg biotin, 10 µg cobalamin, 30 µg p-aminobenzoic acid, 50 µg folic acid, 150 µg pyridoxamine, 0.5 µg thiamine, 0.5 µg riboflavin, 1 mg resazurin, 5 mL lactic acid (∼0.6%) as the carbon source, 20 g agar (used with a solid medium) and 1000 mL water at pH 6.0–6.5. During the test of the transformation characteristics of lactic acid, the lactic acid content and the pH value of the culture medium were added to or adjusted according to the test design.
A reinforced Clostridium medium (RCM medium) was also used, which consisted of 5 g glucose, 3 g yeast extract, 10 g beef extract, 10 g tryptone, 1 g soluble starch, 5 g NaCl, 3 g sodium acetate trihydrate; 0.5 g cysteine hydrochloride, 0.3 g, resazurin and 20 g agar (used with solid medium) and 10,000 mL distilled water at pH 6.5–7.0.
Screening of the strains
For the first screening, 1 g of the collected sample (piglets’ intestinal waste from the caecum, colon, duodenum, jejunum and ileum) was added to 50/250 mL of the LADM liquid medium, and the anaerobic enrichment culture was shaken at 30 °C for 5 days. The sample then continued to be cultured in an anaerobic tube with LADM at a proportion of 5%, and the lactic acid consumption was monitored regularly. When lactic acid could be degraded rapidly, the sample was diluted 10-fold and then evenly spread onto an LADM solid medium plate in an anaerobic chamber. Next, the sample was cultured anaerobically at 37 °C for 3–5 days. After this, a single colony was selected to purify the strains using the streak inoculation method in the LADM solid medium. When the pure strains were isolated, they were inoculated in the agar slant anaerobic tube for preservation.
The strains from the first screening were then inoculated in an anaerobic tube containing 10 mL of the RCM liquid medium. The strains were hot compressed at 80 °C for 10 min and cooled with water afterward. They were then placed in an incubator for an anaerobic enrichment culture at 37 °C for 4 days, and the lactic acid consumption was regularly monitored. Then, the effervescent culture liquids were selected for further enrichment culture and subculture in the RCM solid medium. Finally, strains with a high lactic acid utilisation rate and butyric acid conversion rate were selected as target strains for further research.
Screening the morphological, physiological and biochemical features of the selected strains and identifying the 16S rRNA sequences
The morphology was identified by observing colonies on the plate and by conducting Gram staining and spore staining.
Catalase tests, starch hydrolysis experiments, indole experiments, litmus milk tests, glucose fermentation experiments, nitrate reduction tests, gelatine liquefaction tests and fermentation tests of melibiose, melezitose, esculin, maltose and other carbohydrates were used for physiological and biochemical identification.
A 16S rRNA sequence analysis was used (Clarke and Morris Citation1976). The bacterial genome was extracted by the genomic DNA extraction kit (Sangon Biotech, China), using the total DNA purified from bacteria as template, and universal primers 27F and 1492R were used for PCR primers, 27F: 5′-AGAGTTTGATCCTGGCTCAG-3′; 1492R: 5′-GGTTACCTTGTTACGACTT-3′. PCR reaction system (50μL): 10× buffer 5 μL, dNTPs mixture (10 mol/L) 4 μL, primer F (35 μmol/L) 1 μL, primer R (35 μmol/L) 1μL, Taq polymerase (5 U/μL) 1 μL, DNA template (50ng/μL) 1 μL. PCR amplification was carried out as follows: 30 cycles at 95 °C for 5 min, 94 °C for 1 min, 50 °C for 1 min and 72 °C for 1.5 min. The final setting, 72 °C, was extended to 10 min. Agarose gel electrophoresis was used to detect the length and yield of the amplified product fragments (∼1500 bp). After recovery and purification of the gel by SanPrep Column DNA Gel Extraction Kit (Sangon Biotech, China), the 16s rDNA sequence was ligated with Peasy-T1 vector (TransGen Biotech, China), and the product was transformed into competent E. coli DH5α cells. The identified positive clones were then sent to Shanghai Sangon Biotech Co., Ltd. for sequencing.
Sequence alignment was performed with the 16S rRNA sequence in the NCBI database. The aligned sequence information of the relevant strains 16S rRNA was downloaded, and the 10 strains sequence with the highest homology were selected to construct the phylogenetic tree.
Safety test of the strain
An acute toxicity test was carried out using Horn’s method, i.e. intragastric administration, in accordance with the maximal tolerance criteria of GB15193.3-2014. Based on these methods, 60 mice (30 male and 30 female, Kunming mice) weighing 20–25 g were selected and 0.5 mL of liquid (106 CFU/mL) was given orally 3 times a day. During the following 21 days, we observed whether the rats showed signs of poisoning or death due to drinking the liquid (Giménez and Sugiyama Citation1988). All mice were obtained from Minhou WuShi Experimental Animal Trade Co., Ltd. (Fuzhou, China), and were fed with regular chow and housed in an air-conditioned room with food and water available ad libitum throughout the studies. The method of euthanasia for the mice used in this study consisted of anaesthesia (5% isoflurane), followed by cervical dislocation.
Test of the physiological characteristics of the bacterial strain
A high-temperature resistance test was conducted: 5.0 mL of liquid of bacteria that has completely formed spores in the growth platform was taken out and transferred into anaerobic test tubes and placed in water baths at different temperatures, 60 °C, 65 °C, 70 °C, 75 °C, 80 °C, 85 °C, 90 °C, 95 °C, and 100 °C, for 5 min to obtain the viable count and identification of the maximum temperature at which the bacterial strain could maintain high activity. Another 5.0 mL of liquid was put into the water bath with the highest temperature that was identified in the last step to perform the viable count after 5, 7.5, 10, 12.5 and 15 min and to calculate the survival rate of the strains. Each test was repeated three times.
Following this, bacteria in their last growth phase in liquid were centrifuged for 10 min at 3000 r/m and resuspended after being washed with PBS twice. It was then inoculated into an artificial gastric juice (pH 2.0) at 107 CFU/mL, evenly mixed by shaking and incubated in a water bath at 37 °C. A viable count was performed every half an hour for the survival rate. The same process was repeated for the strains that had gone through the test with the artificially made gastric juice. They were then inoculated into an artificial intestinal juice at 107 CFU/mL, and the same procedure was applied to them as for the gastric juice resistance test.
A bile salt resistance test was used: 0.2 mL of bacteria in liquid was inoculated in 2 mL of RCM liquid media containing 0%, 0.1%, 0.2%, 0.3%, 0.4% and 0.5% pig bile salts. These samples were then cultured in a 37 °C anaerobic incubator for 12 h. A viable count was performed later for the survival rate.
The 108 CFU/mL bacteria liquid was spread onto the RCM solid plate, and various antibiotic susceptibility papers were attached to the surfaces of media (The antibiotic disk diffusion method). They were then cultured in a 37 °C anaerobic incubator for 24 h before the diameter of the inhibition zone was measured. Conclusions were finally drawn according to the standards stated in the instructions.
Test of the transformation of lactic acid by the bacterial strain
The pH of different LADM liquid media was controlled at 4.5, 5.0, 5.5, 6.0, 6.5 and 7.0 before adding 0.6% (66 mmol) lactic acid. They were then cultured in a 37 °C anaerobic incubator for 5 days. Periodic samplings were carried out to check the consumption of lactic acid and the production of acetic acid to observe the effect of initial pH on the growth of the bacterial strains and the transformation of lactic acid.
Based on the above test, the pH of selected LADM liquid media were controlled before adding 0.9% (99 mmol), 1.2% (132 mmol), 1.5% (165 mmol), 1.8% (198 mmol), 2.0% (220 mmol) lactic acid to the medium to observe the effect of the different lactic acid densities on the bacterial strain growth and transformation of lactic acid.
Lactic acid and butyric acid were tested based on Shimadzu high-performance liquid chromatography (Bevilacqua and Califano Citation1989). The chromatographic column was set at HypersilC 184.6 × 150 mm, 5 bar; mobile phase, 2.5 mmol; the pH of ammonium dihydrogen phosphate, 2.65; flow velocity, 1.0 mL/min; and wavelength, 210 nm.
Co-culture of lactic acid bacteria and pathogenic bacteria
After they were cultured to the phase of logarithm growth, 0.1 mL of lactic acid bacteria E. coli and Salmonella were removed for inoculation in 9.9 mL of RCM liquid medium and placed in a 37 °C stasis for 48 h for further cultivation. Their pH was measured, and viable counts were performed every 4 h. Then, 0.1 mL of C. butyricum and lactic acid bacteria, C. butyricum and E. coli, and C. butyricum and salmonella were inoculated in 9.8 mL of RCM liquid media and placed in a 37 °C stasis for 48 h for further cultivation. Their pH was measured, and a viable count was performed every 4 h to compare the outcomes of the individual cultures and co-cultures.
Impact of C. butyricum preparations on weaned piglets
The separated C. butyricum were activated, inoculated in the RCM liquid media at a proportion of 1% and placed into an anaerobic incubator for 42–48 h. They were centrifuged for 10 min at 4000 r/m, dried at 60 °C, mixed with bran until their final density was 108–109 CFU/g, and then kept at 4 °C.
For selection, 60 healthy Duroc-Landrace-Yorkshire ternary hybrid weaned piglets with similar birthdates and weights of ∼20 kg were selected for sampling. All pigs were purchased from Zhangping Shunjia Farming Development Co., Ltd. (Zhangping, China), and were housed in the Animal Room of Longyan University. They were then split into two groups randomly. The control group was fed basic rations, and the experimental group was fed basic rations mixed with 1 × 1012 CFU/t of C. butyricum. During the feeding period (20–90 kg, ∼130 days), the experimental piglets ate and drank water freely, and they were vaccinated and managed according to the routine requirements of pig farms.
To measure the impact on the piglets’ bowel function, 10 pigs were randomly selected from the control group and experimental group during slaughter. All pigs were slaughtered with a normal humane procedure, and all efforts were made to minimise suffering. The pigs were euthanized by electric shock and then dehaired; the carcases were dissected. The caecum, colon, duodenum, jejunum and ileum were ligated under aseptic condition, ∼2 cm in each sample was cut quickly by surgical scissors, respectively. All samples were washed with normal saline and fixed in 10% formalin for the detection of intestinal histology. The intestinal waste from the caecum, colon, duodenum, jejunum and ileum was removed and taken into 10 mL sterile EP tube, and then stored in a sealed container at –20 °C. The intestinal waste from the caecum and colon was used to measure the intestinal ammonia density and pH. Some intestinal sections from the duodenum, jejunum and ileum were used to make frozen sections and HE staining (Hematoxylin-eosin staining), the stained tissue slices were photographed with Image-Pro Express software, and each slice was photographed with a 5-field view. The ratio of villus length/crypt depth (V/C) was calculated by measuring the longest villous height and the deepest depth of the crypt in each photograph, and the average value was taken. The remaining intestinal waste was used to measure the activity of intestinal enzymes. The intestinal waste was cut into pieces, 10 g of which was dissolved and homogenised in 0.14 moL/L KCl. The mixture was then homogenised, and the volume was set at 50 ml. The activity of the enzymes within the supernatant was tested after centrifugation for 10 min at 4000 r/m at 4 °C. The determination indexes and analytical methods for each sample were shown in Table .
Table 1. Determination indexes and analytical methods for each sample.
Statistical analysis
Multiple alignment was carried using CLUSTALW and the phylogenetic tree was constructed with MEGA6.0 using a bootstrap test of phylogeny with neighbor-joining test. Neighbor-joining phylogenetic trees were constructed by maximum composite likelihood method and bootstrap re-sampling (n = 1000) was performed to test the robustness of the tree topologies.
The data obtained were analysed by ANOVA using the SPSS 17.0 software package, and p < .05 was significant. The Duncan method was used for multiple comparisons between groups, and the results were expressed as x ± s (mean and standard deviation).
Results
Result of centrifugation, identification and safety tests for bacteria type
Twenty strains of lactate-utilising bacteria were centrifuged using LADM solid medium, and then one strain that could be transformed effectively with lactic acid and butyric acid was selected and named LY33 according to the serial number of the separated strain. The colony of the selected strain was creamy and round. Its edges were neat, irregular or slightly abrupt, while the surface was moist, smooth, and opaque; the odour was sour. Under the microscope, the bacteria were found to be gram-positive fusiform bacilli, and a spore and ovum were present at one end, (0.6–1.1) µm×(4.0–7.0) µm. Physiological and biochemical identification confirmed that the strain could produce acid and gas. The strain could also decompose galactose, fructose, starch, melibiose, seven-leaf glycoside and maltose, while it could not decompose melezitose or liquify gelatine. Additionally, it could reduce litmus, solidified milk, and indigested coagulum, and it was tested positive via contact with enzymes and nitrates and negative with catalase and indole. 16S rRNA sequencing was performed on LY33, and a homology analysis was conducted with the NCBI database (Figure ). The results showed that the homology between LY33 and C_butyricum_w4 (DQ831126) reached 99.8%. In other words, LY33 belongs to C. butyricum. In the acute toxicity test of LY33, none of the mice were poisoned or killed, which indicates that LY33 is non-toxic. After continued intake of bacteria for 21 days, the tested mice appeared normal and were alive, indicating that the strain is basically non-toxic.
Figure 1. Phylogentic tree of strain LY33 based on 16S rDNA gene sequence. 16srRAN sequencing was performed on the LY33 and their homology analysis was conducted on the NCBI database. The result was that the homology between LY33 and C_butyricum_w4 (DQ831126) reached 99.8%, and strain LY33 was identified as Clostridium butyricum.
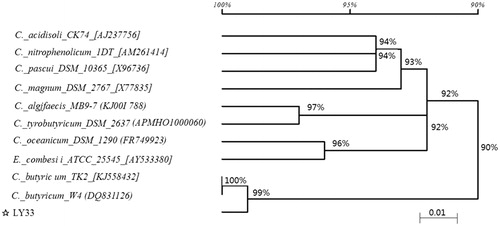
Result of test on the physiological characteristics of the bacterial strain
The results of the temperature-resistance test for LY33 are shown in Figure . The bacteria had a certain resistance to heat, but their resistance to high temperatures was poor. When the culturing temperature was below 75 °C, the activity of the spore was above 83.2%, indicating that 75 °C is the highest temperature at which LY33 can maintain high activity. The spore survival rate dropped sharply to 30.2% when the temperature was increased to 80 °C, and it dropped further to 2.3% when the temperature was increased to 80 °C. LY33 was placed in 75 °C water baths, and as time went by, its survival rate gradually reduced. When the storage time was below 8 min, its survival rate dropped very slowly. However, above 8 min, it dropped sharply, dropping to nearly half after 10 min had passed.
Figure 2. The effect of time at 75 °C on strain viability of LY33. (A) With the increase of temperature, the survival rate of LY33 decreased, and when the temperature was higher than 75 °C, the survival rate declined dramatic. (B) With increasing preservation time, the survival rate of LY33 decreased, and preservation 10min, its survival rate decreased by about half.
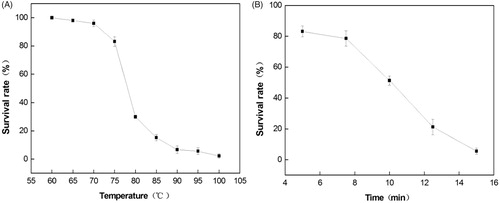
The results of the resistance test for LY33 in artificial gastric juice and intestinal juice are shown in Figure . Man-made gastric juice with pH 2.0 had no significant effect on the survival rate of LY33, and it started to drop significantly after 2.5 h. After digestion in artificial gastric juice, C. butyricum showed great resistance to artificial intestinal juice. Its survival rate did not change significantly until 2.5 h had passed.
Figure 3. Survival of LY33 in simulated gastric fluid and simulated intestinal fluid. With the prolonged action period, the survival rate of LY33 in simulated gastric fluid had no significant decreased, and it started to drop significantly after 2.5 h of effect. While in simulated intestinal fluid, the survival rate of LY33 didn't change significantly until 2.5 h had passed.
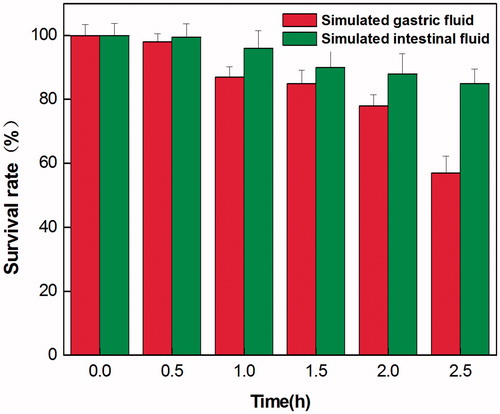
The mass fraction of bile salt in different digestive tracts was different, and the mass fraction of bile salt in the pig intestine was maintained between 0.03% and –0.3%. As illustrated in Figure , LY33 showed a strong resistance to bile salt. It grew well when the bile salt density was 0–0.2%, and the survival rate did not change much. Although the survival rate started to decrease when the density reached 0.3%, it still grew well.
Figure 4. Survival of strain LY33 at different procine bile salt concentrations. The survival rate of strain LY33 was not significantly decreased when the concentration of bile salt was 0–0.2%, and the survival rate started to decrease when the density reached 0.3%.
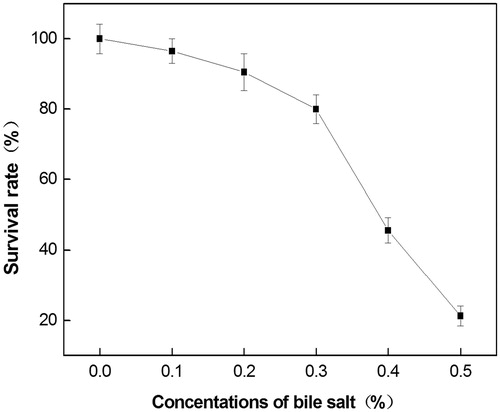
The susceptibility of LY33 to several common antibiotics in current feed production and veterinary medicine is shown in Table . These results indicated that antibiotics such as penicillin, cyclomycin and cephalosporin have stronger bacteriostasis against LY33, so they should not be combined with LY33 in veterinary use. Antibiotics such as macrolide and chloramphenicol have moderate bacteriostasis against LY33, so their combination should be used cautiously in veterinary use. Antibiotics such as amino-glycoside and carbostyril have almost no bacteriostasis against LY33, so they can be combined with LY33 in veterinary use. With endophytic spores, LY33 has a certain resistance to many kinds of antibiotics. The spores can still germinate in animal feed to which antibiotics were added, which is significant for the growth and proliferation of a bacterial strain.
Table 2. Results of antibiotic sensitivity of C. Butyricum LY33.
Results of the tests on the transformation of lactic acid by the bacterial strain
The growth characteristics of LY33 under different pH conditions are shown in Figure . These results indicated that when the pH was at 5.5 and 6.0, LY33 transformed lactic acid faster, and more butyric acid was produced than at other levels of pH. LY33 almost transformed all 66 mmol of lactic acid into ∼17 mmol of butyric acid by the 5th and 6th day of the analysis. When the pH was 7.0, LY33 very ineffectively transformed lactic acid into butyric acid, as only 34.7 mmol of lactic acid was transformed and 7.12 mmol of butyric acid was produced. When the pH was 4.5, 5.0 and 6.5, the transformation of lactic acid and production of butyric acid were affected at a moderate level. LY33 transformed all 66 mmol of lactic acid into only ∼16 mmol butyric acid after 8 days. Conclusively, 5.5 was selected as the initial pH of the LADM medium.
Figure 5. The growth feature of lactate-utilising and butyrate-producing at different initial pH. (A) The curve of lactate-utilising by LY33 at different initial pH. (B) The curve of butyrate-producing by LY33 at different initial pH. When the pH was at 5.5 and 6.0, LY33 transformed lactic acid faster and more butyric acid was produced. And LY33 almost transformed all 66 mmol lactic acid by the 5th and 6th days into around 17mmol butyric acid. When the pH was at 7.0, LY33 transformed very ineffectively, as only 34.7mmol lactic acid was transformed and 7.12mm butyric acid was produced. When the pH was at 4.5, 5.0 and 6.5, the transformation of lactic acid and production of butyric acid were of medium effectiveness, LY33 transformed all 66 mmol lactic acid into around only 16mmol butyric acid after 8 days.
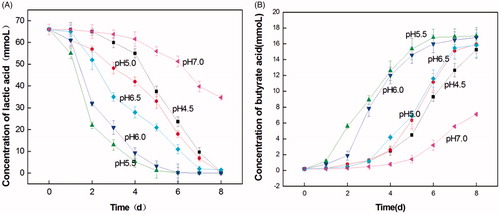
The growth characteristics of LY33 at the initial pH of 5.5 and in the different initial concentrations of lactic acid are shown in Figure . As shown in the figure, with the increase in the initial lactic acid concentration, the transformation of lactic acid decreased, and the production of butyric acid decreased. This suggests that a high lactic acid concentration inhibits the growth of LY33. Generally, when the initial concentration of lactic acid was 132 mmol, the utilisation of lactic acid was higher than that at other concentrations. Within 5 days, all lactic acid was transformed into 21 mmol butyric acid.
Figure 6. The growth feature of lactate-utilising and butyrate-producing at different initial lactate concentrations. (A) The curve of lactate-utilising by LY33 at different initial lactate concentrations. (B) The curve of butyrate-producing by LY33 at different initial lactate concentrations. With the increase of initial lactic acid density, the transformation of lactic acid decreased and the production of butyric acid decreased. And when the initial density of lactic acid was 132mmol, the utilisation of lactic acid was highest.
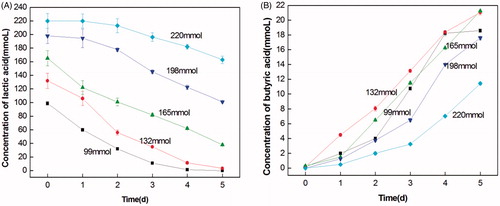
Experimental results for the co-culture of lactic acid bacteria and pathogens
Strain LY33 was co-cultured with E. coli, Salmonella, and lactic acid bacteria, and the effects are shown in Figure . The results showed that compared to the independently cultured E. coli and Salmonella strains, the number of co-cultured strains did not differ greatly at first. Over time, the co-cultured strains decreased gradually compared to the independently cultured strains. After 20 h of the test, the number of co-cultured E. coli was significantly fewer than the number of single cultured E. coli. This particularly affected the Salmonella strain, which after 12 h, had declined significantly in comparison to the single cultured strain. In the case of the lactic acid bacteria, as more time passed, their number of co-cultured strains became gradually higher than that of the single cultured strains. After 20 h had passed, the number of strains in two groups showed significant differences (p < .05), the co-cultured lactic acid bacteria and strain LY33 surpassed the single-cultured bacteria in number.
Figure 7. Co-culture of strain LY33, E.coli, Salmonella and Lactobacillus. (A) As the extension of time, the number of LY33 co-cultured Escherichia coli decreased gradually in comparison to the single cultured Escherichia coli. After 20h, the number of co-cultured Escherichia coli was significantly lower than that of single cultured. (B) As the extension of time, the number of LY33 co-cultured Salmonella decreased gradually in comparison to the single cultured Salmonella. After 12h, the number of co-cultured Salmonella was significantly lower than that of single cultured. (C) As the extension of time, the number of LY33 co-cultured Lactobacillus increased gradually in comparison to the single cultured Lactobacillus. After 20h, the number of co-cultured Lactobacillus was significantly higher than that of single cultured. CFU: Colony-Forming Units.
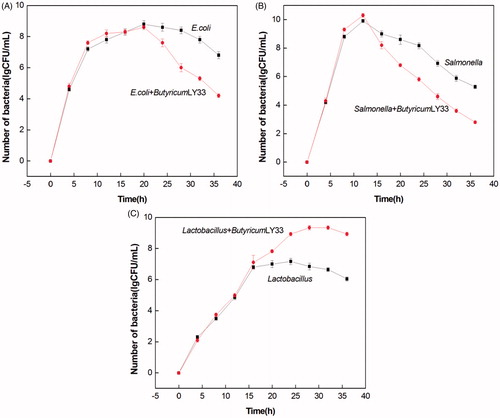
Impacts on the intestinal function of weaned piglets fed with C. butyricum
The impacts on the intestinal function of weaned piglets fed with C. butyricum are shown in Table . The results indicated that the test group fed with C. butyricum had significantly reduced the levels of intestinally concentrated ammonia and pH. The test group showed a 20.49% decrease in the caecum and a 24.92% decrease in the colon in terms of the concentration of ammonia and 10.21% reduction in the caecum and 10.67% reduction in the colon in terms of pH. These results were significantly different from those of the control group (p < .05). The activity of digestive enzymes in the test group was higher compared to that of the control group. In the test group, amylase and protease in the duodenum increased by 19.81% and 9.14%, respectively, and amylase, lipase and protease in the jejunum increased by 8.23%, 10.08%, and 6.79%, respectively, compared to those of the control group (p < .05). In the test group, the villus length and V/C value were greater than those of the control group, but the crypt depth decreased. In the test group, the duodenum villus length and V/C value increased by 18.47% and 25.46%, respectively, and crypt depth decreased by 5.50%; the jejunum villus length and V/C value increased by 16.82% and 26.32%, respectively, resulting in a significant difference (p < .05) from those of the control group.
Table 3. Effects of Clostridium butyricum preparation on intestinal function in weaning piglets.
Discussion
Despite feed fermented by probiotics such as lactic acid bacteria, which can accumulate lactic acid inside animals, many other microbes in the alimentary canal, human or animal, can also generate lactic acid, such as Lactobacillus acidophilus, Streptococcus faecalis and Enterococcus faecium (Heilig et al. Citation2002). Lactic acid plays a large role in the acidification of feed, lowering the pH of the gastric contents of livestock, eradicating intestinal pathogens, improving the microbial communities and stimulating the secretion of digestive enzymes. However, too much lactic acid can cause ulcerative colitis and short bowel syndrome (Lindgren and Dobrogosz Citation1990). Furthermore, great importance is attached to butyric acid because of its ability to maintain the intestinal physiological balance and epithelial cell integrity and prevent enteritis and diabetes. Therefore, research on C. butyricum, which can metabolise lactic acid and generate butyric acid, and its fermented preparations have been brought to the forefront by researchers, and it is important for practical applications to further develop probiotic feed through research into C. butyricum because of its utilisation of lactic acid (Kanai et al. Citation2015).
Drawing attention to the isolation method and study of intestinal lactate-utilising bacteria, this study isolated strain LY33, which rapidly utilises lactic acid and has a high conversion rate of butyric acid from the intestinal contents of healthy piglets. The strain generated butyric acid with the help of lactic acid. It showed a certain amount of resistance to heat, gastric acid, and bile salts, produced hydrogen peroxide and natural antibiotics, and reduced innocuous substances, including ammonia and amines. It also enhanced the activity of digestive enzymes in animal intestines and improved the intestinal function of the piglets. The strain LY33 had a certain inhibitory effect on Escherichia coli, Salmonella and other pathogenic bacteria. Most likely, C. butyricum, as an anaerobic probiotic, consumed large amounts of intestinal oxygen due to its growth and propagation in the alimentary canal, thus decreasing the number of aerobic pathogens by creating an oxygen-free environment to inhibit and kill pathogens (Zhang et al. Citation2012). Additionally, the large amount of growth of C. butyricum generated more butyric acid to lower the pH, while it inhibited the growth of pathogens including E. coli and Salmonella. However, the co-cultured strain LY33 and lactic acid bacteria surpassed the single-cultured bacteria in number, it might be that the isolated C. butyricum LY33 were able to utilise lactic acid as a source of carbon to steadily multiply their number, thus leading to more butyric acid and a lower pH, and lactic acid bacteria could also propagate in an acidic environment with the help of their resistance to acidity. In addition, the addition of LY33 strain preparation can improve the digestive enzyme activity of piglets, the results of this paper research showed that rations with added C. butyricum preparations could help piglets secrete amylase, lipase and protease and enhance the activity of the digestive enzymes, which may accelerate intestinal digestion as well as the absorption of substances in the piglets’ intestines. Furthermore, dietary supplementation of LY33 strain preparation can enlarge the villus length, V/C value and reduce the crypt depth in duodenum, jejunum and ileum, and it was beneficial to improve the shape of intestinal development and promote the absorption of nutrients. Therefore, strain LY33 can be used as a new microbial resource to produce microecology preparations and compounds with probiotics including lactic acid bacteria, bacilli, and bifidobacteria to strengthen the performance of the preparations (Meng et al. Citation2010) compared to the non-spore-forming live bacteria preparations widely used today, C. butyricum, with its co-cultured bacteria preparations, has a broader market prospect than the preparations used today.
In the production of microecology preparations, maintaining more nutrients and avoiding waste requires granulation of the feed. Only by withstanding high temperatures during the granulation process can exogenous probiotics play a role in the intestines of livestock. Before they enter the animal’s intestines, probiotics must go through strongly acidic gastric fluid, at pH lower than 3.5. Exogenous probiotics must offset the impact of gastric acid to function in the intestines. Although bile promotes the digestion and absorption of fat, it is detrimental to microbes. Therefore, probiotics must also be able to tolerate bile salt before arriving in the intestines (De Angelis et al. Citation2006). In this study, the screened strain LY33 was provided with endospores to increase its resistance to certain detrimental elements, such as gastric acid and bile, making it a superior candidate for production, processing, and storage. Strain LY33 had a limited tolerance of heat and could maintain the most activity at temperatures no higher than 75 °C. The test indicated that in the production of preparations of strain LY33, high-temperature spray-drying cannot be applied. Additionally, after fermentation, the dehydration temperature should be lower than 75 °C, and when the feed is processed, the granulation temperature should be under 75 °C.
Conclusions
In this research, the strain C. Butyricum LY33 were isolated from the intestinal contents of healthy piglets fed with 4%-lactic acid-fermented fodder, and it had properties including the rapid utilisation of lactic acid and a high rate of butyric acid conversion. Strain LY33 was innocuous and heat resistant but not able to withstand high temperatures, it could withstand certain acidity, bile salt, and many antibiotics, can make good use of lactic acid, and showed certain defences to pathogens while promoting the growth of lactic acid bacteria. LY33 Strain preparation improves the intestinal function of weaned piglets, as it can significantly reduce the intestinal concentration of ammonia and pH, improve the digestive enzymatic activity and the shape of intestinal development, it has a certain applied value in the field.
Disclosure statement
No potential conflict of interest was reported by the authors.
Additional information
Funding
References
- Bevilacqua AE, Califano AN. 1989. Determination of organic acids in dairy products by high performance liquid chromatography. J Food Sci. 54:1076–1079.
- Ceska M. 1971. A new approach for quantitative and semi-quantitative determinations of enzymatic activities with simple laboratory equipment. Detection of amylase. Clin Chim Acta. 33:135–145.
- Clarke DJ, Morris JG. 1976. Butyricin 7423: a bacteriocin produced by Clostridium butyricum NCIB7423. J Gen Microbiol. 95:67–77.
- De Angelis M, Siragusa S, Berloco M, Caputo L, Settanni L, Alfonsi G, Amerio M, Grandi A, Ragni A, Gobbetti M. 2006. Selection of potential probiotic lactobacilli from pig feces to be used as additives in pelleted feeding. Res Microbiol. 157:792–801.
- Gao QX, Wu TX, Wang JB, Zhuang QC. 2011. Inhibition of bacterial adhesion to HT-29 cells by lipoteichoic acid extracted from Clostridium butyricum. Afr J Biotechnol. 10:7633–7639.
- Giménez JA, Sugiyama H. 1988. Comparison of toxins of Clostridium butyricum and Clostridium botulinum type E. Infect Immun. 56:926–929.
- Hamer HM, Jonkers D, Venema K, Vanhoutvin S, Troost FJ, Brummer RJ. 2008. Review article: the role of butyrate on colonic function. Aliment Pharmacol Ther. 27:104–119.
- Hayden UL, Mcguirk SM, West SEH, Carey HV. 1998. Psyllium improves fecal consistency and prevents enhanced secretory responses in jejunal tissues of piglets infected with ETEC. Dig Dis Sci. 43:2536–2541.
- Heilig HG, Zoetendal EG, Vaughan EE, Marteau P, Akkermans AD, De Vos WM. 2002. Molecular diversity of Lactobacillus spp. and other lactic acid bacteria in the human intestine as determined by specific amplification of 16S ribosomal DNA. Appl Environ Microbiol. 68:114–123.
- Kanai T, Mikami Y, Hayashi A. 2015. A breakthrough in probiotics: Clostridium butyricum regulates gut homeostasis and anti-inflammatory response in inflammatory bowel disease. J Gastroenterol. 50:928–939.
- Kelsic ED, Zhao J, Vetsigian K, Kishony R. 2015. Counteraction of antibiotic production and degradation stabilizes microbial communities. Nature. 521:516–519.
- Krogdahl A, Sell JL. 1989. Influence of age on lipase, amylase, and protease activities in pancreatic tissue and intestinal contents of young turkeys. Poult Sci. 68:1561–1568.
- Lawson P. 2016. The taxonomy of the genus Clostridium: current status and future perspectives. Microbiol Chin. 43:1070–1074.
- Le MH, Galle S, Yang Y, Landero JL, Beltranena E, Gänzle MG, Zijlstra RT. 2016. Effects of feeding fermented wheat with Lactobacillus reuteri on gut morphology, intestinal fermentation, nutrient digestibility, and growth performance in weaned pigs. J Anim Sci. 94:4677–4687.
- Liao XD, Ma G, Cai J, Fu Y, Yan XY, Wei XB, Zhang RJ. 2015. Effects of Clostridium butyricum on growth performance, antioxidation, and immune function of broilers. Poult Sci. 94:662–667.
- Lin BS, Luo J, Dai AL, Yang XY. 2010. Effects of micorbial fermentation feeds on growth performance of weaned piglets. Anim Husbandry Feed Sci. 2:11–13.
- Lindgren SE, Dobrogosz WJ. 1990. Antagonistic activities of lactic acid bacteria in food and feed fermentations. FEMS Microbiol Rev. 7:149–163.
- Mansuy L. 2012. Microbial influences on epithelial integrity and immune function as a basis for inflammatory diseases. Immunol Rev. 245:164–176.
- Meng QW, Yan L, Ao X, Zhou TX, Wang JP, Lee JH, Kim IH. 2010. Influence of probiotics in different energy and nutrient density diets on growth performance, nutrient digestibility, meat quality, and blood characteristics in growing-finishing pigs. J Anim Sci. 88:3320–3326.
- Missotten JAM, Goris J, Michiels J, Coillie EV, Herman L, Smet SD, Dierick NA, Heyndrickx M. 2009. Screening of isolated lactic acid bacteria as potential beneficial strains for fermented liquid pig feed production. Anim Feed Sci Technol. 150:122–138.
- Missotten JAM, Michiels J, Ovyn A, Smet SD, Dierick NA. 2016. Fermented liquid feed for weaned piglets: impact of sedimentation in the feed slurry on performance and gut parameters. Czech J Anim Sci. 60:195–207.
- Niekamp SR, Sutherland MA, Dahl GE, Salak-Johnson JL. 2007. Immune responses of piglets to weaning stress: impacts of photoperiod. J Anim Sci. 85:93–100.
- Sandholm M, Smith RR, Shih JC, Scott ML. 1976. Determination of antitrypsin activity on agar plates: relationship between antitrypsin and biological value of soybean for trout. J Nutr. 106:761–766.
- Smith F, Clark JE, Overman BL, Tozel CC, Huang JH, Rivier JE, Blikslager AT, Moeser AJ. 2010. Early weaning stress impairs development of mucosal barrier function in the porcine intestine. Am J Physiol Gastrointest Liver Physiol. 298:352–363.
- Tsukahara T, Ushida K. 2001. Organic acid profiles in feces of pigs with pathogenic or non-pathogenic diarrhea. J Vet Med Sci. 63:1351–1354.
- Wang XW, Gao PF, Yao GQ, Bao WC, Li J, Liu Q, Hu DG, Zhang HP. 2015. The advantages of lactobacillus fermented feed and its application in livestock and poultry breeding. Cereal Feed Ind. 12:47–50. (in Chinese)
- Whelan W, Nasr H. 1951. The amylase of Clostridium butyricum. Biochem J. 48:416–422.
- Wu N, Schmalz B, Fohrer N. 2011. A comparison of phytoplankton assemblages generated by two sampling protocols in a German lowland catchment. Ann. Limnol. 47:313–323.
- Xiao JF, Wu SK, Wen QQ. 2014. A review about weaned age on performance, digestion and immunity of piglets and discussion of appropriate weaning age. Chin J Anim Sci. 50:57–60. (in Chinese)
- Yang CM, Cao GT, Ferket PR, Liu TT, Zhou L, Zhang L, Xiao YP, Chen AG. 2012. Effects of probiotic, Clostridium butyricum, on growth performance, immune function, and cecal microflora in broiler chickens. Int Rev Poul Sci. 91:2121–2129.
- Zhang XY, Lu Y, Ma YL, Li LT. 2012. Research progress of physiological function and application of Clostridium butyricum. Food Nutr China. 18:31–35. (in Chinese)