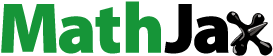
Abstract
Adipose differentiation-related protein (ADRP) is a lipid droplet-associated protein that is expressed early during adipose differentiation. However, the underlying mechanism is still unclear. In this study, the porcine ADRP (pADRP) gene regulatory mechanism and its relationship to intramuscular fat (IMF) content are analysed. We investigated the characteristics of the pADRP gene prompter as well as its transcription level, core promoter and epigenetic modification status using real-time PCR, Dual-luciferase reporter assays and bisulphite sequencing. The results showed that pADRP mRNA expression in Longissimus Dorsi (LD) muscle of the low IMF (LEAN) group was lower than that in the high IMF (FAT) group (p<.01). Maximum promoter activity was found in the −926 bp to −235 bp region. The methylation level of the pADRP of this region (mainly 7th, 10th, 12th CpG sites) in the low IMF group (86.67%) was significantly higher than that in the high IMF group (46.7%) (p<.01). Our result plays an important role in pADRP transcriptional regulation and provides a good prospect for the marbling traits improvement.
pADRP expression differs in divergent IMF content pigs.
Promoter region located −929 to −235 bp, and its methylation contributes to gene expression.
Highlights
Introduction
Intramuscular fat (IMF) influences various meat quality traits including flavour, juiciness and tenderness (Hocquette et al. Citation2010). Therefore, identification of molecular mechanisms of IMF deposition is of prime interest for meat quality research (Suzuki et al. Citation2005). IMF is the percentage of the lipid content in muscle. Lipid droplets are spherical organelles composed of a core of neutral lipids, such as triacylglycerols and cholesterol esters, surrounded by a phospholipid monolayer onto which specific proteins are attached, including the PAT family (named from perilipin, adipose differentiation-related protein (ADRP), tail-interacting protein of 47 kDa (TIP47), S3-12, and OXPAT) (Kim et al. Citation2005; Wolins et al. Citation2006; Brasaemle Citation2007; Bickel et al. Citation2009).
ADRP is a 50-kDa protein expressed in the majority of tissues (Brasaemle et al. Citation1997), involved in the uptake of long chain fatty acids and in the formation and stabilisation of lipid droplets (Jiang and Serrero Citation1992; Brasaemle et al. Citation1997; Imamura et al. Citation2002; Targett-Adams et al. Citation2003). The porcine ADRP gene comprises eight exons and seven introns, spanning 13 kb. The gene was mapped on Sus scrofa chromosome 1 (SSC1) q2.3–2.7 (Kim et al. Citation2005; Nie et al. Citation2005), within a region where several quantitative trait loci (QTLs) affecting meat quality and fat deposition traits have been described (Yue et al. Citation2003; Thomsen et al. Citation2004). Analysis in skeletal muscle of Italian Large White and Italian Duroc pigs divergent for backfat thickness and visible intermuscular fat showed a trend of higher ADRP gene expression level in pigs with higher intermuscular fat (Davoli et al. Citation2011; Gandolfi et al. Citation2011). In addition, single nucleotide polymorphisms (SNPs) in ADRP are significantly correlated with carcass quality traits and the gene expression level in skeletal muscle (Davoli et al. Citation2011).
Methylation of CpG clusters or CpG islands within gene promoters is generally thought to silence gene expression (Jaenisch and Bird Citation2003). Identifying differences in DNA methylation at CpG islands is, therefore, expected to lead to a clearer understanding of the differentiation of normal tissues and the other tissues with the development of complex diseases including cancer (Jones and Takai Citation2001). Although it has been reported that DNA methylation may play a role in the regulation of tissue-specific gene expression (Ching et al. Citation2005), differential DNA methylation patterns among divergent IMF samples in adult pigs were until now not confirmed.
In this study, luciferase reporter gene with serial deletion of pADRP gene 5′-flanking sequences was constructed to identify the core promoter region. The methylation status of specific CpGs in core promoter, as well as the corresponding expression level, was compared between two groups with significantly divergent IMF content, which provided an insight into transcriptional regulation of the pADRP gene.
Materials and methods
Samples collection
The experiments and protocols used in this study were approved by the Nanjing Agricultural University Institutional Animal Care and Use Committee. The samples were collected from 168 healthy commercial cross-breed pigs. Pigs were reared on the same farm, fed with the same diet and slaughtered at approximately 180 d of age. Duplicate Longissimus Dorsi samples at the last rib were taken immediately after slaughter. A copy of the sample was frozen by liquid nitrogen and then stored at −80 °C, whereas another copy of the sample was stored at −20 °C for muscle IMF content determination. The IMF Content was measured via Soxhlet extraction method.
Soxhlet extraction method for IMF content determination
Absorbent filter paper is put into the weighing bottle, dried in oven at 70 °C for 2 hours, and the weight value is recorded (W0) after it is cooled down. About 2 grams of minced Longissimus Dorsi muscle is put into the bag of absorbent filter paper, and the total weight value is precisely recorded (W1). Filter paper bags are dried in oven at 70 °C for 15 hours in oven and record the weight value (W2). Put the bags into the extraction tube, add anhydrous ether to 2/3–3/4 of the total volume of the extraction bottle. Assemble the extraction device and put the extraction tube of Soxhlet extractor in the electric constant 72 °C temperature water bath for extracting about 9 hours. Put paper bag into the weighing bottle and dried it in oven at 70 °C for 10 hours. The weight value is recorded (W3) after it is cooled down.
The IMF content analysis was performed in triplicate for each sample.
W2: weight of dry filter bag with dry sample; W3: weight of dry filter bag with dry sample after extraction; W1: weight of dry filter paper bag with fresh sample. W0: weight of empty dry filter paper bag.
Extraction of DNA and RNA
Total genomic DNA was extracted from longissimus dorsi muscles according to the manufacturer’s protocol using the TIANamp Genomic DNA Kit (TIANGEN, Beijing, China). Total RNA was isolated from longissimus dorsi muscles using Trizol reagent (Invitrogen, CA, USA) according to the manufacturer’s instructions. DNA was stored at −20 °C, while total RNA was stored at −80 °C until use. About 1 µg of total RNA was used to synthesise first-strand cDNA using the M-MLV reverse transcriptase (Promega, San Luis Obispo, CA, USA) and oligo dT(18).
Real-time quantitative PCR
The expression of target genes and the reference gene were quantified by real-time quantitative polymerase chain reaction (RT-PCR) with an ABI 7300 using a commercial kit (SYBR® Premix Ex TaqTM) (TaKaRa, Dalian, China). The gene-specific primers were designed on the basis of porcine mRNA sequences (Table ). Relative expression of mRNA was calculated using the 2−ΔΔCt method, also referred to as the comparative CT method (Schmittgen and Livak Citation2008).
Table 1. List of primer pairs used for construction of promoter, 5′ flanking sequence clone, real-time PCR and bisulfite sequencing PCR.
Cloning of pADRP gene 5′ flanking sequence and bioinformatics analysis
The primers were designed according to pADRP gene 5′ flanking sequence (accession No. ENSSSCG00000005171) from the Ensembl (http://www.ensembl.org/index.html) (Table ). The total 20 μL of PCR reaction mixture contained 2.0 μL 10 × LA PCR buffer II (Mg2+ Plus), 3.2 μL of dNTP (2.5 M), 0.5 μL of forward primer (10 μM), 0.5 μL of reverse primer (10 μM), 50 ng of genomic DNA, and 0.2 μL of LA Taq Polymerase. The reaction condition was as follows: 94 °C for 5 min, then 35 cycles of 94 °C for 30 sec, 58 °C for 30 sec, and 72 °C for 1 min, eventually, at 72 °C for 10 min. The amplification products were detected using 1.0% agarose gel electrophoresis and visualised using a UV transilluminator. Amplicons were cloned using pMD19-T vector TA cloning kits (TaKaRa, Dalian, China) according to the manufacturer’s instructions.
The core promoter region of pADRP gene was predicted using Promoter 2.0 Prediction Server (http://www.cbs.dtu.dk/services/Promoter/) and Promoter Scan (http://www-bimas.cit.nih.gov/cgi-bin/molbio/proscan). Sequence of the pADRP gene 5′ flanking sequence was analysed by Transcription Element Search System (TESS) (http://www.cbil.upenn.edu/cgi-bin/tess/tess) for transcription response elements. The threshold was designed as 85.0 point (highest 100.0) to ensure a good sensitivity and specificity. Predicted important transcription factors that had a score above the threshold were shown in Figure . CpG islands were predicted with CpG Islands 2 (http://ccnt.hsc.usc.edu/cpgislands2/cpg.aspx) and Urogene (http://www.urogene.org/methPrimer) for the promoter region of pADRP. Parameters for CpG island prediction were: size ≥200 nt; GC content = 55% and CpG island observed/expected = 65%.
Construction of promoter reporter
According to above prediction result, the core promoter region of pADRP gene was located. Combining the transcription response elements and CpG islands prediction results, the promoter region was divided into five different lengths of fragments. pADRP gene promoter fragments of five different lengths were amplified by PCR from pMD-19T-ADRP construct using different pairs of primers (Table ). The five PCR products were digested with KpnI and Hind III (TaKaRa, Dalian, China) and immediately inserted into the multiple cloning sites of the pGL3-basic vector (Promega, San Luis Obispo, CA, USA). All constructs were verified by restriction enzyme digestion and sequencing.
Cell culture and transient transfection assay
Porcine PK15 cells (Biowit, Shenzhen, China) were counted in a haemocytometer, and the viability was determined by trypan blue dye exclusion, and then the cells were seeded in 12-well culture plate (Costar; Corning Inc., NY, USA) at a density of 5 × 105 cells per well in 1.0 mL of Dulbecco’s modified Eagle’s medium (DMEM, Gibco,Gaithersburg, MD, USA) supplemented with 10% (v/v) foetal bovine serum (FBS, Gibco, Gaithersburg, MD, USA), 100 units/mL penicillin and 100 μg/mL Streptomycin. Cells were maintained at 37 °C in 5% CO2, and grown for 1–2 days until 70–80% confluent. Exponentially growing cells were used for the transfection experiments.
To normalise transfection efficiency, we employed the Dual-Luciferase reporter assay system, in which the pRL-thymidine kinase (TK) plasmid containing the Renilla luciferase gene under control of the TK promoter was co-transfected as an internal control. Transient transfection of PK15 with pADRP promoter luciferase reporter plasmids (1.6 μg) and pRL-TK vector (0.016 μg) (Promega, San Luis Obispo, CA, USA) by Lipofectamine™ 2000 transfection reagent (Invitrogen, CA, USA) was performed according to the manufacturer’s instructions. Antibiotics were not used during transfection procedures. At 48 h after transfection, cells were lysed using Dual-luciferase Assay System kit (Promega, San Luis Obispo, CA, USA) and assayed for promoter activity using modulusTM single tube utility detector. Each experiment was performed at least three times, in triplicate. Activity was defined as Firefly/Renilla ratio.
As shown in Figure S1, the ADRP gene expression in PK-15 cells is not significantly different with that in SVF cells (stromal vascular fraction cells) and subcutaneous fat, but is significantly lower than that in other tissue such as liver. So PK-15 cells is suitable for promoter activity analysis.
Bisulphite sequencing PCR
DNA samples isolated from Longissimus Dorsi muscles with the lowest IMF and highest IMF (n = 12 per group) were equally mixed as two groups of mixtures for the following bisulphite sequencing separately. A total of 500 ng DNA for every DNA mixture was modified according to the manufacturer’s protocol using MethylCode™ Bisulphite Conversion Kit (Invitrogen, CA, USA), converting thus cytosine into uracil. The methyl-specific primers (Table ) were designed based on bisulphite-modified DNA sequences (Liu et al. Citation2011) to amplify the CpG island. The reaction conditions were as follows: 95 °C for 5 min, then 35 cycles of 95 °C for 30 sec, 56 °C for 30 sec, and 72 °C for 1 min, eventually, at 72 °C for 5 min. The PCR products were purified and ligated into the pMD19-T vector cloning kit (TaKaRa, Dalian, China) according to the manufacturer’s instructions. The pADRP promoters of 20 positive clones (10 positive clones per group) were sequenced in the Invitrogen (Shanghai, China). The positions and percentages of methylated cytosine residues for all non-CpGs were then determined by aligning the sequenced results with the bisulphite-modified DNA sequences converted from Methyl Primer Express 1.0 software.
Statistical analysis
All analyses were performed using the SPSS 17.0 software (IBM, New York, USA). All data were presented as means ± standard errors; between-group differences were analysed using one-way ANOVA (IBM, New York, USA) and Tukey’s post-tests. The results were considered significantly different at p<.05. All experimental treatments were repeated three times.
Results
Individuals with lowest and highest IMF content in the pig population
The IMF percentage was determined in LD muscle samples from 168 pigs. The overall mean ± SE was 3.08 ± 1.35%. In order to constitute two groups with divergent IMF, 12 samples with the lowest IMF (LEAN) and 12 samples with the highest IMF (FAT) were selected. The average IMF were 1.85 ± 0.13% and 4.31 ± 0.32% for LEAN and FAT groups, respectively (p<.01) (Figure ).
Different pADRP expression between LD muscle of LEAN and FAT groups
In order to confirm the effect of ADRP on IMF deposition, firstly the mRNA expression level of pADRP gene was detected by real-time PCR in the LD muscle of LEAN and FAT groups. It was found that pADRP expression in LEAN group was significantly lower than that in FAT (Figure , p<.01). It indicated that pADRP expression was positively associated with IMF content.
Bioinformatics analyses of pADRP gene 5′ flanking sequence
To study the mechanism that pADRP was differentially expressed between samples with low and high IMF, the 1716 bp fragment of ADRP gene 5′ flanking sequence was amplified, which encompassed promoter and partial exon 1 region (Figure ). After analysed by Promoter 2.0 Prediction Server and Promoter Scan, a TATA box sitting at −31 bp upstream of the transcription start site (TSS) was identified in the 5′ flanking region of pADRP gene (Figure ). Further analysis using Transcription Element Search System showed several potential binding sites of transcription factors including HSF, SREBP-, C/EBPβ, RORalp, AML-1a, CdxA, SRY, YY1, ADR1, MyoD, HNF3b, Sp1, GATAs were predicted in the pADRP gene promoter region (Figure ). Moreover, two CpG islands were predicted locating at −253 to −40 bp and −660 to −407 bp (Figure ).
Figure 3. Representative gel photograph of PCR-amplified fragments. (a) Representative gel photograph of PCR-amplified pADRP gene 5′ flanking sequence. (b) Representative gel photograph of BSP analyses using bisulphite-treated DNA. 1: LEAN group; 2: FAT group.
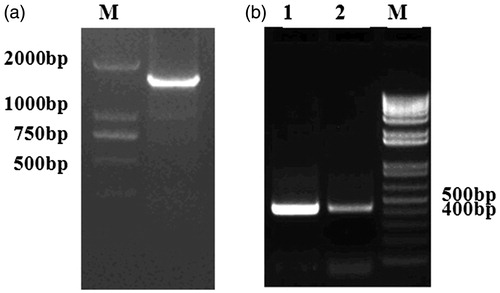
Figure 4. Characterisation of pADRP gene 5′ flanking sequences. Sequences with double underscores and dashed underline represented two CpG islands in the promoter of the pADRP gene region, respectively. Observed/Expected ratio >0.60; the length of CpG islands >200 bp. CpG sites are highlighted in grey. Transcription factor binding sites analysed using TESS are indicated above the sequences.
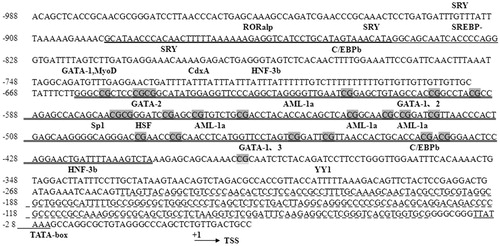
Analyses of the pADRP gene promoter activity
We set out to determine the minimal region required for promoter activity within the upstream pADRP gene sequences. A series of deletion constructs from 5′ flanking sequence were generated via PCR-based approaches (Figure ). These deletion constructs were transiently transfected into PK15 cells, and luciferase activity was detected. Deletion construct (nucleotides −926 to +10 bp and −447 to +10 bp) had significantly elevated promoter activity relative to others (Figure ). Our in vitro data suggested that the core region of pADRP promoter lies in the −926 to −235 bp region. The transcription start site (TSS) was denoted as +1.
Figure 5. Scanning deletion analyses of pADRP promoters in PK cell line. The top panel illustrated the porcine ADRP gene structure. The left side of the diagram illustrated the constructs consisting of the different promoter regions fused upstream of a luciferase reporter, and the right illustrated the relative luciferase activity.
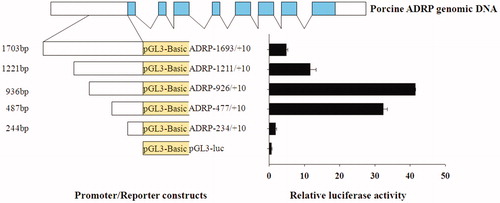
Methylation status of the promoter
Bioinformatics analysis revealed that the 5′ flanking region of the pADRP gene contained two CpG islands located −253 to −40 bp and −660 to −407 bp. Our study indicated a high promoter activity of −926 to −235bp region of pADRP gene. Consequently, we analysed methylation status of the CpG island which located −660 to −407 bp between LEAN and FAT groups. The proportion of the CG content was 56.7% on the whole CpG island. The bisulphite-modified PCR amplicons were showed in Figure . The results showed that the overall methylation levels of 21 analysed CpG sites had no significant difference (p>.05) between LEAN and FAT group (74.76 vs. 66.67%) (Figure ). However, the methylation levels of the 7th, 10th and 12th sites showed extremely significant differences between LEAN and FAT groups (p<.01). The methylated CpG sites in FAT group accounted for 46.7% (14/30), was significantly lower than the LEAN group percentage of 86.67% (26/30) (p<.01) (Figure ).
Figure 6. Methylation status of the pADRP promoter in LEAN group and FAT group LD muscles. (a) Bisulphite sequencing results for LEAN group and FAT group. Each level line represented an individually sequenced clone and circles represented CpG residues. White circles indicated unmethylated CpG sites; black circles represented methylated CpG sites. (b) The histogram of methylation level. **p<.01.
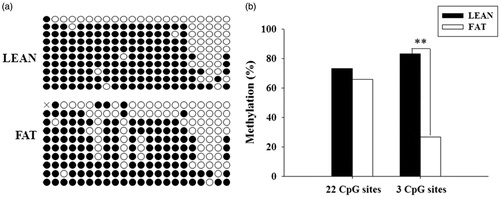
Discussion
The adipose differentiation-related protein (ADRP) was first characterised as a mRNA induced early during adipocyte differentiation (Jiang and Serrero Citation1992). Localisation of ADRP in 1246 cells indicated that ADRP is absent from nuclear and cytosolic fractions and is found as a membrane-associated protein (Jiang and Serrero Citation1992). Further studies demonstrated that ADRP mRNA was expressed in a variety of tissues and cultured cell lines. The specific localisation of adipose differentiation-related protein to lipid droplets in a wide variety of cells suggests that ADRP plays a role in the management of neutral lipid stores (Brasaemle et al. Citation1997; Ye and Serrero Citation1998; Kouba et al. Citation1999; Teixeira et al. Citation2003). ADRP stimulated lipid accumulation and lipid droplet formation without induction of other adipocyte-specific genes or other lipogenic genes in murine fibroblasts (Imamura et al. Citation2002).
PAT family was named from perilipin, which consisted of adipose differentiation-related protein (ADRP), tail-interacting protein of 47 kDa (TIP47), S3-12, and OXPAT (Kim et al. Citation2005; Wolins et al. Citation2006; Brasaemle Citation2007; Bickel et al. Citation2009). Located on the surface of larger TAG droplets in mature adipocytes (Greenberg et al. Citation1991), Perilipin has been postulated to modulate HSL activity and regulate lipolysis and energy balance (Martinez-Botas et al. Citation2000). Genetic variations of perilipin gene, which is the best characterised PAT protein in mammalian, have been associated with metabolic phenotypes, including type 2 diabetes mellitus and obesity (Brasaemle Citation2007; Bickel et al. Citation2009). TIP-47 was thought to act as a cargo selection device for trafficking of mannose-6-phosphate receptors from late endosomes to Golgi (Diaz and Pfeffer Citation1998) in addition to its association with lipid droplets (Wolins et al. Citation2001; Miura et al. Citation2002). S3-12 was originally cloned as a surface/membrane-associated protein in adipocytes (Scherer et al. Citation1998), but later observations suggest that S3-12 coats nascent lipid droplets in adipocytes (Wolins et al. Citation2003).
Although it is well documented that ADRP is implicated in the formation of lipid droplets, few previous study had reported that ADRP expressed in muscle of pigs (Gandolfi et al. Citation2011). In our present study, it was found that mRNA expression levels of pADRP gene in the LD muscle of FAT group was 2 times of that of LEAN group (Figure ). Interestingly, it showed significant positive correlation between pADRP expression level and IMF content. This would suggest that pADRP gene likely played important role in the fat deposition.
To date, there are no research reports about the methylation status of the pADRP gene. In this study, we identified important promoter region of pADRP gene lied in the −926/−235 bp region using luciferase assays. In the promoter region, several adipogenesis-related transcriptional regulatory elements were predicted by online analysis, including C/EBPβ (Rahman et al. Citation2012; Choi et al. Citation2013) and SREBP (Kallin et al. Citation2007; Sekiya et al. Citation2007). Besides, there were several binding sites of GATA transcription factors in this region according to our predicted result. GATA factors belong to zinc finger protein family, and play important roles in eukaryotes development. GATA could regulate adipocyte differentiation by controlling the preadipocyte-adipocyte transition (Tong et al. Citation2000). GATA form protein complexes with C/EBPα and C/EBPβ, which are essential transcription factors for adipogenesis (Tong et al. Citation2005). Transcription binding site for methylation-sensitive transcriptional factor Sp1 (Panigrahi et al. Citation2012) was also found in the promoter region, implying that DNA methylation might play a role in the regulation of pADRP gene expression.
In mammals, DNA methylation is an important mean of epigenetic regulation and controls gene expression through chemical modification without influencing gene sequence. Furthermore, studies showed that DNA methylation was predominantly found within CpG islands of promoter region. We predicted a CpG island of 254 bp length existing in the promoter region of pADRP gene. Bisulphite sequencing results revealed that the methylation levels of this pADRP promoter region within LEAN group was higher than that in FAT group, especially at the 7th, 13th and 17th CpG sites, whose methylation levels showed extremely significant differences (86.67 vs. 46.7% for LEAN vs. FAT). These results were unanimous to the mRNA expression level of the pADRP gene (Gandolfi et al. Citation2011). Overexpression of miR-148a/152 suppressed DNMT1 expression, whereas the expression of adipose differentiation-related protein (ADRP) was enhanced (Yang et al. Citation2017). These studies indicated that promoter methylation contributed greatly to the regulation of pADRP gene expression. Further studies, aimed to investigate the effects of the pADRP gene promoter variation and protein level on fat deposition, could clarify the role of ADRP gene on carcass quality traits in pigs.
Conclusions
To understand the pADRP gene regulatory mechanism and its relationship to IMF content, the present study showed that pADRP mRNA expression in LD muscle of the low IMF group was lower than that in the high IMF group, and the methylation level of the pADRP maximum promoter activity in the low IMF group was significantly higher than that in the high IMF group. Our result plays an important role in pADRP transcriptional regulation and provides a good prospect for the marbling traits improvement.
Ethical approval
The experiments used in this study were approved by the Nanjing Agricultural University Institutional Animal Care and Use Committee (approval number SYXK(SU)2017-0027).
Supplemental Material
Download MS Word (87.6 KB)Disclosure statement
No conflict of interest was reported by the author(s).
Additional information
Funding
References
- Bickel PE, Tansey JT, Welte MA. 2009. PAT proteins, an ancient family of lipid droplet proteins that regulate cellular lipid stores. Biochim Biophys Acta. 1791(6):419–440.
- Brasaemle DL. 2007. Thematic review series: adipocyte biology. The perilipin family of structural lipid droplet proteins: stabilization of lipid droplets and control of lipolysis. J Lipid Res. 48(12):2547–2559.
- Brasaemle DL, Barber T, Wolins NE, Serrero G, Blanchette-Mackie EJ, Londos C. 1997. Adipose differentiation-related protein is an ubiquitously expressed lipid storage droplet-associated protein. J Lipid Res. 38(11):2249–2263.
- Ching TT, Maunakea AK, Jun P, Hong CB, Zardo G, Pinkel D, Albertson DG, Fridlyand J, Mao JH, Shchors K, et al. 2005. Epigenome analyses using BAC microarrays identify evolutionary conservation of tissue-specific methylation of SHANK3. Nat Genet. 37(6):645–651.
- Choi SH, Chung KY, Johnson BJ, Go GW, Kim KH, Choi CW, Smith SB. 2013. Co-culture of bovine muscle satellite cells with preadipocytes increases PPARgamma and C/EBPbeta gene expression in differentiated myoblasts and increases GPR43 gene expression in adipocytes. J Nutr Biochem. 24(3):539–543.
- Davoli R, Gandolfi G, Braglia S, Comella M, Zambonelli P, Buttazzoni L, Russo V. 2011. New SNP of the porcine perilipin 2 (PLIN2) gene, association with carcass traits and expression analysis in skeletal muscle. Mol Biol Rep. 38(3):1575–1583.
- Diaz E, Pfeffer SR. 1998. TIP47: a cargo selection device for mannose 6-phosphate receptor trafficking. Cell. 93(3):433–443.
- Gandolfi G, Mazzoni M, Zambonelli P, Lalatta-Costerbosa G, Tronca A, Russo V, Davoli R. 2011. Perilipin 1 and perilipin 2 protein localization and gene expression study in skeletal muscles of European cross-breed pigs with different intramuscular fat contents. Meat Sci. 88(4):631–637.
- Greenberg AS, Egan JJ, Wek SA, Garty NB, Blanchette-Mackie EJ, Londos C. 1991. Perilipin, a major hormonally regulated adipocyte-specific phosphoprotein associated with the periphery of lipid storage droplets. J Biol Chem. 266(17):11341–11346.
- Hocquette JF, Gondret F, Baeza E, Medale F, Jurie C, Pethick DW. 2010. Intramuscular fat content in meat-producing animals: development, genetic and nutritional control, and identification of putative markers. Animal. 4(02):303–319.
- Imamura M, Inoguchi T, Ikuyama S, Taniguchi S, Kobayashi K, Nakashima N, Nawata H. 2002. ADRP stimulates lipid accumulation and lipid droplet formation in murine fibroblasts. Am J Physiol Endocrinol Metab. 283(4):E775–783.
- Jaenisch R, Bird A. 2003. Epigenetic regulation of gene expression: how the genome integrates intrinsic and environmental signals. Nat Genet. 33(S3):245–254.
- Jiang HP, Serrero G. 1992. Isolation and characterization of a full-length cDNA coding for an adipose differentiation-related protein. Proc Natl Acad Sci U S A. 89(17):7856–7860.
- Jones PA, Takai D. 2001. The role of DNA methylation in mammalian epigenetics. Science. 293(5532):1068–1070.
- Kallin A, Johannessen LE, Cani PD, Marbehant CY, Essaghir A, Foufelle F, Ferre P, Heldin CH, Delzenne NM, Demoulin JB. 2007. SREBP-1 regulates the expression of heme oxygenase 1 and the phosphatidylinositol-3 kinase regulatory subunit p55 gamma. J Lipid Res. 48(7):1628–1636.
- Kim TH, Choi BH, Chang GW, Lee KT, Lee HY, Lee JH, Kim KS, Park CK, Moran C. 2005. Molecular characterization and chromosomal mapping of porcine adipose differentiation-related protein (ADRP). J Anim Breed Genet. 122(4):240–246.
- Kouba M, Bonneau M, Noblet J. 1999. Relative development of subcutaneous, intermuscular, and kidney fat in growing pigs with different body compositions. J Anim Sci. 77(3):622–629.
- Liu Z, Li Q, Pan Z, Qu X, Zhang C, Xie Z. 2011. Comparative analysis on mRNA expression level and methylation status of DAZL gene between cattle-yaks and their parents. Anim Reprod Sci. 126(3–4):258–264.
- Martinez-Botas J, Anderson JB, Tessier D, Lapillonne A, Chang BH, Quast MJ, Gorenstein D, Chen KH, Chan L. 2000. Absence of perilipin results in leanness and reverses obesity in Lepr(db/db) mice. Nat Genet. 26(4):474–479.
- Miura S, Gan JW, Brzostowski J, Parisi MJ, Schultz CJ, Londos C, Oliver B, Kimmel AR. 2002. Functional conservation for lipid storage droplet association among Perilipin, ADRP, and TIP47 (PAT)-related proteins in mammals, Drosophila, and Dictyostelium. J Biol Chem. 277(35):32253–32257.
- Nie T, Zhao XL, Qiu H, Xia T, Chen XD, Gan L, Feng SQ, Lei T, Dai MH, Yang ZQ. 2005. Sequence analysis and map assignment of pig SREBF2 and ADFP. Anim Genet. 36(5):455–457.
- Panigrahi SK, Vasileva A, Wolgemuth DJ. 2012. Sp1 transcription factor and GATA1 cis-acting elements modulate testis-specific expression of mouse cyclin A1. PLOS One. 7(10):e47862.
- Rahman SM, Janssen RC, Choudhury M, Baquero KC, Aikens RM, de la Houssaye BA, Friedman JE. 2012. CCAAT/enhancer-binding protein β (C/EBPβ) expression regulates dietary-induced inflammation in macrophages and adipose tissue in mice . J Biol Chem. 287(41):34349–34360.
- Scherer PE, Bickel PE, Kotler M, Lodish HF. 1998. Cloning of cell-specific secreted and surface proteins by subtractive antibody screening. Nat Biotechnol. 16(6):581–586.
- Schmittgen TD, Livak KJ. 2008. Analyzing real-time PCR data by the comparative C(T) method. Nat Protoc. 3(6):1101–1108.
- Sekiya M, Yahagi N, Matsuzaka T, Takeuchi Y, Nakagawa Y, Takahashi H, Okazaki H, Iizuka Y, Ohashi K, Gotoda T, et al. 2007. SREBP-1-independent regulation of lipogenic gene expression in adipocytes. J Lipid Res. 48(7):1581–1591.
- Suzuki K, Irie M, Kadowaki H, Shibata T, Kumagai M, Nishida A. 2005. Genetic parameter estimates of meat quality traits in Duroc pigs selected for average daily gain, longissimus muscle area, backfat thickness, and intramuscular fat content. J Anim Sci. 83(9):2058–2065.
- Targett-Adams P, Chambers D, Gledhill S, Hope RG, Coy JF, Girod A, McLauchlan J. 2003. Live cell analysis and targeting of the lipid droplet-binding adipocyte differentiation-related protein. J Biol Chem. 278(18):15998–16007.
- Teixeira L, Rabouille C, Rorth P, Ephrussi A, Vanzo NF. 2003. Drosophila Perilipin/ADRP homologue Lsd2 regulates lipid metabolism. Mech Dev. 120(9):1071–1081.
- Thomsen H, Lee HK, Rothschild MF, Malek M, Dekkers JC. 2004. Characterization of quantitative trait loci for growth and meat quality in a cross between commercial breeds of swine. J Anim Sci. 82(8):2213–2228.
- Tong Q, Dalgin G, Xu H, Ting CN, Leiden JM, Hotamisligil GS. 2000. Function of GATA transcription factors in preadipocyte-adipocyte transition. Science. 290(5489):134–138.
- Tong Q, Tsai J, Tan G, Dalgin G, Hotamisligil GS. 2005. Interaction between GATA and the C/EBP family of transcription factors is critical in GATA-mediated suppression of adipocyte differentiation. Mol Cell Biol. 25(2):706–715.
- Wolins NE, Brasaemle DL, Bickel PE. 2006. A proposed model of fat packaging by exchangeable lipid droplet proteins. FEBS Lett. 580(23):5484–5491.
- Wolins NE, Rubin B, Brasaemle DL. 2001. TIP47 associates with lipid droplets. J Biol Chem. 276(7):5101–5108.
- Wolins NE, Skinner JR, Schoenfish MJ, Tzekov A, Bensch KG, Bickel PE. 2003. Adipocyte protein S3-12 coats nascent lipid droplets. J Biol Chem. 278(39):37713–37721.
- Yang A, Sun Y, Gao Y, Yang S, Mao C, Ding N, Deng M, Wang Y, Yang X, Jia Y, et al. 2017. Reciprocal regulation between miR-148a/152 and DNA methyltransferase 1 is associated with hyperhomocysteinemia-accelerated atherosclerosis. DNA Cell Biol. 36(6):462–474.
- Ye H, Serrero G. 1998. Stimulation of adipose differentiation related protein (ADRP) expression by ibuprofen and indomethacin in adipocyte precursors and in adipocytes. Biochem J. 330(2):803–809.
- Yue G, Beeckmann P, Moser G, Muller E, Bartenschlager H, Cepica S, Schroffel J, Stratil A, Geldermann H. 2003. QTL alleles on chromosome 7 from fatty Meishan pigs reduce fat deposition. Sci China Ser C-Life Sci. 46(1):10–17.