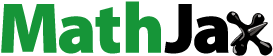
Abstract
Yeast cell wall are a source of prebiotics in animal nutrition, able to improve microbial function in the large intestine. The purpose of this paper was to compare the fermentability of six Saccharomyces cerevisiae (SC) cell wall obtained from three production processes (alcoholic, brewers and bakers). For each production process the two aliquots characterised by the highest the lowest carbohydrate levels were selected. In order to perform the in vitro trial, each SC substrate was incubated at 39 °C under anaerobic condition for 72 h, using cat faecal inoculum. The results showed that the production processes did not affect organic matter disappearance, while significantly (p < .01) influenced cumulative volume of gas which appeared the lowest for alcoholic substrates. The alcoholic SC substrate showed significantly highest (p < .01) fermentation rate. All SCFA were affected by the production processes (p < .01). In particular, brewers substrates showed the highest acetate and butyrate production, while the fermentation of SC cell wall obtained by bakers showed the highest production of valerate, iso-butyrate and iso-valerate. Considering the high fermentability and acetate and butyrate production, the SC cell wall obtained by brewers seem more suitable to be used as prebiotic in cat diet. The bakers extract seems less advisable, due to its less fermentability at which corresponded the highest production of gas and iso-butyrate and iso-valerate, which are considered the less desirable end-products. The study underlines that it is necessary to evaluate the fermentation characteristics of these ingredients before their use in diet formulation as source of prebiotics.
The production process affects the in vitro fermentation parameters differently from the carbohydrate’s concentration.
The production processes significantly influenced cumulative volume of gas and all short chain fatty acids production.
The SC cell walls obtained by brewers seem more suitable to be used as prebiotic in cat diet and the bakers extract seems the less advisable.
Highlights
Introduction
Yeasts and their cell extracts have been recognised as safe supplements for human and animal diets by the US Food and Drug Administration (Rodriguez et al. Citation2015) and EU Reg 2017/1017. These products contain high concentration of mannan-oligosaccharides (MOS, about 14% on dry matter basis), β-glucans (about 24% on dry matter basis), lipids, chitin, vitamins (White et al. Citation2002; Jaehrig et al. Citation2008) and proteins which are often linked to the mannans and referred as the mannan-protein complex (Lipke and Ovalle, Citation1998). β-glucans and MOS are considered beneficial for gut microbiota, may be due to their fermentability in the large intestine or, as reported by Ringø et al. (Citation2016), by their ability to limit the colonisation of pathogen bacteria. Several in vivo and in vitro studies have highlighted the cell wall function as prebiotic in human and animal nutrition (Chen and Seviour, Citation2007; Laroche and Michaud, Citation2007; Hai and Fotedar, Citation2009) by reducing the intestinal pathogen colonisation (Johnson et al. Citation2012; Ringø et al. Citation2016; Fomenky et al. Citation2017), decreasing the immunosuppression caused by stress (Magnani et al. Citation2009; Liu et al. Citation2011; Borchani et al. Citation2016) and supporting the immune system (Newman and Newman Citation2001; O’Quinn et al. Citation2001). Furthermore, β-glucans protect the body from infections (e.g. viral, fungal and bacterial) (Liu et al. Citation2011; Borchani et al. Citation2016).
As pointed out by Shurson (Citation2018), animals have been fed with products containing yeasts from more than 100 years. However, in recent years, due to the need to limit the use of antibiotics, increasing interest has been addressed to the use of yeasts and their products in animal nutrition, in particular in pet-food industry, which produce a wide range of diets and supplements containing yeast, as well as yeast cell walls, both for dogs and cats. Different species of Saccharomyces, in particular S. cerevisiae, are largely adopted as prebiotics in animal nutrition (Desnoyers et al. Citation2009; Mackenthun et al. Citation2013), as well as supplements (Liu et al. Citation2008; Kafilzadeh et al., Citation2019).
Due to their ability to ferment carbohydrates into carbon dioxide and alcohols, Saccharomyces cerevisiae (SC) have been widely used in the food industry for millennia.
Many different processes are developed in order to obtain a better quality of yeast extracts. Yeast extracts may be obtained mechanically after autolysis by acid, alkali hydrolysis or by enzymatic treatment (Freimund et al. Citation2003; Borchani et al. Citation2014). The inclusion of SC cell wall in the diet could have different effects, in function of SC source and yield extraction, which affect the amount of nutrients and their purity (Freimund et al. Citation2003). It is assumed that the production methods can affect the chemical characteristics of yeast cell wall and fermentability in the hindgut and, consequently, their applications in cat nutrition to prevent or treat specific pathologies. The aim of the present study was to use the in vitro gas production technique (IVGPT) with feline faeces as inoculum in order to compare the fermentation characteristics of six yeast cell wall samples obtained by three production processes (alcoholic, brewers and bakers) with two carbohydrates (glucans + mannans) levels (high and low) and to evaluate their possible utilisation in cat-foods’ production. Several authors consider the IVGPT, utilising faecal inocula, useful to evaluate the fermentability of specific ingredients for diets of monogastric species. In literature are present studies conducted with faecal inocula from dogs (Bosch et al. Citation2016), cats (Musco et al. Citation2016), pigs (Musco et al. Citation2015; Mercurio et al. Citation2016; Lee et al. Citation2018) and horses (Murray et al. Citation2014).
Materials and methods
Substrates sampling
At the processing plant the Saccharomyces cerevisiae collected from different food industries: alcoholic (A), brewers (BR) and bakers (BA) were individually hydrolysed and centrifugated in order to separate the cell wall. Then, the obtained pellets were dried with air insufflation (mean temperature 38–40 °C). Three aliquots of daily production were randomly analysed for carbohydrates (mannans + glucans) concentration; samples representative from those with higher and lower carbohydrates concentration of the annual production were chosen and divided in two groups (high and low). Hence, considering the production process and the carbohydrates concentration, the substrates were named as follows: SC-A high, SC-A low, SC-BR high, SC-BR low, SC-BA high and SC-BA low.
Laboratory analyses
Mannans + glucans content was determined on all the aliquots at the laboratory of Biorigin (São Paulo, Brazil) in accordance with Freimund et al. (Citation2005).
The proximate analysis of the substrates was performed (in quadruplicate) at the Feed Evaluation Laboratory of the Department of Veterinary Medicine and Animal Production (Napoli, Italy), according to AOAC (Citation2005) procedures (dry matter ID 934.01, crude protein ID 954.01; ether extract ID 920.39 C; crude fibre ID 978.10; ash ID 942.05).
Each substrate was weighed (0.5033 ± 0.002 g) in quadruplicates into serum flasks with a medium (0.75 mL) containing nitrogen source, fatty acids, vitamins and minerals; a reducing agent solution (4 mL) was added to guarantee the bacterial growth, as well as the anaerobiosis (Calabrò et al. Citation2013). For this last purpose, flasks were continuously blowing with CO2 until they were sealed with butyl rubber stoppers and aluminium crimps. To prepare the inoculum, faecal samples were collected from four healthy adult European cats (mean age: 3.41 ± 0.7 years; mean live weight 3.53 ± 0.500 kg). The cats were progressively adapted (over 10 days) to a commercial standard diet (% as fed: crude protein 36, crude fibre 2.0, ether extract 20) administered daily according to their energy requirements using the equation: 100 kcal/kg0.67 (NRC Citation2006). After collection the faecal samples were immediately transported to the laboratory and processed within 40 min. To reduce the variability (Bosch et al. Citation2017a, Citation2017b), the individual faecal samples were pooled, and successively diluted (1:10 v:v) with 0.9% NaCl sterile solution, homogenised, filtered through six layers of gauze and added (5 mL) to the pre-warmed serum flasks. The flasks were placed in an incubator (PI400 Carbolite, Sheffield, UK) at 39 °C for 72 h (Bosch et al. Citation2017a). In addition, four flasks were incubated without substrate (negative control).
The fermenting gas production was recorded 16 times, at 3–4 h intervals, using a manual pressure transducer (Cole and Parmer Instrument, Vernon Hills, IL, USA). The cumulative volume of gas (OMCV, mL/g) obtained for each sample at 72 h was related to the quantity of incubated organic matter. The fermentation was stopped by cooling the flasks to 4 °C, and the fermenting liquor pH was measured (pH metre model 3030; Alessandrini Instrument SpA glass electrode Jenway, Dunmow, UK). Flask residues were filtered through pre-weighed sintered glass crucibles (porosity #2, DURAN Group GmbH Mainz, Germany), and residual organic matter was determined by burning the sample at 550 °C for 5 h. Organic matter disappearance (OMD, %) was calculated as the difference between incubated and residual OM.
Two aliquots (5 mL) of fermenting liquor were collected and frozen at −15 °C to determine the fermentation end-products. Short chain fatty acids (SCFA) were measured using gas chromatography technique, whereas ammonia (N-NH3) was determined using spectrophotometry technique (Musco et al. Citation2016).
All procedures involving animals were approved by the Ethical Animal Care and Use Committee of the University of Napoli Federico II (Prot. 2019/0013729 of 08/02/2019).
Data processing
The gas profile of each flask was fitted to the sigmoid model described by Groot et al. (Citation1996) as follows:
where G is the total gas produced (mL/g of incubated OM) at t (h) time; A is the asymptotic gas production (mL/g of incubated OM); B is the time at which half of the asymptote is reached (h); C is the curve switching characteristic. The model parameters were utilised (Bauer et al., Citation2001) to calculate the maximum fermentation rate (Rmax, mL/h) and the time at which it occurs (Tmax, h) according to the following formula:
The chemical composition of the SC cell walls and all the fermentation characteristics obtained (OMCV, OMD, SCFA, pH and N-NH3, Tmax, Rmax) were subjected to analysis of variance using the JMP software, version 14 (SAS Institute, Cary, NC, USA, 2014) to detect the differences between the production processes and carbohydrates concentration according to the following equation:
where y is the experimental data; μ is the general mean; PP is the production process (i = alcoholic, brewers, bakers); CARB is the carbohydrates concentration (j = high, low); PP * CARB is the first level of interaction in which the effect of one causal variable on an outcome depends on the state of a second causal variable; ε is the error term.
When significant differences among substrates were found by the analysis of variance, means were compared using the t test employing the JMP software (SAS Institute, Cary, NC, USA, 2014, version 14.0).
Results
Proximate analysis
The chemical composition characteristics of the tested substrates are depicted in Table .
Table 1. Proximate analysis (% a.f.) of the Saccharomyces cerevisiae cell wall obtained from three production processes.
All chemical parameters were affected by the production processes. In particular, bakers extract showed the highest (p < .01) EE and CF compared to the alcoholic and brewer extracts. On the other hand, the alcoholic extract showed the highest (p < .01) crude protein content, and brewers extract the highest ash and glucans + mannans levels, compared to the other samples. Considering the carbohydrates effect, the High SC showed highest (p < .01) EE, while the Low SC showed the high content of CP and CF (p < .01). The interaction was high significant for CP, CF and ash.
In vitro fermentation characteristics
Usually a gas production trial with carnivores inocula is stopped at 48 h of incubation; however, in this study the incubation was prolonged (72 h) until gas production started to decrease clearly. The in vitro fermentation parameters are reported in Table . Neither the production process nor carbohydrates concentration affected the organic matter disappearance, which always resulted higher than 88%. On the contrary, the production processes significantly (p < .01) affected all the other parameters: OMCV appeared lower for the yeast cell wall obtained by alcoholic process than that obtained from brewers and bakers ones, even if the interaction was significant for OMCV (p < .05). Tmax not linearly differs among the production processes, as indicated by the high significance of the interaction (p < .0001). Compared to the other substrates, the fastest fermentation kinetics (p < .01) was observed in SC-A, which reached the highest Rmax values in the shortest time (14 h of incubation), whereas the slowest (p < .01) was observed in SC-BA, which needed more than 19 h to achieve the maximum fermentation rate.
Table 2. In vitro fermentation parameters for Saccharomyces cerevisiae incubated with cat faeces.
The fermentation rate profiles for the three production processes with the two levels of carbohydrates are illustrated in Figure . Considering the carbohydrates levels within the same production process, different trend appear: for A and BR the curves show similar shape between low and high (for both the processes is more rapid and consistent when high carbohydrates content is present), otherwise for BA large differences emerged between low and high carbohydrates level. In particular, the fermentation trend shows a bell-shaped curve when the carbohydrates content is low and a flatter shape when the carbohydrates content is high. The differences between the BA substrates are evidenced also by Rmax value, which was more than double for low (9.13 mL/h) than high carbohydrates ones (4.49 mL/h).
Figure 1. In vitro fermentation kinetics of Saccharomyces cerevisiae cell wall obtained from different extraction processes with different carbohydrates concentration. Saccharomyces cerevisiae obtained from alcoholic extract (SC-A), brewers extract (SC-BR) and bakers extract (SC-BA) with two levels of carbohydrates concentration (high and low).
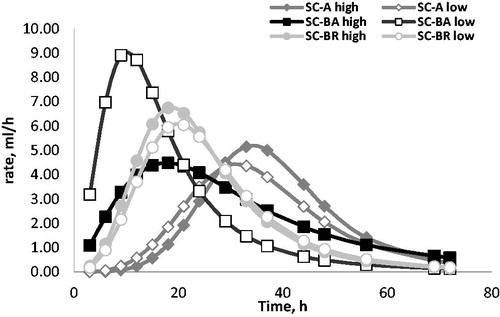
Fermentation end-products
In Table , the concentration of the fermentation end-products registered after 72 h of incubation are reported. The production processes significantly (p < .01) influenced all short chain fatty acids, whereas the carbohydrates levels only few. In particular, cell wall obtained by brewers showed the highest acetate and butyrate production (p < .01), while the fermentation of the cell wall obtained by bakers allowed the highest production of valerate, iso-butyrate and iso-valerate (p < .01). The fermentation of yeast cell wall characterised by high mannans + glucans concentration produced a significantly (p < .01) higher concentration of propionate and iso-valerate and a lower amount of valerate. No statistical differences were detected for pH and N-NH3 production due to both factors. As regards to N-NH3 content, only the interaction between the production system and carbohydrates concentration was statistically significant (p < .01).
Table 3. Fermentation end-products (mmol/g of incubated organic matter) and pH at 72 h of Saccharomyces cerevisiae cell wall.
Discussion
Fermentable carbohydrates are important components of pet diets due to their widely known properties. These polysaccharides remain almost intact in the first part of the intestine until they reach the foregut, where they become a substrate available for the bacteria attack (Musco et al. Citation2017). They are a source of organic matter for the large intestine microbiota and stimulate the microorganism growth throughout the production of short chain fatty acids. Instead, butyric acid represents the most important source of energy for the colonocytes, as described by Vastolo et al. (Citation2019). All yeast cell walls, and in particular that one from Saccharomyces cerevisiae, have been recognised to be a newsworthy source of fermentable carbohydrates. All fungal cell walls consist of polysaccharides; in particular, the chitin and various glucans homopolymers of D-glucose were most frequently found in building blocks used by nature to construct a barrier that separates the fungus from the external environment. In the yeast cell, the α- and β-glucans and the α-mannan are the major polysaccharides utilised in cell wall construction (only chitin represents 1–2%) (Kwiatkowski and Kwiatkowski, Citation2012). In addition some authors (Freimund et al. Citation2005; Pinto et al. Citation2015) reported that the chemical composition of the yeast cell walls, in terms of ether extract, crude protein and crude fibre, is highly affected by the starting materials but also by the production process. The differences in nutrient composition noticeably affect the functional effect when cat diets are supplemented with yeast cell wall (Aquino et al. Citation2013; Santos et al. Citation2018). As a consequence, it is quite difficult to predict the real effects of a new functional product and to compare the results of different studies using supplementations based on yeast cell wall, because their availability and fermentability in the large intestine could differ a lot. Furthermore, only a few studies have been performed using in vitro technique to evaluate the fermentation characteristics of carbohydrate-rich products using cat faces as inoculum (Musco et al. Citation2016; Bosch et al. Citation2017a, Citation2017b).
In this study, some significant differences in chemical composition among the tested substrates were observed, but the trend in fermentation parameters was not always easy explainable by these differences. These last could be due to the yeast strains and/or to the temperature of processing used in the food industries. For example, the significantly highest amount of mannans + glucans in Saccharomyces cerevisiae brewers extracts surely contributed to the high volume of gas produced, whereas the low gas production of the alcoholic extracts could be due to their high protein content. Probably, the significantly highest lipid concentration of bakers extract could explain the lower OMD of these substrates. However, it is difficult to explain the high gas production, even if produced slowly, recorded in these substrates.
The short chain fatty acids are the main end-products of the bacterial fermentation in the mammal’s colon, and they are considered as indicators of nutritional evaluation of ingredients rich in carbohydrates used in pet-food (Cutrignelli et al. Citation2009). The main SCFA (acetate, propionate and butyrate) are rapidly absorbed and then metabolised by the gut epithelium, liver and muscle and have a trophic effect on the intestinal epithelium, maintaining the mucosal defence barrier against pathogens organisms (Mroz, Citation2005).
All the tested substrates ranged SCFA as follows: acetate > propionate > butyrate. This observation, together with the pH values registered at the end of fermentation, suggests that the fermentation falls in the physiological ranges for cats (Younes et al. Citation2001). Notwithstanding, for all the samples the SCFA production and the proportion of each fatty acid were significantly affected by the production process, while the concentration of carbohydrates (high vs. low) significantly affected only the propionate, valerate and iso-valerate production.
It is interesting to compare these results with those obtained in our previous study (Musco et al. Citation2018), incubating the same substrates, in the same experimental conditions, but using dog faecal inoculum. All tested substrates were fermented by both inocula, but the faecal microorganism activities seem different in terms of fermentation pattern and obtained final products. In particular, the cat intestinal microbiota seems more influenced by the variations due to the chemical composition of substrates compared to dog ones, in fact several differences in the proportion of SCFA were observed in cat in function of carbohydrates concentration unlike dog. These differences could be due to the specific microbial populations and/or activities in the two animal species. Our observation is in accordance with that reported by Garcia-Mazcorro et al. (Citation2015), which evaluating in vivo the effect of different prebiotic supplementation in dog and cat microbiota, concluded that the two species respond differently to the same treatment.
Conclusions
From the findings obtained, it seems evident that the Saccharomyces cerevisiae cell wall production process affects most of the in vitro fermentation parameters differently from the carbohydrates (mannans + glucans) concentration. In particular, brewers extracts seem more useful supplement for cat diets. The high butyrate production obtained incubating these substrates suggest a better trophic effect on gut epithelium. Whereas the high gas production and fermentation rate registered suggest the importance of the dosage. In fact, the use of wrong quantity may cause undesirable effects, such as meteorism and abdominal pains. The bakers extracts seem a less advisable substrate because of its lower fermentability at which correspond the highest production of gas and iso-butyrate and iso-valerate, which are considered the less desirable end-products. These last, in fact, derived from the fermentation of branched-chain amino acids (valine, leucine and iso-leucine).
Therefore, it is suggested to test ingredients with functional characteristics, such as yeast, not only for chemical composition (e.g. protein/carbohydrates ratio) but also for their in vitro fermentation patterns, before introducing them in cat diets.
Author contributions
M.I.C. and S.C. contributed to the project administration, conceptualisation and writing-original draft; N.M. and A.V. performed the analyses; F.R. contributed to the collection of samples; M.I.C., M.C., L.E. supported the acquisition and interpretation of data and contributed in writing the article; N.M. editing the paper. All the authors gave final approval to the manuscript and any revised version submitted.
Acknowledgements
The authors acknowledge the financial support of Biorigin (São Paulo, Brazil) and Dr Maria Ferrara for her technical support at the Department of Veterinary Medicine and Animal Production (Napoli, Italy).
Disclosure statement
No potential conflict of interest was reported by the authors.
Additional information
Funding
References
- AOAC. 2005. Official methods of analysis. 18th ed. Arlington (VA): Association of Official Analytical Chemists.
- Aquino AA, Saad F, Santos JPF, Leite CAL, Sampaio GR, Feliciano M. 2013. Efeitos da parede de levedura em dieta úmida na microbiota fecal, na produção de gás e na morfologia intestinal de gatos adultos [Effects of the yeast cell wall in a moist diet in fecal microbial, gas production and intestinal morphology of adult cats]. Arq Bras Med Vet Zootec. 65:1673–1680.
- Bauer E, Williams BA, Voigt C, Mosenthin R, Verstegen M. 2001. Microbial activities of faeces from unweaned and adult pigs, in relation to selected fermentable carbohydrates. Anim Sci. 73:313–322.
- Borchani C, Fonteyn F, Jamin G, Paquot M, Blecker C, Thonart P. 2014. Enzymatic process for the fractionation of baker’s yeast cell wall (Saccharomyces cerevisiae). Food Chem. 163:108–113.
- Borchani C, Fonteyn F, Jamin G, Paquot M, Thonart P, Blecker C. 2016. Physical, functional and structural characterization of the cell wall fractions from baker’s yeast Saccharomyces cerevisiae. Food Chem. 194:1149–1155.
- Bosch G, Heesen L, de Melo Santos K, Cone JW, Pellikaan WF, Hendriks WH. 2017a. Evaluation of an in vitro fibre fermentation method using feline faecal inocula: inter-individual variation. J Nutr Sci. 6:e24.
- Bosch G, Heesen L, de Melo Santos K, Pellikaan WF, Cone JW, Hendriks WH. 2017b. Evaluation of an in vitro fibre fermentation method using feline faecal inocula: repeatability and reproducibility. J Nutr Sci. 6:e25.
- Bosch G, Vervoort JJM, Hendriks WH. 2016. In vitro digestibility and fermentability of selected insects for dog foods. Anim Feed Sci Technol. 221:174–184.
- Calabrò S, Carciofi AC, Musco N, Tudisco R, Gomes MOS, Cutrignelli MI. 2013. Fermentation characteristics of several carbohydrate sources for dog diets using the in vitro gas production technique. Italian Journal of Animal Science. 12:e4–27.
- Chen J, Seviour R. 2007. Medicinal importance of fungal beta-(1->3), (1->6)-glucans. Mycol Res. 111:635–652.
- Cutrignelli MI, Bovera F, Tudisco R, D’Urso S, Marono S, Piccolo G, Calabrò S. 2009. In vitro fermentation characteristics of different carbohydrate sources in two dog breeds (German shepherd and Neapolitan mastiff). J Anim Physiol Anim Nutr. 93:305–312.
- Desnoyers M, Giger-Reverdin S, Bertin G, Duvaux-Ponter C, Sauvant D. 2009. Meta-analysis of the influence of Saccharomyces cerevisiae supplementation on ruminal parameters and milk production of ruminants. J Dairy Sci. 92:1620–1631.
- Fomenky BE, Chiquette J, Bissonnette N, Talbot G, Chouinard PY, Ibeagha-Awemu EM. 2017. Impact of Saccharomyces cerevisiae boulardii CNCMI-1079 and Lactobacillus acidophilus BT1386 on total lactobacilli population in the gastrointestinal tract and colon histomorphology of Holstein dairy calves. Anim Feed Sci Technol. 234:151–161.
- Freimund S, Sauter M, Käppeli O, Dutler H. 2003. A new non-degrading isolation process for 1,3-b-D-glucan of high purity from baker’s yeast Saccharomyces cerevisiae. Carbohydr Polym. 54:159–171.
- Freimund S, Janett S, Arrigoni E, Amadò R. 2005. Optimised quantification method for yeast-derived 1,3-β-D-glucan and α-D-mannan. Eur Food Res Technol. 220:101–105.
- Garcia-Mazcorro JF, Barcenas-Walls JR, Suchodolski JS, Steiner JM. 2015. Molecular assessment of the fecal microbiota in healthy cats and dogs before and during supplementation with fructo-oligosaccharides (FOS) and inulin using high-throughput 454-pyrosequencing. Peer J. 3184:20–26.
- Groot JCJ, Cone JW, Williams BA, Debersaques FMA, Lantinga EA. 1996. Multiphasic analysis of gas production kinetics for in vitro fermentation of ruminant feeds. Anim Feed Sci Technol. 64:77–89.
- Hai NV, Fotedar R. 2009. Comparison of the effects of the prebiotics (Bio-Mos® and β-1,3-D-glucan) and the customised probiotics (Pseudomonas synxantha and P. aeruginosa) on the culture of juvenile western king prawns (Penaeus latisulcatus Kishinouye, 1896). Aquaculture. 289:310–316.
- Jaehrig SC, Rohn S, Kroh LW, Wildenauer FX, Lisdat F, Fleischer LG, Kurz T. 2008. Antioxidative activity of (1-3), (1-6)-b-D-glucan from Saccharomyces cerevisiae grown on different media. Food Sci Technol. 41:868–877.
- Johnson S, Maziade PJ, McFarland LV, Trick W, Donskey C, Currie B, Low DE, Goldstein EJ. 2012. Is primary prevention of Clostridium difficult infection possible with specific probiotics? Int J Infect Dis. 16:e786–792.
- Kafilzadeh F, Payandeh S, Gómez-Cortés P, Ghadimi D, Schiavone A, Martínez Marín AL (2019) Effects of probiotic supplementation on milk production, blood metabolite profile and enzyme activities of ewes during lactation, Italian J Anim Sci. 18:134–139.
- Kwiatkowski S, Kwiatkowski SE. 2012. Yeast (Saccharomyces cerevisiae) glucan polysaccharides – occurrence, separation and application in food, feed and health industries. In: Karunaratne DN, editor. The complex world of polysaccharides. Rijeka (Croatia): InTech; p. 2.
- Laroche C, Michaud P. 2007. New developments and prospective applications for beta (1,3) glucans. Recent Pat Biotechnol. 1:59–73.
- Lee JW, Patterson R, Woyengo TA. 2018. Porcine in vitro degradation and fermentation characteristics of canola co-products without or with fiber-degrading enzymes. Anim Feed Sci Technol. 241:133–140.
- Lipke PN, Ovalle R. 1998. Cell wall architecture in yeast: new structure and new challenges. J Bacteriol. 180:3735–3740.
- Liu HZ, Wang Q, He Y. 2011. Immunoactivities and antineoplastic activities of Saccharomyces cerevisiae mannoprotein. Carbohydr Polym. 83:1690–1695.
- Liu X, Wang Q, Cui S, Liu H. 2008. A new isolation method of b-D-glucans from spent yeast Saccharomyces cerevisiae. Food Hydrocoll. 22:239–247.
- Mackenthun E, Coenen M, Vervuert I. 2013. Effects of Saccharomyces cerevisiae supplementation on apparent total tract digestibility of nutrients and fermentation profile in healthy horses. J Anim Physiol Anim Nutr. 97:115–120.
- Magnani M, Calliari CM, de Macedo FC, Mori MP, de Syllos Cólus IM, Castro-Gomez R. 2009. Optimized methodology for extraction of (1-3) (1-6)- β-D-glucan from Saccharomyces cerevisiae and in vitro evaluation of the cytotoxicity and genotoxicity of the corresponding carboxymethyl derivative. Carbohydr Polym. 78:658–665.
- Mercurio M, Cappelletti P, de Gennaro B, de Gennaro M, Bovera F, Iannaccone F, Grifa C, Langella A, Monetti V, Esposito L. 2016. The effect of digestive activity of pig gastro-intestinal tract on zeoliterich rocks: an in vitro study. Microporous Mesoporous Mater. 225:133–136.
- Mroz Z. 2005. Organic acids as potential alternatives to antibiotic growth promoters for pigs. In: Ball RO, Zijlstra RT, editors. Advance in pork production. Vol. 16. Edmonton (AB): University of Alberta Department of Agricultural, Food and Nutritional Science; p. 169–182.
- Murray JMD, McMullin P, Handel I, Hastie PM. 2014. Comparison of intestinal contents from different regions of the equine gastrointestinal tract as inocula for use in an in vitro gas production technique. Anim Feed Sci Technol. 187:98–103.
- Musco N, Calabrò S, Infascelli F, Tudisco R, Lombardi P, Grossi M, Addi L, Neto BP, Cutrignelli MI. 2015. In vitro fermentation of structural carbohydrate-rich feeds using faecal inoculum from pigs. Italian J Anim Sci. 14:3875–3562.
- Musco N, Calabrò S, Roberti F, Grazioli R, Tudisco R, Lombardi P, Cutrignelli MI. 2018. In vitro evaluation of Saccharomyces cerevisiae cell wall fermentability using a dog model. J Anim Physiol Anim Nutr. 102:24–30.
- Musco N, Cutrignelli MI, Calabrò S, Tudisco R, Infascelli F, Grazioli R, Lo Presti V, Gresta F, Chiofalo B. 2017. Comparison of nutritional and antinutritional traits among different species (Lupinus albus L., Lupinus luteus L., Lupinus angustifolius L.) and varieties of lupin seeds. J Anim Physiol Anim Nutr. 101:1227–1241.
- Musco N, Lombardi P, Calabrò S, Mastellone V, Tudisco R, Grossi M, Addi L, Grazioli R, Cutrignelli MI. 2016. Aloe arborescens supplementation in cat diet: evaluation of effects by in vitro gas production technique. Italian J Anim Sci. 15:407–411.
- National Research Council (NRC). 2006. Nutrient requirements of dogs and cats. Washington (DC): National Academy Press.
- Newman KE, Newman MC. 2001. Evaluation of mannan oligosaccharides on the microflora and immunoglobulin status of sows and piglet performance. J Anim Sci. 79:189.
- O’Quinn PR, Funderburke DW, Tibbetts GW. 2001. Effects of dietary supplementation with mannan oligosaccharides on sow and litter performance in a commercial production system. J Anim Sci. 79:212.
- Pinto M, Coelho E, Nunes A, Brandão T, Coimbra MA. 2015. Valuation of brewers spent yeast polysaccharides: A structural characterization approach. Carbohydr Polym. 116:215–222.
- Ringø E, Zhou Z, Vecino JLG, Wadsworth S, Romero J, Krogdahl Å, Olsen RE, Dimitroglou A, Foey A, Davies S, et al. 2016. Effect of dietary components on the gut microbiota of aquatic animals. A never-ending story? Aquacult Nutr. 22:219–282.
- Rodriguez A, Kildegaard KR, Li M, Borodina I, Nielsen J. 2015. Establishment of a yeast platform strain for production of p-coumaric acid through metabolic engineering of aromatic amino acid biosynthesis. Metab Eng. 31:181–188.
- Santos JPF, Aquino AA, Glória M, Avila-Campos MJ, Oba PM, Santos KM, Vendramini THA, Carciofi AC, Junior AR, Brunetto MA. 2018. Effects of dietary yeast cell wall on faecal bacteria and fermentation products in adult cats. J Anim Physiol Anim Nutr (Berl). 102:1091–1101.
- Shurson GC. 2018. Yeast and yeast derivatives in feed additives and ingredients: sources, characteristics, animal responses, and quantification methods. Anim Feed Sci Technol. 235:60–76.
- Vastolo A, Calabrò S, Liotta L, Musco N, Di Rosa AR, Cutrignelli MI, Chiofalo B. 2019. In vitro fermentation and chemical characteristics of Mediterranean by-products for swine nurition. Animals. 9:556.
- White LA, Newman MC, Cromwell GL, Lindemann MD. 2002. Brewers dried yeast as a source of mannan oligosaccharides for weanling pigs. J Anim Sci. 80:2619–2620.
- Younes H, Coudray C, Bellanger J, Demignè C, Rayssiguier Y, Rèmèsy C. 2001. Effects of two fermentable carbohydrates (inulin and resistant starch) and their combination on calcium and magnesium balance in rats. Br J Nutr. 86:479–485.