Abstract
Limousine and Charolais cattle breeds are assuming increasing importance in the Italian beef cattle sector. Indeed, both breeds are involved in a national rural development project. The success of a breeding programme depends heavily not only on the defined breeding objective but also on the selected criteria used to attain it. Data from the Charolais and Limousine Italian Association (born between 1960 and 2017) were used to estimate both genetic parameters and trends for calving interval (CI) and age at first calving (AFC) and genetic diversity. Population structures were investigated through probabilities of gene origin approach whereas variance components estimation was used to obtain breeding values of reproductive parameters. Heritabilities for AFC and CI were 0.24 and 0.03 for Limousine and 0.32 and 0.02 for Charolais, respectively. An increasing genetic trend was observed for AFC. For CI, no significant gains of genetic origin have been verified. In both breeds, pedigree completeness was moderate, and the observed inbreeding coefficient was low. No herd is classified as a nucleus or isolated and they all use external bulls. Heritability for AFC in both breeds suggests possible improvements when selected. Limited improvements are expected for CI by means of a traditional genetic breeding scheme. A reduction in the generation interval might represent a cost-effective solution to increase annual genetic gain. Pedigree analysis suggests population bottleneck in both breeds.
Limousine and Charolais breeds are involved in the national project for the genetic improvement of cattle populations raised in Italy.
Genetic indexes will be developed following a genomic approach. This study is preparatory for the implementation of a selective programme of this type.
The breeds presented a reduced number of animals in the genetic formation. High ‘bottleneck effect’. Moderate heritability for age at first calving.
HIGHLIGHTS
Introduction
The Limousine and Charolais breeds have been worldwide exported for production purposes in pure or crossbred systems (Bouquet et al. Citation2011). Characteristics such as robust profile, high carcase growth, muscular performance, good feed conversion rate and extreme calving ease (Giorgetti et al. Citation1992) make these the main French beef breeds. These breeds are assuming increasing importance day by day in terms of raised animals in Italy; the Limousine breed is today the second beef breed in Italy after the Piedmontese.
The two breeds are also involved in the Italian national rural development project for the genetic improvement of cattle populations raised in Italy (I-BEEF) and, in this context, genetic indexes will be developed following a genomic approach both for classic traits such as growth, morphology and reproductive parameters, and for innovative traits such as heat resistance, and functional longevity. To activate and make functional a selective programme of this type, however, it is essential to know in detail the parameters of the populations studied with particular regard to the population structure and inbreeding.
In Italy, enrolled animals in Limousine and Charolais Herd Books sum up to 263,987 and 83,944 females, respectively ( National Association of Breeders of Charolais and Italian Limousine cattle breeds- ANACLI Citation2017). As far as we know, in Italy, there are no studies that have investigated the possible relationship between population structure parameters and genetic trend for reproductive traits of both breeds.
The reproductive efficiency of the females is a relevant factor in the economic viability of the cattle production system (Berry et al. Citation2014), mainly due to the cost of maintenance of the cows in the flock (Malhado et al. Citation2013). Among the reproductive traits, age at first calving (AFC) and calving interval (CI) play an important role in the beef cattle production system (Berry et al. Citation2014). Females calving early and regularly in life tend to produce a larger amount of calves in a smaller time (Åby et al. Citation2012), increasing the females’ reproductive life (Short et al. Citation1994) and optimising the return on investment. Indeed, it is important to evaluate the genetic parameters of those traits in order to maximise the response to selection. This can be obtained by estimating the genetic parameters as well as genetic and phenotypic trends, which allows quantifying how much of the observed annual herd gain is due to genetic, as well as to verify possible environmental effects (Vergara et al. Citation2009).
The productivity of a herd is elevated when prioritising mating of genetically superior individuals (Boligon et al. Citation2008). In contrast, this process makes most breeds to be considered as populations of limited size in relation to an unequal contribution from a limited number of breeding animals for the next generation. This situation, especially if submitted to a constant selection programme, is considered a major cause of inbreeding (Pedrosa et al. Citation2010). In these populations, theoretically, there is no introduction of animals from other herds, and those considered inappropriate for reproduction are discarded with increased frequencies of selected alleles but also reduced overall genetic variability.
The Italian Limousine and Charolais Herd books are closed, and the sole route of genetic flow depends on the use of elite foreign animals (mainly French) previously accepted by the breeders’ association. Elite animals have a positive effect on the genetic improvement of the importing country (i.e. Italy) but at the same time, they might increase the possibility of inbreeding because most of them are strictly related.
The knowledge of intra-population structure and variability using pedigree data allows the estimation of some parameters, such as inbreeding, effective population size and generation intervals. This information provides an effective basis for the appropriate management of genetic resources, taking advantage of maximum diversity in the selection and/or conservation programmes (Gutierrez et al. Citation2003), breeding strategies (Carneiro et al. Citation2007), evolution and/or history of the generations (Valera et al. Citation2005). A study at the European level has recently evaluated the pedigree of Limousine and Charolais populations in different countries (Bouquet et al. Citation2011).
However, the Italian population was not included, suggesting the need for further investigation. Herds are hypothesised to become genetically differentiated over time, since populations do not present the same demographic history and are isolated by limited gene flows. The study is therefore to be considered preparatory and indispensable for the subsequent implementation of genomic selection in Limousine and Charolais cattle breeds raised in Italy. The objective of the present study was (a) to investigate the population structure of the Limousine and Charolais cattle raised in Italy, (b) to estimate variance components and genetic trends for two reproductive traits in both breeds.
Material and methods
Data
Data come from the Italian Association of Charolais and Limousine Cattle Breeders (ANACLI) and refer to 321,286 and 96,480 Limousine and Charolais animals born between 1960 and 2017. In order to study the population structure, pedigree information was used for genetic parameters and genetic and phenotypic trends were calculated using information from animals born between 1999 and 2017. The total number of available records was 48,105 and 18,500 Limousine and 9228 and 4330 Charolais females for CI and AFC, respectively.
Variance components estimation and genetic trends
Variance components obtained by REML were used to predict the genetic values of all animals by the REMLF90 package of Misztal (Citation1997). For AFC, the model contained the direct additive and residual random effects, plus the fixed effects of herd, sex, month, and year of birth of the animal, type of calving (single or twin), and inbreeding as co-variable since each animal contained its respective value. For CI, the model contained the direct additive random effects, permanent and residual, as well as fixed effects of herd, calf sex, month, and year of birth of the previous calving, type of calving, calving order, and inbreeding as a co-variable. Inbreeding was calculated using the PEDIG software (Boichard Citation2002).
The genetic and phenotypic values were separated by year of birth of the animals so that the annual averages were calculated, and the genetic and phenotypic trends were obtained by regression of the annual averages of the predicted genetic values in relation to the year of birth of the animals, weighted by the respective frequencies (SAS Institute Citation2017).
Pedigree analysis
Parameters estimation and pedigree analysis, based on gene origin probabilities, were calculated with the ENDOG 4.8 software (Gutierrez and Goyache Citation2005). Pedigree completeness index was calculated as proposed by Maccluer et al. (Citation1983). The number of complete generations traced, the maximum number of generations traced, and the equivalent number of complete generations were calculated as proposed by Maignel et al. (Citation1996). In order to calculate the inbreeding coefficient, the algorithm proposed by Meuwissen and Luo (Citation1992) was used. The changes in inbreeding were calculated as described by Gutierrez et al. (Citation2009). The individual inbreeding coefficient and the equivalent generation for the individual were calculated as proposed by Boichard et al. (Citation1997). The expression relating inbreeding in generation t with inbreeding rate was calculated as proposed by Gutierrez et al. (Citation2009). The average relatedness coefficient of each individual was calculated as proposed by Gutierrez and Goyache (Citation2005). The generation interval was calculated as proposed by James (Citation1977). The effective size was calculated as proposed by Hill (Citation1979). The effective number of founders and ancestors was calculated as proposed by Boichard et al. (Citation1997).
Results
Variance components estimation and genetic trends
The means and standard deviations in days for AFC and CI were 1191.19 ± 307.10 and 438.09 ± 18.50 in the Charolais breed and 1099.26 ± 232.15 and 425.18 ± 14.42 in Limousine breed, respectively. Variance component estimates presented similar values between the breeds (Table ), with moderate heritabilities for AFC and low for CI.
Table 1. Estimates of genetic parameters for reproductive traits in Limousine and Charolais cattle raised in Italy.
The phenotypic trends for AFC were significant (p < .001) in both breeds. It was possible to observe a reduction in the last years for Charolais and Limousine (Figure ), with great variations between the years, which did not allow a high coefficient of determination (R2). The most adequate function was quadratic, since it is noted that up to the year 2006 events caused worsening in the phenotypic values, and later adjustments allowed again phenotypic gains. Phenotypic trends for CI (Figure ) were also significant with an average reduction of 1.9 days per year in both breeds, however, in absolute terms represented a difference of 31.2 and 30.90 days less for Charolais and Limousine.
Figure 1. Phenotypic trends for age at first calving (A) and calving intervals (B) in Limousine and Charolais cattle raised in Italy.
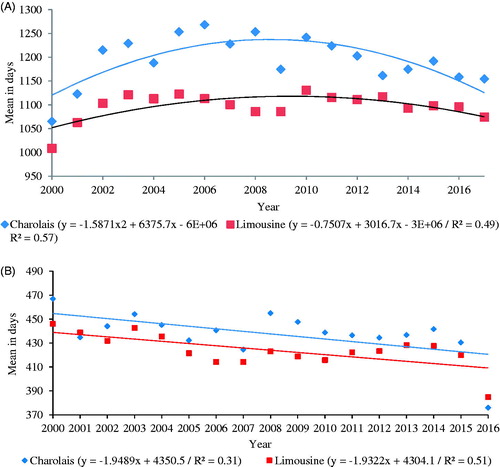
The genetic trends for AFC resulted significantly (p < .001) and led to a reduction of 3.64 days/year for Charolais and 4.70 days/year for Limousine (Figure ) respectively. In both breeds, improvements were observed in the genetic trend from the year 2009. These improvements of AFC represented a total decrease of 61.90 and 79.90 days for Charolais and Limousine breeds respectively. Genetic trends of CI (Figure ), for both breeds, were significant (p < .001), but with large variations over the years, as confirmed by the moderate (Charolais) and low (Limousine) magnitude of R2. In general, reduction of CI was low, 1.40 and 0.50 days throughout the studied period for Charolais and Limousine, respectively.
Pedigree analysis
Estimates of generation intervals for Limousine were 7.26 ± 10.13 (sire-son), 6.22 ± 6.90 (sire-daughter), 9.11 ± 12.51 (dam-son) and 7.52 ± 8.05 (dam-daughter), resulting in the average generation interval of 7.05 ± 8.14 years. Generation intervals for Charolais breed were the following: 6.30 ± 7.29 (sire-son), 5.44 ± 4.73 (sire-daughter), 7.57 ± 8.57 (dam-son) and 7.81 ± 8.18 (dam-daughter) with an average of 6.70 ± 7.01 years.
In the Charolais breed, the number of bulls and cows increased with a linear (low variation) trend (Figure ) from 1977 to 2007 and there was an increase in the effective size. Charolais showed a marked increase in effective size starting from 1991 reaching the maximum value (1000.31) in the last period (2005–2011), followed by a decline in effective size. Limousine presented similar behaviour and, in the period from 1997 to 2007, the number of bulls and cows increased markedly, as well as the effective size (Figure ). Starting from 2009 the number of bulls and cows declined whereas the effective number increased steadily since 1997. During the last interval of evaluation (2005–2011) effective number was four-fold higher than the value found in the first interval (1974–1980).
Figure 3. Number of breeders, average number of calves per breeder and effective size, at intervals of 6 years, from 1960 to 2013 for Charolais (A) and Limousine (B) cattle raised in Italy. Ne: effective size.
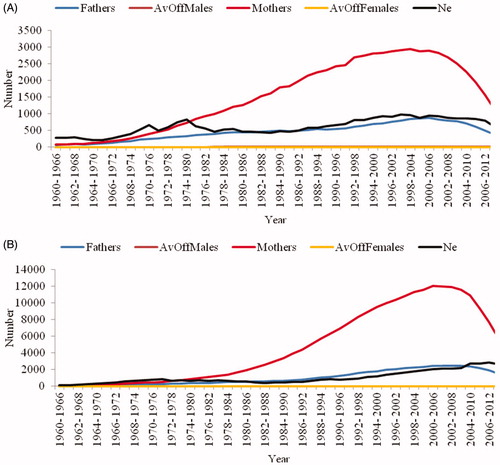
All the herds of both breeds use external bulls, and no herd has been classified as a nucleus or isolated (Table ). Herds selling bulls were 39.13% and 29.55% for Limousine and Charolais breeds, respectively, while 59.47% and 70.50% were commercial herds.
Table 2. Classification of registered herds of Limousine and Charolais cattle raised in Italy, according to the use and origin of bulls.
The pedigree completeness of Limousine breed was moderate, in the first three generations, where values of 84.14, 68.72 and 60.11 percent were obtained (Figure ), whereas for Charolais breeds the values of 77.27, 59.70 and 51.03 percent for the first, second and third generation were recorded. It should be noted that Limousine values are slightly better than Charolais.
Figure 4. Percentage of ancestors known in the first ten generations in the herd of Limousine and Charolais.
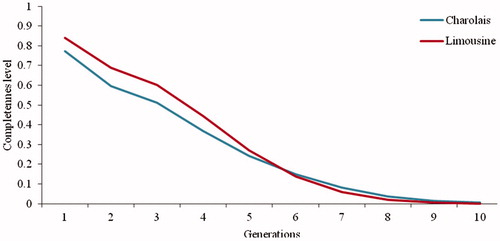
The effective number of founders (fe) and ancestors (fa) were 1079 and 513 (fe/fa = 2.10) for Limousine and 1310 and 470 (fe/fa = 2.78) for Charolais, with the fe/fa ratio always higher than 2.00 for both breeds, indicating a low balance between the founders’ and ancestors’ contributions and strong presence of the ‘bottleneck effect’. Three hundred ancestors are needed to account for almost 50% of genetic variability in the Limousine population whereas only 213 in Charolais breed. Other population parameters are shown in Table .
Table 3. Population parameters of Limousine and Charolais cattle raised in Italy.
The low average number of generations is related to the low number of known ancestors with a more precise estimation of the inbreeding with deeper pedigrees (Table ). The average number of traced generations was directly proportional to effective number whereas inversely to inbreeding for the three-generation types considered (complete, equivalent, and maximum). Results for equivalent generation types are positioned in the middle for all the parameters confirming that a shallow pedigree tends to both underestimate inbreeding and overestimate the effective size.
Table 4. Average number of traced generations (ANTG), inbreeding increase (ΔF) and effective size (Ne) by generation type in Charolais and Limousine cattle.
The coefficient of inbreeding for the maximum generations increased through the generations, going from 0.06% and 0.09%, in the second, to 3.70% and 7.56% in the fifth, for Limousine and Charolais breeds respectively (Table ). Considering the average inbreeding of individuals from the mating between relatives, a peak was observed in the first generation, reducing gradually until the fourth generation, with a large increase in the following generation. The average relationship coefficient increased from first to the fifth generation for both breeds. The absence of Ne for Limousine at generation 3 is due to the calculation performed by the Endog software, which estimates Ne from the increase in the average inbreeding coefficient between two subsequent generations. In this case, an inbreeding reduction was observed and because of that, the software did not provide a value.
Table 5. Average inbreeding coefficient (F%), percentage of inbred individuals (Inb%), mean F for inbred animals (FInb%), mean ratio coefficient (AR%) and effective size (Ne).
Among the ten most important ancestors (founders or not), with a contribution to genetic variability in the reference population, there were no females for Charolais and only one for Limousine. In Limousine, the most important animal contributed to 1.78% of the genetic variability, leaving 323 descendants throughout the generations, and in general, the ten main ancestors of the population contributed to 9.08% of the genetic variability and they left 1885 descendants throughout the generations. For Charolais, the most important animal contributed to 1.40% of the genetic variability, leaving 50 descendants throughout the generations, and in general, the ten main ancestors of the population, contributed to 8.87% of the genetic variability and they left 1211 descendants throughout the generations.
Discussion
Considering the heritability observed for AFC, the possibility of genetic gain through selection is verified. As a strategy it is suggested to precociously breed the females, which helps to identify bulls that produce precocious daughters during the selective process; this allows some animals to express their genetic potential for sexual precocity. The management and selection that have been practiced in the last years provided improvements to this trait in Charolais breed. The limited expression of the genetic potential in Limousine breed as shown by the phenotypic trend may be related to several management factors or lack of selection (did not have EBV for the reproductive trait).
For CI, the great influence of both non-additive and environmental effects indicates that the variation of this trait depends more on the appropriate management conditions pointing out that there is a greater difficulty of direct selection. For CI, the almost null trend of genetic origin can be partially explained by the small genetic variability or to the absence of selection for this trait; however, as showed by the phenotypic trend, environmental improvements allowed a reduction of ≈ 33 days of CI for Charolais and Limousine breeds, respectively. This indicates that Limousine breeders had directly or indirectly larger control under environmental management. In general, it should be noted that, although the genetic gains obtained for AFC and CI characteristics present medium and low values, respectively, the genetic trend should be considered, since genetic changes are stable, cumulative and permanent over the years.
Genetic values for AFC constantly decrease from 2000 to 2016 in both breeds, with a reduction of 56 days for the Charolais breed. For the CI, there were peaks in different periods, highlighting 2005 (1.06 days less), 2015 (0.98 days less) and 2016 (1.59 days less). The Limousine breed presented a constant increase in the genetic value of the animals for the AFC since 2012 (18.55 days less) with at present (2017) the greatest reduction (45.23 days less). In contrast, the genetic gain for CI in the Limousine breed was low, with no easily identifiable trend.
Genetic gain and the effective number throughout the years increased similarly in the Charolais breed for both AFC and CI. It can be assumed that the size effect of Charolais tended to reduce after the peak observed between 2005 and 2011, due to the reduced use of foreign bulls starting from this period. Italian breeders owned enough local animals necessary to establish a selective process without resorting to foreign genetics.
For Limousine, the effective size continued to increase from 1997, with slight oscillations between 2003 and 2009. Also, the AFC genetic gain was continuous, with a peak in 2017 that is almost twenty-two times the value of 2007, where the increase of the average genetic value provided the first significant results. Due to great instability and low gain of CI, no conclusive explanation can be drawn. It should be noted that for Limousine, the low increase in effective size between 1994 and 1997 can be explained by the excessive use of some bulls and may be reinforced by the relationship between bull and offspring.
The continuous increase in the effective size of the Limousine breed from 1997 can be explained by the diffusion of the breed in Italy, considered as the most expressive in herd destined to beef cattle. The herdbook was officially started in 1999 whereas the first EBVs for Italian bulls (only Limousine) were available a few years later and they were related to productive characteristics (weights). According to data available at ANACLI (Citation2017), the number of Limousine herds increased from 337 in 2005 to 1685 in 2016, which is 12% more compared than the increase observed for Charolais in the same period; in total, Limousine breed has 3 times more herds than Charolais breed.
Another factor to be highlighted is the optimisation of the generation interval for both breeds since large intervals decrease the annual genetic gain in relation to the characteristics selected, which leads to a lower economic return of the breeding programme. In order to increase genetic gains, it is fundamental to reduce generation interval, to increase effective size, to use breeding herds, and to control mating of related animals (Malhado et al. Citation2008). Additionally, it is reported that prolonged use of breeding animals allows animals of advanced ages to be harnessed for long periods. This process is demonstrated in all the gametic passages, mainly in mother to son and mother to daughter which obtained the highest values. Caution is suggested when considering reproductive longevity in the production system because when there is no careful mating strategy, there is a risk of cross-breeding between animals of the same family, which may lead to an inbreeding increase, or decrease in Ne, and consequently in the genetic gain (Rezende et al. Citation2017).
It was verified that the increase of known generations allows more accurate estimations on the population structure, fundamental for procedures of genetic improvement of both breeds, and to improve the reliability in the population parameters, as also stated by Carneiro et al. (Citation2007). The extension of the genetic ‘bottleneck effect’ expressed by the fe/fa ratio was present in both breeds, with risk from this point of view, since the ideal is that the number of effective animals is as close as possible to the size of the founding population (Vozzi et al. Citation2006).
Charolais still has a great influence on the exchange and/or trade of animals from other countries, while Limousine already has a large distribution of herds in Italy. This variability is due to gene flow and not to a few founders since the relation fe/fa indicates the presence of the bottleneck effect and the participation of the ancestors is smaller, which results in the reduction of variability and, consequently, increased endogamy. This was observed over the generations in which Ne had a reduction in both breeds. The values of the average relationship were also increasing throughout the generations, evidencing that some animals were more intensely used.
It is known that the genetic variability in a given population is fundamental to increase the short and long-term return of selection, as well as for conservation purposes; indeed, both breeds use external bulls, which is adequate for gene flow among the herds, because it makes possible to avoid subdivision, which may aggravate the problems of conservation of genetic groups (Rezende et al. Citation2017). The purpose of both breeds is focussed on commercial herds and proper use of inbreeding by producers can ensure breed uniformity and/or trait fixation in lineages whose products are most commercially accepted .
In both breeds, the average value of complete generations traced indicates that only a small number of ancestors is known justifying the greatest differences of the inbreeding increase with the three types of generations studied; however, the greatest number of ancestors can be observed for maximum generation method, reflecting a smaller increase in inbreeding and a larger effective size. It is essential to make estimates of the average effective size, estimated with different types of generations since they are useful to indicate the upper limit (complete traced generations), lower limit (maximum traced generations) and the real limit (traced equivalent generations) in populations where genealogical information is scarce (Gutierrez and Goyache Citation2005).
The gradual increase of the inbreeding coefficient as well as the percentage of inbred individuals during the generations can be explained by the increase of information available for the estimation. In this context, the more an individual’s complete generations are known, the greater the possibility of an ancestor appearing in their pedigree. The reduction of the average inbreeding may indicate the possible care in avoiding inbreeding. It should be noted that the substantial implementation of assisted reproductive technologies contributes to the increase of inbreeding (Scraggs et al. Citation2014) when not used correctly, which causes concern in cattle industry (Santana et al. Citation2016). A suggested alternative would be to control the inbreeding coefficient of offspring by choosing parents with low average kinship coefficient values, since this is defined as the probability of an allele, chosen at random, belonging to a specific animal; in other words, the representation of the animal in its pedigree (Gutiérrez and Goyache Citation2005).
Practically, it is suggested to initiate the activity of selection for reproductive characteristics with adequate control of the population parameters. This process can be successful if field technicians will be previously prepared to execute the recommendations of suitable mating. Another suggestion is the creation of more specific Sires’ EBV Summary, which could be adapted to each production system or farm; Rezende et al. (Citation2020) studying these two breeds from Italy found that the genotype-environment interaction should be considered in the selection of reproductive traits in both breeds. Anyway, it is still a not easy task to accomplish but, as already reported, all these results will be used in the implementation of the genomic selection project for both breeds in Italy. Thus, it is believed that with genomic selection it would be possible to anticipate the genetic progress of these reproductive traits, to monitor the inbreeding, and to define more appropriate strategies for the maintenance of genetic diversity. Several studies have shown that advances can be achieved in cattle production using genomic selection (Calus et al. Citation2013; Hayes et al. Citation2013; Gaddis et al. Citation2014).
Conclusions
Genetic gain for AFC is possible using selection. AFC improvements endured from the beginning of the animals’ evaluation to the present day; however, genetic gains can be optimised given the existence of moderate additive genetic variability. The gain in CI even if stable is of low magnitude. Thus, to improve this parameter, it is necessary to make changes in the farm’s management environment in order to reduce environmental effects.
The Charolais and Limousine breeds presented a reduced number of animals in the genetic formation and moderate completeness of the pedigree, which was sufficient to estimate the population parameters. The ‘bottleneck’ effect was of high magnitude in both breeds. One of the possible ways to increase the annual genetic gain could foresee the reduction of generation interval.
Ethical approval
All authors declare that this study follows the principles of the Declaration of Helsinki.
Acknowledgement
The authors thank the University of Florence (Unifi) for physical support and collaboration in project coordination. The research was funded by ANACLI through the I-BEEF (Italian Beef Environment, Efficiency and Fitness) project PSRN 2014-2020.Sottomisura 10.2: Animal Biodiversity. We acknowledge the Italian Breeders Association (AIA) and Associazione Nazionale Allevatori delle razze bovine Charolaise e Limousine (ANACLI) for providing the data.
Disclosure statement
No potential conflict of interest was reported by the author(s).
Additional information
Funding
References
- Åby BA, Aass L, Sehested E, Vangen O. 2012. Effects of changes in external production conditions on economic values of traits in Continental and British beef cattle breeds. Livest Sci. 150(1–3):80–93.
- National Association of Breeders of Charolais and Italian Limousine cattle breeds -[Associazione Nazionale Allevatori delle razze bovine Charolaise e Limousine Italiane (ANACLI)]. 2017. http://www.anacli.it/WEBSITE/index.php?&pagid=2455&sessione=
- Berry DP, Wall E, Pryce JE. 2014. Genetics and genomics of reproductive performance in dairy and beef cattle. Animal. 8(s1):105–121.
- Boichard D. 2002. Pedig: a fortran package for pedigree analysis suited for large populations. Paper presented at the 7th World Congress on Genetics Applied to Livestock Production; August 19–23; Montpellier, Fance.
- Boichard D, Maignel L, Verrier E. 1997. The value of using probabilities of gene origin to measure genetic variability in a population. Genet Sel Evol. 29(1):5–23.
- Boligon AA, Albuquerque LG, Rorato PRN. 2008. Genetic associations among weights and reproductive traits in Nellore cattle. R Bras Zootec. 37(4):596–601.
- Bouquet A, Venot E, Laloë D, Forabosco F, Fogh A, Pabiou T, Moore K, Eriksson JA, Renand G, Phocas F. 2011. Genetic structure of the European Charolais and Limousin cattle metapopulations using pedigree analyses. Anim Sci J. 89(6):1719–1730.
- Calus MPL, de Haas Y, Pszczola M, Veerkamp RF. 2013. Predicted accuracy of and response to genomic selection for new traits in dairy cattle. Animal. 7(2):183–191.
- Carneiro PLS, Malhado CHM, Euclydes RF, Carneiro APS, Cunha EE. 2007. Inbreeding, alleles fixation and selection limit in populations under traditional or marker assisted selection methods. R Bras Zootec. 36(2):369–375.
- Gaddis KLP, Cole JB, Clay JS, Maltecca C. 2014. Genomic selection for producer-recorded health event data in US dairy cattle. J Dairy Sci. 97(5):3190–3199.
- Giorgetti A, Lucifero M, Acciaioli A, Sargentini C, Martini A. 1992. Caratteristiche produttive di vitelloni Chianini, Charolais e Limousins. Rilievi Alla Sezionatura Commerciale. Zootec Nutr Anim. 18:84–85.
- Gutierrez JP, Altarriba J, Diaz C, Quintanilla R, Canon J, Piedrafita J. 2003. Pedigree analysis of eight Spanish beef cattle breeds. Genet Sel Evol. 35(1):43–63.
- Gutierrez JP, Goyache F. 2005. A note on ENDOG: a computer program for analysing pedigree information. J Anim Breed Genet. 122(3):172–176.
- Gutierrez JP, Cervantes I, Goyache F. 2009. Improving the estimation of realized effective population sizes in farm animals. J Anim Breed Genet. 126(4):327–332.
- Hayes BJ, Lewin HA, Goddard ME. 2013. The future of livestock breeding: genomic selection for efficiency, reduced emissions intensity, and adaptation. Trends Genet. 29(4):206–214.
- Hill WG. 1979. A note on effective population size with overlapping generations. Genetics. 92(1):317–322.
- James JW. 1977. A note on selection differentials and generation length when generations overlap. Anim Sci. 24(1):109–112.
- Maccluer J, Boyce AJ, Dyke B, Weitkamp LR, Pfenning QW, Parsons CJ. 1983. Inbreeding and pedigree structure in Standardbred horses. J Hered. 74(6):394–399.
- Maignel L, Boichard D, Verrier E. 1996. Genetic variability of French dairy breeds estimated from pedigree information. Inter Bull. 14:49–54.
- Malhado CHM, Carneiro PLS, Pereira DG, Martins Filho R. 2008. Progresso genético e estrutura populacional do rebanho Nelore no Estado da Bahia. Pesq Agropec Bras. 43(9):1163–1169.
- Malhado CHM, Malhado ACM, Martins Filho R, Carneiro PLS, Pala A, Carrillo JA. 2013. Age at first calving of Nellore cattle in the semi-arid region of northeastern Brazil using linear, threshold, censored and penalty models. Livest Sci. 154(1–3):28–33.
- Meuwissen TI, Luo Z. 1992. Computing inbreeding coefficients in large populations. Genet Sel Evol. 24(4):305–313.
- Misztal I. 1997. Estimation of variance components with large-scale dominance models. J Dairy Sci. 80(5):965–974.
- Pedrosa VB, Santana ML, Oliveira PS, Eler JP, Ferraz JBS. 2010. Population structure and inbreeding effects on growth traits of Santa Inês sheep in Brazil. Small Ruminant Res. 93(2–3):135–139.
- Rezende MPG, Conde E, Borges AC, Carneiro PLS, Martins Filho R, Malhado CHM. 2017. Population structure of the Nellore herd reared in the Brasilian Northeast Semi-arid. Ciênc Anim Bras. 18:1–14.
- Rezende MPG, Malhado CHM, Biffani S, Carneiro PLS, Carrillo JA, Bozzi R. 2020. Genotype-environment interaction for age at first calving in Limousine and Charolais cattle raised in Italy, employing reaction norm model. Livest Sci. 232:103912.
- Santana ML, Pereira RJ, Bignardi AB, Ayres DR, Menezes GRO, Silva LOC, Leroy G, Machado CHC, Josahkian LA, Albuquerque LG. 2016. Structure and genetic diversity of Brazilian Zebu cattle breeds assessed by pedigree analysis. Livest Sci. 187:6–15.
- SAS Institute. 2017. Statistical analysis system: user’s guide. Cary (NC): SAS.
- Scraggs E, Zanella R, Wojtowicz A, Taylor JF, Gaskins CT, Reeves JJ, Avila JM, Neibergs HL. 2014. Estimation of inbreeding and effective population size of full-blood Wagyu cattle registered with the American Wagyu Cattle Association. J Anim Breed Genet. 131(1):3–10.
- Short RE, Staigmiller RB, Bellows RA, Greer RC. 1994. Breeding heifers at one year of age: biological and economic considerations. In Fields MJ, Sand RS, Yelich JV, editors. Factors affecting calf crop. London (UK): CRC Press; p. 55–68.
- Valera M, Molina A, Gutiérrez JP, Gómez J, Goyache F. 2005. Pedigree analysis in the Andalusian horse: population structure, genetic variability and influence of the Carthusian strain. Livest Prod Sci. 95(1–2):57–66.
- Vergara OD, Elzo MA, Cerón-Muñoz MF. 2009. Genetic parameters and genetic trends for age at first calving and calving interval in an Angus-Blanco Orejinegro Zebu multibreed cattle population in Colombia. Livest Sci. 126(1–3):318–322.
- Vozzi PA, Marcondes CR, Magnabosco CU, Bezerra LAF, Lobo RB. 2006. Structure and genetic variability in Nellore (Bos indicus) cattle by pedigree analysis. Genet Mol Biol. 29(3):482–485.