Abstract
The aim of the study was to investigate element concentrations in the hair of growing and adult dogs. Overall, 39 dogs were recruited in a kennel, split in 4 groups: G1, 10 puppies of small size breeds; G2, 6 puppies of medium size breed; G3, 11 adult dogs of medium size breeds; G4, 12 adult dogs of toy breeds. The dogs of each group were fed 4 different complete diets, according to the requirements. Hairs were sampled at the beginning of the study (D0) and after 60 days (D60). Elements were analysed in triplicate using ICP-OES. In the hair of the adult dogs of the G3 group the concentration of Al (p < .01) was lower and in the G2 group the concentration of Zn was higher (p < .01) than in the other groups. Cu and Mn concentrations in the hair were higher (p < .01 and p < .05, respectively) in puppies fed G1 and G2 diets in comparison to adult dogs fed G3 and G43 diets. Hair concentrations of K, Li and Na were higher at D60 in comparison with D0 (p < .01). Correlations between concentrations in the hair of Al and Fe, Al and Mg and Fe and Mg were highly significant (p < .01). Present data do not support the use of element concentrations in the hair to assess their nutritional supply but, likely, they can be used in relation to physiological status of the animals. Further studies are required to investigate the factors affecting element concentrations in the hair of dogs.
Concentrations of Cu, Mn and Zn in the hair differed between growing and adult dogs;
Al concentration in hair was highly correlated with Fe and Mg;
Several factors other than nutritional supply regulate element deposition in the hair
Highlights
Introduction
The element status of an organism can be investigated in various biological fluids and tissues, as blood, urine and teguments (Długaszek Citation2019), and in some studies, hair has been considered a matrix to assess retrospectively element concentrations in relation to the nutritional status of an individual (Wójciak et al. Citation2004). According to these authors, the hair closer to the scalp is highly related to the nutrient intakes of minerals in the previous 6–8 weeks, even though the chemical form of element supplementations has also to be taken into consideration (Kuhlman and Rompala Citation1998).
The biological mechanisms involved in element deposition in hair are very complex, depending upon the concentration in the blood (Długaszek Citation2019), even though the breed of the dogs can in some circumstances be another factor to be considered (Davies et al. Citation2017). Nonetheless, some physiological conditions can influence the utilisation of some elements, as was reported in dogs (So et al. Citation2016) and humans (Song et al. Citation2007; Suliburska Citation2011). For these reasons, other authors did not considered hair a reliable matrix to assess dietary intakes of elements in animals (Combs Citation1987) and humans (Cho and Yang Citation2018; Długaszek Citation2019).
The analysis of elements can also be named as ionome, this term referring to the amount of mineral and trace elements found in an organism (Salt et al. Citation2008). This concept was recently applied to dogs (So et al. Citation2016), thanks to the recent availability of high-throughput screening (HTS) technologies of inductively coupled plasma (ICP) spectroscopy, often integrated with mass spectrometry. Nevertheless, the definition of reference values of essential macro and micro nutrient and toxic elements can form an interesting integrating diagnostic tool to investigate, at least in part, the healthy condition of an individual (Klevay et al. Citation2004; Davies et al. Citation2017; Skalnaya et al. Citation2018; Stepanova et al. Citation2018). In fact, the advantages of using hair matrix is that it is easy to collect and, in the case of pets, can be sampled directly from the owner. Moreover, hair analysis allow to trace back the exposure to polluted environment or to high ingestion of toxic elements, as Pb, Cd, Ni, Cr and Hg (Nadal et al. Citation2005; Xie et al. Citation2017; Stepanova et al. Citation2018). However, in the case of dogs, there is still a paucity of data about the element concentrations in hair and on the factors affecting them (So et al. Citation2016; Davies et al. Citation2017), which makes all these aspects interesting to unravel.
The aim of this study was to gain additional information on element concentrations in hair of growing and adult dogs housed in a common environment and under controlled feeding regimes in a defined window time. In the study, attention was paid especially for macro and micro nutrients, considered the important role they have in the regulation of physiological processes.
Material and methods
Animal, diets and experimental design
For the study, 39 dogs were recruited in the same kennel. The inclusion criteria were a healthy condition, as confirmed by clinical examinations, free from external and internal parasites, and without drugs administration in the last 4 months. All protocols, procedures and the care of the animals complied to Italian legislation on animal care (DL n.116, 27/1/1992) and the study was approved by the ethical committee of the University of Udine (OBPA University of Udine, Protocol #2/2017). At the end of the study, dogs returned to the diet regularly fed in the kennel.
According to kennel availability, the 39 dogs were allocated to 4 groups: G1 group, 10 puppies of small size breeds; G2 group, 6 puppies of medium size breed; G3 group, 11 adult dogs of medium size breeds; G4 group, 12 adult dogs of toy breeds. Dogs were housed alone in box with a free access to an external area and free access to tap water from municipality. Details of the dogs enrolled for the study are reported in Supplementary Table S1. The adult dogs (G3 and G4 groups) were resident in the kennel from at least 6 months and received the commercial kibbles K3 and K4, respectively, since at least 2 months from the beginning of the study. The puppies (G1 and g2 groups) were born in the kennel and weaned with commercial kibbles K1 and K2, respectively. These foods had chemical and nutritive compositions appropriated for the age and the size of the dogs and the daily amount of kibble offered to the dogs followed the doses indicated in the commercial labels.
At the beginning of the study (D0), a sample of hair was collected with a scissor from the medial part of the left leg, taking care to cut as closer to the skin as possible. The area was chosen since it is not in contact with leash or collar and not easy visible. The same area was sampled with the same procedure after 60 days (D60). The D0 hairs were sampled on 3rd of June and the D60 on 2nd of August. Just after the collections, the samples were stocked in a paper envelope and stored until element analysis.
Chemical and element analysis of the kibbles and hair samples
Before the beginning of the study, a sample of each of the 4 kibbles was collected and analysed for dry matter, crude protein, crude fibre, lipids and ash using the methods prescribed by AOAC (Citation2005).
For element analysis, about 1000 g of kibbles were grinded into fine powder with a blender and dried in an oven at 105 °C for 24 h and then left to equilibrate in a desiccator for 30 min before weighting. For hair about 500 mg of sample was cleaned by soaking for 2 h in a 2:1 mixture of methanol and chloroform, followed by washing twice in MilliQ water (O’Connell et al. Citation1999). This procedure was applied to remove the hair samples from environmental contaminants before the element analysis. Samples were then dried to constant weight at 105 °C for 24 h and left to equilibrate in a desiccator for 30 min before weighting. After thoroughly mix, a sample of 200 mg of each kibble and hair was mineralised at 180 °C for 10 min with a microwave system Mars 5 (CEM, MARS Xpress, Matthews, NC, USA) in a Teflon digestion tube containing 1 ml of H2O2 at 30% (v/v) (Sigma Aldrich, Milan, Italy) and 9 ml of HNO3 at 65% (v/v) (PanReac AppliChem VWR, Milan, Italy), according to US Environmental Protection Agency (USEPA) procedure number 3052 (USEPA Citation1996). After mineralisation, the digestion tubes were cooled to room temperature and the liquid samples were filtered (0.45 μm PTFE), diluted (1:20) and analysed for the element contents using an inductively coupled plasma optical emission spectroscopy ICP-OES (Vista MPX, Varian Inc., Palo Alto, CA, USA) with detection limit <1 ppm.
The accuracy of the analytical procedure adopted for ICP-OES analysis was checked by running standard solutions of elements of 0.005, 0.05, 0.5, 5.0 and 50 ppm every 20 samples. Yttrium was used as the internal standard. Quality control for ICP-OES was performed using reagent blank samples, and triplicate readings for each sample. Certified standard reference material (tomato leaves 1573a from the National Institute of Standards and Technology, Gaithersburg, MD, USA) was treated as the samples (n = 3). The recovery of the elements in the standard material was on average 97% of the certified values with an RSD average of 1.3%. Method detection limits (μg/l) in ICP-OES were: 4.0 for Al, 1.0 for Ag, 0.7 for Ba, 0.3 for Ca, 0.5 for Cd, 1.2 for Co, 1.0 for Cr, 1.5 for Cu, 0.9 for Fe, 4.0 for K, 1.0 for Li, 0.1 for Mg, 0.13 for Mn,1.5 for Na, 2.1 for Ni, 25.0 for P, 8.0 for Pb, 0.1 for Se and 0.8 for Zn.
Cortisol determination
For the extraction of cortisol from the hair, 150 mg of sample were weighted from each sample and placed into 15 ml glass vial (Accorsi et al. Citation2008). Samples were washed three times with 2.5 ml of isopropanol (2-propanol 99.5%, Sigma Aldrich, Milan, Italy) and 3 min of vortex. Isopropanol was discarded after each wash and after the final wash hair samples were placed on a plastic disk and let dry for 48 h at room temperature. Dried hair samples were trimmed with a blade and 50 mg of trimmed hair were weighted and placed into a 15 ml glass centrifuge tube with 5 ml of methanol. Samples were incubated in water bath at 45 °C for 18 h under moderate shaking. At the end of incubation, tubes were centrifuged at 5000 g for 10 min and 2 ml of supernatant was transferred to a 1.5 ml Eppendorf tube and centrifuged in a spin-vacuum (Centrifugal System, RC 10.10, Jouan, Cologno Monzese, Italy) at 40 °C until completed evaporation of methanol. Dried samples were then reconstituted with 0.6 ml of PBS, with 0.1% bovine serum albumin (Sigma Aldrich, Milan, Italy) and frozen until analysis. Cortisol concentration was measured according to the RIA procedure described by Sgorlon et al. (Citation2015). All samples were assayed in duplicate, the sensitivity of the assay was 3.125 pg/well and the intra-assay and inter-assay coefficients of variation in high and low cortisol reference samples were 5.9% and 9.1% and 13.5% and 15.1%, respectively.
Statistical analysis
Data were imputed on a spreadsheet and analysed with the SPSS software (SPSS Citation2018). Before statistical analysis, normality of distribution was checked for each element with the nonparametric Kolmogorov-Smirnoff test. Data of elements were not normally distributed and a natural logarithmic transformation was then applied. The Kolmogorov–Smirnoff test run on logarithmic data indicated a Gaussian distribution. The natural logarithmic concentrations of the elements in the hair were analysed using a mixed model procedure, with fixed factors for group (4 levels; G1, G2, G3 and G4), time of sampling (2 levels, D0 and D60), sex (2 levels, male and female), group and time of sampling (G x D) and group and sex (G x S) interactions. For each element, the covariate for element intake was applied and dogs, repeated within time, were considered as random factor.
The rejection of null hypothesis was considered for p<.05, with the least square differences method and correction for multiple testing with FDR. Pearson correlations among elements were calculated. Cortisol concentration of hair was analysed using the ANOVA model, with group (4 levels; G1, G2, G3 and G4) as fixed factor.
Results
The crude protein and crude fibre contents of the complete diets () differed between puppies (G1 and G2) and adult dogs (G3 and G4), whilst the oil and fat contents were lower for the G3 group in comparison to the other groups. The element concentrations varied widely between diets, but not relevant differences between puppies and adult kibbles were observed. Only Al content was consistently higher in the puppy diets. The concentrations of Ag, Cd, Co, Cr, Ni and Pb elements were below the detection limits of the ICP-OES instrument for all or for some of the kibbles.
Table 1. Proximate analysis and concentrations of elements of the kibbles administered to the dog during the study.
According to the labelling of the 4 kibbles, the form of mineral additives supplemented in the diets was the same for Ca (calcium carbonate and calcium phosphate), Cu (copper sulphate pentahydrate), Fe (iron carbonate), Mn (manganese oxide), Na (sodium chloride) P (calcium phosphate) and Zn (zinc oxide). The mean intake of kibbles in the 4 groups () was significantly higher (p < .01) for the adult dogs of medium size (G3) in comparison to the others groups. The lowest kibbles intakes was recorded for the puppies of small size (G1) and adult of small size (G4).
Figure 1. Average daily intake of kibbles during the study. A, B and C denote means significantly different for p < .01. G1: Growing dogs of small size, fed K1 diet; G2: Growing dogs of medium size, fed K2 diet; G3: Adult dogs of medium size, fed K3 diet; G4: Adult dogs of small size, fed K4 diet. For K1, K2, K3 and K4 diets, see .
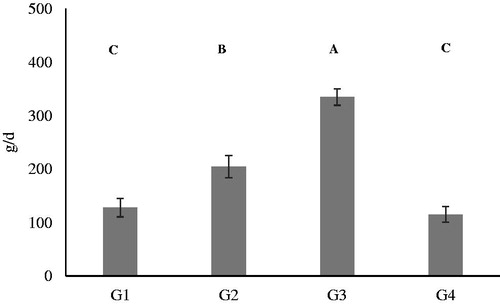
The mean and standard deviation of the concentrations of the element in the hair samples before natural logarithm transformation between the 4 groups at D0 and D60 is reported in the . The hair concentrations of Ag, Cd, Co, Cr, Ni and Pd were lower than the detection limits for most of the hairs samples of the dogs collected either at D0 or D60 and no statistical analysis was performed. Statistical analysis with mixed model of the natural logarithmic transformed data of element concentrations revealed significant differences between diets for Al, Cu, Mn and Zn ( and ). In particular, the adult dogs of the G3 group showed the lowest hair concentrations of Al, Cu (p < .01), Mn and Zn (p < .05), while the hairs of puppies fed K1 and K2 diets (G1 and G2 groups) had a significant higher content of Cu and Mn. The G2 group showed a significantly higher concentration of Zn in comparison to G3 and G4 groups.
Figure 2. Effect of the diets on the mean concentrations of Al, Cu, Mn and Zn in the hair of dogs and reported as natural logarithm. These elements significantly differed between groups of dogs () and the data reported are the average concentrations of the hair sampled at the beginning of the study (D0) and after 60 days (D60). Within each diagram, a, b denote means which significantly differ for p < .05 and A, B denote means which significantly differed for p < .01. G1: Growing dogs of small size, fed K1 diet; G2: Growing dogs of medium size, fed K2 diet; G3: Adult dogs of medium size, fed K3 diet; G4: Adult dogs of small size, fed K4 diet. For K1, K2, K3 and K4 diets, see Table 1.
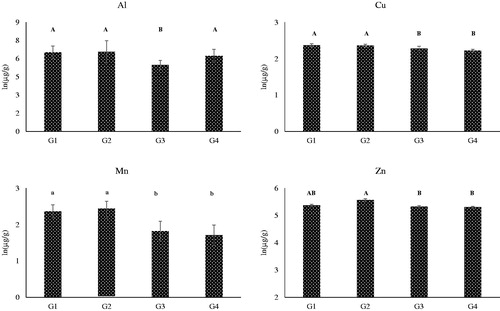
Table 2. Mean concentrations and number of samples below the detection limits of the elements in the hair sampled from dog fed commercial diets at the beginning of the study (D0) and after 60 days (D60).
Table 3. Results of the statistical analysis and estimated mean concentrations of the elements in the hair sampled from dog fed commercial diets at the beginning of the study (D0) and after 60 days (D60). Since data were not normally distributed, a natural logarithmic transformation was applied.
Time of sampling () caused a significant increase of K, Li and Na (p < .01) concentrations in the hair at D60 in comparison to D0. Cortisol concentration in hairs was measured at the end of the study (D60) and represented the deposition of the hormone during the time window of 60 days of regrowth. No significant differences were observed between the 4 dietary groups ().
Figure 3. Effect of the time of sampling on the concentration of K, and Li and Na in the hair of dogs at the beginning (D0) and after 60 days (D60) of the study. These elements significantly differed between time of sampling for the groups G1, G2 G3 and G4 (). G1: Growing dogs of small size, fed K1 diet; G2: Growing dogs of medium size, fed K2 diet; G3: Adult dogs of medium size, fed K3 diet; G4: Adult dogs of small size, fed K4 diet. For K1, K2, K3 and K4 diets, see Table 1.
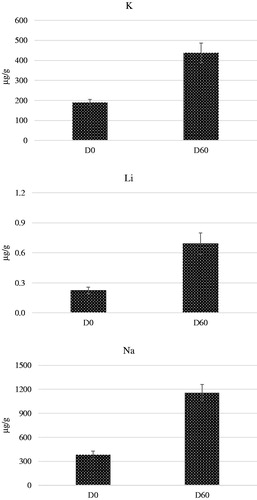
Figure 4. Estimated means of cortisol concentrations in the hair samples regrowth after 60 days from the beginning of the study. G1: Growing dogs of small size, fed K1 diet; G2: Growing dogs of medium size, fed K2 diet; G3: Adult dogs of medium size, fed K3 diet; G4: Adult dogs of small size, fed K4 diet. For K1, K2, K3 and K4 diets, see Table 1.
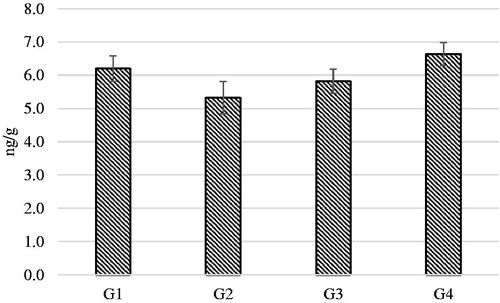
The correlations between concentrations in the hair of Al and Fe, Al and Mg and Fe and Mg were highly significant (p < .01), with coefficients equal to 0.922, 0.715 and 0.978, respectively ().
Figure 5. Significant correlations (p < .01; n = 78) between the concentrations of Aluminium and Iron (Al vs Fe; r = 0.922), Aluminium and Magnesium (Al vs Mg; r = 0.715) and Magnesium and Iron (Fe vs Mg; r = 0.978) in the hair of the 4 groups of dogs fed different diets. Correlations were calculated for the hairs sampled at the beginning of the study and after 60 days of regrowth (n = 78).
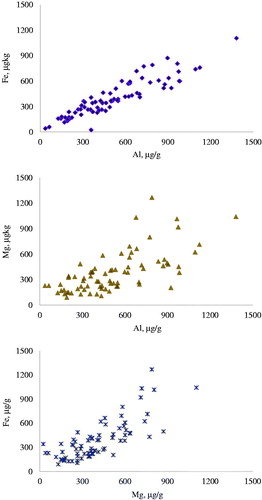
Discussion
From a nutritional point of view, some elements are considered essential for vertebrates and they fulfil several roles, as structural, physiological, catalytic and regulatory. From a nutritional point of view, elements are often classified as macroelements, meaning that the inclusion in the diet is in the order of g/kg (Ca, Cl, K, Mg, Na, P, S) and microelements, which are included in mg/kg (Cr, Cu, F, Fe, I, Mn, Si, Se, Zn). However, other elements are involved in biological processes, and their inadequate supply or excess of intake can lead to imbalances and toxicity.
Available data on the concentrations of elements in the hair of dogs are very limited, but the identification of threshold values of elements – if any – in the hair of healthy dogs could be of interest as a complementary diagnostic tools. Davies et al. (Citation2017) analysed the element concentrations in the hair of Labrador and other breeds of dogs. The animals were healthy or affected from medial coronoid process disease and the authors found significant variations for some elements as a function of disease and breed. The concentrations measured by Davies et al. (2017) differed from the data reported in the present study for Al, Fe, K and Mn (Al: from 13 to 50 mg/kg; Fe: from 17 to 59 mg/kg; K: 3 to 89 mg/kg; Mn: 0.4 to 2.9 mg/kg); instead, for other elements, the concentrations were similar (). So et al. (Citation2016) published the ionomic profile of canine hair pre and post lipopolysaccharide (LPS) induced stress. Before the treatment, the concentrations in the hair of Al was close to that reported by Davies et al. (2017), but for Fe, K and Mn data differed from those reported in present study and from those of Davies et al. (2017). Other differences between the 2 studies and the present data were shown for Cu, Zn, Mg. The reasons of the observed discrepancy are not easy to explain, and the lack of systematic data on the element profile of hair in dogs in relation to the diet, breed and environment do not allow to explain these differences.
For Cu and Mn, significant differences were observed between young (G1 and G2) and adult (G3 and G4) dogs (), but for Al the lowest concentration was measured in G3 dogs and for Zn the highest concentrations were detected in G2 dogs. The additives of the 4 kibbles had the same form and, but the bioavailability of elements depends from several factors, other than their chemical form (Kuhlman and Rompala Citation1998). Once absorbed from the gut, the incorporation of elements in the hair follicles depends upon the metabolic activity and serum concentrations (Suliburska et al. Citation2011; So et al. Citation2016; Długaszek Citation2019). It must be considered that Cu, Mn and Zn are enzymes cofactors and components of redox enzymes (Cu: superoxide dismutase, cytochrome oxidase; Mn: arginase, glycosyltranferase; Zn: superoxide dismutase), metalloproteins (Cu: ceruloplasmin; Zn: metallothionein) and hormones (Zn: insulin, growth hormone, sexual hormones). Thus, the observed variations are presumably related to the variable physiological involvements of these elements, especially when comparing growing with adult animals.
Moreover, positive and negative interactions among elements and other nutrients of the diet (Długaszek Citation2019), age (Suliburska Citation2011) and healthy conditions (Suliburska et al. Citation2011; So et al. Citation2016) have been reported to affect element concentrations in the hair. For instance, dietary intake of Ca, P and Fe affects the uptake of other elements in the hair (Długaszek Citation2019). The content of Ca, Mg, Fe and Zn in the diets of women correlated inversely with Cu level in the hair (Suliburska Citation2011). Pathological conditions can modify element concentrations in the hair both in humans (Cho and Yang Citation2018; Skalnaya et al. Citation2018) and dogs (So et al. Citation2016; Davies et al. Citation2017). Nonetheless, contact of the hair with the secretions of sebaceous, apocrine and eccrine glands can contribute to the elemental profile and, probably, for this reason significant correlations between concentrations in hair and elemental intakes are usually low (Combs Citation1987). Environmental pollution too has been reported to affect element concentrations in the hair (Combs Citation1987; Nouioui et al. Citation2018; Stepanova et al. Citation2018; Długaszek Citation2019).
The high difference of Li, K and Na concentrations (p < .01) measured in the hairs sampled at D0 in comparison to D60 (Figure ) would indicate that weather is another factor playing a role in the regulation of element concentrations in hairs. The D0 samples were collected at the beginning of June and the D60 at the beginning of August, and the hair concentrations of these cations could have traced the variation of depositions from the mild to the hot period of the year. In 2017, the weather was particularly dump and hot in June and July in North-East of Italy, as can be confirmed from the climate records. So et al. (Citation2016) reported a relevant and significant increase of Na and K in hair after LPS treatment and the authors considered this as an effect of the enhancement of adrenal aldosterone in the serum of the dogs after immune stress. A similar mechanism can be claimed for the dogs exposed to high temperature. It is noteworthy that also the study of Davies et al. (2017) a significant increase of some elements in seek dogs was observed.
The dogs enrolled in the present study were clinically healthy and during the 60 days of the trial no signs of diseases were reported. To assess if dogs were exposed to environmental stressors, hair cortisol concentrations in the hair samples collected at D60 was also measured (). Cortisol is considered an endocrine biomarkers of environmental stress (Sandri et al. Citation2015; So et al. Citation2016; Colussi et al. Citation2018) and its concentration in the hair has been used to assess medium term exposure to unfavourable conditions (Accorsi et al. Citation2008; Bryan et al. Citation2013). The concentration of hair cortisol did not differ between groups and were within the normal values reported from the authors, suggesting that dogs did not have any particular challenge during the 60 days of the study.
Interestingly, high (p < .01) coefficients of correlation were found for Al, Fe and Mg (Figure ). Fe metabolism is highly regulated at intestinal, cellular and renal levels and that this element participates to several activity, including oxygen transportation at blood and muscular levels. In the research of Davies et al. (2017), Fe concentration in the hair was not related to breed or disease conditions and in the study of So et al. (Citation2016), LPS treatment had very small effect on Fe in the hair. Fe binds to transferrin, entering the cellular through specific transferrin receptors (Pérez et al. Citation2001), but also a non-transferrin bound iron system of transport has been reported (Pérez et al. Citation2005). Al and Fe have physical and chemical characteristics in common, as ionic radius, charge density, chelation by particular compounds (Ward et al. Citation2001), and a competition with abiogenic Al3+ ions in protein binding sites has been reported (Dudev et al. Citation2018; Długaszek Citation2019). This can explain the strict correlation between Al and Fe reported in Figure . If the presence of Al was related to the iron-aluminium alloy used in feed manufacturing is not possible to state, but the G1 and G2 diets (with high concentrations of Al) were manufactured by the same company, and the G3 and G4 by another industry (with low concentrations of Al). These aspects deserves further investigations, since Al accumulation in the body was considered responsible for the etiopathogenesys of erythropoietic (Nesse and Garbosa Citation2001) and nervous (Zatta et al. Citation2003) systems related diseases. For Mg, quantitative relationship with Fe in body fluids and red cells (Długaszek Citation2019) and hair (Długaszek et al. Citation2014) have been reported, but for these elements other factors, as breed, probably interacted with the deposition in the hair.
Conclusions
The study was performed to evaluate the concentrations of elements in the hair of growing and adult dogs fed diets differing for composition and elemental supply. To assess the mechanisms of element uptake and transfer from blood to tissues and hair, deeper investigations are required, as blood and biopsy samples, but bioethical issues limit the accessibility of these types of specimen. Instead, hair is a very simply, inexpensive and not invasive matrix which can offer the opportunity to retrospectively investigate the clinical conditions of dogs.
The data gathered from this study can implement the information on the concentrations of elements in the hair, offering preliminary data for further investigations the factors regulating elemental concentrations in the hair of dogs.
Disclosure statement
No potential conflict of interest was reported by the authors.
References
- Accorsi PA, Carloni E, Valsecchi P, Viggiani R, Gamberoni M, Tamanini C, Seren E. 2008. Cortisol determination in hair and faeces from domestic cats and dogs. Gen Comp Endocrinol. 155:398–402.
- Association of Official Analytical Chemists (AOAC). 2005. Official methods of analysis. 18th ed. Gaithersburg, MA (USA): Official Methods of Analysis of AOAC International.
- Bryan HM, Adams AG, Invik RM, Wynne-Edwards KE, Smits JE. 2013. Hair as a meaningful measure of baseline cortisol levels over time in dogs. J Am Assoc Lab Anim Sci. 52:189–196.
- Cho JM, Yang HR. 2018. Hair mineral and trace element contents as reliable markers of nutritional status compared to serum levels of these elements in children newly diagnosed with inflammatory bowel disease. Biol Trace Elem Res. 185:20–29.
- Colussi A, Stefanon B, Adorini C, Sandri S. 2018. Variations of salivary cortisol in dogs exposed to different cognitive and physical activities. It J Anim Sci. 17:1–8.
- Combs DK. 1987. Hair analysis as an indicator of mineral status of livestock. J Anim Sci. 65:1753–1758.
- Davies M, West J, Williams C, Gardner DS. 2017. Mineral status in canine medial coronoid process disease: a cohort study using analysis of hair by mass spectrometry. Vet Rec. 180:448.
- Długaszek M. 2019. Studies on relationships between essential and toxic elements in selected body fluids, cells and tissues. Chem Biol Interact. 297:57–66.
- Długaszek M, Skrzeczanowski W, Kaszczuk M. 2014. Age-related mineral status of females and males in human health risk assessment. Trace Elem Electroly. 31:131–140.
- Dudev T, Cheshmedzhieva D, Doudeva L. 2018. Competition between abiogenic Al3+ and native Mg2+, Fe2+ and Zn2+ ions in protein binding sites: implications for aluminum toxicity. J Mol Model. 24:55.
- Klevay LM, Christopherson DM, Shuler TR. 2004. Hair as a biopsy material: trace element data on one man over two decades. Eur J Clin Nutr. 58:1359–1364.
- Kuhlman G, Rompala RE. 1998. The influence of dietary sources of zinc, copper and manganese on canine reproductive performance and hair mineral content. J Nutr. 128:2603–2605.
- Nadal M, Bocio A, Schuhmacher M, Domingo JL. 2005. Monitoring metals in the population living in the vicinity of a hazardous waste incinerator: levels in hair of school children. Biol Trace Elem Res. 104:203–213.
- Nesse A, Garbossa G. 2001. Aluminium toxicity on erythropoiesis. Mechanisms related to cellular dysfunction in Alzheimer’s disease, in: C. Exley (Ed.), Aluminium and Alzheimer’s disease. The science that describes the link. Amsterdam (NL): Elsevier Science B.V, pp. 261–277.
- Nouioui MA, Araoud M, Milliand ML, Bessueille-Barbier F, Amira D, Ayouni-Derouiche L, Hedhili A. 2018. Evaluation of the status and the relationship between essential and toxic elements in the hair of occupationally exposed workers. Environ Monit Assess. 190:731.
- O’Connel TC, Hedges RE. 1999. Investigations into the effect of diet on modern human hair isotopic values. Am J Phys Anthrop. 108:409–425.
- Pérez G, G, Garbossa G, Di Risio C, Vittori D, Nesse A. 2001. Disturbance of cellular iron uptake and utilisation by aluminium. J Inorg Biochem. 87:21–27.
- Pérez G, Pregi N, Vittori D, Di Risio C, Garbossa G, Nesse A. 2005. Aluminum exposure affects transferrin-dependent and -independent iron uptake by K562 cells. Biochim Biophys Acta. 1745:124–130.
- Salt DE, Baxter I, Lahner B. 2008. Ionomics and the study of the plant ionome. Annu Rev Plant Biol. 59:709–733.
- Sandri M, Colussi A, Perrotta MG, Stefanon B. 2015. Salivary cortisol concentration in healthy dogs is affected by size, sex, and housing context. J Vet Behav Clin App Res. 10:302–306.
- Sgorlon S, Fanzago M, Guiatti D, Gabai G, Stradaioli G, Stefanon B. 2015. Factors affecting milk cortisol in mid lactating dairy cows. BMC Vet Res. 11:259.
- Skalnaya MG, Skalny AV, Grabeklis AR, Serebryansky EP, Demidov VA, Tinkov AA. 2018. Hair trace elements in overweight and obese adults in association with metabolic parameters. Biol Trace Elem Res. 186:12–20.
- So KM, Lee Y, Bok JD, Kim EB, Chung MI. 2016. Analysis of ionomic profiles of canine hairs exposed to lipopolysaccharide (LPS)-induced stress. Biol Trace Elem Res. 172:364–371.
- Song CH, Barrett-Connor E, Chung JH, Kim SH, Kim KS. 2007. Associations of calcium and magnesium in serum and hair with bone mineral density in premenopausal women. Biol Trace Elem Res. 118:1–9.
- Statistical Package for Social Science (SPSS). 2018. Advanced Statistics 25. Bologna (Italy): SPS srl.
- Stepanova NV, Fomina SF, Valeeva ER, Ziyatdinova AI. 2018. Heavy metals as criteria of health and ecological well-being of the urban environment. J Trace Elem Med Biol. 50:646–651.
- Suliburska J. 2011. A comparison of levels of select minerals in scalp hair samples with estimated dietary intakes of these minerals in women of reproductive age. Biol Trace Elem Res. 144:77–85.
- Suliburska J, Bogdański P, Pupek-Musialik D, Krejpcio Z. 2011. Dietary intake and serum and hair concentrations of minerals and their relationship with serum lipids and glucose levels in hypertensive and obese patients with insulin resistance. Biol Trace Elem Res. 139:137–150.
- US Environmental Protection Agency (USEPA). 1996. Soil screening guidance: technical background document. USEPA Rep. 540/R-95/128. Washington, DC (US): US Gov. Print. Office.
- Ward RJ, Zhang Y, Crichton RR. 2001. Aluminium toxicity and iron homeostasis. J Inorg Biochem. 87:9–14.
- Wojciak RW, Krejpcio Z, Czlapka-Matyasik M. 2004. Comparison of the hair bioelements in vegetarian and non-vegetarian women. Trace Elem Electrol. 21:141–144.
- Xie W, Peng C, Wang H, Chen W. 2017. Health risk assessment of trace metals in various environmental media, crops and human hair from a mining affected area. Int J Environ Res Public Health. 14:E1595.
- Zatta P, Lucchini R, van Rensburg SJ, Taylor A. 2003. The role of metals in neurodegenerative processes: aluminum, manganese, and zinc. Brain Res Bull. 62:15–28.