Abstract
To prepare broad-spectrum and specific monoclonal antibodies (mAb) against deoxynivalenol (DON), DON-BSA was synthesised by the carbonyl diimidazole method as an artificial antigen to immunise Balb/C mice, and the coated DON-OVA was synthesised by the carbodiimide method to detect anti-DON antibodies. Monoclonal antibodies were screened by indirect ELISA and indirect competitive ELISA. A hybridoma cell line (4F3) capable of stably secreting DON antibody was obtained. The titre of antibody in the culture supernatant was 1:1.28 × 103, and the titre of the ascites antibody was 1:3.2 × 105. The monoclonal antibody homology was IgG1/λ. The half inhibitory concentration (IC50) of DON was 9.84 ng/mL. The cross-reaction rates with 3-Ac-DON and 15-Ac-DON were 60.44% and 52.04%, respectively, but no cross-reaction with other mycotoxins was observed. The results showed that the anti-DON monoclonal antibodies prepared in this experiment could recognise not only DON but also 3-Ac-DON and 15-Ac-DON, which could provide materials for the next step to establish a method to detect DON and its similar compounds.
This study demonstrated that an artificial antigen was synthesised by carbonyl diimidazole method, and a novel broad-spectrum, high affinity monoclonal antibody was developed.
The anti-DON mAb developed in this study has better sensitivity, moreover the antibody was sensitive to DON and its derivatives. Therefore, it could be used for simultaneous monitoring of three mycotoxins.
Results of this study will further lay the foundation for the establishment of immunological assays for the total amount of similar compounds.
Highlights
Introduction
Vomitoxin, also known as deoxynivalenol (DON), is a mycotoxin and is mainly produced by Fusarium graminearum and Fusarium culmorum (Zuo et al. Citation2018). A vomit-inducing toxin isolated from corn contaminated by Fusarium graminearum in 1970 was called vomiting toxin (Abouzied et al. Citation1993). Its chemical name is 3a, 7a, 15-trihydroxyfusarium-9-ene-8-one, and the toxin belongs to the B-group of trichothecenes (Jiang et al. Citation2018; Kong et al. Citation2019). The structure of the toxin is tetracyclic sesquiterpene, its molecular formula is C15H20O6, and its molecular weight is 296.32 (Pestka and Smolinski Citation2005). Its structure is presented in Figure .
In recent years, vomitoxin has caused a high rate of food contamination in the world, so it has become a hot research topic in veterinary and medical fields (Goncalves and Stroka Citation2016). Vomiting toxins are widely found in cereal crops such as wheat, barley and maize (Ennouari et al. Citation2013). Therefore, the symptoms of human and animal poisoning diseases caused by food intake include anorexia, vomiting, diarrhoea, fever, growth retardation, cardiotoxicity and teratogenicity; these symptoms are closely related to immune suppression, Keshan disease, oesophageal cancer and other diseases, which seriously threaten human and animal health (Qiu et al. Citation2015; Kim et al. Citation2016; Freire and Sant'Ana Citation2018; Tima et al. Citation2018). Vomiting toxins were classified as class III carcinogens in the evaluation report published by the International Agency for Research on Cancer in 1998 (Rai et al. Citation2015). In addition, the derivatives of DON, 3-Ac-DON and 15-Ac-DON have equal or even more toxicity than DON (Wu and Wang Citation2015). Therefore, a standard maximum limit of total DON and its derivatives in cereals has been set at home and abroad (Belluco et al. Citation2017).
At present, strengthening the synchronous detection of vomitin and its derivatives in cereals is a key measure to ensuring the health of animals and human beings. The commonly used methods for DON detection include TLC, high-performance liquid chromatography (HPLC), gas chromatography (GC), mass spectrometry (MS), gas chromatography-mass spectrometry (GC-MS), high-performance liquid chromatography-tandem mass spectrometry (HPLC-MS/MS), etc (Almeida et al. Citation2016; Vidal et al. Citation2017). In addition, some rapid screening methods have been reported, such as ELISA (Li et al. Citation2015), sidestream immunoassay (Anfossi et al. Citation2013), Quenchbody (Yoshinari et al. Citation2015), and surface plasmon resonance immunochromatography (Kadota et al. Citation2010). However, at present, the methods for simultaneous determination of DON and its derivatives are GC-MS and HPLC-MS/MS, since GC-MS assays require a complex derivation process before analysis. Therefore, HPLC-MS/MS has become the main analytical instrument used for the simultaneous determination of DON, 3-AC-DON and 15-AC-DON (Trombete et al. Citation2016). But it has some shortcomings, HPLC-MS/MS requires expensive equipment, a complex sample pre-treatment, and skilled technicians and there are a limited number of laboratories capable of the required operations. Therefore, it is necessary to develop a simple, rapid and effective method for the determination of DON, 3-AC-DON and 15-AC-DON in cereals. The aim of this experiment was to screen anti-DON monoclonal antibodies that may recognise DON, 3-AC-DON and 15-AC-DON and to lay a foundation for the establishment of immunological assays to quantify the total amount of similar compounds.
Materials and methods
Reagents and materials
DON (purity ≥ 98.00%), 3-Ac-DON, 15-Ac-DON, Nivalenol (NIV), Fusarenon-X, T-2 toxin, Zearalenone (ZEN), Aflatoxin B1 (AFB1) and other standards were purchased from Sigma-Aldrich Co., Ltd. (Augsburg, Germany). Bovine serum albumin (BSA, 96%), chicken ovalbumin (OVA, 98%), N,N′-carbonyldiimidazole (CDI, 98%), 1-ethyl-3-(3-dimethylamino)propyl) carbodiimide hydrochloride (EDC, 98%), Freund’s complete adjuvant (FCA), Freund’s incomplete adjuvant (FIA) and monoclonal antibody isotype kit (mAb isotyping kit) were purchased from Pierce company. PEG-1500 (polyethylene glycol) was purchased from Roche. RPMI-1640 cell culture medium, HAT (50×) medium, HT (50×) medium, 8-AG (8-azaguanine) medium, colchicine and phenol red (Phenol red) were purchased from Beijing Solaire. Foetal bovine serum (FBS) was purchased from Gibco. Female Balb/c mice (6-8 weeks old) were provided by Beijing SPF Biotech Co., Ltd. and were reared in our laboratory animal house.
Equipment and instruments
A Galaxy S-type CO2 cell incubator was purchased from Biotech. A Multiskan MK3 microplate reader was purchased from Thermo. An inverted MIC00949 microscope was purchased from Nikon Corporation. In addition, 96-well microtiter plates as well as 24-well and 96-well cell culture plates were purchased from Iwaki Co., Ltd. A LDZX-30KB vertical pressure steam steriliser was purchased from Shanghai Shen’an Medical Instrument Factory.
Synthesis of the artificial antigen
According to the molecular structure of DON, the artificial antigen DON-BSA was synthesised by the carbonyl diimidazole (CDI) method with reference to the method of Maragos and Mccormick (Citation2000). First, a chemical modification was carried out to introduce a reactive group carbonyl group (–C = O–) into a hapten by reacting DON with N,N′-carbonyldiimidazole (CDI). The introduced –C = O– was coupled with the -NH2 of the carrier protein BSA in the form of a monoamide bond (–CONH–) to synthesise a complete antigen. Specifically: 5 mg of DON standard was dissolved in 1 mL of anhydrous tetrahydrofuran (THF), was added 60 mg of CDI and reacted at 70 °C for 4 h, then the reaction product was evaporated to dry, which was hapten. The hapten product was added 500 μL N,N-dimethylformamide (DMF) to make it completely dissolve. 20 mg BSA was dissolved in 1 mL PBS, and quickly mixed with the hapten solution. The artificial antigen DON-BSA was prepared by stirring in dark for 24 h at room temperature and dialysised for 3 days, −20 °C for storage. The synthetic route was shown in Figure . SDS-polyacrylamide gel electrophoresis (SDS-PAGE)、ultraviolet (UV) and infra-red (IR) scanning spectroscopy was used to confirm the success of artificial antigen coupling.
Figure 2. The synthetic route of deoxynivalenol artificial antigen. Note: CDI: N,N'-carbonyldiimidazole; BSA: bovine serum albumin.
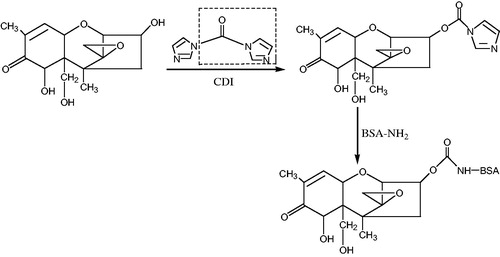
The synthesis of the coating DON-OVA was improved by referring to the method of Li et al (Li et al. Citation2012). The DON molecule was first derivatized with maleic anhydride, and the hapten was synthesised by introducing the active group (–COOH). Then, the hapten was combined with OVA couple to the coating DON-OVA by the carbodiimide (EDC) method.
Establishment of the DON mAb hybridoma cell line
Five 6-week-old female Balb/C mice were immunised with the artificial antigen DON-BSA. The immunisation method used a low dose and long time intervals. The injection point was 4 points subcutaneously on the back. The immunisation dose was 30 μg/head. A total of 1 mL of immunogen was prepared. The artificial antigen DON-BSA was first immunised with mixed emulsification of FCA and following replaced with FIA. Five immunisation times were performed. The interval between the first immunisation and the second immunisation was 4 weeks, followed by several immunisation intervals of 3 weeks, and 10 days after the last immunisation, DON pAb was isolated from tail-amputated blood. Then, the indirect ELISA method was used to detect the serum titre of the polyclonal antibody. An indirect competitive ELISA assay was used to detect the half-inhibitory concentration (IC50) of DON. Mice with the titre >1:1600, the lowest IC50 and the best specificity were selected for cell fusion.
Cell fusion and screening of positive hybridoma cell lines was performed according to routine operation methods (Burmistrova et al. Citation2009). The karyotype analysis of hybridomas was performed using the colchicine blocking method (Li et al. Citation2011). The stability analysis used indirect ELISA and indirect competition ELISA to test the antibody titre and sensitivity in the supernatant that was obtained from the selected hybridoma cells after repeated freezing and thawing; these assays were also used to compare the changes of the antibodies according to the detection results.
Preparation and characterisation of DON mAb
This experiment adopted the in vivo-induced ascites method to mass produce DON mAb (Tang et al. Citation2011). The collected ascites were purified by caprylic/ammonium sulphate precipitation (Kuang et al. Citation2013), and their immunological characteristics were analysed. The identification of homotype was conducted as follows: the operation was carried out according to the kit instructions. The titre determination was conducted as follows: indirect ELISA was used. The sensitivity determination was conducted as follows: indirect competitive ELISA was used to determine the inhibition rate of the ascites antibodies of the monoclonal cell lines to different concentrations of DON. Then, the standard inhibition curve was drawn. The longitudinal coordinate was the inhibition rate B/B0 (the ratio value of absorbance with a series of concentrations of DON standards and in the absence of DON), and the abscissa was the common logarithm of the different mass concentrations of DON, allowing the calculation of the IC50. The affinity determination was conducted as follows: referring to the Batty saturation method described by Beatty et al. (Citation1987) the affinity constant (Ka) was also determined by the indirect ELISA method, BSA-DON was coated plates with concentrations of 2 μg/mL and 4 μg/mL respectively, 30 μg/mL of anti-DON mAb was diluted in multiple ratio, then the absorbance was measured at the wavelength of 450 nm. The results were calculated according to the formula: Ka = (n − 1)/2(n[Ab’]t − [Ab]t). In the formula, n = [Ag]t/[Ag’]t. [Ag]t and [Ag’]t represent different concentrations of coating (2、4 μg/mL), [Ab]t and [Ab’]t represent the corresponding half of the absorption value of corresponding DON mAb concentration when the coating with different concentrations. The specificity identification was conducted as follows: according to the cross-reactions, the structural analogues of DON were selected as inhibitors and determined the IC50 of an-DON mAb to each inhibitor by indirect competitive ELISA. The percentage of IC50 of an-DON mAb to DON and IC50 of an-DON mAb to each inhibitor was taken as the cross reaction rate, and the cross reaction rate was calculated by the following formula: CR(%) = [IC50 (DON)/IC50 (structural analogue)] × 100% (Sakamoto et al. Citation2017).
Results
Identification of physicochemical properties of artificial antigens
SDS-PAGE identification
The molecular weights of BSA and DON were approximately 66.4 KD, 0.296 KD. The SDS-PAGE electrophoresis identification is shown in Figure . The protein band of artificial antigen DON-BSA was slightly higher than that of BSA, indicating that the DON-BSA migration is slower than the BSA migration. The results showed that the molecular weight of DON-BSA was higher than that of BSA. Therefore, it was initially proved that the conjugation of artificial antigen was successful.
UV identification
The results are shown in Figure . The maximum absorption peak of BSA was 276 nm, the characteristic peak of DON was 213 nm, and the maximum absorption peak of artificial antigen DON-BSA was 275 nm. The shift of absorption peak proved that the conjugation of artificial antigen was successful. The molecule binding ratio of DON to BSA was calculated to be 12:1 according to the conjugation rate of artificial antigen.
IR identification
The results are shown in Figure . Compared DON-BSA with BSA had similar IR absorption in the regions of 2500–3200 cm−1 and 1660–1500 cm−1, which was the characteristic peak of amino acid produced by carrier protein BSA, indicating the presence of BSA in artificial antigen DON-BSA. Compared DON-BSA with DON had similar absorption in the region of 1050–1150 cm−1, and BSA had no absorption here. This was the characteristic peak of alcohol hydroxyl in DON, indicating that DON-BSA contains DON. Therefore, the synthesis of artificial antigen DON-BSA could be confirmed successfully.
Cell fusion mouse pAb titre and sensitivity determination results
The pAb titres of all five mice reached 1: (1.6 × 103), the titre of M4 was as high as 1: (6.4 × 103), and the sensitivity of M4 was also the highest. The regression equation of the inhibition curve was y = −37.056x + 112.23, R2 was 0.9744, and the lowest IC50 was 47.75 ng/mL. Therefore, M4 could be selected for cell fusion. See Figure and .
Screening and identification of hybridoma cell lines
Screening of hybridoma cell lines
On the 7th day after fusion, the effect of cell fusion was observed. A total of 236 cloned hybridoma cells were observed in 384 holes of the 96-well cell culture plates. The effective fusion rate was 61.5%. The titre was detected by indirect ELISA. There were 52 positive wells, with a positive rate of 22%. Blocking ELISA was used to inhibit titre, and then three subclones were used to select the inhibiting wells. Finally, a hybridoma cell named 4F3 was screened out.
Identification of hybridoma cell lines
Karyotype identification results
Karyotype identification was carried out by the colchicine blocking method. The chromosomes of the cell line obtained in this experiment were 99.5, while the chromosomes of mouse spleen cells were 40, and the chromosomes of myeloma NS0 cells were 62–68. Thus, the number was larger than that of the two parents, indicating that the hybridoma cells, after fusion of the two parents, were hybrids.
Analysis results of stability
The selected hybridoma cells were passed to the 8th generation, and the titre of the supernatant of each generation was essentially the same as that of the mother generation. After repeated freeze-thaw cultures, the titre and sensitivity of the supernatant were tested, and no significant changes were found. The results showed that the mAb-secreting hybridoma cells had good genetic stability. See Figure .
Analysis of immunological characteristics of DON mAb
Homotype identification
The mouse mAb homology kit was used for identification. The antibody homology produced by the positive cell line 4F3 was IgG1/λ.
Determination of potency
The culture supernatants and ascites of the 4F3 hybridoma cell lines had good antibody titres. The supernatant titre reached 1: (1.28 × 103), and the ascites titre reached 1: (3.2 × 105).
Sensitivity determination
The curve of the anti-DON mAb blocking results secreted by the 4F3 hybridoma cell line is shown in Figure . The curve regression equation is y = −35.661x + 85.429, the R2 value is 0.9809, and the IC50 is 9.84 ng/mL, indicating that there was good sensitivity.
Affinity determination
According to the formula, the Ka value of the 4F3 cell line was 1.51 × 109L/mol. Therefore, the DON mAb prepared in this experiment was a high-affinity antibody. The results are shown in Figure .
Specific identification
The results are shown in Table . The IC50 of the 4F3 strain mAb to DON was 9.84 ng/mL, and the cross-reaction rate was 100%. The IC50 of the 4F3 strain mAb to 3-Ac-DON and 15-Ac-DON were 16.28 ng/mL and 18.91 ng/mL, respectively. The cross-reaction rates were 60.44% and 52.04%, respectively. The cross-reaction rate of 4F3 mAb with other DON structural analogues was less than 5%. Therefore, this monoclonal antibody could recognise DON, 3-Ac-DON and 15-Ac-DON.
Table 1. The percent cross-reactivity of DON mAb with other structural complexes.
Discussion
Synthesis of artificial antigen
Due to the particularity of the molecular structure of DON, there are hydroxyl groups at the C3, C7 and C15 sites and keto groups at the C8 sites. Theoretically, four sites can be introduced into the bridge structure, but due to the influence of the ketone group on C8, the hydroxyl group on C7 is inactive, and it is generally difficult to undergo a derivatization reaction. In most of the literature reporting the preparation of DON antibodies, most of them selected sites for the synthesis of the DON antigen, and one of the hydroxyl groups was selectively used to couple the proteins. For example, the specificity of antibodies used to immunise mice with the C8 ketone group activated by Casale et al. (Citation1988) was not good. However, after immunising mice with artificial antigens prepared by activating the hydroxyl groups on C3, a hybridoma cell line stably secreting anti-DON monoclonal antibody was obtained, and immunological detection methods were established. Some studies, such as Li et al. (Citation2012) reported that better artificial antigens were obtained by blocking the hydroxyl groups at the 7th and 15th positions and then deriving the hydroxyl groups at position 3. However, the preparation process of these methods was complicated, and the operation was tedious. Therefore, in the complete antigen coupling, we tried a variety of methods to prepare antigens and immunise animals and used the comparison results to screen out the best complete antigen. In this study, a variety of coupling methods were evaluated by comparative experiments. The results showed that the improved CDI method of Maragos and Mccormick (Citation2000) had the best efficacy and that the three hydroxyl groups on the DON molecule might react. Therefore, in this experiment, the C3 and C15 hydroxyl groups on the DON molecule were successfully, covalently coupled with amino groups on the carrier protein BSA by the CDI activation method to obtain the immunogen DON-BSA. Without blocking the hydroxyl group at any position, the imidazole group was directly introduced to realise coupling with the carrier protein BSA. The artificial antigen synthesised by this method had no obvious difference from the artificial antigen synthesised by Lee et al. (Citation2013) reported in the previous literature. The immunisation effect of mice was better, but the steps were simple and convenient for operation, so the application cost could later be reduced. At the same time, we used the EDC method to synthesise the coated proton. The DON compound was directly derivatized from maleic anhydride without blocking, and a better coating agent for detecting anti-DON antibody was obtained. Then, we identified the DON complete antigen by SDS-PAGE, ultraviolet (UV) and infra-red (IR) scanning spectra, and preliminarily proved the success of artificial antigen coupling. By immunising mice with artificial antigens and ELISA analysis with a mouse polyclonal antibody, it was observed that there was a higher titre and that the DON molecule could inhibit the polyclonal antibody in mice, which proved that the artificial antigen synthesis was successful.
Heterogeneity detection
Because the molecular weight of DON is small, it only has reactivity but has no immunogenicity. DON cannot directly stimulate the animal body to produce corresponding antibodies. Instead, DON must be coupled with carrier proteins with larger molecular weights. In this experiment, two different methods, the carbonyl diimidazole (CDI) method and the carbodiimide (EDC) method, were used to prepare artificial antigens and coated antigens, respectively. The heterologous detection mode was established, which not only reduced the chance of false positives but also solved the influence of bridge antibodies on hybridoma cell screening. This is an effective method for hybridoma cell screening (Guo et al. Citation2014). So far, the application of heterologous ELISA in hybridoma screening is rare. In this study, we explored the applicability of this method for hybridoma cell screening. The results showed that the monoclonal antibodies screened by heterologous detection were broad-spectrum and efficient against DON and its analogues, and differed from other previous antibodies. Therefore, eliminating the influence of bridging antibodies and establishing a reasonable screening method will lay a solid foundation for screening for bridging-free antibody, high-titre and high-affinity hybridoma cell lines.
Immunological characteristics of DON monoclonal antibodies
The binding capacity, affinity and selectivity of the antigen-antibody reaction are the main manifestations of the immunological characteristics of the antibody. In this experiment, indirect ELISA was used to determine the pAb titres of 5 mice which reached 1: (1.6 × 103), and the highest titre of M4 was 1: (6.4 × 103). The affinity of antibodies is often measured by the size of the affinity constant (Ka). The affinity constant is very important for the quality of the antibody, which determines the binding ability between the antibody and the hapten or antigenic determinants. The affinity constant is an important index of antibody stability. The Ka of DON mAb determined by the Batty saturation method is 1.51 × 109 L/mol . James (1986) showed that the affinity of the antibody was high when the Ka value was 107 to 1012 L/mol, which indicates that a DON mAb with a high affinity has been obtained. Selectivity is determined by the specificity of the antibody. The specificity of the antibody was identified by a cross-reaction test in this assay. The results showed that the cross-reactivity of the derivatives 3-Ac-DON and 15-Ac-DON were 60.44% and 52.04%, respectively, because they were closer to DON in spatial structure. Generally, for DON monoclonal antibodies, depending on the binding sites (3 and 15) of immune antigens, there was a high cross-reaction to acetylated analogues at the conjugation sites (Xu et al. Citation2010). The other two derivatives, NIV and Fusarenon-X, differed greatly from DON in spatial structure, so the cross-reaction rate was very low. In addition, the IC50 of anti-DON mAb is 9.84 ng/ml, compared with the IC50 of anti-DON mAb developed by Li et al. (Citation2018), which is 25.2 ng/ml, there was better sensitivity. Therefore, the antibody produced by immunising mice with artificial antigen DON-BSA is sensitive to DON, 3-Ac-DON and 15-Ac-DON, which indicates that the antibody can recognise not only DON but also 3-Ac-DON and 15-Ac-DON. Therefore, a monoclonal antibody that can simultaneously detect DON and its similar compounds (DON, 3-Ac-DON, 15-Ac-DON, etc.) was prepared, which has laid the foundation for the establishment of detection methods for DON and similar compounds.
Conclusions
In this study, DON-BSA was synthesised by the carbonyl diimidazole (CDI) method as an artificial antigen to immunise mice. The coated DON-OVA was synthesised by the carbodiimide (EDC) method to detect the anti-DON antibody. This heterologous detection technique was also used to screen hybridoma cells. A novel broad-spectrum and high affinity monoclonal antibody, 4F3, against DON was produced. The monoclonal antibody was sensitive to DON, 3-Ac-DON and 15-Ac-DON. So far, this is the first broad-spectrum, high-affinity monoclonal antibody against DON and its two derivatives. This antibody can be used for simultaneous monitoring of three mycotoxins. Therefore, this antibody can be further applied to different kinds of DON-related immunoassays and lay a foundation for the establishment of immunological assays for the total amount of similar compounds.
Ethical approval and animal rights
The studies with animals were performed following all institutional and national guidelines for the care and use of laboratory animals.
Author contributions
Ziliang Wang and Wenju Zhang designed the research and interpreted the results. Li Han and Yuetao Li conducted experiments and drafted the manuscript. Zhuang Lei and Haojie Wang collected and Processed data. Jinqing Jiang, Renfeng Li and Guoying Fan revised the manuscript.
Disclosure statement
There are no conflicts to declare.
Additional information
Funding
References
- Abouzied MM, Azcona-Olivera JI, Yoshizawa T, Pestka JJ. 1993. Production of polyclonal antibodies to the trichothecene mycotoxin 4,15- diacetylnivalenol with the carrier-adjuvant cholera toxin. Appl Environ Microbiol. 59(5):1264–1268.
- Almeida APD, Lamardo LCA, Shundo L, Silva SAD, Navas SA, Alaburda J, Ruvieri V, Sabino M. 2016. Occurrence of deoxynivalenol in wheat flour, instant noodle and biscuits commercialised in brazil. Food Addit Contam Part B Surveill. 9(4):251–255.
- Anfossi L, Baggiani C, Giovannoli C, D’Arco G, Giraudi G. 2013. Lateral-flow immunoassays for mycotoxins and phycotoxins: a review. Anal Bioanal Chem. 405(2–3):467–480.
- Beatty JD, Beatty BG, Vlahos WG. 1987. Measurement of monoclonal antibody affinity by non-competitive enzyme immunoassay. J Immunol Methods. 100(1–2):173–179.
- Belluco B, de Camargo AC, da Gloria EM, Dias C. T d S, Button DC, Calori-Domingues MA. 2017. Deoxynivalenol in wheat milling fractions: A critical evaluation regarding ongoing and new legislation limits. J Cereal Sci. 77:284–290.
- Burmistrova NA, Goryacheva IY, Basova EY, Franki A-S, Elewaut D, Van Beneden K, Deforce D, Van Peteghem C, De Saeger S. 2009. Application of a new anti-zearalenone monoclonal antibody in different immunoassay formats. Anal Bioanal Chem. 395(5):1301–1307.
- Casale WL, Pestka JJ, Hart LP. 1988. Enzyme-linked immunosorbent assay employing monoclonal antibody specific for deoxynivalenol (vomitoxin) and several analogues. J Agric Food Chem. 36(3):663–668.
- Ennouari A, Sanchis V, Marín S, Rahouti M, Zinedine A. 2013. Occurrence of deoxynivalenol in durum wheat from morocco. Food Control. 32(1):115–118.
- Freire L, Sant'Ana AS. 2018. Modified mycotoxins: an updated review on their formation, detection, occurrence, and toxic effects. Food Chem Toxicol. 111:189–205.
- Goncalves C, Stroka J. 2016. Cross-reactivity features of deoxynivalenol (don)-targeted immunoaffinity columns aiming to achieve simultaneous analysis of don and major conjugates in cereal samples. Food Addit Contam A. 33(6):1053–1062.
- Guo Y, Sanders M, Galvita A, Heyerick A, Deforce D, Bracke M, Eremin S, De Saeger S. 2014. Heterologous screening of hybridomas for the development of broad-specific monoclonal antibodies against deoxynivalenol and its analogues. World Mycotoxin J. 7(3):257–265.
- James WG. 1986. Monoclonal antibodies: principles and practice. London: Academic Inc Ltd.
- Jiang D, Chen J, Li F, Li W, Yu L, Zheng F, Wang X. 2018. Deoxynivalenol and its acetyl derivatives in bread and biscuits in Shandong province of China. Food Addit Contam Part B Surveill. 11(1):43–48.
- Kadota T, Takezawa Y, Hirano S, Tajima O, Maragos CM, Nakajima T, Tanaka T, Kamata Y, Sugita-Konishi Y. 2010. Rapid detection of nivalenol and deoxynivalenol in wheat using surface plasmon resonance immunoassay. Anal Chim Acta. 673(2):173–178.
- Kim D-H, Hong S-Y, Jeon M-H, An J-M, Kim S-Y, Kim H-Y, Yoon BR, Chung SH. 2016. Simultaneous determination of the levels of deoxynivalenol, 3-acetyldeoxynivalenol, and nivalenol in grain and feed samples from south korea using a high-performance liquid chromatography-photodiode array detector. Appl Biol Chem. 59(6):881–887.
- Kong DZ, Wu XL, Li Y, Liu LQ, Song SSh, Zheng QK, Kuang H, Xu CL. 2019. Ultrasensitive and eco-friendly immunoassays based monoclonal antibody for detection of deoxynivalenol in cereal and feed samples. Food Chem. 270:130–137.
- Kuang H, Xing C, Hao C, Liu L, Wang L, Xu C. 2013. Rapid and highly sensitive detection of lead ions in drinking water based on a strip immunosensor. Sensors. 13(4):4214–4224.
- Lee H-M, Song S-O, Cha S-H, Wee S-B, Bischoff K, Park S-W, Son S-W, Kang H-G, Cho M-H. 2013. Development of a monoclonal antibody against deoxynivalenol for magnetic nanoparticle-based extraction and an enzyme-linked immunosorbent assay. J Vet Sci. 14(2):143.
- Li M, Sun MN, Hong X, Duan JSh, Du DL. 2018. Survey of deoxynivalenol contamination in agricultural products in the Chinese market using an ELISA kit. Toxins. 11(1):6–15.
- Li PS, Zhang CY, Li SJ, Chen Y, Xiang MJ, Li FH, Zhang H. 2011. Preparation of monoclonal antibody against neisseria meningococcal serogroup a polysaccharide (GAMP) by using the conjugate vaccine of both GAMP and tetanus toxoid. Herald Med. 30(6):709–712.
- Li Y, Liu G, Fu X, He J, Wang Z, Hou J, Cao X, Shi W, Zhang S. 2015. High-sensitive chemiluminescent ELISA method investigation for the determination of deoxynivalenol in rice. Food Anal Methods. 8(3):656–660.
- Li Y, Shi W, Shen J, Zhang S, Cheng L, Wang Z. 2012. Development of a rapid competitive indirect ELISA procedure for the determination of deoxynivalenol in cereals. Food Agr Immunol. 23(1):41–49.
- Maragos CM, Mccormick SP. 2000. Monoclonal antibodies for the mycotoxins deoxynivalenol and 3-Acetyl-deoxynivalenol. Food Agr Immunol. 12(3):181–192.
- Pestka JJ, Smolinski AT. 2005. Deoxynivalenol: toxicology and potential effects on humans. J Toxicol Environ Health B. 8(1):39–69.
- Qiu Y-L, He Q-H, Xu Y, Bhunia AK, Tu Z, Chen B, Liu Y-Y. 2015. Deoxynivalenol-mimic nanobody isolated from a naïve phage display nanobody library and its application in immunoassay. Anal Chim Acta. 887:201–208.
- Rai M, Jogee PS, Ingle AP. 2015. Emerging nanotechnology for detection of mycotoxins in food and feed. Int J Food Sci Nutr. 66(4):363–370.
- Sakamoto S, Nagamitsu R, Yusakul G, Miyamoto T, Tanaka H, Morimoto S. 2017. Ultrasensitive immunoassay for monocrotaline using monoclonal antibody produced by N, N′ -carbonyldiimidazole mediated hapten-carrier protein conjugates. Talanta. 168:67–72.
- Tang JJ, Li XB, Liu GW, Kong T, Li DN, Zhang L, Zh W. 2011. Production of two anti-cadmium monoclonal antibodies by hock immunization. Chin Vet Sci. 41(9):936–940.
- Tima H, Berkics A, Hannig Z, Ittzés A, Kecskésné Nagy E, Mohácsi-Farkas C, Kiskó G. 2018. Deoxynivalenol in wheat, maize, wheat flour and pasta: surveys in hungary in 2008–2015. Food Addit Contam Part B Surveill. 11(1):37–42.
- Trombete F, Barros A, Vieira M, Saldanha T, Venancio A, Fraga M. 2016. Simultaneous determination of deoxynivalenol, deoxynivalenol-3-Glucoside and nivalenol in wheat grains by HPLC-PDA with immunoaffinity column cleanup. Food Anal Methods. 9(9):2579–2586.
- Vidal JC, Bertolín JR, Ezquerra A, Hernández S, Castillo JR. 2017. Rapid simultaneous extraction and magnetic particle-based enzyme immunoassay for the parallel determination of ochratoxin A, fumonisin B1 and deoxynivalenol mycotoxins in cereal samples. Anal Methods. 9(24):3602–3611.
- Wu L, Wang B. 2015. Evaluation on levels and conversion profiles of DON, 3-ADON, and 15-ADON during bread making process. Food Chem. 185:509–516.
- Xu Y, Huang ZB, He QH, Deng SZ, Li LS, Li YP. 2010. Development of an immunochromatographic strip test for the rapid detection of deoxynivalenol in wheat and maize. Food Chem. 119(2):834–839.
- Yoshinari T, Ohashi H, Abe R, Kaigome R, Ohkawa H, Sugita-Konishi Y. 2015. Development of a rapid method for the quantitative determination of deoxynivalenol using quenchbody. Anal Chim Acta. 888:126–130.
- Zuo HG, Zhu JX, Shi L, Zhan CR, Guo P, Wang Y, Zhang Y, Liu J. 2018. Development of a novel immunoaffinity column for the determination of deoxynivalenol and its acetylated derivatives in cereals. Food Anal Methods. 11(8):2252–2260.