Abstract
Arginine plays an important role during reproduction, however, the amount of supplementation in sow diet is still uncertain. The aim of the study was to verify the efficacy of a gestating diet enriched or not with a low dose of L-arginine (Arg) on sow productive performance in terms of numbers and weight of piglets at birth and at weaning, frequency of intrauterine growth retardation (IUGR) and piglets’ mortality, sow placenta weight and salivary humoral immunity and intestinal microbial balance of the sows. 205 sows (Landrace x Large White) were divided into two experimental groups: a control group (CON) (102 sows) and a group supplemented with 0.25% of Arg (ARG) for the whole pregnancy period. Saliva and faecal samples were collected two days before farrowing and used for immunoglobulins and microbial analysis, respectively. Arg improved the number of total born piglets (p = .043) and tended to improve the number of total born alive (p = .086) and to reduce IUGR % (p = .090) and dead piglets at d0–d3 (p = .088). The weight of placenta and humoral immunity were not influenced by Arg. Arg did not modify the faecal microbial structure (alpha and beta indices) but increased the relative abundance of Bacteroidaceae family and Bacteroides genera (p = .0001). The results support the knowledge that Arg plays a key role in nutrition and physiology of pregnant sows without compromising gut eubiosis.
Arg supplementation of sows’ gestation diet increased the number of total borns
Arg supplementation of sows’ gestation diet did not negatively affect the sows’ gut eubiosis
Arg plays a significant role in the nutrition of pregnant sows.
Highlight
Keywords:
Introduction
The modern genetic lines of pigs are characterised by high prolificacy, but this is often to the detriment of a greater weight dis-homogeneity of the single individuals in the litter with a higher percentage of underweight animals (<1 kg) than in crossbreeds not selected for this trait (Yuan et al. Citation2015). The latter factor is positively correlated to the mortality and morbidity rate in the weaning-birth period. In fact, lower birth weight is associated with reduced vitality and lower physiological maturity (Yuan et al. Citation2015). In addition to the problems highlighted, the incapacity to produce colostrum and milk of adequate quantity and quality to support the nutritional needs of all born piglets was found, especially for highly prolific sows (Theil and Hurley Citation2016).
As regards the foetal development of piglets, adequate vasculogenesis and angiogenesis are necessary to guarantee blood circulation of the placenta, this is essential for the correct development of foetuses and a reduction in the rate of embryonic mortality. Sexually mature sows can gestate a relatively small number of foetuses (8.5 live pigs/litter; Easter and Baker, Citation1976) when fed diets without Arginine, because of their ability to synthesise the needed amount of this amino acid. However, this endogenous synthesis may be challenged in high prolific sow. Recent studies have shown how the integration of L-Arginine (Arg) in the initial stage of gestation of the sow, has favoured embryonic survival, the number of births and birth weight (Gonçalves et al. Citation2016), as well as an integration in the final stages, can reduce stillborn and underweight piglets (Nuntapaitoon et al. Citation2018). Moreover, Arg is a precursor for several physiological processes such as the lipid metabolism in gestation and the production of polyamines and nitric oxide that exert a stimulating effect for placental angiogenesis (Wu et al. Citation2017). Release of polyamines can also originate in the gut after the Arg utilisation by intestinal bacteria (Matsumoto et al. Citation2019). Furthermore, Arg can modulate amino acids utilisation of pure culture of intestinal bacteria or mixed bacteria obtained from porcine intestines (Dai et al. Citation2012). Thus, it might also be hypothesised that Arg could change the gut microbial profile, as observed in mice fed supplementary Arg together with immune modulators (Ren et al. Citation2014). The importance of the intestinal microbiota is becoming more and more evident as a factor capable of modulating the physiological development and health status of pigs, preventing colonisation by pathogenic bacteria in the piglet (Schokker et al. Citation2014). It is not known, however, if the Arg supplementation to a gestation diet could have an impact on the composition of the sow’s intestinal microbiota and therefore, in the first days of life of the piglet, this could lead favourably to the establishment of a ‘beneficial’ microbial profile (eubiosis) contributing to maintaining a good health status of suckling piglets (Mach et al. Citation2015; Stokes et al. Citation2017; Trevisi et al. Citation2018).
To establish an integration plan with this amino acid, aspects relating to the pregnancy period, supplementation levels and the source of Arg must be well defined (Palencia et al. Citation2018), but also aspects related to the cost-efficacy of Arg. Indeed, most of the reported tests were done with very high additions, included in a tested level ranging from 0.4% to 1.3% and with 1% as the most tested level (Palencia et al. Citation2018).
The objective of this study was to verify the efficacy of a gestating diet enriched or not with Arg on the number of piglets born, born alive and malformed or presenting the intrauterine growth retardation (IUGR), the intestinal microbial balance of the sows and their response in humoral immunity, and on their maternal performance, evaluated in terms of homogeneity of the litter at birth, survival, and weight of the litter at weaning.
Materials and methods
The trial was conducted in a commercial multiplication unit located in the so-called ‘Italian Food Valley’ and sows were deputed in producing pigs reared for the production of PDO Parma ham. The animals involved in the present study were sows and piglets subjected to conventional farm rearing condition in Europe (EU) by the Dir. 120/2008 EC. Sows were reared in groups since the fourth week of gestation, on day 110 of gestation, were moved to individual farrowing crates 5 m2 with a slatted floor.
According to a proper feeding programme, on days 1–30 of gestation, gilts and multiparous sows were daily fed 2.2 and 2.4 kg diet (on an as-fed basis), respectively; on days 31–90 of gestation, gilts and multiparous sows were daily restricted to 1.9 and 2.1 kg diet respectively, and after day 90 of gestation, gilts and multiparous sows were fed 2.4 and 2.6 kg of the diet. Pregnant and lactating sows were provided free access to drinking water and consumed all the feed offered throughout the experiment.
A total of 205 sows (Landrace × Large White) were included in the trial. The experiment was designed in a 2 × 5 factorial arrangement with 2 dietary treatments and 5 classes of sow parity. The sows were divided into the two experimental groups, balanced for parity order (3.26 ± 2.2 and 3.37 ± 2.2, respectively for i and ii groups): (i) control group (CON) fed the standard gestation diet usually used at the farm (102 sows); (ii) Arginine group (ARG) fed the CON diets supplemented with 0.25% of total Arg (103 sows). Sow parity was considered as parity class in the following five groups: 1: parity one; 2 = parity 2; 3 = parity three; 4= parities 4 and 5; 5 = parities from 6 to 9.
The CON diet was formulated to cover or surpass the nutrient requirements reported for gestation sows by the American National Research Council (2012); while the ARG diet was obtained by adding to the CON diet a supplement of 0.25% L-Arg with a final concentration of 0.97% of total Arg. The selected Arg level was chosen to test a practical dose suitable for the whole duration of pregnancy period, that could result in a beneficial effect for the performance of the sows in a field condition, thus without increasing to much the economic cost for feed the sows. The ingredients and the analysed composition of the CON diet are reported in Table . The two experimental diets were administrated in crumble form by the automated system throughout the whole gestation period.
Table 1. Ingredients and composition of the sows’ gestating control diet expressed on as fedTable Footnotea.
Sampling and measurements
The duration of the gestation period was recorded for each sow. At the end of farrowing, the placenta was individually collected and weighted. The total number of piglets, the total number of alive piglets, the number of stillbirth piglets and number of IUGR piglets were recorded for each sow. The evaluation criteria for IUGR piglets referring to the pre-weaning piglet head-shape indicators were assessed as recommended and developed by PROHEALTH European project. Piglets were defined as ‘normal’, ‘slightly IUGR’ or ‘moderate IUGR’. Criteria for growth restriction were (i) steep, dolphin-like forehead, (ii) bulging eyes, (iii) wrinkles perpendicular to the mouth (Hales et al. Citation2013).
Inside each dietary treatment, a subgroup of sows balanced for parity order (3.29 ± 2.18 for CON and 3.52 ± 2.17 for ARG group) was chosen (62 sows for CON and 63 sows for ARG group) to record the individual piglets weight at birth (BW0) and at weaning (BW1; 23 ± 1 days after birth).
Two days before the farrowing, a faecal swab and a saliva sample (collected two hours after the morning meal) were collected from 36 sows for each dietary subgroup, again balanced for the parity order. For each sow, a cotton swab was introduced into their mouths thus stimulating chewing for averagely 3 min. Recovery of the saliva sample was achieved by returning the swab to a Salivette tube (SARSTED, Numbrecht, Germany) and centrifuging the container at 900 x g for 10 min. In doing so, the impurities remained in the bottom of the tube, whereas the clear supernatant was collected in a vial. All the samples were stored at −20° C for further analyses.
Microbiota analysis
Total bacterial DNA for microbiota analysis was extracted from the faecal samples by using FastDNATMSpin Kit for Soil, MP Biomedicals Europe, (LLC). DNA quantity and quality were evaluated using Nanodrop ND 1000 spectrophotometer (Nanodrop Technologies Inc., Wilmington, DE, USA). The Next Generation Sequencing (NGS) was performed as reported by Luise et al. (Citation2019a). Briefly, the DNA was amplified for the V3-V4 hypervariable regions of the 16S rRNA gene amplicons were produced using the primersPro341F: 50-TCGTCGGCAGCGTCAGATGTGTATAAGAGACAGCCTACGGGNBGCASCAG-30 and Pro805R: 50GTCTCGTGGGCTCGGAGATGTGTATAAGAGACAGGACTACNVGGGTATCTAATCC-30 using the Platinum™ Taq DNA Polymerase High Fidelity (Termo Fisher Scientific, Italy). The libraries were prepared using the standard protocol for MiSeq Reagent Kit v3 and sequenced on MiSeq platform (Illumina Inc., San Diego, Ca, USA).
The raw reads obtained are publicly available at the European Nucleotide Archive (ENA) under the accession number PRJEB36359.
Saliva analysis
Saliva samples were analysed for immunoglobulin (Ig) profile through an enzyme-linked immunosorbent assay (ELISA) for IgA and IgG by using pig-specified antibodies goat anti-pig IgA/IgG-affinity purified and goat anti-pig sIgA/slgG-HRP conjugate (BETHYL Laboratories, Montgomery, TX). Pig Immunoglobulin Reference Serum (BETHYL, Laboratories, Montgomery, TX) was used as standard. The samples were analysed in duplicate at dilutions of 1:4000 and 1:2000 for IgA and IgG, respectively. Absorbance was set at 405 nm on the Multiskan multiplate reader (Multiskan™ FC Microplate Photometer - Thermo Fisher Scientific) and Igs concentrations were calculated by interpolating them from a parametric curve of four standard points.
Statistical analyses
Pig weight, gestation length and saliva Igs were analysed by using the GLM procedure of SAS 9.3 (SAS Inst. Inc., Cary, NC) through a linear model including batch, diet and parity order class as factors. The interaction between diet and parity order was tested and resulted not significant, thus it was removed from the model. The number of pigs born alive and the number of pigs per litter after cross-fostering was included as covariates respectively for placental weight, gestation length and Ig contents, and for data of piglets’ weight at weaning and growth.
The GENMOD procedure of SAS 9.3 was used with Poisson distribution and log function to analyse data on litter size (number of pigs per litter) at birth and at weaning and with a binomial distribution and Logit function to analyse mortality parameters and data on litter size ratio expressed in percentage. The models included cycle, diet, class of parity order and interaction between diet and parity order as factors. With the same model, the GENMOD procedure of SAS 9.3 was used with beta distribution for the coefficient of variation of newborn pig weights, calculated as the ratio between the standard deviation and the mean within each litter. The gestation length and the number of pigs per litter after adoptions were included as covariates for mortality calculations.
Microbiota analysis was performed by using DADA2 pipeline (Callahan et al. Citation2016) and taxonomic categories were assigned by using Silva Database (release 132) as reference (Quast et al. Citation2012). Alpha (Shannnon, Chao1 and Simpson indices) and Beta diversity (calculated as Bray Curtis distance matrix), as well as the abundance of taxonomic categories, were analysed with R software 3.6, by using the PhyloSeq (McMurdie and Holmes Citation2013), Vegan (Dixon Citation2003) and lme4 (Bates et al. Citation2015) packages. Alpha diversity indices were analysed with an ANOVA model, considering batch experimental treatment and parity order classes as factors. Beta diversity was analysed with a PERMANOVA model (‘Adonis’ procedure) including treatment and class of parity order as factors. The batch was initially included and then removed because not significant. Effect of diet and parity class on Bray Curtis distance were visualised using a Non Metric Multidimensional Scaling (NMDS) approach. The differences in taxonomic abundances between the two experimental groups (CON and ARG) were analysed with DESeq2 package (Love et al. Citation2014), based on negative binomial generalised linear models and applying the Benjamini-Hochberg method for multiple testing correction (Love et al. Citation2014).
Results
Sow reproductive performance
Table shows the effect of diet treatment and litter sow parity order on the litter characteristics and its survival. Arg inclusion did not affect the number of stillborn, mummified, dead in the period d0–d27 and weaned piglets and the percentage of stillborn/total born, mummified/mummified and total born, dead piglets d0–d3/total post-adoption and dead piglets d0–d27/total post-adoption. Tendencies for the Arg supplementation to improve the number of total born alive (p = .086) and the number of dead piglets at d0–d3 (p = .088) were observed. Conversely, in the ARG group, the number of total born pigs was higher than in the CON group (p = .043). An effect of the class of parity was also observed for the number of mummified (p < .001), of dead piglets d0–d3 (p = .001), of dead piglets d0–d27 (p = .007), and for the percentage of mummified/mummified and total born (p < .0001), dead piglets d0–d3/total post-adoption (p = .01) and dead piglets d0–d27/total post-adoption (p = .003). Moreover, a positive effect for the litter size was observed for the percentage of dead piglets at d0–d3 and d0–d27 (p = .001).
Table 2. Influence of Agr supplementation to gestation sow on litter characteristics and survival.
Figure shows the effect of diet treatment on the percentage of IUGR piglets. The Arg supplementation tended to reduce the percentage of slight and total IUGR piglets (p = .09). In addition, the parity class significantly influenced the percentage of slight IUGR (p = .04) and tended to influence the percentage of total IUGR piglets (p = .07).
Figure 1. Influence of Arginine supplementation to sow on percentage of IUGR piglets. IUGR: intrauterine growth retardation; ARG: L-arginine; CON: control group.
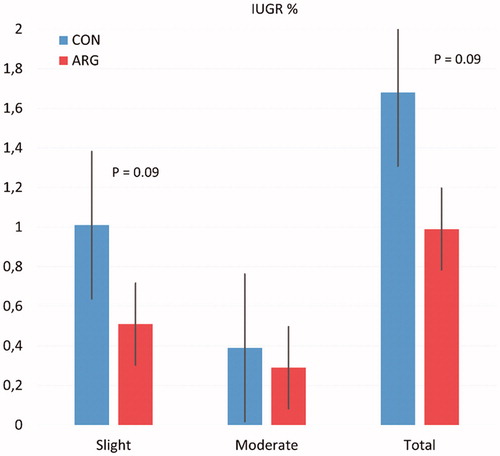
Table shows the effect of diet and sow parity class on weight and ADG of piglets at different times points. No significant differences were observed for the diet on piglet weight and ADG at any time, and also for the coefficient of variation of the individual piglet weight at birth within the litter. The parity class significantly influenced the weight of piglets of the litter , the homogeneity at birth and at weaning and the piglets’ ADG during the suckling period (p < .005).
Table 3. Influence of Arginine supplementation to gestation sow on piglet and litter performance.
Furthermore, the litter size at d1 significantly influenced the piglet's weight at weaning (coefficient −83 g, + 1 pig in the litter; p = .027) and the litter ADG during the suckling period (coefficient + 99 g, + 1 pig n the litter; p = .001).
Table reports the effect of diet treatment and sow parity class on the placenta weight and on the duration of the gestation period. No significant effects were observed for the diet on the placenta weight and on the duration of the gestation period, while the sow parity class had a linear and quadratic effect on the placenta weight (p < .001).
Table 4. Influence ofArginine supplementation to sow and sow parity on piglet and litter performance.
Saliva immunoglobulins
Table reports the effect of diet and sow parity class on the salivary IgA and IgM concentration. The diet did not influence the Igs salivary concentrations. The class of parity had a quadratic effect on the IgA concentration (p < .04); a maximum concentration of IgA was observed in the sow of third parity, then the concentration decreased in older sows.
Table 5. Influence of Arginine supplementation to sow and sow parity on sow salivary Igs.
Microbial profile
A total of 2,706,443 reads were attributed to a total of 3010 (ASV) distributed among samples as shown in S1 Table. The relative rarefaction curves are reported in Figure , it shows the tendency to the plateau for all samples suggesting that the sequencing depth was sufficient to describe the variability within the analysed microbial communities. The taxonomic assignment allows obtaining 18 phyla, 38 classes, 85 families and 212 genera.
Figure 2. Rarefaction curve of samples resulted by sequencing of V3–V4 regions with MiSeq platform (Illumina Inc., San Diego, Ca, USA). Diet: ARG: arginine supplementation (0.25%) to sows during gestation period (36 sows); CON: control group, fed the standard diet (36 sows).
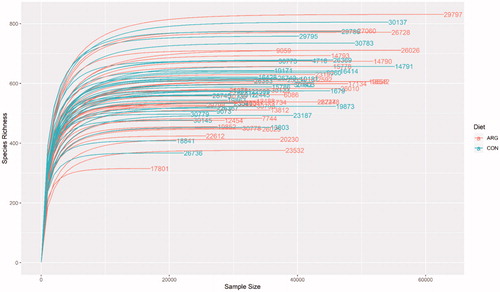
The diet did not influence the alpha diversity indices (Figure ). The beta diversity was not influenced by the diet treatment, as obtained by the Adonis procedure, indeed the NMDS plot did not evidence any cluster of samples due to the diet (Figure ).
Figure 3. Box plot of Shannon index values. Diet: ARG: arginine supplementation (0.25%) to sows during gestation period (36 sows); CON: control group, fed the standard diet (36 sows).
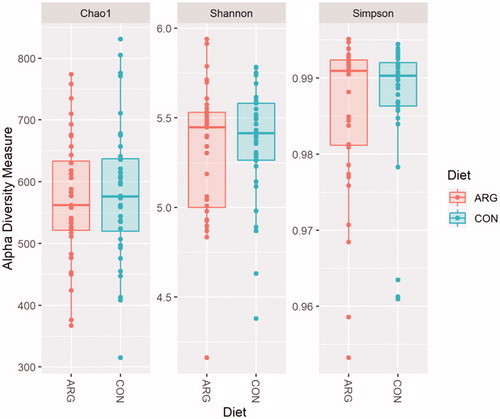
Figure 4. Non-metric multi-dimensional scaling (NMDS) on Bray-Curtis distances at ASVs level. Diet: ARG: arginine supplementation (0.25%) to sows during gestation period (36 sows); CON: control group, fed the standard diet (36 sows).
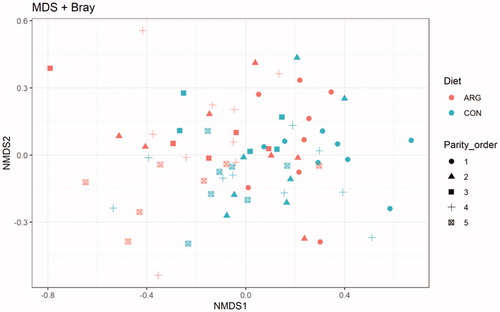
Considering the microbial composition, the diet significantly influenced some taxa (Table ). At class level, the ARG group had a lower relative abundance of Negativicutes than CON group (p = .003); at order level, the ARG group had lower relative abundance of Selenomonadales (p = .005) and Aeromonadales (p = .005) than CON group; at family level, the ARG group had a higher relative abundance of Bacteroidaceae (p < .0001) and a lower relative abundance of Succinivibrionaceae (p = .006), Acidaminococcaceae and Veillonellaceae (p < .05) than CON group; at genus level, the ARG group had had a higher relative abundance of Bacteroides (p < .0001) and a lower relative abundance of Succinivibrio (p = .02) than CON group; finally considering the ASV level, the ARG group had had a higher relative abundance of Treponema_2 (p < .0001) and a lower relative abundance of Prevotella_9 and Ruminococcaceae_UCG-014 (p < .0001) than CON group.
Table 6. Influence of Arginine supplementation to sow during gestation on sow faecal taxa abundancy.
Discussion
Increased intake of some amino acids compared to the nutrient requirements of maintenance and growth, may result in better animal health (Babinszky and Halas Citation2009). On this regards, maternal nutrition plays an important role in influencing the intrauterine environment and in regulation the foetal growth, development and survival (Wu et al. Citation2004), especially Arg seems to have an important role for the sow performance. The present study shows that an integration of 0.25% of Arg to a commercial gestation diet, resulting in a final concentration of 0.97% of total Arg, improved the total number of born piglets and tended to improve the number of born alive piglets, parameters that are considered an important economic outcome for pig production (Bee Citation2007). Our data are in accord with previous studies in which an increase of total born (1.31 piglets) (Gao et al. Citation2012) and of born alive piglets (1.1 and 2.03 piglets) (Gao et al. Citation2012; Mateo et al. Citation2007, respectively) was observed with supplementation of 1.0% of L-Arginine-HCl from day 2 to day 114 of gestation (Gao et al. Citation2012) and with the same dose from day 30 to day 114 of gestation in gilts (Mateo et al. Citation2007). It worth to add that in this last research the basal diet contained 0.88% total Arg, compared to our trial in which the basal diet had 0.72% of total Arg. Thus the overall content of Arg of supplemented diet in this research was far more than in the present one. The effect of the Arg supplementation on the number of offspring can be attributed to its influence on the arginine-nitric oxide and polyamine pathways; indeed, Arg can be used as a substrate for the nitric oxide via nitric oxide synthase and of polyamine synthesis via ornithine decarboxylase (Flynn et al. Citation2002; Wu et al. Citation2004). Both nitric oxide and polyamine are considered key factors for angiogenesis and for improving the placenta vascularisation (Hazeleger et al. Citation2007) enabling the foetus to obtain sufficient nutrients and oxygen supply (Wu et al. Citation2006) and resulting in a more efficient embryo/foetal development (Wu et al. Citation2004; Kim and Wu Citation2009). Indeed, it has been demonstrated that Arg supplementation can improve myofiber formation and foetus survival (Berard and Bee Citation2010).
In the present study, the placenta was individually weighted and no influences of Arg supplementation has been observed between groups; our result is not consistent with the result obtained by Gao et al. (Citation2012) in which 1.0% L-Arginine–HC improved the placenta weight. Considering that a positive trend for the number of live pigs born per sows was seen in the present trial, it could be hypothesised that the Arg supplementation affected the functional capacity and the vascularisation of the placenta, which are not always positively correlated with the placenta weight (Bazer et al. Citation1988), however further elucidation may be needed to confirm this hypothesis. To support the hypothesis that Arg can improve the placenta efficiency in providing foetus sufficient nutrients and oxygen, in the present study it has been observed that Agr group tended to reduce the percentage of IUGR pigs. The foetal growth restriction has a negative effect on new-born, leading to a high rate of pre-weaning mortality for the largest part of the moderate and severe IUGR pigs (Hales et al. Citation2013). Among the factors that have been associated with IUGR piglets including mother’s genetics and farrowing parity (Matheson et al. Citation2018), correct maternal nutrition during pregnancy plays a crucial role. Conversely, it worth to observe that while parity class powerfully affected the placental weight in the present work, with younger and older sow having lower values, this was not translated in an evident effect on IUGR incidence (p = .07, data not shown).
The Arg supplementation did not modify the piglets' weight, neither at birth not at the weaning, or the piglets' ADG. The effect of Arg on reproductive performance of sows in terms of piglets’ weight is controversial. Some studies reported a significant improvement in the weight of piglets at birth (Gao et al. Citation2012; Nuntapaitoon et al. Citation2018) while others, in agreement with our study, indicate no effects on the piglets and litters weights (Gonçalves et al. Citation2016; Bass et al. Citation2017). This difference among studies can be explained by the difference in the amount and in the duration of Arg supplementation in the gestation period. In our study, although the supplement in Arg had been included for the whole duration of the gestation period, the control group had a relatively high amount of Arg (0.72%); therefore, the lack of effect could be due to the fact that the Arg level among the two groups was not too different. Although the average piglets’ weight was not different between diets, in our study it has been observed a tendency to reduce the piglet mortality during the first three days of life. Previous studies suggested the absence of an effect of Arg on pre-weaning mortality. In these studies, Arg supplementation was done just from day 85 of gestation to farrowing (Nuntapaitoon et al. Citation2018) or from 15 to 45 days of gestation (Hines et al. Citation2019). On the contrary, Hines et al. (Citation2019), shows that the same inclusion of Arg supplemented from 15th day of gestation to farrowing reduced the piglets’ post-weaning mortality. Thus, supplementation of Arg on piglets’ mortality is still to be clarified and its response varies depending on dose and period of supplementation.
Considering the additional parameters related to the sows’ health status collected in the present study, no effect of Arg supplementation has been observed on the salivary Igs concentration. Saliva collection has the advantage of being non-invasive sampling as it causes minimal stress during collection, thus it represents an ideal tool for stress physiology and health evaluation (Luise et al. Citation2019b; Escribano et al. Citation2015). The concentrations of IgA and IgG observed in the present study are in line with the values observed by Escribano et al. (Citation2012); although the diet did not affect the Igs concentrations, it is noteworthy the quadratic effect observed for the sow parity on salivary IgA, in which a maximum concentration has been observed for the third parity sows.
The host-microbial interplay is recently rising the scientific interest. Indeed, the microbiota can influence the host metabolism as it is involved in many functions including the production of volatile fatty acid and vitamin K, can improve energy harvesting capacity and enhance resistance against pathogenic bacteria (Stokes Citation2017; Luise et al. Citation2019a). These actions can overall contribute to an improvement of the host performance (Mach et al. Citation2015; Yang et al. Citation2017; Kiros et al. Citation2019; Quan et al. Citation2018). The diet, together with additional factors such as host genetics and environment (Xiao et al. Citation2016; Luise et al. Citation2019a; Massacci et al. Citation2020) represents one of the main factors able to rapidly modified microbial profile (David et al. Citation2014). Therefore, it can be assumed that supplementation of free Arg can affect the composition of the intestinal microbiota, as observed in mice supplemented with Arg and immunostimulants (Ren et al. Citation2014). Free Arg has been considerably used in the small intestine by the microbiota, as reported for the porcine digesta content by Dai et al. (Citation2010). Previous in vitro study showed that Arg can significantly influence, in a species- and gut tract- dependent manner, the bacterial metabolism of the Arg-related AAs and of the serine- and aspartate-related family of AAs, and the utilisation of most AAs, since Arg can be used as a nitrogen source (Dai et al. Citation2012). In the present study, the small intestinal contents were not analysed thus the previous results cannot be confirmed. However, to our knowledge, this is the first study investigating the effect of long-term supplementation of Arg of sow’s gestation diet for the whole pregnancy period on sows’ faecal microbiota. Arg supplementation did not significantly influence the faecal microbial structures. Indeed, no differences in the alpha and beta indices were observed. The results suggest that Arg supplementation did not affect the sows intestinal eubiosis and as a consequence, it can be assumed that it did not affect the environmental microbiome in which new-born piglets were born and raised. This aspect is of crucial importance since piglet at birth is not colonised by bacteria and the early and proper establishment of a eubiotic microbiota, which occurs through the contact with the microbiota-derived by sows and environmental, is mainly important for the establishment of stable microbial community structures (Guevarra et al. Citation2019) that can favour the development of the immune system (Stokes Citation2017; Trevisi et al. Citation2018) and growth performance of pigs later in life (Mach et al. Citation2015; Kiros et al. Citation2019).
Nevertheless, although the microbial structure was not profoundly affected, some taxa which are common in sow intestinal microbiota were influenced by the Arg supplementation. The Arg supplementation increased both the Bacteroidaceae family and the Bacteroides genus. The present observation is in accordance with the study of Wu et al. (Citation2011) in which a higher abundance of this bacterial family has been associated with a diet with high levels of animal proteins and amino acids in humans, suggesting that this family can use protein source and amino acids for its metabolism. In addition, in the present study Arg reduced several bacterial families (Succinivibrionaceae, Acidaminococcaceae, Veillonellaceae) and bacteria of the genus Succinovibrio. The reduced abundance of Succinovibrio in the ARG group could be associated with the limited use of Agr from these bacteria as suggested by Dai et al. (Citation2010) for Succinivibrio dextrinosolvens.
Conclusions
In conclusion, the supplementation of 0.25% of Arg to the sow diet for the complete duration of the pregnancy increased the sow productive performance in terms of the number of born piglets per sows and tended to reduce the IUGR piglets’ frequency and piglets’ mortality without modifying the sow intestinal microbial structure and the gut eubiosis. The results support the knowledge that Arg plays a key role in nutrition and physiology of pregnant sows.
Ethics approval
The procedures complied with Italian law pertaining to experimental animals and were approved by the Ethic-Scientific Committee for Experiments on Animals of the University of Bologna, Italy (Prot. N.106453 − 16 May 2019).
Supplemental Material
Download MS Word (17.7 KB)Acknowledgement
The authors acknowledge the support and assistance of Agricola Tre Valli Soc. Coop., Veronesi Group.
Disclosure statement
No potential conflict of interest was reported by the author(s).
Additional information
Funding
References
- Babinszky L, Halas V. 2009. Innovative swine nutrition: some present and potential applications of latest scientific findings for safe pork production. Ital J Anim Sci. 8(sup3):7–20.
- Bass BE, Bradley CL, Johnson ZB, Boyd RD, Usry JL, Maxwell CV, Frank JW. 2017. Influence of dietary l -arginine supplementation of sows during late pregnancy on piglet birth weight and sow and litter performance during lactation. J Anim Sci. 95:248–256.
- Bates D, Mächler M, Bolker B, Walker S. 2015. Fitting linear mixed-effects models using lme4. J Stat Softw. 67:1–48.
- Bazer FW, Thatcher WW, Martinat-Botte F, Terqui M. 1988. Conceptus development in Large White and prolific Chinese Meishan pigs. J Reprod Fertil. 84(1):37–42.
- Bee G. 2007. Birth weight of litters as a source of variation in postnatal growth, and carcass and meat quality. Adv Pork Prod. 18:191–196.
- Berard J, Bee G. 2010. Effect of dietary L-arginine supplementation to gilts during early gestation on foetal survival, growth and myofiber formation. Animal. 4:1680–1687.
- Callahan BJ, McMurdie PJ, Rosen MJ, Han AW, Johnson AJA, Holmes SP. 2016. DADA2: high-resolution sample inference from Illumina amplicon data. Nat Methods. 13(7):581–583.
- Dai ZL, Li XL, Xi PB, Zhang J, Wu G, Zhu WY. 2012. Regulatory role for L-arginine in the utilization of amino acids by pig small-intestinal bacteria. Amino Acids. 43(1):233–244.
- Dai ZL, Zhang J, Wu G, Zhu WY. 2010. Utilization of amino acids by bacteria from the pig small intestine. Amino Acids. 39(5):1201–1215.
- David L A, Maurice C F, Carmody R N, Gootenberg D B, Button J E, Wolfe B E, Ling A V, Devlin A S, Varma Y, Fischbach M A, et al. 2014. Diet rapidly and reproducibly alters the human gut microbiome. Nature. 505(7484):559–563. doi:10.1038/nature12820.
- Dixon P. 2003. VEGAN, a package of R functions for community ecology. J Veg Sci. 14(6):927–930.
- Easter R A, Baker D H. 1976. Nitrogen Metabolism and Reproductive Response of Gravid Swine Fed an Arginine-free Diet during the Last 84 Days of Gestation. The Journal of Nutrition. 106(5):636–641. doi:10.1093/jn/106.5.636.
- Escribano D, Gutiérrez AM, Tecles F, Cerón JJ. 2015. Changes in saliva biomarkers of stress and immunity in domestic pigs exposed to a psychosocial stressor. Res Vet Sci. 102:38–44.
- Escribano D, Gutiérrez AM, Subiela SM, Tecles F, Cerón JJ. 2012. Validation of three commercially available immunoassays for quantification of IgA, IgG, and IgM in porcine saliva samples. Res Vet Sci. 93(2):682–687.
- Flynn NE, Meininger CJ, Haynes TE, Wu G. 2002. Dossier: free amino acids in human health and pathologies – the metabolic basis of arginine nutrition and pharmacotherapy. Biomed Pharmacother. 56(9):427–438.
- Gao K, Jiang Z, Lin Y, Zheng C, Zhou G, Chen F, Yang L, Wu G. 2012. Dietary L-arginine supplementation enhances placental growth and reproductive performance in sows. Amino Acids. 42(6):2207–2214.
- Gonçalves MA, Gourley KM, Dritz SS, Tokach MD, Bello NM, DeRouchey JM, Woodworth JC, Goodband RD. 2016. Effects of amino acids and energy intake during late gestation of high-performing gilts and sows on litter and reproductive performance under commercial conditions. J Anim Sci. 94(5):1993–2003.
- Guevarra RB, Lee JH, Lee SH, Seok MJ, Kim DW, Kang BN, Johnson TJ, Isaacson RE, Kim HB. 2019. Piglet gut microbial shifts early in life: causes and effects. J Anim Sci Biotechnol. 10:1.
- Hales J, Moustsen VA, Nielsen MBF, Hansen CF. 2013. Individual physical characteristics of neonatal piglets affect preweaning survival of piglets born in a noncrated system. J Anim Sci. 91(10):4991–5003.
- Hazeleger W, Ramaekers R, Smits C, Kemp B. 2007. Effect of Progenos on placenta and fetal development in pigs. Paper presented at: 11th Annual Conference of the European Society for Domestic Animal Reproduction (ESDAR); Sep 21–22; Celle, Germany.
- Hines EA, Romoser MR, Kiefer ZE, Keating AF, Baumgard LH, Niemi J, Benjamin H, Williams NH, Kerr BJ, Touchette BJ, et al. 2019. The impact of dietary supplementation of arginine during gestation in a commercial swine herd: II. Offspring performance. J Anim Sci. 97(9):3626–3635.
- Kim SW, Wu G. 2009. Regulatory role for amino acids in mammary gland growth and milk synthesis. Amino Acids. 37(1):89–95.
- Kiros TG, Luise D, Derakhshani H, Petri R, Trevisi P, D’Inca R, Auclair E, van Kessel AG. 2019. Effect of live yeast saccharomyces cerevisiae supplementation on the performance and cecum microbial profile of suckling piglets. PLoS One. 14(7):e0219557.
- Love MI, Huber W, Anders S. 2014. Moderated estimation of fold change and dispersion for RNA-seq data with DESeq2. Genome Biol. 15(12):550.
- Luise D, Motta V, Bertocchi M, Salvarani C, Clavenzani P, Fanelli F, Pagotto U, Bosi P, Trevisi P. 2019a. Effect of Mucine 4 and Fucosyltransferase 1 genetic variants on gut homoeostasis of growing healthy pigs. J Anim Physiol Anim Nutr. 103(3):801–812.
- Luise D, Lauridsen C, Bosi P, Trevisi P. 2019b. Methodology and application of Escherichia coli F4 and F18 encoding infection models in post-weaning pigs. J Anim Sci Biotechnol. 10:53.
- Mach N, Berri M, Estellé J, Levenez F, Lemonnier G, Denis C, Leplat J-J, Chevaleyre C, Billon Y, Doré J, et al. 2015. Early-life establishment of the swine gut microbiome and impact on host phenotypes. Environmental Microbiology Reports. 7(3):554–569. doi:10.1111/1758-2229.12285.
- Massacci FR, Tofani S, Forte C, Bertocchi M, Lovito C, Orsini S, Tentellini N, Marchi L, Lemonnier G, Luise D, et al. 2020. Host genotype and amoxicillin administration affect the incidence of diarrhoea and faecal microbiota of weaned piglets during a natural multiresistant ETEC infection. J Anim Breed Genet. 137(1):60–72.
- Mateo RD, Wu G, Bazer FW, Park JC, Shinzato I, Kim SW. 2007. Dietary L-arginine supplementation enhances the reproductive performance of gilts. J Nutr. 137(3):652–656.
- Matheson, S. M., G. A. Walling, and S. A. Edwards. 2018. Genetic selection against intrauterine growth retarda- tion in piglets: a problem at the piglet level with a solu- tion at the sow level. Genet. Sel. E 50:46. doi:10.1186/s12711-
- Matsumoto M, Kitada Y, Naito Y. 2019. Endothelial Function is improved by Inducing Microbial Polyamine Production in the Gut: A Randomized Placebo-Controlled Trial. Nutrients. 11(5):1188. doi:10.3390/nu11051188.
- McMurdie PJ, Holmes S. 2013. phyloseq: An R package for reproducible interactive analysis and graphics of microbiome census data. PloS One. 8(4):e61217.
- Nuntapaitoon M, Muns R, Theil PK, Tummaruk P. 2018. L-arginine supplementation in sow diet during late gestation decrease stillborn piglet, increase piglet birth weight and increase immunoglobulin G concentration in colostrum. Theriogenology. 121:27–34.
- NRC 2012. Nutrient requirements of swine. 11th rev ed. Washington (DC): The National Academies Press.
- Quan J, Cai G, Ye J, Yang M, Ding R, Wang X, Zheng E, Fu D, Li S, Zhou S, et al. 2018. A global comparison of the microbiome compositions of three gut locations in commercial pigs with extreme feed conversion ratios. Sci Rep. 8(1):4536.
- Quast C, Pruesse E, Yilmaz P, Gerken J, Schweer T, Yarza P, Peplies J, Glöckner FO. 2012. The SILVA ribosomal RNA gene database project: improved data processing and web-based tools. Nucleic Acids Res. 41(D1):D590–D596.
- Palencia JYP, Lemes MAG, Garbossa CAP, Abreu MLT, Pereira LJ, Zangeronimo MG. 2018. Arginine for gestating sows and foetal development: a systematic review. J Anim Physiol Anim Nutr. 102(1):204–213.
- Ren W, Duan J, Yin J, Liu G, Cao Z, Xiong X, Chen S, Li T, Yin Y, Hou Y, et al. 2014. Dietary l‑glutamine supplementation modulates microbial community and activates innate immunity in the mouse intestine. Amino Acid. 46(10):2403–2413.
- Schokker D, Zhang J, Zhang L-l, Vastenhouw S A, Heilig H G H J, Smidt H, Rebel J M J, Smits M A. 2014. Early-Life Environmental Variation Affects Intestinal Microbiota and Immune Development in New-Born Piglets. PLoS One. 9(6):e100040. doi:10.1371/journal.pone.0100040.
- Stokes CR. 2017. The development and role of microbial-host interactions in gut mucosal immune development. J Anim Sci Biotechnol. 8:12.
- Theil PK, Hurley WL. 2016. The protein component of sow colostrum and milk, milk proteins - from structure to biological properties and health aspects. In: I. Gigli, editor. Milk proteins—from structure to biological properties and health aspects. Rijeka (Croatia): InTech; p. 183–195.
- Trevisi P, Priori D, Jansman AJM, Luise D, Koopmans SJ, Hynönen U, Palva A, van der Meulen J, Bosi P. 2018. Molecular networks affected by neonatal microbial colonization in porcine jejunum, luminally perfused with enterotoxigenic Escherichia coli, F4ac fimbria or Lactobacillus amylovorus. PLoS One. 13(8):e0202160
- Wu G, Bazer FW, Cudd TA, Meininger CJ, Spencer TE. 2004. Maternal nutrition and fetal development. J Nutr. 134(9):2169–2172.
- Wu G, Baze FW, Johnson GA, Herring C, Seo H, Dai Z, Wang J, Wu Z, Wang X. 2017. Functional amino acids in the development of the pig placenta. Mol Reprod Dev. 84(9):870–882.
- Wu G, Bazer FW, Wallace JM, Spencer TE. 2006. Intrauterine growth retardation: implications for the animal sciences. J Anim Sci. 84(9):2316–2337.
- Wu GD, Chen J, Hoffmann C, Bittinger K, Chen YY, Keilbaugh SA, Bewtra M, Knights D, Walters WA, Knight R, et al. 2011. Linking long-term dietary patterns with gut microbial enterotypes. Science. 334(6052):105–108.
- Wu G, Knabe DA, Kim SW. 2004. Arginine nutrition in neonatal pigs. J Nutr. 134(10):2783S–2790S.
- Yuan TL, Zhu YH, Shi M, Li TT, Li N, Wu GY, Bazer FW, Zang JJ, Wang FL, Wang JJ. 2015. Within-litter variation in birth weight: impact of nutritional status in the sow. J Zhejiang Univ Sci B. 16(6):417–435.
- Xiao L, Estellé J, Kiilerich P, Ramayo-Caldas Y, Xia Z, Feng Q, Liang S, Pedersen AØ, Kjeldsen NJ, Liu C, et al. 2016. A reference gene catalogue of the pig gut microbiome. Nat Microbiol. 1(12):16161.
- Yang H, Huang X, Fang S, He M, Zhao Y, Wu Z, Yang M, Zhang Z, C AND Huang L. 2017. Unraveling the Fecal Microbiota and Metagenomic Functional Capacity Associated with Feed Efficiency in Pigs. Front. Microbiol. 8(1555). doi:10.3389/fmicb.2017.01555Gut.