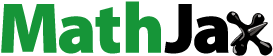
Abstract
Bananas are one of the most popular fruits worldwide, producing over 100 million tons in 2013. Banana peel, a byproduct that represents 40% of the entire banana’s weight, is rich in bioactive compounds and has a high antioxidant capacity. Therefore, the aim of this study is to use banana peel powder (BPP) as an antioxidant broiler feed additive. The bioactive compound and antioxidant capacity of BPP were determined. The results showed that BPP contained 872.7 GAE/100 g DM of total phenolic content, and gallocatechin was detected at a concentration of 91 mg/100 g DM, and BPP’s ferrous chelating ability, ferric ion reducing ability power, Trolox equivalent antioxidant capacity, and 1,1-diphenyl-2-picrylhydrazyl scavenging capacity indicated its high antioxidant capacity. A total of 216 one-day-old Ross 308 female broiler chicks were randomly assigned to one of four groups that received the basal diet supplemented with 0% (control), 0.5%, 1%, or 2% dry BPP for 35 d. The results showed that the 0.5% BPP-supplemented group had increased serum SOD activity and lowered serum malondialdehyde levels, while the liver mRNA expression of Nrf2, SOD, CAT, GPx, and HO-1 were upregulated in the 0.5% and 1% BPP-supplemented groups. In conclusion, the current study shows that BPP has a potent antioxidant capacity and dietary supplementation with 0.5% BPP enhances antioxidative status, suggesting that BPP has potential as an antioxidant feed additive.
Banana peel has abundant bioactive compounds and antioxidant capacity.
Banana peel enhance the antioxidant status of broilers by up-regulating Nrf2/ARE associated genes.
Banana peel has potential as an antioxidant feed additive in broiler chickens.
Highlights
Keywords:
Introduction
In the poultry industry, various environmental factors could negatively impact broilers during their growth period, including disease infection, stocking density, and heat stress. All of these can result in higher mortality, undesirable growth performance, and low meat quality, leading to economic losses (Estevez Citation2007; Heckert et al. Citation2002; Lara and Rostagno Citation2013). Oxidative stress is an imbalance in redox reactions, which is linked to environmental stressors, especially heat stress, and causes lipid peroxidation, protein deactivation, functional damage to mitochondria, and damage to DNA (Huang et al. Citation2015; Lee et al. Citation2019). In addition, lots of poultry industries across the globe are located in tropical and subtropical regions, suggesting that heat stress and oxidative stress could be one of the main factors causing economic losses.
Nuclear factor (erythroid-derived 2)-like 2 (Nrf2) is a transcription factor that binds to specific DNA sequences, namely antioxidant response elements (ARE) and downstream genes. These are responsible for triggering elements of the cell’s defense system, such as superoxide dismutase (SOD), catalase (CAT), glutathione reductase (Gr), and haem oxygenase-1 (HO-1), to raise the cell’s resistance to stress. According to our previous studies, phytochemicals from various plant materials exerted free radical scavenging effects and the ability to upregulate Nrf2 expression to further trigger downstream antioxidant mRNA expression, which had suggested that the plant materials could serve as natural antioxidants to solve the issue of oxidative stress in the poultry industry (Lee et al. Citation2017; Lin et al. Citation2017; Huang and Lee Citation2018).
Banana is a popular fruit worldwide with a mild flavour and special aroma. Its global production reached over 100 million tons in 2013, which is nearly 2 times higher than its production in 1993 (Vu et al. Citation2018). Banana peel, a byproduct of the banana processing industry, makes up approximately 40% of the whole fruit’s weight but has no further application except as general waste or compost. However, banana peel has been shown to have potent antimicrobial and antioxidant properties (Mokbel and Hashinaga Citation2005), which might be due to its abundant phenolic content, including flavan-3-ols and flavonols (Vu et al. Citation2018). In addition, many bioactive compounds have been detected in dried banana peel powder, including catechin, epicatechin, gallocatechin, and procyanidin (Rebello et al. Citation2014), which promoted the effects of the Nrf2-mediated defense system (Zhang and Tsao Citation2016). Furthermore, gallocatechin was 5 times higher in banana peel than in its pulp, indicating the peel is a high source of antioxidant compounds (Someya et al. Citation2002).
Due to its antioxidant effects, banana peel has potential to be utilised in the poultry industry since environmental stress during the growth period is often associated with oxidative stress, one of the major obstacles affecting chickens’ growth performance (Surai Citation2016). Therefore, the aim of this study is to use the peel derived from Taiwan’s banana industry as a feed additive in broiler chickens, with the expectation that it will enhance their antioxidant capacity.
Materials and methods
Sample preparation
Banana peel (derived from mature bananas, Musa AAA) was obtained from the Taiwan Banana Research Institute and dried at 40–50 °C for 2 d. The dried banana peel was then milled and ground into powder.
Total phenolic content (TPC) and detection of catechins
The total phenolic content was determined using a Folin–Ciocalteu reagent according to the methods described by Androes et al. (Citation1963). Briefly, 1 mL of Folin–Ciocalteu reagent was mixed with 200 μL sample and 800 μL 7.5% sodium carbonate. The mixture was then incubated for 30 min at room temperature. Several standard gallic acid (GA) solutions containing 0.01–0.1 mg/mL of GA, were made to determine the phenolic content of each sample. Total phenolic content was expressed in GA equivalents (milligram of the GA equivalent per 100 gram fresh fruit weight, mg GAE/100 g FW). All measurements were made in triplicate. The gallocatechin, epigallocatechin, and epigallocatechin gallate content of BPP was determined via high-performance liquid chromatography (HPLC) with the column (C18-AR, 250 × 4.8 mm, maintained at 40 °C), pump (5110), and column oven (5310) set to the manufacturer’s protocols (HITACHI, Kyoto, Japan). BPP was diluted 10 times with water and the extract was filtered through a 0.22-μm membrane. The conditions of the mobile phase were (A) 0.05% v/v H3PO4 and (B) CH3OH/CH3CN solution (3:2, v/v), flow rate 1.0 ml/min, and UV detection at 280 nm. The gradient programme was as follows: (A) 90% (B) 10% at time 0; (A) 75% (B) 25% at 15 min; (A) 40% (B) 60% at 25 min; (A) 90% (B) 10% at 25.1 min; and (A) 90% (B) 10% at 40 min. The results were calculated via comparison with a standard curve and the final data was expressed as mg/100 g DM.
Ferrous chelating capacity assay
The ferrous chelating capacity assay was conducted as per the methods described by Yuris and Siow (Citation2014). Briefly, 250 μL of sample was added to 25 μL ferrous chloride solution (2 mM), followed by 0.8 mL deionised water. The mixture was kept at room temperature for 10 min before 50 μL of ferrozine was added. The absorbance at 562 nm was measured by spectrophotometer and the result calculated by the following formula:
where, A0 is the absorbance of the control and A1 is the absorbance of the sample.
Determination of ferric ion reducing ability power (FRAP)
FRAP was determined using the methods described by Shi and Dalal (Citation1991) with little modification. Briefly, the sample was mixed with a phosphate buffer (0.2 M, pH 6.6) and potassium ferricyanide (1%) at a ratio of 1:1:1, and the mixture placed in a water bath at 50 °C for 20 min. The same volume of trichloroacetic acid (10%) as potassium ferricyanide was added to the mixture, as well as the same volume of deionised water and 0.1% ferric trichloride. The mixture was left to stand for 30 min, before the absorbance at 700 nm was measured. The higher the absorbance, the better the reducing power.
Determination of trolox equivalent antioxidant capacity (TEAC)
For the TEAC assay, the protocol was according to Re et al. (Citation1999). First, 20 mL of peroxidase solution (44 U/mL), 20 mL of peroxide (5 mM), and 20 mL of ABTS solution (1000 μM) were mixed, and then allowed to react in a dark room for an hour before use. The sample was added to the TEAC solution at a ratio of 1:9 and allowed to react for 10 min. The measured absorbance at 734 was compared to the blank TEAC solution, and the results were expressed as the Trolox antioxidant equivalent per 100 g dry matter (Trolox mM/100 g DM).
1,1-diphenyl-2-picrylhydrazyl (DPPH) scavenging capacity assay
The DPPH free radical scavenging capacity was investigated via the methods described by Blois (Citation1958). The DPPH (1 mM) ethanol solution was mixed with the sample at a ratio of 1:4 then incubated in darkness for 30 min, before the absorbance at 517 nm was measured. The result was calculated by the following formula:
where, A0 is the absorbance of the control reaction and A1 is the absorbance in the presence of the sample.
Experimental broiler chickens and their housing
A total of 216 one-day-old female Ross 308 broiler chicks were assigned to 4 groups with 3 pen replicates. Each pen (approximately 8 square meters) contained 18 birds with an average initial body weight of 50 g. Vaccination for Marek’s disease, Newcastle disease and infectious bronchitis was provided to chicks immediately after birth. All chicks were raised in a temperature controlled house. The temperature was kept at 33 ± 1 °C for the first 7 d of the experiment, then the temperature was lowered when the birds were 21 d old and maintained at to 26 ± 1 °C. The birds were kept in floor pens (2.5 × 4.0 m) with wire floors and rice bran litter. In the starter phase (1–21 d), two fountain drinkers and one feed tray were installed per pen. In the grower phase (22–35 d), these were replaced with nipple drinkers and a feeder. The experiment was approved by the Animal Care and Use Committee of the National Chung Hsing University (IACUC NO: 108-040).
Feeding schedule and diet composition
There were two phases in the 35 d experimental period, a starter phase (1–21 d) and a grower phase (22–35 d). Broilers had free access to water and feed, as per the requirements of the NRC (Citation1994). About 0.5%, 1% and 2% of BPP were added to the basal diet (corn and soybean meal). The compositions of the starter and finisher basal diets are listed in Table .
Table 1. Ingredients and chemical composition of the experimental diets for broiler chickens.
Sample collection
Two broilers from each pen (6 birds per treatment) were euthanized by electric shock at 35 d, and the blood, guts, and livers were harvested for further analysis. Blood was taken from brachial veins and loaded in a vacuum blood collection tube, then allowed to coagulate at room temperature. After centrifugation at 3000×g for 15 min, the serum was separated and collected, then stored at −20 °C for later analysis of enzyme activity. The ileal and caecal contents were collected and placed in a petri dish to count the intestinal microbes. The liver was preserved in RNAzol reagent at a ratio of 50 mg/ml at −20 °C for mRNA expression analysis.
Determination of intestinal microbe concentration
Operational protocols were according to the methods of Teng et al. (Citation2017). One gram of ileal and caecal contents was serially diluted with 9 ml of sterile PBS. Chromogenic medium agar (CHROMagarTM 129 ECC) and MRS medium (de Man Rogosa and Sharpe agar, Difco 288130, BD, Franklin Lakes, NJ, USA) were used for isolation of coliform and lactic acid bacteria, respectively. The medium powder was dissolved in deionised water and sterilised according to the manual from CHROMagarTM (Chromogenic medium agar) and de Man Rogosa and Sharpe (MRS medium). The medium plates were prepared before euthanasia. 200 μl of diluted gut contents were added to the corresponding medium plate. There were three replicates in each dilution group. The chromogenic medium plate and TSC agar medium plate were placed in a 37 °C environment for 48 h, and the MRS medium plate was incubated in an anaerobic environment for 48 h. The results are expressed as log CFU/g.
Serum antioxidant enzyme activity
Serum enzyme activity assays for SOD and GPx were processed according to the protocols from Cayman Chemical Co. (Ann Arbor, MI, USA). Briefly, SOD activity was measured by adding 200 μl of the radical detector and 10 μl of serum to a 96-well plate. To initiate the reaction, 20 μl of xanthine oxidase was added and the plate was shaken for a few seconds to allow mixing. The plate was incubated on a shaker for 30 min before the absorbance was read at 450 nm by spectrophotometer. The amount of enzyme exhibiting 50% dismutation of the superoxide radical was defined as one unit of SOD activity. GPx activity was triggered by the addition of 100 μl of buffer, 50 μl of co-substrate mixture, and 20 μl of serum to a 96-well plate. Reactions were initiated by the addition of 20 μl of cumene hydroperoxide and the plate shaken to mix the solutions well. Absorbance was read at 340 nm by plate reader. One unit was defined as the amount of enzyme that causes the oxidation of 1.0 nmol of NADPH to NADP + per min at 25 °C.
Serum malondialdehyde (MDA) concentration
The serum MDA level was determined as per the protocols from the Cayman Chemical Co. (Ann Arbor, MI, USA). 100 μl of serum was mixed with 100 μl of SDS solution and then 4 ml colour reagent was added. To start the reaction, the mixture was boiled at 100 °C for 1 h then immediately placed in an ice bath to cool for 10 min. The mixture was centrifuged for 10 min at 1600×g at 4 °C, before the clean supernatant was extracted to detect its absorbance at 530 nm. The result was calculated using the MDA standard curve and presented as μM/ml serum.
mRNA expression of liver
The total mRNA of the broiler liver was isolated using RNAzol reagent (Molecular Research Centre, Inc., USA.), according to the manufacture’s protocols. Total mRNA was reverse-transcribed into cDNA and the expression levels were analysed by qPCR, following the methods of Lin et al. (Citation2014). All determined genes were normalised using β–actin as the internal control and calculated via the 2−ΔΔCt method. The primers for qPCR are shown in Table .
Table 2. Characteristics and performance data of the primers used for broiler chickens liver q-PCR analysis.
Statistical analysis
Collected data on the variables were statistically analysed using the general liner models procedure of SAS software following a random arrangement. The mathematical model was as follows:
where, Yij = observed response of a bird in a pen; µ = overall mean; Ti = fixed effect of BPP supplementation; and εij = residual error when a pen was regarded as an experimental unit,
Determination of significant statistical differences among the mean values of the 4 BPP groups was analysed by Duncan’s multiple range test, and p < .05 is consider to be significant difference.
Results
Total phenolic and catechin content
The chemical composition and bioactive compounds of BPP are shown in Table . Crude protein, NDF, ADF, and ash levels were 11.2, 36.4, 40.8, and 0.73 g/100 g DM, respectively. The total phenolic content of BPP was 872.7 mg of GAE/100 g DM. Gallocatechin, epigallocatechin, and epigallocatechin gallate content of BPP were 91.9, 65.9 and 10.3 mg/100 g DM, respectively.
Table 3. The chemical composition and bioactive compounds of banana peel powderTable Footnotea.
In vitro antioxidant capacity
The results of the assay for ferrous chelating capacity are shown in Figure , BPP had 64% Fe2+ chelating power compared to EDTA at a concentration of 80 mg/ml, and had slightly increasing ferrous chelating capacity at a concentration of 100 mg/ml. The FRAP results are presented in Figure . The absorbance of BPP at concentrations of 5, 10, 20, 40, 60, 80, and 100 mg/ml were 0.150, 0.336, 0.655, 0.687, 0.745, 0.765, and 0.810, respectively. BPP had 32.4% FRAP when compared to BHT at a concentration of 100 mg/ml. Figure shows that BPP at concentrations of 10, 20, 30, 40, 60, 80, and 100 mg/ml had 40.5%, 45.6%, 40.3%, 42%, 45.7%, 48%, and 50.1% DPPH free radical scavenging capacity, respectively. BPP exerted limited changes in DPPH free radical scavenging capacity above concentrations of 10 mg/ml. Compared to BHT, BPP exerted 50.1% DPPH free radical scavenging capacity at a concentration of 100 mg/ml. Figure illustrates the TEAC value of BPP. At a concentration of 20 mg/ml, BPP reached the same TEAC value (1270 µM trolox eq) as BHT.
Figure 1. The results of (A) ferrous chelating capacity, (B) ferric ion reducing ability power, (C) DPPH free radical scavenge capacity, and (D) TEAC of banana peel (BPP). Results are 3 replicates and expressed as mean ± SD. DPPH: 2,2-Diphenyl-1-picrylhydrazyl; TEAC: Trolox equivalent antioxidant capacity.
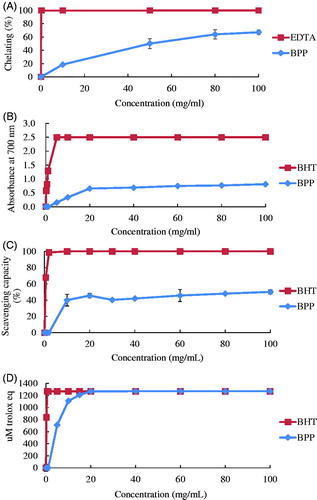
Growth performances
The effects of BPP dietary supplementation on the growth performance of broiler chickens are listed in Table . Body weight, feed consumption, weight gain, and FCR were not significantly different among the tested groups during phases 1–21 d, 22–35 d, or 1–35 d.
Table 4. The effect of banana peel powder supplemented in diets on growth performance of 1–35 d broiler chickens.
Effect on biochemical parameters in broiler chickens
Table shows the effect of BPP supplementation on the biological parameters of 35-day-old broiler chickens. These included serum uric acid, creatinine, serum glutamic-oxalocetic transaminase (SGOT), serum glutamic-pyruvic transaminase (SGPT), total protein, albumin, globular protein, total cholesterol, and total triglycerides. The concentrations of serum uric acid in the groups that received 0.5% and 1% BPP were significantly higher than that of the control group (p < .05).
Table 5. The effect of banana peel powder supplemented in diets on biological parameters of 35d broiler chickensTable Footnotea.
Intestinal microbiota concentration in broiler chickens
Table shows the effect of BPP supplementation on the intestinal microbiota of 35-day-old broiler chickens. Coliform and lactic acid bacteria counts were not significantly different among the groups.
Table 6. The effect of banana peel powder supplemented in diets on intestinal microbiota concentration of 35 d broiler chickensCitationa.
Serum MDA concentration, SOD activity and GPx activity
Table shows the effect of BPP supplementation on serum MDA concentration, SOD activity and GPx activity in 35-day-old broiler chickens. MDA concentration decreased in the 0.5% group (p > .05) but increased significantly in the 2% BPP group (p < .05). SOD activity increased significantly in the 0.5% BPP group (p < .05), but no difference was found between the 1% BPP and 2% BPP groups and the control group. GPx activity was not significantly different among the groups.
Table 7. The effect of banana peel powder supplemented in diets on serum MDA concentration, SOD activity and GPx activity of 35d broiler chickensCitationa.
Liver antioxidant mRNA expression in broiler chickens
Figure illustrates the effect of BPP on liver antioxidant-related mRNA expression in 35-day-old broiler chickens. Chickens that received 0.5% and 1% BPP supplementation had significantly increased mRNA expression of Nrf2, Keap1, SOD, GPx, CAT, and HO-1, compared to the control group (p < .05). Moreover, the 2% BPP group had significantly higher HO-1 mRNA expression than the control group.
Figure 2. The effect of banana peel powder (BPP) on liver (A) Nrf2, (B) Keap1, (C) SOD, (D) GPx, (E) CAT, and (F) HO-1 mRNA expression in 35d broiler chickens. Results are mean ± SD, and 6 birds per group. a,b,cMeans within the same rows without the same superscript letter are significantly different (p < .05).
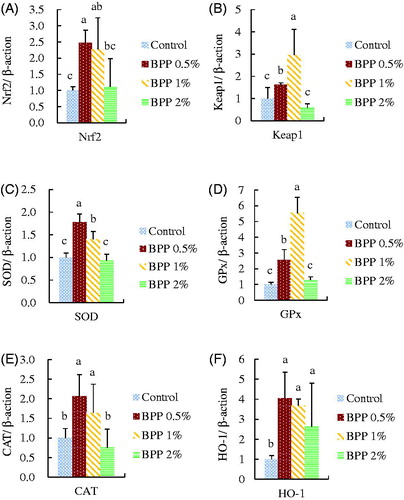
Discussion
Oxidative stress is a serious problem in the poultry industry but using plant material could alleviate ROS damage via several mechanisms (Karre et al. Citation2013). In order to efficiently utilise a banana processing byproduct, we used BPP as a feed additive in broiler chickens to enhance their antioxidant capacity. Banana peel makes up about 35–40% of the entire fruit’s weight and is rich in phenolic compounds, which can range from 4.95 to 47 mg gallic acid equivalent per DM, depending on the cultivar. It is estimated that the total phenolic content of banana peel is three times higher than its fruit (Pereira and Maraschin Citation2015). According to Rebello et al. (Citation2014), dried banana peel contains high levels of flavan-3-ols, mainly in the form of tannin or proanthocyanidin polymers, while dimers and monomers are mainly represented by procyanidin B2 and gallocatechin, which is similar to our own results, as shown in Table . In fresh banana peel, more bioactive compounds are detected that belong to four groups: hydroxycinnamic acids, flavonols, flavan-3-ols and catecholamines (Vu et al. Citation2018). Banana peel showed higher FRAP and DPPH free radical scavenging capacity than pineapple, papaya, passion fruit, watermelon, or melon peel (Morais et al. Citation2015). The ABTS assay by Rebello et al. (Citation2014) showed banana peel from Cavendish had 242.2 mg Trolox equivalent (TE)/g DM, indicating potent free radical scavenging capacity. The FRAP of banana peel is in a dose dependent matter, as shown by the report of Aboul-Enein et al. (Citation2016), which had similar results to our own (Figure ).
Previous studies have reported that green banana peel contains a large amount of tannic acid which could exert negative effects on animal nutrient absorption; this effect decreases with banana maturation (Babatunde et al. Citation1992). The banana peel used in our study was mature and yellow, therefore BPP supplementation would not have a significantly negative effect on growth performance, as shown in Table . Studies revealed that uric acid levels could decrease after the consumption of flavonoid-rich food due to xanthine oxidase inhibition (Zhao et al. Citation2014). Ghazalah and Ali (Citation2008) fed broilers a corn-based diet supplemented with 0.5%, 1%, and 2% rosemary leaves and found that serum uric acid concentration was significantly decreased in the 0.5% and 2% groups. Grape pomace also causes a similar decrease in serum uric acid levels (Lichovnikova et al. Citation2015). However, some human studies reported that plasma urate levels were elevated after the consumption of flavonoid-rich foods like raisins or apple juice, while the fructose content in fruit causes the rapid consumption of ATP in the liver. The AMP produced was degraded into uric acid (Kanellos et al. Citation2013), a contributor to serum antioxidant capacity (Lotito and Frei Citation2004). The fructose content in ripe banana peel is high and ranges from 12.6–26.2% of DM (Emaga et al. Citation2007). Therefore, given the above description, the significant elevation in serum uric acid in the 0.5% and 1% BPP groups might be due to the high fructose content of BPP (Table ).
Maintaining the balance in intestinal microflora is critical to broiler health. Green banana peel contains high amounts of resistant starch, a prebiotic that cannot be digested by the upper intestine but can be fermented by microbes in the colon, releasing short chain fatty acids (SCFA) (Fuentes-Zaragoza et al. Citation2011). SCFA have been proven to lower lumen pH value and exhibit protective effects, including maintenance of IEC integrity and immune homeostasis, and suppression of inflammation (van der Beek et al. Citation2017). When they ripen, bananas not only change colour from green to yellow but the resistant starch is degraded into sugar (Ramli et al. Citation2009), decreasing the releasing potential of SCFA. Mokbel and Hashinaga (Citation2005) demonstrated that the EtOAc extract of green banana peel inhibited the Gram positive bacteria Staphylococcus aureus, Bacillus subtilis, and Bacillus cereus, and the Gram negative bacteria Salmonella enteritidis and Escherichia coli. Conversely, yellow banana peel had only minor antimicrobial effects, indicating that antimicrobial compounds degrade during ripening. As such, in the current study there was no difference in the coliform and lactic acid bacteria counts in the ileum and caecum between the BPP-supplemented groups and the control, as shown in Table .
SOD and GPx is the first line of enzymatic antioxidant Defence in organisms, with the ability to directly convert free radicals into water and oxygen, maintaining the intracellular redox balance. MDA is an end product of lipid peroxidation and its concentration could indicate the oxidative status of the cell since cell membranes are made of lipids. Our previous research indicated that using plant-based feed additives increases serum CAT activity but not SOD activity in laying hens (Lin et al. Citation2017). Broilers fed a diet supplemented with soy bean isoflavones had increased plasma CAT and SOD activity while plasma MDA concentration was decreased (Jiang et al. Citation2007). In the current study, the 0.5% BPP group had significantly increased serum SOD activity, and had equal MDA concentration level compared to the control as shown in Table . This may indicate that serum SOD activity could be enhanced by BPP supplementation and the scavenging of free radicals increased, leading to lower serum MDA levels. The group supplemented with 2% BPP had increased serum MDA levels, which is in line with our previous report (Lin et al. Citation2017) when the diet of laying hens was supplemented with 2% mulberry leaves.
Since the discovery that phytochemicals could modulate Nrf2-associated genes, studies have focussed on the antioxidant ability of phytochemicals at the molecular level (Lee et al. Citation2017). The key step in the antioxidant modulation pathway is the activation of the Nrf2 gene which is responsible for the expression of downstream antioxidant and detoxification genes (Ma, Citation2013). Among the target genes, HO-1 is indispensable in dealing with oxidative stress, the inflammatory response, and detoxification, which prevents the cell from undergoing apoptosis (Gozzelino et al. Citation2010). The cytoprotective mechanisms of HO-1 include releasing the antioxidants CO, iron, and biliverdin from haem, and it might also induce the cell survival pathways PI3K/AKT and P38 (Hori et al. Citation2002). Chow et al. (Citation2005) reported that HO-1 can be induced to protect H9c2 cells from H2O2 damage by treatment with quercetin. In the current study, supplementation with 0.5% and 1% BPP seemed to result in a better activation of target antioxidant genes than 2% BPP supplementation, as shown in Figure , indicating that BPP increased the antioxidant capacity of broiler chickens.
Conclusions
Based on the above results, BPP exerted a potent an antioxidant effect. Dietary supplementation with 0.5% BPP enhanced the antioxidant status of broilers by increasing serum SOD activity and stimulating the expression of ARE-associated genes, suggesting that BPP supplemented at low levels has potential as an antioxidant feed additive.
Acknowledgment
The authors would like to express the sincere gratitude to the National Chung Hsing University (NCHU-CSMU 10804) and the Ministry of Health and Welfare, Taichung Hospital for financially supporting the study (MHW10721), and Ministry of Science and Technology (MOST 107-2313-B-005-037-MY2) and The iEGG and Animal Biotechnology Center from The Feature Areas Research Center Program within the framework of the Higher Education Sprout Project by the Ministry of Education (MOE) in Taiwan for supporting this study.
Disclosure statement
We certify that there is no conflict of interest with any financial organization regarding the material discussed in the manuscript.
References
- Aboul-Enein AM, Salama ZA, Gaafar AA, Aly HF, Faten A, Ahmed H. 2016. Identification of phenolic compounds from banana peel (Musa paradaisica L.) as antioxidant and antimicrobial agents. J Chem Pharm Res. 8:46–55.
- Androes GM, Singleton MF, Biggins J, Calvin M. 1963. Photo-induced electron paramagnetic resonance in mutant photosynthetic species lacking carotenoids or chlorophyll. Biochim Biophys Acta. 66:180–187.
- Babatunde G, Machin D, Nyvold S, editors. 1992. Proceedings of the FAO Expert Consultation held in CIAT, Cali, Colombia; Jan 21–25; Roma, Italy.
- Blois MS. 1958. Antioxidant Determinations by the use of a stable free radical. Nature. 181:1199–1200.
- Chow JM, Shen SC, Huan SK, Lin HY, Chen YC. 2005. Quercetin, but not rutin and quercitrin, prevention of H2O2-induced apoptosis via anti-oxidant activity and heme oxygenase 1 gene expression in macrophages. Biochem Pharmacol. 69:1839–1851.
- Emaga TH, Andrianaivo RH, Wathelet B, Tchango JT, Paquot M. 2007. Effects of the stage of maturation and varieties on the chemical composition of banana and plantain peels. Food Chem. 103:590–600.
- Estevez I. 2007. Density allowances for broilers: where to set the limits? Poult Sci. 86:1265–1272.
- Fuentes-Zaragoza E, Sánchez-Zapata E, Sendra E, Sayas E, Navarro C, Fernández-López J, Pérez-Alvarez JA. 2011. Resistant starch as prebiotic: a review. Starch. 63:406–415.
- Ghazalah A, Ali A. 2008. Rosemary leaves as a dietary supplement for growth in broiler chickens. Int J Poult Sci. 7:234–239.
- Gozzelino R, Jeney V, Soares MP. 2010. Mechanisms of cell protection by heme oxygenase-1. Annu Rev Pharmacol Toxicol. 50:323–354.
- Heckert R, Estevez I, Russek-Cohen E, Pettit-Riley R. 2002. Effects of density and perch availability on the immune status of broilers. Poult Sci. 81:451–457.
- Hori R, Kashiba M, Toma T, Yachie A, Goda N, Makino N, Soejima A, Nagasawa T, Nakabayashi K, Suematsu M. 2002. Gene transfection of H25A mutant heme oxygenase-1 protects cells against hydroperoxide-induced cytotoxicity. J Biol Chem. 277:10712–10718.
- Huang CM, Lee TT. 2018. Immunomodulatory effects of phytogenics in chickens and pigs - A review. Asian-Australas J Anim Sci. 31:617–627.
- Huang C, Jiao H, Song Z, Zhao J, Wang X, Lin H. 2015. Heat stress impairs mitochondria functions and induces oxidative injury in broiler chickens. J Anim Sci. 93:2144–2153.
- Jiang Z, Jiang S, Lin Y, Xi P, Yu D, Wu T. 2007. Effects of soybean isoflavone on growth performance, meat quality, and antioxidation in male broilers. Poult Sci. 86:1356–1362.
- Kanellos P, Kaliora A, Gioxari A, Christopoulou G, Kalogeropoulos N, Karathanos V. 2013. Absorption and bioavailability of antioxidant phytochemicals and increase of serum oxidation resistance in healthy subjects following supplementation with raisins. Plant Foods Hum Nutr. 68:411–415.
- Karre L, Lopez K, Getty KJ. 2013. Natural antioxidants in meat and poultry products. Meat Sci. 94:220–227.
- Lara L, Rostagno M. 2013. Impact of heat stress on poultry production. Animals (Basel). 3:356–369.
- Lee MT, Lin WC, Lee TT. 2019. Potential crosstalk of oxidative stress and immune response in poultry through phytochemicals – A review. Asian-Australas J Anim Sci. 32:309–319.
- Lee MT, Lin WC, Yu B, Lee TT. 2017. Antioxidant capacity of phytochemicals and their potential effects on oxidative status in animals – A review. Asian-Australas J Anim Sci. 30:299–308.
- Lichovnikova M, Kalhotka L, Adam V, Klejdus B, Anderle V. 2015. The effects of red grape pomace inclusion in grower diet on amino acid digestibility, intestinal microflora, and sera and liver antioxidant activity in broilers. Turk J Vet Anim Sci. 39:406–412.
- Lin CC, Lin LJ, Wang SD, Chiang CJ, Chao YP, Lin J, Kao ST. 2014. The effect of serine protease inhibitors on airway inflammation in a chronic allergen-induced asthma mouse model. Mediators Inflamm. 2014:1.
- Lin WC, Lee MT, Chang SC, Chang YL, Shih CH, Yu B, Lee TT. 2017. Effects of mulberry leaves on production performance and the potential modulation of antioxidative status in laying hens. Poult Sci. 96:1191–1203.
- Lotito SB, Frei B. 2004. The increase in human plasma antioxidant capacity after apple consumption is due to the metabolic effect of fructose on urate, not apple-derived antioxidant flavonoids. Free Radic Biol Med. 37:251–258.
- Ma Q. 2013. Role of Nrf2 in oxidative stress and toxicity. Annu Rev Pharmacol Toxicol. 53:401–426.
- Mokbel MS, Hashinaga F. 2005. Antibacterial and antioxidant activities of banana (Musa, AAA cv. Cavendish) fruits peel. Am J Biochem Biotechnol. 1:125–131.
- Morais DR, Rotta EM, Sargi SC, Schmidt EM, Bonafe EG, Eberlin MN, Sawaya A, Visentainer JV. 2015. Antioxidant activity, phenolics and UPLC–ESI(–)–MS of extracts from different tropical fruits parts and processed peels. Food Res Int. 77:392–399.
- National Research Council. 1994. Nutrient requirements of poultry. 9th rev. ed. Washington D. C: National Academy Press.
- Pereira A, Maraschin M. 2015. Banana (Musa spp) from peel to pulp: ethnopharmacology, source of bioactive compounds and its relevance for human health. J Ethnopharmacol. 160:149–163.
- Ramli S, Alkarkhi AF, Shin Yong Y, Min-Tze L, Easa AM. 2009. Effect of banana pulp and peel flour on physicochemical properties and in vitro starch digestibility of yellow alkaline noodles. Int J Food Sci Nutr. 60:326–340.
- Re R, Pellegrini N, Proteggente A, Pannala A, Yang M, Rice-Evans C. 1999. Antioxidant activity applying an improved ABTS radical cation decolorization assay. Free Radic Biol Med. 26:1231–1237.
- Rebello LPG, Ramos AM, Pertuzatti PB, Barcia MT, Castillo-Muñoz N, Hermosín-Gutiérrez I. 2014. Flour of banana (Musa AAA) peel as a source of antioxidant phenolic compounds. Food Res Int. 55:397–403.
- Shi X, Dalal N. 1991. Flavoenzymes reduce vanadium(V) and molecular oxygen and generate hydroxyl radical. Arch Biochem Biophys. 289:355–361.
- Someya S, Yoshiki Y, Okubo K. 2002. Antioxidant compounds from bananas (Musa Cavendish). Food Chem. 79:351–354.
- Surai PF. 2016. Antioxidant systems in poultry biology: superoxide dismutase. J Anim Res Nutr. 1:8.
- Teng PY, Chang CL, Huang CM, Chang SC, Lee TT. 2017. Effects of solid-state fermented wheat bran by Bacillus amyloliquefaciens and Saccharomyces cerevisiae on growth performance and intestinal microbiota in broiler chickens. Ital J Anim Sci. 16:552–562.
- van der Beek CM, Dejong CH, Troost FJ, Masclee AA, Lenaerts K. 2017. Role of short-chain fatty acids in colonic inflammation, carcinogenesis, and mucosal protection and healing. Nutr Rev. 75:286–305.
- Vu HT, Scarlett CJ, Vuong QV. 2018. Phenolic compounds within banana peel and their potential uses: a review. J Func Food. 40:238–248.
- Yuris A, Siow LF. 2014. A comparative study of the antioxidant properties of three pineapple (Ananas comosus L.) varieties. J Food Stud. 3:40–56.
- Zhang H, Tsao R. 2016. Dietary polyphenols, oxidative stress and antioxidant and anti-inflammatory effects. Curr Opin Food Sci. 8:33–42.
- Zhao M, Zhu D, Sun-Waterhouse D, Su G, Lin L, Wang X, Dong Y. 2014. In vitro and in vivo studies on adlay-derived seed extracts: phenolic profiles, antioxidant activities, serum uric acid suppression, and xanthine oxidase inhibitory effects. J Agric Food Chem. 62:7771–7778.