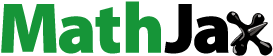
Abstract
Four different palm kernel cake (PKC) including untreated PKC, enzyme fermented PKC (EPKC), extruded PKC (XPKC) and lactic acid bacteria fermented PKC (LPKC) were compared for their passage (ROP), apparent metabolisable energy (AME) and apparent ileal amino acid (AA) digestibility in broilers under the hot and humid tropical climate. The various PKC diets were formulated by replacing 25% of the basal diet with PKC, EPKC, XPKC or LPKC. The AME of various PKC were enumerated by the difference between the AME of basal and PKC diets. The same diets were used to analyse the feed passage time, where the time required excreting the indigestible index (titanium dioxide) was measured. Ileal AA digestibility was determined using PKC, EPKC, XPKC and LPKC as the sole source of AA in the diet. The inclusion of 25% treated PKC in the diet did not improve the ROP of digesta regardless of treatment applied. The AME values of EPKC, XPKC and LPKC, were significantly increased by 39.2, 44.9 and 43.1%, respectively, compared to untreated PKC. In comparison with the untreated PKC, the ileal crude protein (CP) digestibility of LPKC and EPKC were significantly increased by 30.5% and 20.3%, respectively, while no difference was observed for XPKC. The average ileal AA digestibility of LPKC was significantly higher by 22.8% compared to the untreated PKC. In conclusion, bacterial fermentation, enzymatic fermentation and thermal extrusion improved the AME of PKC the hot and humid tropical climate, while only bacterial fermentation resulted in significant improvements in the CP and AA digestibility.
Nutrients availability of PKC can be improved by fermentation.
Passage time of digesta may not change by 25% inclusion of treated PKC.
Highlights
Introduction
Over the past few decades, there has been a dramatic increase in the prices of the conventional feedstuffs accompanied by a shortage in their supply. Hence, there is a necessity for the utilisation of other non-conventional ingredients such as palm kernel cake (PKC) in poultry feed formulation. PKC is being produced abundantly as a by-product of the palm oil industry and has the potential to serve as a feed ingredient for livestock, both ruminants and non-ruminants (Zulkifli et al. Citation2009). However, due to its high crude fibre content and several non-starch polysaccharides (NSP), PKC has limited nutritional value for chickens (Sundu et al. Citation2006). Consequently, it has been reported that PKC has a relatively low apparent metabolisable energy (AME) and digestibility of crude protein (CP) and amino acids (AA) (Alimon Citation2004; Abdollahi et al. Citation2015).
The presence of high NSP and fibre in poultry diet has been reported to increase the viscosity along the intestinal tract and subsequently to alter the feed passage time, which may contribute to the low digestibility of nutrients in NSP containing feed (Choct Citation1997; Alshelmani et al. Citation2016). Sieo et al. (Citation2005) reported that chickens fed with a barley-based diet supplemented with transformed Lactobacillus strains had faster passage rate compared to those provided control diet and this could be attributed to depolymerisation of the complex structure of NSP by the enzymes produced by the transformed bacteria. On the contrary, Rochell et al. (Citation2012) found that feeding a diet containing higher insoluble fibre led to a faster passage time and lower apparent ileal amino acid digestibility (AIAAD). This inconsistency leads to an unclear explanation of the effects of PKC on the passage time in chicken’s gastrointestinal tract.
Various treatments have been proposed to improve the nutritional value of PKC, including solid-state fermentation (SSF), physical treatment and exogenous enzyme supplementation (Marini et al. Citation2005; Saenphoom et al. Citation2011; Alshelmani et al. Citation2014; Roslan et al. Citation2015). SSF of PKC by cellulolytic and hemicellulolytic bacteria has shown reduction of cellulose, hemicellulose, xylans and mannans molecules (Alshelmani et al. Citation2014). Besides, enzymatic hydrolysis of PKC via SSF has been reported to increase the monosaccharides reduction and enhanced the release of prebiotic oligosaccharides (Chen et al. Citation2015). Extrusion has also been proven to enhance the nutritive value of PKC as the reduction of total NSP and crude fibre were accompanied by the increase of mannose, glucose, fructose, 1,4-β-d-mannobiose, 1,4-β-d-mannotriose, 1,4-β-d-mannotetraose and 1,4-β-d-mannopentaose concentration (Roslan et al. Citation2017).
To date, only a limited number of studies on the AIAAD of treated PKC and its AME are available (Muangkeow and Chinajariyawong Citation2013; Saenphoom et al. Citation2013; Hanafiah et al. Citation2017). Moreover, Hanafiah et al. (Citation2017) found that both AME and apparent ileal crude protein digestibility (AICPD) of PKC are highly affected by environmental temperature, as the PKC consumed by heat-stressed chickens had significantly lower AICPD and AME compared to their thermoneutral counterparts. Hence, the contribution of information on the AME, AICPD and AIAAD of treated PKC of broilers under tropical climate, an environment characterised by the hot and humid environmental conditions, would be an informative comparison with the prior findings.
Therefore, this study aims to compare the rate of passage (ROP), AME and AIAAD of the enzyme-fermented PKC, extruded PKC and lactic acid bacteria-fermented PKC in broilers under tropical climate.
Materials and methods
Ethical note
The experimental protocol was approved by the institutional animal care and use committee (IACUC) of the Universiti Putra Malaysia (UPM/IACUC/AUP-R021/2018).
PKCs treatment
PKC was purchased from a commercial kernel oil extraction factory in Klang, Selangor, Malaysia and ground to uniform size of about 3.0 mm before treatment. The PKC was subjected to the following treatments: (1) enzyme treatment (EPKC), (2) extrusion (XPKC); and (3) lactic acid bacteria treatment (LPKC).
The EPKC preparation started with the production of crude enzyme mixture containing endogluconase, mannanase and xylanase, which were produced from solid-state fermentation of PKC using Aspergillus terreus K1 as an inoculum (Chen et al. Citation2013). Briefly, PKC was mixed with distilled water at the pH value of 5.8 to obtain 62.7% moisture content prior to inoculation with 6.0 × 105 A. terreus spore per gram of PKC, following an incubation period of 7 days at 30.5 °C. Upon fermentation, the crude enzyme produced was extracted by shaking the inoculated PKC in distilled water (4 °C) for 24 h. The mixture was centrifuged at 10,000 × g for 10 min and filtered using a filter paper (Whatman No. 1). The extracted crude enzyme was lyophilised and kept at 4 °C for subsequent use.
Following the enzyme production, PKC was mixed with distilled water to achieve a moisture content of about 60% before adding 10 U of previously produced enzymes per gram of PKC for solid-state fermentation. The mixture was incubated at 55 °C for 24 h, dried at 60 °C for 72 h or until achieving constant weight and kept at 4 °C until later use (Chen et al. Citation2015).
The extrusion method was carried out following Roslan et al. (Citation2017), with a few modifications, using a co-rotating twin-screw extruder with a screw diameter of 50 mm and length to diameter ratio of 24. The extruder was equipped with six thermocouples to measure the temperature of the barrel and the temperature inside of the barrel and three heaters to control the heating of the barrel. Two nozzles with a 13 mm diameter hole were fitted at the end of the barrel, for the extrudate to come out. The extrusion device was set up following several conditions; cooking temperature of 80 °C, 90 °C and 100 °C orderly, a screw speed of 150 rpm and hopper speed of 4.5 Hz. The PKC was mixed with water to achieve 50% moisture content prior to extrusion treatment. Once the extrusion is completed, all extrudates were dried at 60 °C for 3 days or until achieving a constant weight.
For LPKC preparation, lactic acid bacteria of strain Weisella confusa SR-17b was used as an inoculum for the PKC fermentation using solid-state fermentation technique following the procedure established by Tan (Citation2016), with few modifications. Briefly, W. confusa SR-17b isolates was activated on the De Man, Rogosa and Sharpe medium (MRS) agar containing (g/L); peptone from casein (10.0), meat extract (8.0), yeast extract (4.0), d(+)glucose (20.0), dipotassium hydrogen phosphate (2.0), Tween 80 (1.0), di-ammonium hydrogen citrate (2.0), sodium acetate (5.0), magnesium sulphate (0.2) and manganese sulphate (0.04). The seed culture broth was prepared by picking five colonies of 16–18 h W. confusa culture into a 100 mL MRS broth. Then, 2 mL of the cell suspension was transferred into another 100 mL MRS broth, and the culture was inoculated statically at 37 °C for 14 h. A minimal salt solution (MSM) with a glucose concentration of 1.0% was prepared as a moisturising agent, and the pH of the solution was adjusted to pH 6.5. Upon completion, inoculum broth was used for the subsequent PKC fermentation where 500 g autoclaved PKC was inoculated with 250 mL of prepared inoculum broth, and MSM solution was supplemented at the ratio of 1:4 (w/v) to the weight of PKC. The mixture was incubated at 37.5 °C for 96 h. As fermentation completed, LPKC was dried at 60 °C for 3 days or until achieving a constant weight and kept at 4 °C for subsequent use.
Birds, housing and management
A total of 300 day-old Cobb 500 male broiler chicks were obtained from a local hatchery. Upon arrival (day 1), chicks were weighed and randomly allocated to 25 battery cages (120 × 120 × 45 cm, length × width × height) with wire floors in a conventional open-sided house with 12 chicks per cage. The experimental temperature ranged from 34 ± 1.0 °C to 21 ± 1.5 °C, and the relative humidity was between 62 ± 1.0% and 88 ± 1.3%. Birds were vaccinated (intraocular route) against Newcastle disease (ND) on days 7 and 21. Birds had ad libitum access to drinking water and feed. The lighting programme consisted of continuous 24 h of light throughout the study.
Experimental diets
The AME of PKC was determined by the difference method (Abdollahi et al. Citation2015; Hanafiah et al. Citation2017) while ileal CP and AA digestibility of PKC were determined by the direct method (Jia et al. Citation2012; Ahmed et al. Citation2014). For the ROP assay, the same experimental diet from the AME assay was used. In the difference method, a corn and soybean meal-based diet was used as the basal diet. Basal starter and finisher diet were formulated to meet or exceed the NRC (Citation1994) requirements as appropriate. From day 1 to 14, birds were fed a standard broiler diet with 21.5% CP and 3035 kcal/kg ME (). Five experimental diets were then developed by including untreated PKC, EPKC, XPKC or LPKC at 25% of the basal diet (). While for the direct method, PKC, EPKC, XPKC or LPKC served as the sole source of CP and AA in the assay diets (). All diets contained titanium dioxide (TiO2) at 0.50%, as an indigestible index. All diets were randomised in a complete randomised design with five replicates per treatment.
Table 1. Ingredient and nutrient compositions of starter (day 1–14).
Table 2. Ingredient and nutrient compositions of experimental diets used in the rate of passage (ROP) and apparent metabolisable energy (AME) assays.
Table 3. Ingredient and nutrient compositions of experimental diets used in ileal crude protein and amino acids digestibility assays.
Experimental procedure
The first 4 days (day 15 to 18) of feeding the experimental diets were considered as an adaptation period, followed by an assay to determine the ROP on day 19 (Rochell et al. Citation2012). Each PKC diet treatment group consisted of 5 replicates of 12 chicks. The assay was initiated with a 2 h fasting, followed by 2 h feeding of experimental diets with TiO2 before replaced with experimental diets without TiO2 to allow ad libitum feed intake for the remainder of the ROP assay. Consumption of diets containing TiO2 was used to determine the total TiO2 intake. Upon the 2 h of feeding index containing diet, total excreta collections were conducted at an interval of 1 h for 12 consecutive hours, where all excreta from each cage were collected, weighed and frozen until later analysis.
On day 20, each treatment group for the ROP assay was divided into two subgroups with six birds per cage arrangement. One group from all treatments has proceeded with AME assay, where the same experimental diets (from ROP assay) with TiO2 were fed from day 20 until day 23. For 4 consecutive days, on a daily basis, feed intake was monitored, and the excreta were collected, weighed and pooled within a cage. The pooled excreta were mixed thoroughly, and representative samples were obtained for freeze-drying. Then, the dried excreta samples were ground and stored in airtight plastic containers at −20 °C for further laboratory analyses. Both dry matter and gross energy of the excreta samples were analysed for AME determination.
On the other hand, on day 20, the remaining group (except basal diet) was offered the assay diets of the direct method according to their previous PKC group during ROP assay. The birds were allowed to consume the feed for 4 days (day 20 until day 23) for an adaptation period. After the adaptation period ended, the birds were fasted for 24 h to remove the gastrointestinal content and induce appetite on the assay diet feeding. On day 25, the birds were allowed for ad libitum feeding of the assay diets for 1.5 h. After 4 h of feeding, all birds were euthanised by injecting their wing veins with sodium pentobarbitone. The ileal contents were collected by gentle flushing with distilled water and pooled within each cage. The samples were freeze-dried and stored in airtight containers at −20 °C. The dry matter (DM), CP, AA and TiO2 were analysed for ileal AA and CP digestibility determination.
All PKC and diets samples were analysed for their DM, CP, crude fibre (CF), ether extract (EE), organic matter (OM), ash, gross energy (GE), neutral detergent fibre (NDF), acid detergent fibre (ADF), acid detergent lignin (ADL), hemicellulose, cellulose, AA concentration and titanium concentration.
Laboratory analyses
Proximate analysis for DM, nitrogen content (N), CF, EE, OM and ash were determined following the procedure of (AOAC Citation1990). The CP was calculated as N × 6.25. The GE was analysed using a bomb calorimeter (IKA2000©, GmbH & Co.KG, Satufen, Germany). The NDF, ADF and ADL were determined according to the methods described by Goering and Van Soest (Citation1970), while hemicellulose and cellulose were calculated as NDF – ADF and ADF – ADL, respectively. Amino acids were determined using high performance liquid chromatography (HPLC) (Law et al. Citation2018). Briefly, samples were hydrolysed by 6 N HCl at 110 °C for 24 h and followed by the addition of an internal standard (l-α-amino-N-butyric acid; AABA) to the hydrolysate. Then, samples were paper and syringe filtered, mixed with borate buffer and ACCQ reagent (Waters Corporation, Milford, MA, USA) prior to the peak’s separation by an AA column (AccQ Tag3.9 150 mm; Waters). The latter was detected by a fluorescent detector (2475; Waters) using HPLC. Cysteine and methionine were analysed as cystic acid and methionine sulphone, respectively by oxidation with performic acid for 16 h at 4 °C and neutralisation with hydrobromic acid before hydrolysis. For titanium determination, the samples were ashed at 580 °C for 13 h to burn all organic material and the remaining minerals were digested (using 7.4 M sulphuric acid) to release titanium dioxide content, which was determined using a spectrophotometer (SPECORD PLUS©, Analytic Jena AG, Jena, Germany) at a wavelength of 410 nm (Short et al. Citation1996).
Calculations
The total titanium excreted by the birds was considered as a unity. The titanium excreted at each hourly collection was expressed as the cumulative fraction of the total titanium excreted. The cumulative data was plotted, and the time to excrete 50% of titanium (T50) was determined from the cumulative curve using the following model (Almirall and Esteve-Garcia Citation1994):
where t = time (hour), y = cumulative excreted titanium, m = slope of the line and k = correlation coefficient.
The goodness of fit of the data to the Hill-type equation was tested. Then, the curves were transformed into a linear equation to determine the value of m and k, as follows:
where Y = ln (y/1−y), a = m (gradient of the line), X = ln (t) and b = −ln (k).
The mean retention time (MRT) was calculated according to the method established (Coombe and Kay Citation1965):
where x is the amount of excreted titanium of the ith collection at the tth hour.
The AME, AICPD and AIAAD of PKC and treated PKC were determined following the calculations described by Abdollahi et al. (Citation2015) and Awad et al. (Citation2016):
Statistical analysis
All data were subjected to 1-way ANOVA using the GLM procedure of SAS software (SAS Institute Inc., Cary, NC, USA). The mean values of the four types of PKC were separated by Duncan’s multiple range test. Results were considered statistically significant at p < .05.
Results
The chemical compositions of untreated and treated PKC are shown in . The DM of EPKC, XPKC and LPKC were higher (p < .001) than the untreated PKC. The GE of treated PKCs was improved (p < .001) as compared to the untreated one, where the EPKC showed the highest value (4294 kcal/kg) followed by XPKC and LPKC which had similar values (4201 kcal/kg). The CP of EPKC and LPKC but not those of XPKC were higher (p < .001) than the untreated PKC. The EE of EPKC was similar (p > .05) to LPKC and XPKC, but significantly higher than untreated PKC. The CF value was lower (p < .001) in LPKC, XPKC and EPKC compared to the untreated PKC. Except for XPKC, LPKC and EPKC had lower (p < .001) NDF content than the untreated PKC. The ADF of LPKC was lower (p < .001) than the other groups. The LPKC, EPKC and XPKC showed reductions (p < .001) in their hemicellulose, cellulose and lignin contents as compared to untreated PKC.
Table 4. Chemical compositions of untreated and treated PKC.
The amino acid concentrations of various types of PKC are presented in . As compared to the untreated PKC, LPKC showed improved (p < .001) lysine, methionine, valine, leucine, tyrosine, alanine, glutamic acid, aspartic acid and proline concentrations. The concentrations of glycine, alanine and aspartic acid of EPKC were higher (p < .001) than the untreated PKC. Only alanine and glutamic acid concentrations were enhanced (p < .001) in XPKC when compared to the untreated PKC. Irrespective of treatment, the concentrations of threonine, arginine, isoleucine, histidine, phenylalanine, cysteine and serine were not affected.
Table 5. Amino acid concentrations of various treated PKC.
The type of PKC had negligible (p > .05) effect on T50 or MRT (). The results of AME, AICPD and AIAAD of various PKC are shown in and . The AME values of EPKC, XPKC and LPKC were higher (p < .001) than the untreated PKC. Both LPKC and EPKC showed higher (p < .001) AIDCP percentages than the untreated PKC. The total AA digestibility of LPKC but not those EPKC and XPKC was higher (p < .001) compared to the untreated PKC. On the other hand, all treatments had a negligible effect on the digestibility of methionine, arginine, histidine, cysteine and glycine.
Table 6. Effect of various treated PKC on the time of 50% excretion (T50) and mean retention time (MRT) of feed in broiler chickens kept under tropical environment.
Table 7. Apparent metabolisable energy (AME) and apparent ileal crude protein digestibility (AICPD) of various treated PKC in broiler chickens kept under tropical environment.
Table 8. Apparent ileal amino acids digestibility of various treated PKC in broiler chickens kept under tropical environment.
Discussion
In the current experiment, the experimental diets were designed to evaluate the effect of a single dietary component on the passage time of feed, besides determining AME and AIAAD of various treated PKC under the tropics. The present findings showed that the analysed values for NDF were 75.0, 72.6, 75.4 and 70.3%, for RPKC, EPKC, XPKC and LPKC, respectively. Whereas, the values for ADF were 37.3, 38.7, 39.3 and 33.8% for RPKC, EPKC, XPKC and LPKC, respectively. Although there were significant differences in the insoluble fibre content (NDF and ADF) in different types of PKC, no differences in T50 and MRT were observed among PKC diets. These findings suggest that the various types of PKC had no effect on ROP. Ravindran et al. (Citation1984) observed faster ROP when swine fed increased insoluble fibre content (13.5 to 20.2%), which could be associated with increased digesta bulk. The lack of diet effect on ROP in the present study could be associated with the lower level of PKC inclusion in the diets. According to Mateos et al. (Citation1982) there a negative relationship between the ROP and dietary fat content as higher levels of dietary fat was shown to increase the time taken for the first appearance of the index in excreta (slower ROP) in laying hens. In the current study, however, all the diets were supplemented with similar levels of oil (3.75%), and thus the ROP was not affected.
The determined AME of untreated PKC reported in this study was 1397.6 kcal/kg, which is comparable to the values reported by Saenphoom et al. (Citation2013), Abdollahi et al. (Citation2015) and Hanafiah et al. (Citation2017). However, it is lower than the AME determined by other researchers (Sundu et al. Citation2005). The variations in the AME values of PKC could be attributed to several factors such as the method of oil extraction, the crude fat content of PKC (Abdollahi et al. Citation2015), and the age of the experimental birds (Sundu et al. Citation2006).
The determined AME of EPKC, XPKC and LPKC were 1946.9, 2025.3 and 1999.9 kcal/kg, respectively. This improvement in the AME of LPKC, XPKC and EPKC could be explained by the reduction in CF content in treated PKC. In the present results, LPKC had a significantly lower CF compared to XPKC and EPKC, which suggests that the fermentation process with lactic acid bacteria led to a higher reduction in polysaccharides. The NSP of PKC is mostly appeared as linear, insoluble and highly crystalline β-mannans, with some galactose substitution, that accounts for 78% of the total NSP. The other constituents include 12% cellulose, 3% (4-O-methyl)-glucuronoxylans, and 3% arabinoglycans (Daud and Jarvis Citation1992). Upon fermentation, a major part of fibres in PKC is reduced to various monosaccharides, which mostly found as mannose (Saenphoom et al. Citation2011; Alshelmani et al. Citation2014; Chen et al. Citation2015). However, it has been reported that the assimilation of mannose is much lower than glucose, particularly when glucose is existing in the system (Chen et al. Citation2015). Although LPKC had lower CF than XPKC and EPKC in the present study, no significant differences in AME values among the treated PKC groups were observed. These findings suggest that even though depolymerisation of PKC releases a great number of sugars, they are primarily mannose, which is weakly absorbed and thus of little benefit to the chicken’s energy metabolism (Saenphoom et al. Citation2013).
The AICPD of untreated PKC found in this study was 45.9%, a value that considered close to the 41.6% reported by Hanafiah et al. (Citation2017) and Abdollahi et al. (Citation2015), but was lower than the value reported by Bryden et al. (Citation2009) who reported CP digestibility of 54%. In the present results, the average AIAAD of untreated PKC (45.1%) reported in this study was close to the value of 48.2% reported by Abdollahi et al. (Citation2015). In terms of treated PKC, LAB fermentation resulted in a significant improvement in the digestibility of all AA except for methionine, glycine and histidine. On the other hand, enzymatic fermentation of PKC only improved the digestibility of lysine, threonine, leucine, alanine and aspartic acid. As for extrusion, a significant improvement was only observed for alanine.
The low AICPD and AIAAD of untreated PKC and XPKC could be related to the high ADF and NDF value, instead of crude fibre in general. Alshelmani et al. (Citation2016) postulated that the abundance of NDF, ADF, insoluble fibres and NSPs might result in lower apparent ileal digestibility of nutrients in PKC for broilers. This argument was also supported by Szczurek (Citation2009), who suggested that the lower digestibility of lysine, threonine, arginine, cystine and some other AA in oilseed rape could be attributed to the high fibre content, and probably the high proportion of NSP and phytates. Consequently, the digestion and absorption of nutrients may be disrupted, due to altered viscosity of intestinal lumen and digesta passage time along the intestinal tract (Sieo et al. Citation2005; Szczurek Citation2009; Rochell et al. Citation2012). On the other hand, the reduction in ADF, NDF and insoluble fibre as reported for EPKC and LPKC can lead to better CP and AA digestibility (Hanafiah et al. Citation2017).
We previously observed that the AME and digestibility of nutrients of PKC were highly reduced in chickens exposed to heat stress conditions. However, the AME value of PKC (1397.6 kcal/kg) observed in this study was substantially higher than that reported earlier (Hanafiah et al. Citation2017). The inconsistency could be attributed to differences in the severity of heat stress between the two environmental conditions.
Conclusions
From this experiment, we found that bacterial fermentation, enzymatic fermentation and thermal extrusion have the potential to improve the AME of PKC. Both bacterial and enzymatic fermentation enhanced the CP digestibility. Compared to untreated PKC, only bacterial fermentation improved the AA digestibility significantly. Our findings suggested that 25% inclusion of untreated or treated PKC in the diet had a negligible effect on the passage time of digesta, thus the measurement of passage time may not be a good indicator of nutritive quality of feed.
Disclosure statement
The authors certify that there is no conflict of interest with any financial organisation regarding the material discussed in the manuscript.
Additional information
Funding
References
- Abdollahi MR, Hosking B, Ravindran V. 2015. Nutrient analysis, metabolisable energy and ileal amino acid digestibility of palm kernel meal for broilers. Anim Feed Sci Technol. 206:119–125.
- Ahmed A, Zulkifli I, Farjam AS, Abdullah N, Liang JB, Awad EA. 2014. Effect of solid state fermentation on nutrient content and ileal amino acids digestibility of palm kernel cake in broiler chickens. Ital J Anim Sci. 13:3293.
- Alimon AR. 2004. The Nutritive Value of Palm Kernel Cake for Animal Feed. Palm Oil Dev. 40:12–14.
- Almirall M, Esteve-Garcia E. 1994. Rate of passage of barley diets with chromium oxide: influence of age and poultry strain and effect of beta-glucanase supplementation. Poult Sci. 73:1433–1440.
- Alshelmani MI, Loh TC, Foo HL, Lau WH, Sazili AQ. 2014. Biodegradation of palm kernel cake by cellulolytic and hemicellulolytic bacterial cultures through solid state fermentation. Sci World J. 2014:1–852.
- Alshelmani MI, Loh TC, Foo HL, Sazili AQ, Lau WH. 2016. Effect of feeding different levels of palm kernel cake fermented by Paenibacillus polymyxa ATCC 842 on nutrient digestibility, intestinal morphology, and gut microflora in broiler chickens. Anim Feed Sci Technol. 216:216–224.
- AOAC; Helrich K, editor. 1990. Official methods of analysis of the Association of Official Analytical Chemists. 15th ed. Arlington (VA): Association of Official Analytical Chemist.
- Awad EA, Zulkifli I, Farjam AS, Loh TC, Hossain MA, Ahmed A. 2016. Effect of low-protein diet, gender and age on the apparent ileal amino acid digestibility in broiler chickens raised under hot-humid tropical condition. Indian J Anim Sci. 86:696–701.
- Bryden W, Li X, Ravindran G, Hew L, Ravindran V. 2009. Ileal digestible amino acid values in feedstuffs for poultry. Barton (ACT): RIRDC.
- Chen WL, Liang JB, Jahromi MF, Abdullah N, Ho YW, Tufarelli V. 2015. Enzyme treatment enhances release of prebiotic oligosaccharides from palm kernel expeller. BioResources. 10:196–209.
- Chen WL, Liang JB, Jahromi MF, Ho YW, Abdullah N. 2013. Optimization of multi-enzyme production by fungi isolated from palm kernel expeller using response surface methodology. BioResources. 8:3844–3857.
- Choct M. 1997. Feed non-starch polysaccharides: chemical structures and nutritional significance. Feed Milling Int. June Issue:13–26.
- Coombe JB, Kay RN. 1965. Passage of digesta through the intestines of the sheep retention times in the small and large intestines. Br J Nutr. 19:325–338.
- Daud MJ, Jarvis MC. 1992. Mannan of oil palm kernel. Phytochemistry. 31:463–464.
- Goering HK, Van Soest PJ. 1970. Forage fiber analyses (apparatus, reagents, prcedures, and some applications). USDA Agriculture Handbook. Washington (DC): Agricultural Research Service, U.S. Dept. of Agriculture.
- Hanafiah HA, Zulkifli I, Soleimani AF, Awad EA. 2017. Apparent metabolisable energy and ileal crude protein digestibility of various treated palm kernel cake based diets for heat-stressed broiler chickens. Eur Poult Sci. 81:1–13. doi:10.1399/eps.2017.198
- Jia W, Mikulski D, Rogiewicz A, Zduńczyk Z, Jankowski J, Slominski BA. 2012. Low-fiber canola. Part 2. Nutritive value of the meal. J Agric Food Chem. 60:12231–12237.
- Law FL, Zulkifli I, Soleimani AF, Liang JB, Awad EA. 2018. The effects of low-protein diets and protease supplementation on broiler chickens in a hot and humid tropical environment. Asian-Australas J Anim Sci. 31:1291–1300.
- Marini AM, Daud MJ, Noraini S, Azahan E. 2005. Performance of locally isolated microorganism in degrading palm kernel cake (PKC) fibre and improving the nutritional value of fermented PKC. J Trop Agric Food Sci. 33:311–319.
- Mateos GG, Sell JL, Eastwood JA. 1982. Rate of food passage (transit time) as influenced by level of supplemental fat. Poult Sci. 61:94–100.
- Muangkeow N, Chinajariyawong C. 2013. Diets containing fermented palm kernel meal with Aspergillus wentii TISTR 3075 on growth performance and nutrient digestibility of broiler chickens. Walailak J Sci Technol. 10:131–147.
- NRC. 1994. Nutrient requirements of poultry. 9th rev ed. Washington (DC): National Academy Press.
- Ravindran V, Kornegay ET, Webb KE. 1984. Effects of fiber and virginiamycin on nutrient absorption, nutrient retention and rate of passage in growing swine. J Anim Sci. 59:400–408.
- Rochell SJ, Applegate TJ, Kim EJ, Dozier W a. 2012. Effects of diet type and ingredient composition on rate of passage and apparent ileal amino acid digestibility in broiler chicks. Poult Sci. 91:1647–1653.
- Roslan MAH, Abdullah N, Abdul Murad NZ, Halmi MIE, Zulkifli I, Mustafa S. 2017. Optimisation of extrusion for enhancing the nutritive value of palm kernel cake using response surface methodology. BioResources. 12:6679–6697.
- Roslan MAH, Abdullah N, Mustafa S. 2015. Removal of shells in palm kernel cake via static cling and electrostatic separation. J Biochem Microbiol Biotechnol. 3:1–6.
- Saenphoom P, Liang JB, Ho YW, Loh TC, Rosfarizan M. 2011. Effect of enzyme treatment on chemical composition and production of reducing sugars in palm (Elaeis guineenis) kernel expeller. African J Biotechnol. 10:15372–15377.
- Saenphoom P, Liang JB, Ho YW, Loh TC, Rosfarizan M. 2013. Effects of enzyme treated palm kernel expeller on metabolizable energy, growth performance, villus height and digesta viscosity in broiler chickens. Asian Australas J Anim Sci. 26:537–544.
- Short FJ, Gorton P, Wiseman J, Boorman KN. 1996. Determination of titanium dioxide added as an inert marker in chicken digestibility studies. Anim Feed Sci Technol. 59:215–221.
- Sieo CC, Abdullah N, Tan WS, Ho YW. 2005. Influence of β-glucanase-producing Lactobacillus strains on intestinal characteristics and feed passage rate of broiler chickens. Poult Sci. 84:734–741.
- Sundu B, Kumar A, Dingle J. 2005. Comparison of feeding values of palm kernel meal and Copra meal for broilers. Proceedings of the Recent Advances in Animal Nutrition in Australia. Vol. 15. 28A.
- Sundu B, Kumar A, Dingle J. 2006. Palm kernel meal in broiler diets: effect on chicken performance and health. Worlds Poult Sci J. 62:316–325.
- Szczurek W. 2009. Standardized ileal digestibility of amino acids from several cereal grains and protein-rich feedstuffs in broiler chickens at the age of 30 days. J Anim Feed Sci. 18:662–676.
- Tan WL. 2016. Fermentation of palm kernel cake by indigenous lactic acid bacteria as potential poultry feed ingredients. Serdang: Universiti Putra Malaysia.
- Zulkifli I, Iman Rahayu HS, Alimon AR, Vidyadaran MK, Babjee SA. 2009. Gut microflora and intestinal morphology of commercial broiler chickens and Red Jungle Fowl fed diets containing palm kernel meal. Arch Fur Geflugelkd. 73:49–55.